Abstract
Context
5-Caffeoylquinic acid (5-CQA) is one of the most abundant compounds found in natural foods including coffee.
Objective
We investigated whether 5-CQA had a cytoprotective effect through the NF-E2-related factor 2 (Nrf2)-antioxidant response element (ARE) signalling pathway.
Materials and methods
Nrf2 activation in response to 5-CQA treatment at the concentration of 10–100 μM is evaluated by Western blotting of Nrf2 and ARE reporter gene assay as well as its target gene expression in HepG2 cells. Intracellular reactive oxygen species (ROS) and glutathione (GSH) levels were measured in the tert-butyl hydroperoxide-induced hepatocytes to examined cytoprotective effect of 5-CQA (10–100 μM). The specific role of 5-CQA on Nrf2 activation was examined using Nrf2 knockout cells or Nrf2 specific inhibitor, ML-385.
Results
Nuclear translocation of Nrf2 is increased by 5-CQA in HepG2 cells which peaked at 6 h. Consequently, 5-CQA significantly increases the ARE reporter gene activity and downstream antioxidant proteins, including glutamate cysteine ligase (GCL), hemeoxygenase-1 (HO-1), NAD(P)H quinone oxidoreductase 1, and Sestrin2. Nrf2 deficiency or inhibition completely antagonized ability of 5-CQA to induce HO-1 and GCL expression. Cells pre-treated with 5-CQA were rescued from tert-butyl hydroperoxide-induced ROS production and GSH depletion. Nrf2 activation by 5-CQA was due to increased phosphorylation of MAPKs, AMPK and PKCδ.
Discussion and conclusions
Taken together, our results demonstrate that as a novel Nrf2 activator, 5-CQA, may be a promising candidate against oxidative stress-mediated liver injury. Additional efforts are needed to assess 5-CQA, as a potential therapeutic in liver diseases in vivo and in humans.
Introduction
Oxidative stress is thought to contribute to the development of a wide range of diseases, including heart disease, cancer, chronic inflammation, and osteoarthritis (Liou and Storz Citation2010; Liguori et al. Citation2018). Excessive reactive oxygen species (ROS) can overwhelm the antioxidative scavenging capacity within a cell and cause oxidative damage to DNA, lipids, and proteins, as well as concomitant cellular damage. The antioxidant defense system protects the cells from substantial oxidative stress-induced damage mainly by stimulating the expression of phase II detoxification enzymes.
NF-E2-related factor 2 (Nrf2) is a basic leucine zipper (bZIP) protein that regulates the expression of antioxidative proteins which protect against oxidative stress triggered by injury and inflammation. Numerous studies have demonstrated that Nrf2 is a master regulator of the antioxidative defense system because it regulates the expression of several detoxification or antioxidant enzymes. Nrf2 is controlled by dimeric Kelch-like ECH-associated protein 1 (Keap1), a substrate adaptor protein for Cullin3/RING-box protein 1 ubiquitin ligase, which normally targets Nrf2 for ubiquitination and degradation, but loses this ability in response to electrophiles and oxidants (Baird et al. Citation2013). It has been well established that cells and tissues are protected by the activation of Nrf2 in response to oxidative injury. As a master regulator of the antioxidative system, Nrf2 upregulates a diversity of detoxifying and antioxidant phase II enzymes, such as hemeoxygenase 1 (HO-1) (Kweon et al. Citation2006), glutathione S-transferase A1/2 (Hayes et al. Citation2000), glutamate-cysteine ligase (GCL) (Bea et al. Citation2003), NAD(P)H: quinone oxidoreductase 1 (NQO1) (McMahon et al. Citation2001), and sestrin2 (Sesn2) (Shin et al. Citation2012). Following a direct attack by ROS or resulting indirect actions, such as phosphorylation, Nrf2 dissociates from Keap1 and thereby translocates into the nucleus and activates its target genes via the antioxidant response element (ARE) (Suzuki and Yamamoto Citation2015). A number of reports have addressed the possible roles played by several upstream kinases, including extracellular signal-regulated kinase (ERK1/2) (Zipper and Mulcahy Citation2003), protein kinase C delta (PKCδ) (Huang et al. Citation2002), phosphoinositide 3-kinase (PI3K) (Kang et al. Citation2002), and 5′-AMP-activated protein kinase (AMPK) (Joo et al. Citation2016) in Nrf2 activation.
