Abstract
Context
As an inhibitor cytochrome P450 family 2 subfamily C polypeptide 8 (CYP2C8), quercetin is a naturally occurring flavonoid with its glycosides consumed at least 100 mg per day in food. However, it is still unknown whether quercetin and selexipag interact.
Objective
The study investigated the effect of quercetin on the pharmacokinetics of selexipag and ACT-333679 in beagles.
Materials and methods
The ultra-performance liquid chromatography-tandem mass spectrometry (UPLC-MS/MS) was used to investigate the pharmacokinetics of orally administered selexipag (2 mg/kg) with and without quercetin (2 mg/kg/day for 7 days) pre-treatment in beagles. The effect of quercetin on the pharmacokinetics of selexipag and its potential mechanism was studied through the pharmacokinetic parameters.
Results
The assay method was validated for selexipag and ACT-333679, and the lower limit of quantification for both was 1 ng/mL. The recovery and the matrix effect of selexipag were 84.5–91.58% and 94.98–99.67%, while for ACT-333679 were 81.21–93.90% and 93.17–99.23%. The UPLC-MS/MS method was sensitive, accurate and precise, and had been applied to the herb-drug interaction study of quercetin with selexipag and ACT-333679. Treatment with quercetin led to an increased in Cmax and AUC0–t of selexipag by about 43.08% and 26.92%, respectively. While the ACT-333679 was about 11.11% and 18.87%, respectively.
Discussion and conclusion
The study indicated that quercetin could inhibit the metabolism of selexipag and ACT-333679 when co-administration. Therefore, the clinical dose of selexipag should be used with caution when co-administered with foods high in quercetin.
Introduction
Characterised by a gradual increase in pulmonary vascular resistance and pulmonary artery pressure, Pulmonary Arterial Hypertension (PAH) is a progressive, debilitating and chronic life-threatening disease (Sardana et al. Citation2016; Bruderer et al. Citation2017; Bhadru et al. Citation2019; Highland et al. Citation2019; Ilyin et al. Citation2019; Klose et al. Citation2019, Citation2021; Yazıcı & Güngör Citation2019; A Xe Lsen et al. Citation2021; Genecand et al. Citation2021). PAH may cause right ventricular dysfunction and potential failure and the average survival time of patients is only 2.8 years if not treated (Gnerre et al. Citation2018; Highland et al. Citation2019). There is strong evidence to support early intervention and the achievement of all treatment objectives with monotherapy or combination therapy has been critical to date (Ilyin et al. Citation2019). Prostacyclin, produced by prostaglandin H2 (PGH2) endothelial cells via prostacyclin synthase, is a potent vasodilator with anti-proliferative, anti-thrombotic, and anti-inflammatory effects (Bhadru et al. Citation2019). The role of prostacyclin or prostacyclin receptor (IP receptor) agonists in the treatment of PAH is reasonable because PAH is associated with vasoconstriction, proliferation, and thrombosis (Gnerre et al. Citation2018).