Chlorogenic acids (CGAs), an abundant group of plant polyphenols, are esters formed between caffeic acid and quinic acids. 5-Caffeoylquinic acid (5-CQA) is one of the major phenolic acids in the CGA group (Liang and Kitts Citation2015). Within this family, 5-CQA has been reported to exhibit a broad spectrum of pharmacological properties and applications, including anti-inflammatory, antioxidative (Rocha et al. Citation2012), anticancer (In et al. Citation2016), and neuroprotective effects (Han and Isoda Citation2011). Although antioxidative activities of 5-CQA have been reported, little is known about Nrf2 activation and its molecular mechanism in hepatocytes.
This study investigated whether 5-CQA has the capability to activate Nrf2 and to induce its downstream target genes in hepatocytes. Here, we found that 5-CQA exhibits antioxidative activities which were mediated via decreased ROS levels and increased antioxidant gene expression. Our current results suggested that 5-CQA acts as an essential regulator of the Nrf2 activity, thereby protecting hepatocytes from oxidative damage.
Materials and methods
Material
5-CQA (purity > 98%) was provided by Fei Yu Chem (Nanjing, China) (). Antibodies against Nrf2, phospho-Nrf2, and PARP were obtained from Santa Cruz Biotechnology (Santa Cruz, CA, USA). Phospho-ERK 1/2, ERK 1/2, phospho-p38, lamin A/C, caspase-3, phospho-PKCδ, phospho-AMPK, and AMPK antibodies were bought from Cell Signalling (Danvers, MA, USA). Sesn2 antibody was obtained from Proteintec (Chicago, IL, USA) and GCL antibody from Abcam (Cambridge, MA, USA). HO-1 antibody was provided by Enzo Life Sciences (Plymouth Meeting, PA, USA) and PD98059 (PD), Rottlerin (ROTT), SP600125 (SP), SB203580 (SB), Compound C (Comp. C), MTT, metaphosphoric acid, DCFH-DA, tert-butylhydroperoxide (t-BHP), dimethyl sulfoxide (DMSO), and β-actin antibody were purchased from Sigma Chemicals (St. Louis, MO, USA). ML-385 (Nrf2 inhibitor) was provided by MCE (Monmouth Junction, NJ, USA).
Figure 1. Effect of 5-CQA on cytotoxicity effect. (A) Chemical structure of 5-CQA. (B) MTT assays for cell viability. The effect of 5-CQA (0–200 μM, 24 h treatment) on cell viability was assessed using MTT assays. (C) Time course of nuclear translocation of Nrf2 by 5-CQA in HepG2 cells. Western blot was performed using nuclear fractions of cells incubated in the presence of 100 μM 5-CQA for 0–6 h. (D) Effect of varying concentrations of 5-CQA on Nrf2 nuclear translocation in HepG2 cells (6 h). (E) Increases in Nrf2 transactivation by 5-CQA. NQO1-ARE luciferase activity was measured in lysates of HepG2 cells stably transfected with NQO1-ARE luciferase construct exposed to 10–100 μM 5-CQA for 12 h. Data represent the mean ± SE of three replicates, with statistically significant differences between each treatment group and the control defined by *p < 0.05 or **p < 0.01.
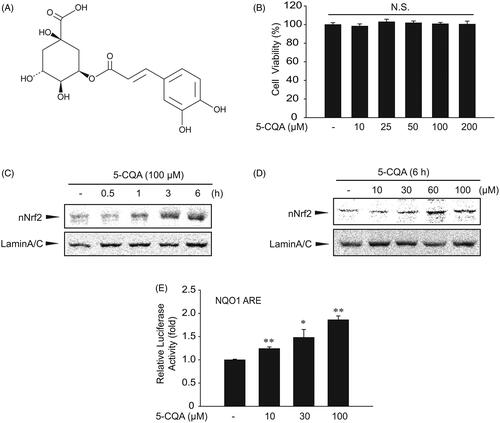
Cell culture
HepG2 cells were purchased from the American Type Culture Collection (ATCC) (Manassas, VA, USA). Nrf2 knockout (KO) and wild-type (WT) MEF cells were kindly donated by Dr. Rong Cai (Shanghai Jiaotong University, China). Cells were plated at a density of 1 × 105 per well in six-well plates. Cells were maintained in Dulbecco’s Modified Eagle Medium (DMEM) containing 10% fetal bovine serum, 50 units/mL of penicillin, and 50 μg/mL streptomycin at 37 °C in a humidified 5% CO2 atmosphere. 5-CQA dissolved in DMSO was added to cells and incubated at 37 °C for the indicated time period. Cells were then washed twice with ice-cold phosphate-buffered saline before sample preparation.
MTT cell viability assay
To measure cytotoxicity, HepG2 cells were plated at a density of 1 × 105 cells/well in 24-well plates and treated with t-BHP in the presence or absence of 5-CQA (10–100 μM, 1 h pre-treatment). After treatment, viable cells were stained with MTT (0.2 mg/mL, 4 h). The media were removed and then formazan crystals produced in the wells were dissolved with the addition of 200 μL of DMSO. Absorbance at 540 nm was measured using an enzyme-linked immunosorbent assay microplate reader (SpectraMAX, Molecular Device, Sunnyvale, CA).
Measurement of ROS production
Cells grown in 12-well plates were treated with 10 μM DCFH-DA and maintained at 37 °C, after which they were harvested by trypsinization. The collected cells were then washed twice with a wash buffer and H2O2 production was determined using a fluorescence-detecting microplate reader (Infinite F200, TECAN, CH) with excitation/emission wavelengths of 485/530 nm. ROS production was normalized to the protein concentration of each treated sample and defined as relative to the vehicle-treated control.
Western blot analysis
Protein extraction and subcellular fractionation, SDS-polyacrylamide gel electrophoresis, and western blot analyses were performed as previously described (Yang et al. Citation2019). Briefly, samples were separated by gel electrophoresis and electrophoretically transferred to the nitrocellulose paper. The nitrocellulose paper was first incubated with the indicated primary antibody and then with the horseradish peroxidase-conjugated secondary antibody. Immunoreactive proteins were visualized by ECL chemiluminescence (Amersham Biosciences, Buckinghamshire, UK). Equal amounts of loaded protein were verified using β-actin.
Luciferase assay
To demonstrate the ARE-luciferase activity, a HepG2 cell line stably transfected with NQO1-ARE firefly luciferase construct was established, which contained three tandem repeats of the ARE in the 5′-upstream region of NQO1 as previously reported (Cho et al. Citation2019). Firefly luciferase activity was measured using a luminometer (Promega, Madison, WI, USA) following the addition of Luciferase Assay Reagent II (Promega), according to the manufacturer’s instructions.
Determination of GSH content in cells
The GSH contents in the cells were quantified using a commercial GSH determination kit (Cayman Chemical, Ann Arbour, MI, USA) as previously described (Han et al. Citation2015). Briefly, HepG2 cells were plated in 6-well plates (1 × 106 cells/well) and treated with 30–100 μM 5-CQA for 1 h. Cells where then either incubated in 500 μM t-BHP for 12 h or detached from the dishes. The detached cells were lysed in a buffer containing 5% metaphosphoric acid to precipitate the proteins. After centrifugation at 10,000 g for 10 min, the supernatants were used to measure the GSH concentrations according to the manufacturer’s manual. Absorbance at 410 nm was measured using a microplate reader.
Statistical analysis
One-way analysis of variance (ANOVA) was used to assess the statistical significance of differences among the treatment groups. The Newman–Keul test was used to determine the significance of differences between the means of multiple groups. Results are expressed as means ± standard error (SE).
Results
Nrf2 activation by 5-CQA
Since 5-CQA had no effect on the viability of HepG2 cells at concentrations up to 200 μM in the MTT assays (), concentrations ranging from 10 to 100 μM were used for further experiments. To examine the effect of 5-CQA on Nrf2 activation, HepG2 cells were first treated with 100 μM 5-CQA for 0–6 h and its effect on nuclear accumulation of Nrf2 was investigated. Nuclear Nrf2 levels were increased and the expression levels peaked after 6 h of the 5-CQA treatment (). Next, HepG2 cells were treated with 5-CQA at different concentrations for 6 h on the nuclear accumulation of Nrf2 and 5-CQA increased nuclear Nrf2 levels (). Reporter gene assay was then performed using an ARE luciferase plasmid as a reporter to verify 5-CQA-induced Nrf2 transactivation. NQO1-ARE luciferase constructs that contained three tandem repeats of ARE in the 5′-upstream region of NQO1 were stable transfected into HepG2 cells to examine transactivation by 5-CQA. Exposure of the transfected cells to 5-CQA resulted in a significant increase in luciferase activity of the NQO1-ARE reporter construct ().
Nrf2 target gene induction by 5-CQA
To explore whether Nrf2 accumulation in the nucleus leads to the expression of its target gene, protein levels of HO-1, GCL, NQO-1, and Sesn2 were examined. As a rate-limiting enzyme of glutathione biosynthesis, GCL expression is mainly regulated by the Nrf2-ARE pathway (Wild et al. Citation1998). GCL plays a critical role in maintaining GSH homeostasis and its expression level is usually proportional to GSH concentration. HO-1 and NQO-1 are also well-known target genes of Nrf2 and have been shown to protect cells from oxidative stress-associated with free iron (Willis et al. Citation1996; Kim et al. Citation2012). We have previously reported that the novel antioxidant protein Sesn2 contains a functional ARE site in its promoter region and that the expression of Sesn2 is regulated by Nrf2 activation (Shin et al. Citation2012). Consistent with this observation, 5-CQA increased the expression of GCL, HO-1, NQO-1, and Sesn2 in a time-dependent manner ().