Due to short-term benefits (efficacy) related to the short half-life, chemical instability, and delivery systems, the prostanoids epoprostenol, treprostinil, iloprost, and beraprost are not widely used (Badesch et al. Citation2004). As a novel, orally available, long-acting (half-life of 6.2–13.5 h), highly selective IP receptor agonist, selexipag () discovered by Nippon Shinyaku Co., Ltd. was approved in the treatment of PAH by the US Food and Drug Administration (FDA) in 2015, the European Medicines Agency and the Japanese Pharmaceuticals and Medical Devices Agency in 2016 (Asaki et al. Citation2015; Highland et al. Citation2019; Imai et al. Citation2019). It is recommended that the initial dose of selexipag is 200 μg twice daily, and it can be increased to a maximum dose of 1600 μg twice daily based on the individual patient’s highest tolerated dose (Gnerre et al. Citation2018; Yazıcı and Güngör 2019; Klose et al. Citation2021). After oral administration, selexipag is rapidly metabolised by carboxylesterase hydrolysis to the active metabolite ACT-333679 (). The liver is the key organ for selexipag and ACT-333679 metabolism and clearance. The parent drug and active metabolite are mainly metabolised by CYP2C8, and to a lesser extent by cytochrome P450 family 3 subfamily A polypeptide 4 (CYP3A4) in vivo, and processed by organic anion-transporting polypeptide (OATP) 1B1 and OATP1B3 in vitro (Kaufmann et al. Citation2016; Gatfield et al. Citation2017; Gnerre et al. Citation2018; Imai et al. Citation2019). Furthermore, the uridine 5′-diphosphoglucuronosyltransferase (UGT) enzymes, UGT1A3 and UGT2B7 are involved in the metabolism of ACT-333679 (Gnerre et al. Citation2018). Some studies had indicated that ACT-333679 was approximately 3- to 4-fold higher than the parent drug at a steady-state after oral selexipag administration, while only about 1.3-fold higher than the parent drug after intravenous administration (Imai et al. Citation2019). It has been verified that ACT-333679 is the main contributor to the pharmacological effect due to 37-fold more potent than selexipag in activating the prostacyclin receptor (Kaufmann et al. Citation2015a,Citationb; Bruderer et al. Citation2017; Gatfield et al. Citation2017).
Figure 1. The chemical structure of selexipag (A), its active metabolite ACT-333679 (B), and quercetin (C).
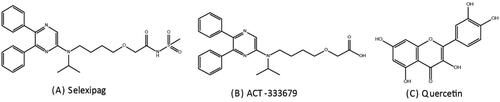
It will cause a marked increase in ACT-333679 exposure when concomitant administration of selexipag and a strong CYP2C8 inhibitor. Therefore, this drug combination is contraindicated. Quercetin () is a naturally occurring flavonoid with its glycosides found in a variety of food, including grains, fruits, red wine, and other beverages (Kim et al. Citation2005; Dabeek and Marra Citation2019; Elbarbry et al. Citation2019). A daily intake of quercetin glycosides of at least 100 mg is conventional (Chandrasekaran et al. Citation1978). As previous studies mentioned, quercetin has the potential to inhibit cytochrome P450 enzymes, especially CYP2C8 (Chandrasekaran et al. Citation1978; Projean et al. Citation2003; Pang et al. Citation2012; Cao et al. Citation2017). However, it is still unclear whether quercetin and selexipag interact in any way. This study assesses the effect of quercetin on the pharmacokinetics of selexipag and its active metabolite in beagles.
Materials and methods
Chemicals and reagents
The selexipag, ACT-333679 and Marimastat (internal standard, IS), with ≥98% purity of each substance, were provided by Beijing Sun-flower and Technology Development CO., Ltd. (Beijing, China). Quercetin (purity ≥98%) was purchased from Shanghai Chuangsai Technology CO., Ltd. (Shanghai, China). Acetonitrile and methanol obtained from Merck Company (Darmstadt, Germany) were high-performance liquid chromatography (HPLC) grade. A Milli Q system (Millipore, Bedford, USA) was used to prepare the ultrapure water. All other chemicals were of analytical grade or better.
Instruments and conditions
The analysis was performed on the UPLC-MS/MS system (Waters, Milford, MA, USA) including the Acquity UPLC H-Class system and a XEVO TQ-S triple quadrupole mass spectrometer equipped with an electrospray ionisation (ESI) source. Selexipag, ACT-333679, and IS were separated on an Acquity BEH C18 column (2.1 mm × 50 mm, 1.7 μm) by gradient elution using the mobile phase of acetonitrile (solvent A) and water with 0.1% formic acid (solvent B). The flow rate was set at 0.4 mL/min and the gradient program was as follows: 0.0–1.4 min, 70 − 15% B; 1.4 − 2.6 min, 15 − 70% B; and 2.6 − 3.0 min, 70% B. The injection volume was 0.2 μL for analysis.