Figure 2. Effect of 5-CQA on expression of Nrf2 target genes. (A) Time course of Nrf2 target gene expression by 5-CQA. HepG2 cells were treated with 100 μM 5-CQA for 0 to 12 h. Glutamate-cysteine ligase (GCL), hemeoxygenase 1 (HO-1), NAD(P)H: quinone oxidoreductase 1 (NQO1) and Sestrin2 (Sesn2) were immunoblotted from the lysates of cells. (B) Effect of 5-CQA on tert-butyl hydroperoxide (t-BHP)-induced ROS production. HepG2 cells were treated with 500 μM t-BHP and/or 0–100 μM 5-CQA. Cells were stained with 10 μM DCFH-DA for 30 min at 37 °C. (C) Measurement of intracellular GSH. GSH was analysed in lysates of cells treated with 500 μM t-BHP and/or 0–100 μM 5-CQA. Data represent the mean ± SE of four replicates, where statistically significant differences between each treatment group and the control were defined by **p < 0.01 for vehicle-treated control and #p < 0.05 or ##p < 0.01 for t-BHP alone.
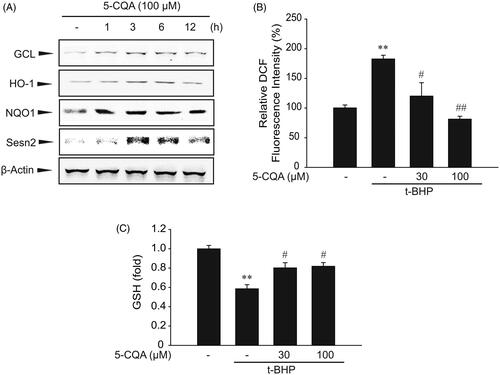
t-BHP is a compound that induces oxidative stress, which may cause a significant increase in ROS formation. It is widely accepted that t-BHP forms a more stable radical species than H2O2 without damaging DNA (Slamenova et al. Citation2013). Therefore, we examined whether t-BHP-induced ROS production was blocked by 5-CQA. As expected, 5-CQA pre-treatment almost completely inhibited ROS generation by t-BHP. Suppression of ROS production by 5-CQA suggests that 5-CQA has antioxidant property against t-BHP-induced oxidative stress (). In addition, t-BHP treatment markedly decreased the GSH level, which was maintained in cells exposed to both t-BHP and 5-CQA ().
Inhibition of t-BHP-induced cell death by 5-CQA
Next, we investigated whether antioxidative effect of 5-CQA results in cytoprotection. Treatment with t-BHP into HepG2 cells led to cell death, whereas pre-treatment with 5-CQA completely prevented it (). And then we examined whether 5-CQA can attenuate the apoptotic sequelae against t-BHP treatment. Cell lysates were used for the apoptosis marker proteins PARP and caspase-3. Compared to the control group, t-BHP exposure decreased the precursor forms of PARP and caspase-3 in cellular lysates, which were inhibited by 5-CQA treatment ().
Figure 3. Cytoprotective effect of 5-CQA. (A) MTT assay. The effect of 5-CQA (0–100 μM, 1 h pre-treatment) in the presence or absence of t-BHP (500 μM, 6 h or12 h) on cell viability was assessed using MTT assays. Data represent the mean ± SE of four replicates, where statistically significant differences between each treatment group and the control were defined by **p < 0.01 for vehicle-treated control and #p < 0.05 or ##p < 0.01 for t-BHP alone. (B) Western blot analysis of apoptosis-associated proteins. Cells were incubated with 10–30 μM 5-CQA for 1 h and then treated with 500 μM t-BHP for 12 h. Precursor PARP, precursor caspase-3 and cleaved caspase-3 were immunoblotted. Equal amounts of loaded protein were confirmed by β-actin. Results were confirmed in three separate experiments.
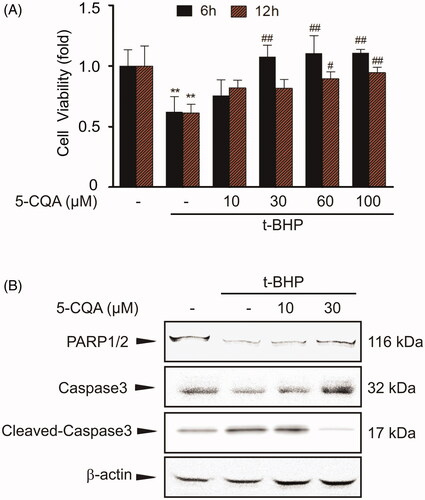
Nrf2 phosphorylation by 5-CQA
After phosphorylation, Nrf2 dissociates from Keap1 and translocates into the nucleus, and therein transactivates its target genes through ARE (Niture et al. Citation2010). It was found that 5-CQA markedly increased the Nrf2 phosphorylation between 3 and 6 h (). To probe the underlying molecular basis for Nrf2 phosphorylation by 5-CQA, upstream kinases were examined in an effort to identify the signalling pathway responsible for Nrf2 activation. 5-CQA significantly increased the phosphorylation of ERK1/2, p38, AMPK and PKCδ (). Furthermore, chemical inhibitors of p38 (SB), JNK (SP), AMPK (Comp C) and PKCδ (Rott) inhibited the GCL and HO-1 induction via 5-CQA (). Since no differences were present in the inhibitor of ERK (PD), it was hypothesized that there are other ways to compensate for the ERK pathway. These results suggest that 5-CQA activates Nrf2 and protects hepatocytes from oxidant-induced injury by activating p38, AMPK and PKCδ.
Figure 4. Activation of p38, AMPK and PKCδ by 5-CQA. (A) Western blot analysis for Nrf2 phosphorylation by 5-CQA. Cells were treated with 100 μM 5-CQA for 1 to 12 h. Total and phosphorylated Nrf2 was determined by western blotting. (B) Effects of 5-CQA on phosphorylation of Nrf2 upstream kinases. Western blot analyses for ERK1/2, p38, AMPK and PKCδ phosphorylation were performed on cell lysates incubated with 100 μM 5-CQA for 1 to 12 h. (C, D) Inhibition of 5-CQA induced GCL and HO-1 expression by chemical inhibitors. Cells were concomitantly treated with 100 μM 5-CQA and 10 μM PD98059 (PD), 10 μM SB203580 (SB), 10 μM SP600125 (SP), 5 μM Compound C (Comp C) or 2.5 μM Rottlerin (Rott) for 12 h. Results were confirmed in three replicate experiments.
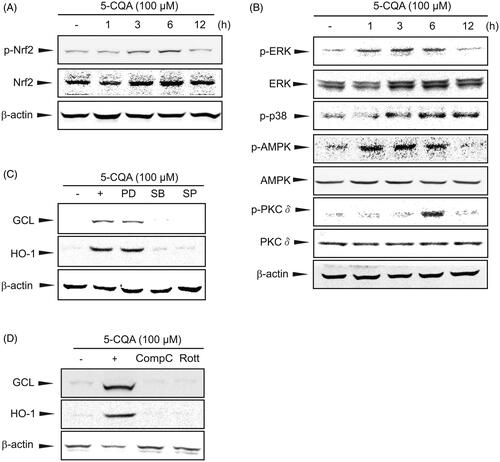
Specific role of Nrf2 in 5-CQA-mediated cytoprotective effect
To explore the role of Nrf2 activation in 5-CQA cytoprotective effect, Nrf2 inhibitor or Nrf2 Knockout (KO) MEF cells were adopted. Recently ML-385 was reported to bind to Neh1, the Cap'n'Collar basic leucine zipper (CNC-bZIP) domain of Nrf2 that specifically and selectively inhibits the Nrf2 function (Singh et al. Citation2016). When GCL and HO-1 expression levels were increased by 5-CQA, Nrf2 inhibitor ML-385 blocked the 5-CQA-induced GCL and HO-1 expression (). To confirm whether Nrf2 activation is required for the 5-CQA effect, we adopted wild type (WT) and Nrf2-KO MEF cells. First, western blot analysis showed that 5-CQA can increase the GCL and HO-1 expression levels in WT cells. However, 5-CQA-induced downstream target gene expression was completely abolished in Nrf2 KO MEF cells (). Next, MTT assay was carried out on cells treated with t-BHP in WT or Nrf2-KO MEF cells. The MTT assay result showed that Nrf2-KO significantly aggravated cell death in response to t-BHP treatment (). These observations suggest that the cytoprotective effect of 5-CQA requires activation of Nrf2.