The quantitative test was carried out with the precursor-to-product ion transitions of m/z 496.95 → 302.04 for selexipag, m/z 419.98 → 378.20 for ACT-333679, and m/z 332.20 → 301.20 for IS under the selected multiple reaction monitoring (MRM). The cone voltage for selexipag, ACT-333679 and IS were 30 eV, 30 eV, 10 eV, respectively. Meanwhile, the collision energy of selexipag, ACT-333679 and IS were 30 eV, 25 eV, 10 eV, respectively. The MS/MS conditions were optimised as follows: desolvation temperature 600 °C, capillary voltage 2.0 kV, cone gas 150 L/h, desolvation gas 1000 L/h, collision gas 0.15 mL/min. All data (included sample quantitation, data analysis) and instrument control were operated by Masslynx V4.1 software (Waters, Milford, MA, USA).
Method validation
The UPLC-MS/MS method was validated for the specificity, linearity, sensitivity, accuracy and precision, and matrix effect according to the ‘Guidance for Industry – Bioanalytical Method Validation’ recommended by the FDA (Health UDo et al. Citation2001). The specificity of the method was assessed by analysing blank beagle plasma, blank plasma spiked with selexipag, ACT-333679 and IS, and a beagle plasma sample.
Calibration curves were constructed at 1.0–4000 ng/mL for selexipag and ACT-333679. The linearity of the assay was assessed by analysing the calibration curves using a weighted (1/χ2) least-squares linear regression method by measuring the peak area ratio of the analytes to the IS. The acceptance criterion for each back-calculated standard concentration was ±15% deviation from the nominal value, except for the lower limit of quantification (LLOQ) that a deviation of ± 20% was permitted.
The precision and accuracy were assessed by the determination of quality control (QC) samples at low, medium, and high concentration levels in six replicates. The intra-day precision and accuracy were evaluated on the same day, while the inter-day precision and accuracy were calculated by continuous measurement within 3 days. Precision required to be within ±15% was expressed as the relative standard deviation (RSD%) and accuracy not to exceed 15% as the relative error (RE%).
Selexipag and ACT-333679 extraction recoveries were calculated by comparing the peak area of the analytes in QC samples to which the analytes were added post-protein precipitation at equivalent concentrations. The matrix effect was evaluated by comparison of the peak areas obtained from samples where the extracted matrix was spiked with standard solutions to those obtained from the pure reference standard solution at equivalent concentrations.
The stability of the analytes was conducted at three QC levels in several different storage conditions: room temperature for 12 h, autosampler 4 °C for 12 h, three freeze-thaw from −20 °C to room temperature, −40 °C for 4 weeks. The analytes were considered to be stable when the calculated concentration was within ± 15% of the nominal concentration.
Animal experiments
Six beagles (3 male and 3 female, weighing 7.5–9.0 kg and aged 2.4 years) were purchased from Hubei Yizhicheng Biological Technology Co., Ltd with the animal certificate SCXK (Hubei) 2016-0020. The beagles were maintained in a temperature-controlled room (24 ± 2 °C) with a 12 h dark/light cycle for a seven-day acclimation period. All the operations involved in the experiment followed the National Institutes of Health Guide for the Care and Use of Laboratory Animals.
Pharmacokinetic study
Six beagles were given 1% CMC orally for one week. On the seventh day, the beagles were given selexipag 2 mg/kg orally. At 0.5, 1, 1.5, 2, 3, 4, 6, 9, 12, 24, and 48 h, 1 mL blood samples were taken into the 1.5 mL heparinised Eppendorf tubes from a leg vein through an indwelling vein catheter. Two h after the start of the experiment, the beagle had free access to water and food to reduce animal suffering. Plasma (100 µL) was obtained after centrifuging at 4000 g for 8 min, and then 1.0 M hydrochloric acid was added (10% plasma volume). If not analysed immediately, the samples were stored at −40 °C freezer. For convenience, the six beagles here we call the control group.
After a 14-day washout period, the same six beagles were given quercetin 2 mg/kg orally for one week. On the seventh day, the beagles were given selexipag 2 mg/kg orally after oral quercetin 2 mg/kg. The following procedure is the same as the previous experiment. These six beagles here we call the treatment group.