Figure 5. Specific role of Nrf2 on cytoprotective effect of 5-CQA. (A) Inhibition of 5-CQA-induced GCL and HO-1 expression by Nrf2 inhibitor. Cells were concomitantly treated with 100 μM 5-CQA or 5 μM ML-385. (B) Effect of 5-CQA on GCL and HO-1 induction in wild type (WT) or Nrf2-knockout (KO) MEF cells. Western blot assay was performed on lysates of WT or Nrf2-KO MEF cells treated with 5-CQA for 12 h. (C) The effect of 5-CQA (10–100 μM, 1 h pre-treatment) in the presence or absence of t-BHP (500 μM, 12 h) on cell viability was assessed using MTT assays in WT or Nrf2-KO MEF cells. Data represent the mean ± SE of four replicates; **p < 0.01, significant versus vehicle-treated control; ##p < 0.01, significant versus t-BHP alone.
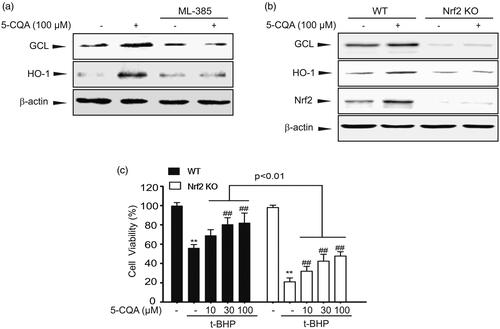
Discussion
The most exciting recent finding concerning the oxidative stress response has been the clarification of the signalling pathway by which such responses are controlled. Nrf2 is a major transcription factor of the resolution for oxidative stress, which regulates expression a diversity of antioxidant enzymes. Along with its partners, interacting proteins, and regulatory molecules, the Nrf2 signalling pathway has revealed as the most significant cellular defense and survival pathway against oxidative injury and toxicants (Zhang et al. Citation2015). Therefore, Nrf2 is regarded as a promising therapeutic target for the prevention and treatment of oxidative stress-mediated liver diseases (Shin et al. Citation2013).
Studies have found that the equilibrium of oxidant/antioxidant is maintained in a dynamic way through regulation of antioxidant levels in response to oxidative stress. Expression of antioxidant enzymes GCL, which catalyses the first step in GSH synthesis, NQO-1, HO-1, and many others, plays a critical role in the antioxidant defense and survival pathway against oxidative stress (Zhu et al. Citation2012). Recently, molecular docking and quantitative structure–activity relationship (QSAR) model of a specific target biological macromolecule to predict experimental activity in silico. Sun et al. (Citation2014) reported the receptor–ligand binding pharmacophore model of Nrf2–Keap1. Zhuang et al. (Citation2014) also reported the molecules which subjected to docking against the C-terminal Kelch region of human Keap1 at the “ETGE” domain of the Nrf2 peptide site. These results support the notion that further studies are still required to investigate QSAR of 5-CQA on Nrf2 activation and its antioxidative effect.
In this study, 5-CQA increased the nuclear translocation of Nrf2, which led to transcription augmentation of its downstream targets, including GCL, HO-1, NQO-1 and Sesn2 in hepatocytes. Nrf2 activation by 5-CQA was verified with Nrf2 inhibitor and Nrf2 KO MEF cells. Nrf2 deficiency completely abolished the 5-CQA-induced HO-1 and GCL expression. Furthermore, this study demonstrated that 5-CQA increases the phosphorylation of Nrf2, which is accompanied by p38, AMPK and PKCδ phosphorylation. In addition, ARE-mediated gene expression was inhibited by specific chemical inhibitors of P38, AMPK and PKCδ which confirmed the role of upstream kinases in Nrf2 activation by 5-CQA. In the current study, we investigated the in vitro effects of the 5-CQA in the cultured human hepatocytes. Based on this study, further studies are also needed to examine the efficacy and effectiveness of 5-CQA using in vivo animal models and even clinical trials.
As one of the most abundant polyphenols found in herbs and vegetables, 5-CQA has received much attention for its numerous positive effects on human health and benefits in the treatment of cancer, inflammation, cardiovascular disease, diabetes, and neurologic diseases (Chen and Wu Citation2014; Liang and Kitts Citation2015; Yan et al. Citation2015). Moreover, Liang and Kitts (Citation2018) recently reported chlorogenic acid isomers on Nrf2 activation in Caco-2 cells, human epithelial colorectal adenocarcinoma cells. However, the antioxidative effect and its molecular mechanism of 5-CQA in hepatocytes remains to be elucidated. We adopted HepG2 cells and examined Nrf2 activation and its concise molecular mechanism by 5-CQA for the first time. Based on the results of current study, 5-CQA was found to be involved in antioxidation and cytoprotection through Nrf2 activation. As the one core regulator of Nrf2, 5-CQA protects against oxidative damage in hepatocytes via GCL, HO-1, NQO-1 and Sesn2 induction ().