Sample preparation
Acetonitrile (300 μL) with IS (100 ng/mL) was added into 100 μL the plasma sample thawed at room temperature. After vortexed for 1.0 min and centrifugation at 13,000 g for 10 min, the supernatant (0.2 μL) was transferred and injected into the UPLC-MS/MS system for analysis.
Pharmacokinetic analysis
The pharmacokinetic parameters of selexipag and ACT-333679 after orally administered selexipag with and without quercetin pre-treatment in beagles were calculated by the DAS 2.0 software (Shanghai University of Traditional Chinese Medicine, China) in non-compartmental analysis. Cmax was the maximum observed plasma concentration, Tmax was the time to reach the maximum observed plasma concentration. AUC0–t and AUC0–∞ were the area under the concentration-time curve to the last time point and area to infinity, respectively. The apparent volume of distribution (Vd) refers to the ratio of the drug dose to blood concentration after a drug has reached dynamic equilibrium in the body. Plasma clearance (CL) is the total clearance rate of drugs in the liver and kidney, that is, how much volume of plasma is cleared of drugs per unit time.
Data analysis
The results are expressed as mean ± SD, or RSD%, or RE%. Differences in pharmacokinetic parameters of selexipag and ACT-333679 with or without treatment of quercetin are assessed by Student’s paired t-test. The SPSS 13.0 software (IBM Corporation, New York, NY, USA) is used for statistical analysis. There is considered to be statistical significance when p < 0.05.
Results
The results of method validation
As previously reported (Xie et al. Citation2020), the Acquity BEH C18 column (2.1 mm × 50 mm, 1.7 μm) had a better separation and resolution, and a short analysis time. Meanwhile, acetonitrile could also provide better separation, a sharper peak shape, and higher recovery when compared to methanol. The water phase improved the sensitivity, reproducibility and ion intensity of the analytes with the addition of formic acid (containing 0.1%). Compared with isocratic elution, gradient elution could purify the chromatographic column, provide a proper retention time, avoid the matrix effects by reducing the interference of endogenous substances. The deviation would lead to the pharmacokinetic parameters of selexipag and ACT-333679 being disorganised due to the value of pH between plasma batches. Finally, we found that when the volume ratio of 1.0 M hydrochloric acid to plasma was equal to 1:10, it was optimal in avoiding the acyl glucuronide of ACT-333679 hydrolysed to ACT-333679 in sample preparation as described previously (Kaufmann et al. Citation2015a).
There was not apparent interference of endogenous substances in the mass spectrum () or chromatograms (). The retention times of selexipag, ACT-333679 and IS were 1.72 min, 1.70 min, 0.52 min, respectively. The method exhibited good linear relationships in the range of 1–4000 ng/mL for both selexipag and ACT-333679. 1 ng/mL was the LLOQ for both selexipag and ACT-333679. The accuracy and precision for selexipag were from −9.84% to 10.66% and 2.70% to 7.22% (), respectively. While for ACT-333679 were 2.88–11.24% and −8.30–6.19% (), respectively. Meanwhile, the recoveries of selexipag and ACT-333679 were 84.55–91.58% and 81.21–93.90%, respectively. The matrix effect met the requirements of the bioanalytical method (). The results of stability in different conditions (room temperature for 12 h, autosampler 4 °C for 12 h, three times freeze-thaw, −40 °C for 4 weeks) were summarised in , and it was in accord with the demand of the experiment.
Figure 2. The product-ion mass spectrum of the analytes in the present study: (A) Selexipag; (B) ACT-333679; (C) Marimastat (IS).
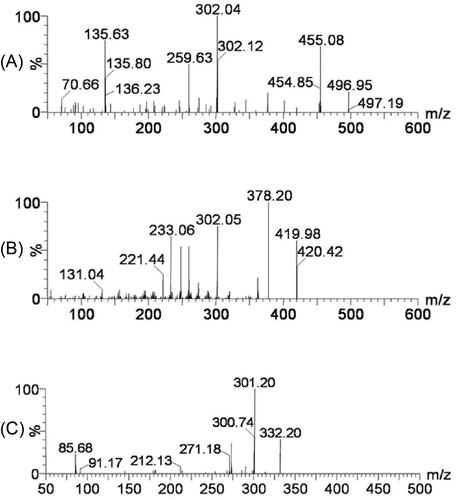
Figure 3. The representative chromatograms of the analytes in the present study: (A) a blank plasma sample; (B) a blank plasma sample spiked with selexipag, ACT-333679, and Marimastat (IS); (C) a beagle plasma sample after oral administration of selexipag.
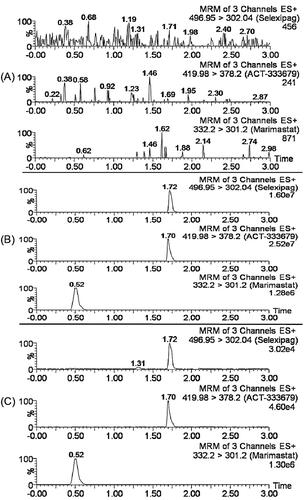
Table 1. Intra- and Inter-day accuracy and precision of selexipag and ACT-333679 in beagle plasma (n = 6, RSD%, RE%).
Table 2. The recoveries and matrix effect of selexipag and ACT-333679 in beagle plasma (n = 6, mean ± SD, RSD).
Table 3. Stability results of selexipag and ACT-333679 in beagle plasma in different conditions (n = 6, RSD%, RE%).
The effect of quercetin on the pharmacokinetics of selexipag and ACT-333679
Mean plasma concentration-time profiles of selexipag and ACT-333679 in beagle dogs after orally administered selexipag (2 mg/kg) with and without quercetin pre-treatment were presented in . While the semi-log transformed mean plasma concentration-time profiles of selexipag and ACT-333679 were shown in . As shown in and , mean plasma concentration-time profiles of selexipag and ACT-333679 in the treatment group were higher than the control group at most time points. The figures showed that the Tmax of selexipag in the two groups was similar, but the Tmax of ACT-333679 in the control group was slightly later.
Figure 4. Mean plasma concentration-time profiles of selexipag (A) and ACT-333679 (B) in beagle dogs after orally administered selexipag (2 mg/kg) with and without quercetin pre-treatment (n = 6, Mean ± SD).
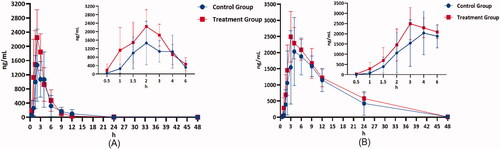
Figure 5. The semi-log transformed mean plasma concentration-time profiles of selexipag (A) and ACT-333679 (B) in beagle dogs after orally administered selexipag (2 mg/kg) with and without quercetin pre-treatment (n = 6, Mean ± SD).
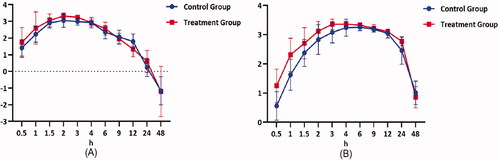
The pharmacokinetic parameters of selexipag and ACT-333679 with or without treatment of quercetin (2 mg/kg/day for 7 days) were presented in . For selexipag, t1/2 (3.12 ± 0.91 vs. 4.61 ± 2.77), Cmax (1789.35 ± 855.23 vs. 2560.15 ± 472.94, p < 0.05), AUC(0-t) (6471.39 ± 2724.72 vs. 8213.31 ± 2560.97) were increased when the beagles were pre-treated with quercetin. While for ACT-333679, t1/2 (5.34 ± 1.14 vs. 8.04 ± 2.89), Cmax (2486.32 ± 820.92 vs. 2762.67 ± 561.56, p < 0.05), AUC(0-t) (31502.97 ± 9102.83 vs. 37446.69 ± 6455.51) were also increased. On the contrary, Tmax (3.10 ± 1.88 vs. 2.33 ± 0.52), CL (0.36 ± 0.15 vs. 0.27 ± 0.12, p < 0.05) of selexipag, and Tmax (6.20 ± 2.78 vs. 3.83 ± 1.17), CL (0.07 ± 0.02 vs. 0.05 ± 0.01, p < 0.05) of ACT-333679 were decreased. The results indicated that quercetin might inhibit the metabolism of both selexipag and ACT-333679 in beagles with quercetin pre-treatment.