Conclusions
Taken together, our results demonstrate that as a novel Nrf2 activator, 5-CQA, maybe a promising candidate against oxidative stress-mediated liver injury. Based on the results of the current study, additional efforts are needed to examine of 5-CQA as a potential therapeutic in liver disease in vivo and in humans.
Disclosure statement
The authors declare that there are no conflicts of interest.
Additional information
Funding
References
- Baird L, Lleres D, Swift S, Dinkova-Kostova AT. 2013. Regulatory flexibility in the Nrf2-mediated stress response is conferred by conformational cycling of the Keap1-Nrf2 protein complex. Proc Natl Acad Sci U S A. 110:15259–15264.
- Bea F, Hudson FN, Chait A, Kavanagh TJ, Rosenfeld ME. 2003. Induction of glutathione synthesis in macrophages by oxidized low-density lipoproteins is mediated by consensus antioxidant response elements. Circ Res. 92:386–393.
- Chen WP, Wu LD. 2014. Chlorogenic acid suppresses interleukin-1beta-induced inflammatory mediators in human chondrocytes. Int J Clin Exp Pathol. 7:8797–8801.
- Cho SS, Yang JH, Seo KH, Shin SM, Park EY, Cho SS, Jo GU, Eo JH, Park JS, Oh DS, et al. 2019. Cudrania tricuspidata extract and its major constituents inhibit oxidative stress-induced liver injury. J Med Food. 22:602–613.
- Han J, Isoda H. 2011. Neuroprotective effects of 3,5-di-O-caffeoylquinic acid in vitro and in vivo. BMC Proc. 5 Suppl 8:P20.
- Han JY, Cho SS, Yang JH, Kim KM, Jang CH, Park DE, Bang JS, Jung YS, Ki SH. 2015. The chalcone compound isosalipurposide (ISPP) exerts a cytoprotective effect against oxidative injury via Nrf2 activation. Toxicol Appl Pharmacol. 287:77–85.
- Hayes JD, Chanas SA, Henderson CJ, McMahon M, Sun C, Moffat GJ, Wolf CR, Yamamoto M. 2000. The Nrf2 transcription factor contributes both to the basal expression of glutathione S-transferases in mouse liver and to their induction by the chemopreventive synthetic antioxidants, butylated hydroxyanisole and ethoxyquin. Biochem Soc Trans. 28:33–41.
- Huang HC, Nguyen T, Pickett CB. 2002. Phosphorylation of Nrf2 at Ser-40 by protein kinase C regulates antioxidant response element-mediated transcription. J Biol Chem. 277:42769–42774.
- In JK, Kim JK, Oh JS, Seo DW. 2016. 5-Caffeoylquinic acid inhibits invasion of non-small cell lung cancer cells through the inactivation of p70S6K and Akt activity: involvement of p53 in differential regulation of signaling pathways. Int J Oncol. 48:1907–1912.
- Joo MS, Kim WD, Lee KY, Kim JH, Koo JH, Kim SG. 2016. AMPK facilitates nuclear accumulation of Nrf2 by phosphorylating at serine 550. Mol Cell Biol. 36:1931–1942.
- Kang KW, Lee SJ, Park JW, Kim SG. 2002. Phosphatidylinositol 3-kinase regulates nuclear translocation of NF-E2-related factor 2 through actin rearrangement in response to oxidative stress. Mol Pharmacol. 62:1001–1010.
- Kim J, Lee S, Shim J, Kim HW, Kim J, Jang YJ, Yang H, Park J, Choi SH, Yoon JH, et al. 2012. Caffeinated coffee, decaffeinated coffee, and the phenolic phytochemical chlorogenic acid up-regulate NQO1 expression and prevent H2O2-induced apoptosis in primary cortical neurons. Neurochem Int. 60:466–474.
- Kweon MH, Adhami VM, Lee JS, Mukhtar H. 2006. Constitutive overexpression of Nrf2-dependent heme oxygenase-1 in A549 cells contributes to resistance to apoptosis induced by epigallocatechin 3-gallate. J Biol Chem. 281:33761–33772.
- Liang N, Kitts DD. 2015. Role of chlorogenic acids in controlling oxidative and inflammatory stress conditions. Nutrients. 8:16.
- Liang N, Kitts DD. 2018. Amelioration of oxidative stress in Caco-2 cells treated with pro-inflammatory proteins by chlorogenic acid isomers via activation of the Nrf2-Keap1-ARE-signaling pathway. J Agric Food Chem. 66:11008–11017.