Table 4. The pharmacokinetic parameters of selexipag and ACT-333679 in beagle plasma after oral administration 2.0 mg/kg selexipag with or without treatment of quercetin (n = 6, Mean ± SD).
Discussion
A fast, simple and sensitive UPLC-MS/MS method can simultaneously determine the selexipag and ACT-333679 in beagle plasma. The intra-day and inter-day precision and accuracy, sensitivity, recovery, and matrix effect of this method are following FDA guidelines. The bioanalytical method based on UPLC-MS/MS has been successfully applied for pharmacokinetic or pharmacokinetic interaction studies. This study adopts the design of self-controlled, which can effectively reduce the interference caused by individual differences.
It is widely believed that the phytochemicals derived from natural products are usually safe. However, people hardly realise that it may lead to serious clinically significant interactions when combined with prescription or over-the-counter drugs. Quercetin used for more than 160 years is a naturally polar auxin transport inhibitor (Fischer et al. Citation1997). Some researchers have reported that although cytochrome P450 family 1 subfamily A polypeptide 1 (CYP1A1), cytochrome P450 family 2 subfamily A polypeptide 6 (CYP2A6), and cytochrome P450 family 2 subfamily E polypeptide 1 (CYP2E1) are not affected by the quercetin, quercetin has the potential to inhibit CYP2C8 and CYP3A4 (Chandrasekaran et al. Citation1978; Elbarbry et al. Citation2019). The in vitro study has demonstrated that selexipag is hydrolysed by CYP3A4 and CYP2C8 enzymes to the main active metabolite, ACT-333679 (Gnerre et al. Citation2018). However, ACT-333679 is not only metabolised by CYP3A4 and CYP2C8 but also metabolised by other ways such as the uridine 5″-diphosphoglucuronosyltransferase (UGT) enzymes, etc. (Gnerre et al. Citation2018).
shows that mean plasma concentration-time profiles of selexipag and ACT-333679 in the treatment group were higher than the control group at most time points. Metabolised mainly by CYP2C8, selexipag is a strong inhibitor of CYP2C8 at the same time. Meanwhile, CYP2C8 is an extent inhibited by quercetin. ACT-333679 is also metabolised primarily by CYP2C8 and may compete with selexipag for CYP2C8. In addition, quercetin can increase the bioavailability of selexipag by inhibiting P-glycoprotein (P-gp), since selexipag is the substrate of P-gp protein (Kim et al. Citation2005; Bruderer et al. Citation2017; A Xe Lsen et al. Citation2021). Therefore, this can account for the fact that the plasma concentration-time profile of selexipag is significantly higher in the treatment group than in the control group.
Under normal circumstances, selexipag is rapidly absorbed after oral administration. Meanwhile, selexipag is rapidly metabolised to ACT-333679, and the plasma concentration of ACT-333679 is about 4 times that of the parent drug (Gnerre et al. Citation2018). The present results indicate that the maximum plasma concentration of ACT-333679 is much higher than selexipag, but not a four-fold difference. As quercetin and selexipag are the inhibitors of CYP2C8 and cytochrome P450 family 2 subfamily C polypeptide 9 (CYP2C9), the plasma concentration-time of ACT-333679 in the treatment group is slightly higher than the control group. In addition to the slower metabolism of ACT-333679 than selexipag (Ichikawa et al. Citation2019), species differences may account for this difference.
Conclusions
We developed a hypothesis about the effect of quercetin on selexipag, but the precise mechanism is still unknown. More rigorous studies are needed to confirm this in the future. The current research results indicate that caution is needed when quercetin and selexipag are applied at the same time. Because the plasma concentrations of the parent drug and the active metabolite that plays a major pharmacological role are increased. It is necessary to reduce the dose of selexipag or minimise the intake of quercetin in the treatment of pulmonary hypertension.
Disclosure statement
The authors in this manuscript declare no potential conflicts of interest.
References
- Asaki T, Kuwano K, Morrison K, Gatfield J, Hamamoto T, Clozel M. 2015. Selexipag: an oral and selective IP prostacyclin receptor agonist for the treatment of pulmonary arterial hypertension. J Med Chem. 58(18):7128–7137.
- A Xe Lsen LN, Poggesi I, Rasschaert F, Ruixo J, Bruderer S. 2021. Clopidogrel, a CYP2C8 inhibitor, causes a clinically relevant increase in the systemic exposure to the active metabolite of selexipag in healthy subjects. Br J Clin Pharmacol. 87(1):119–128.
- Badesch DB, McLaughlin VV, Delcroix M, Vizza CD, Olschewski H, Sitbon O, Barst RJ. 2004. Prostanoid therapy for pulmonary arterial hypertension. J Am Coll Cardiol. 43(12 Suppl S):56S–61s.
- Bhadru B, Rao VV, Vidhyadhara S. 2019. Development and validation of bioanalytical method for the quantitative estimation of selexipag in biological matrices using LC-MS/MS. Pharm Sci Res. 11:2722–2727.
- Bruderer S, Hurst N, Remenova T, Dingemanse J. 2017. Clinical pharmacology, efficacy, and safety of selexipag for the treatment of pulmonary arterial hypertension. Expert Opin Drug Saf. 16(6):743–751.
- Bruderer S, Petersen-Sylla M, Boehler M, Remeňová T, Halabi A, Dingemanse J. 2017. Effect of gemfibrozil and rifampicin on the pharmacokinetics of selexipag and its active metabolite in healthy subjects. Br J Clin Pharmacol. 83(12):2778–2788.
- Cao L, Kwara A, Greenblatt DJ. 2017. Metabolic interactions between acetaminophen (paracetamol) and two flavonoids, luteolin and quercetin, through in-vitro inhibition studies. J Pharm Pharmacol. 69(12):1762–1772.
- Chandrasekaran SK, Benson H, Urquhart J. 1978. Methods to achieve controlled drug delivery: the biomedical engineering approach. In: Robinson JR, ed. Sustained and controlled release drug delivery systems. New York: Marcel Dekker; p. 557–593.
- Dabeek WM, Marra MV. 2019. Dietary quercetin and kaempferol: bioavailability and potential cardiovascular-related bioactivity in humans. Nutrients. 11(10):2288–2306.
- Elbarbry F, Ung A, Rao D, Abdelkawy K. 2019. Effect of dietary doses of quercetin on hepatic drug metabolizing enzymes in spontaneously hypertensive rats. Eur J Drug Metab Pharmacokinet. 44(6):761–770.
- Fischer C, Speth V, Fleig-Eberenz S, Neuhaus G. 1997. Induction of zygotic polyembryos in wheat: influence of auxin polar transport. Plant Cell. 9(10):1767–1780.
- Gatfield J, Menyhart K, Wanner D, Gnerre C, Monnier L, Morrison K, Hess P, Iglarz M, Clozel M, Nayler O. 2017. Selexipag active metabolite ACT-333679 displays strong anticontractile and antiremodeling effects but low β-arrestin recruitment and desensitization potential. J Pharmacol Exp Ther. 362(1):186–199.
- Genecand L, Wacker J, Beghetti M, Lador F. 2021. Selexipag for the treatment of pulmonary arterial hypertension. Expert Rev Respir Med. 15(5):583–595.
- Gnerre C, Segrestaa J, Seeland S, Äänismaa P, Pfeifer T, Delahaye S, de Kanter R, Ichikawa T, Yamada T, Treiber A. 2018. The metabolism and drug-drug interaction potential of the selective prostacyclin receptor agonist selexipag. Xenobiotica. 48(7):704–719.
- Health UDo, Human services F, Drug Administration CfDE, Research CfVm. 2001. Guidance for industry, bioanalytical method validation. Fed Regist. 66:206–207.