- Liguori I, Russo G, Curcio F, Bulli G, Aran L, Della-Morte D, Gargiulo G, Testa G, Cacciatore F, Bonaduce D, et al. 2018. Oxidative stress, aging, and diseases. Clin Interv Aging. 13:757–772.
- Liou GY, Storz P. 2010. Reactive oxygen species in cancer. Free Radic Res. 44:479–496.
- McMahon M, Itoh K, Yamamoto M, Chanas SA, Henderson CJ, McLellan LI, Wolf CR, Cavin C, Hayes JD. 2001. The Cap'n'Collar basic leucine zipper transcription factor Nrf2 (NF-E2 p45-related factor 2) controls both constitutive and inducible expression of intestinal detoxification and glutathione biosynthetic enzymes. Cancer Res. 61:3299–3307.
- Niture SK, Kaspar JW, Shen J, Jaiswal AK. 2010. Nrf2 signaling and cell survival. Toxicol Appl Pharmacol. 244:37–42.
- Rocha LD, Monteiro MC, Teodoro AJ. 2012. Anticancer properties of hydroxycinnamic acids-a review. Cancer Clin Oncol. 1:109–121.
- Shin BY, Jin SH, Cho IJ, Ki SH. 2012. Nrf2-ARE pathway regulates induction of Sestrin-2 expression. Free Radic Biol Med. 53:834–841.
- Shin SM, Yang JH, Ki SH. 2013. Role of the Nrf2-ARE pathway in liver diseases. Oxid Med Cell Longev. 2013:763257.
- Singh A, Venkannagari S, Oh KH, Zhang YQ, Rohde JM, Liu L, Nimmagadda S, Sudini K, Brimacombe KR, Gajghate S, et al. 2016. Small molecule inhibitor of NRF2 selectively intervenes therapeutic resistance in KEAP1-deficient NSCLC tumors. ACS Chem Biol. 11:3214–3225.
- Slamenova D, Kozics K, Hunakova L, Melusova M, Navarova J, Horvathova E. 2013. Comparison of biological processes induced in HepG2 cells by tert-butyl hydroperoxide (t-BHP) and hydroperoxide (H2O2): the influence of carvacrol. Mutat Res. 757:15–22.
- Sun HP, Jiang ZY, Zhang MY, Lu MC, Yang TT, Pan Y, Huang HZ, Zhang XJ, You QD. 2014. Novel protein–protein interaction inhibitor of Nrf2–Keap1 discovered by structure-based virtual screening. MedChemComm. 5:93–98.
- Suzuki T, Yamamoto M. 2015. Molecular basis of the Keap1-Nrf2 system. Free Radic Biol Med. 88:93–100.
- Wild AC, Gipp JJ, Mulcahy T. 1998. Overlapping antioxidant response element and PMA response element sequences mediate basal and beta-naphthoflavone-induced expression of the human gamma-glutamylcysteine synthetase catalytic subunit gene. Biochem J. 332:373–381.
- Willis D, Moore AR, Frederick R, Willoughby DA. 1996. Heme oxygenase: a novel target for the modulation of the inflammatory response. Nat Med. 2:87–90.
- Yan Y, Li J, Han J, Hou N, Song Y, Dong L. 2015. Chlorogenic acid enhances the effects of 5-fluorouracil in human hepatocellular carcinoma cells through the inhibition of extracellular signal-regulated kinases. Anticancer Drugs. 26:540–546.
- Yang JH, Kim KM, Cho SS, Shin SM, Ka SO, Na CS, Park BH, Jegal KH, Kim JK, Ku SK, et al. 2019. Inhibitory effect of Sestrin 2 on hepatic stellate cell activation and liver fibrosis. Antioxid Redox Signal. 31:243–259.
- Zhang H, Davies KJA, Forman HJ. 2015. Oxidative stress response and Nrf2 signaling in aging. Free Radic Biol Med. 88:314–336.
- Zhu R, Wang Y, Zhang L, Guo Q. 2012. Oxidative stress and liver disease. Hepatol Res. 42:741–749.
- Zhuang C, Narayanapillai S, Zhang W, Sham YY, Xing C. 2014. Rapid identification of Keap1-Nrf2 small-molecule inhibitors through structure-based virtual screening and hit-based substructure search. J Med Chem. 57:1121–1126.
- Zipper LM, Mulcahy RT. 2003. Erk activation is required for Nrf2 nuclear localization during pyrrolidine dithiocarbamate induction of glutamate cysteine ligase modulatory gene expression in HepG2 cells. Toxicol Sci. 73:124–134.