- Highland KB, Hull M, Pruett J, Elliott C, Tsang Y, Drake W. 2019. Baseline history of patients using selexipag for pulmonary arterial hypertension. Ther Adv Respir Dis. 13:1753466619843774.
- Ichikawa T, Yamada T, Treiber A, Gnerre C, Segrestaa J, Seeland S, Nonaka K. 2019. Cross-species comparison of the metabolism and excretion of selexipag. Xenobiotica. 49(3):284–301.
- Ilyin NV, Ivanov KI, Martynyuk TV. 2019. New treatment options for pulmonary arterial hypertension — the first selective IP-receptor agonist selexipag. Cardiovasc Ther Prev. 18(6):80–87.
- Imai S, Ichikawa T, Sugiyama C, Nonaka K, Yamada T. 2019. Contribution of human liver and intestinal carboxylesterases to the hydrolysis of selexipag in vitro. J Pharm Sci. 108(2):1027–1034.
- Kaufmann P, Cruz HG, Krause A, Ulč I, Halabi A, Dingemanse J. 2016. Pharmacokinetics of the novel oral prostacyclin receptor agonist selexipag in subjects with hepatic or renal impairment. Br J Clin Pharmacol. 82(2):369–379.
- Kaufmann P, Niglis S, Bruderer S, Segrestaa J, Äänismaa P, Halabi A, Dingemanse J. 2015a. Effect of lopinavir/ritonavir on the pharmacokinetics of selexipag an oral prostacyclin receptor agonist and its active metabolite in healthy subjects. Br J Clin Pharmacol. 80(4):670–677.
- Kaufmann P, Okubo K, Bruderer S, Mant T, Yamada T, Dingemanse J, Mukai H. 2015b. Pharmacokinetics and tolerability of the novel oral prostacyclin IP receptor agonist selexipag. Am J Cardiovasc Drugs. 15(3):195–203.
- Kim KA, Park PW, Kim HK, Ha JM, Park JY. 2005. Effect of quercetin on the pharmacokinetics of rosiglitazone, a CYP2C8 substrate, in healthy subjects. J Clin Pharmacol. 45(8):941–946.
- Klose H, Chin K, Ewert R, Gall H, Parambil J, Poch D, Seyfarth H, Axelsen L, Schmitz SH, Stein C, et al. 2019. Safety, tolerability and pharmacokinetics study in patients with pulmonary arterial hypertension (PAH) temporarily switching from oral to IV selexipag. J Heart Lung Transplantation. 38(4):S490–S490.
- Klose H, Chin KM, Ewert R, Gall H, Parambil J, Poch D, Seyfarth H-J, Axelsen LN, Hsu Schmitz S-F, Stein C, et al. 2021. Temporarily switching from oral to intravenous selexipag in patients with pulmonary arterial hypertension: safety, tolerability, and pharmacokinetic results from an open-label, phase III study. Respir Res. 22(1):34–42.
- Pang CY, Mak JW, Ismail R, Ong CE. 2012. In vitro modulatory effects of flavonoids on human cytochrome P450 2C8 (CYP2C8). Naunyn Schmiedebergs Arch Pharmacol. 385(5):495–502.
- Projean D, Morin PE, Tu TM, Ducharme J. 2003. Identification of CYP3A4 and CYP2C8 as the major cytochrome P450 s responsible for morphine N-demethylation in human liver microsomes. Xenobiotica. 33(8):841–854.
- Sardana M, Moll M, Farber HW. 2016. Pharmacokinetic drug evaluation of selexipag for the treatment of pulmonary arterial hypertension. Expert Opin Drug Metab Toxicol. 12(12):1513–1520.
- Xie S, Shi L, Chen J, Xu RA, Ye X. 2020. Simultaneous quantification and pharmacokinetic investigation of selexipag and its main metabolite ACT-333679 in rat plasma by UPLC-MS/MS method – ScienceDirect. J Pharm Biomed Anal. 190:113496–113501.
- Yazıcı O, Güngör H. 2019. Selexipag: a new treatment agent for pulmonary arterial hypertension. Meandros. 20(1):1–6. DOI:10.4274/meandros.galenos.2017.20592.