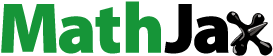
Abstract
Context
Qiangli Wuhu (QLWH) mixture is a concoction approved and registered by Ningxia Medical Products Administration. It has therapeutic effects on various types of pneumonia.
Objective
To clarify the mechanisms of QLWH in treating pneumonia.
Materials and methods
The potential targets of QLWH in the treatment of pneumonia were predicted by network pharmacology. Male, Institute of Cancer Research (ICR) mice were randomly divided into five groups of 12 mice, control, vehicle, QLWH (10 and 20 mg/kg) and dexamethasone (DXM), and orally treated twice daily with normal saline, QLWH or DXM. The pneumonia model was established by tracheal instillation of lipopolysaccharide (LPS). After treatment five days, ELISA, H&E staining and Western blot were used to investigate protective effects of QLWH.
Results
Nine hundred and ninety-four active ingredients were found through network pharmacology, corresponding to 135 targets for the treatment of pneumonia; compared to the vehicle group, QLWH (10 and 20 mg/kg) significantly decreased the levels of TNF-α (14.3% and 28.8%), IL-1β (23.9% and 42.8%) and IL-6 (13.2% and 16.1%), increased the levels of IL-10 (134.3% and 172.9%); in terms of mechanism, QLWH down-regulated TLR4/NF-κB/NLRP3 axis related proteins in lung tissue of pneumonia model mice (p < 0.05).
Discussion and conclusions
This study combined network pharmacology and animal experiments, providing effective evidence for the clinical promotion of QLWH. Meanwhile, it is of significance for further development.
Introduction
Respiratory diseases are common and occur frequently. They can severely endanger human health, and in recent years, they have posed a tremendous burden on global health and the economy (Bhutta and Das Citation2013). It has been reported that respiratory diseases rank third in terms of the global mortality rate for non-communicable diseases. Pneumonia is one of the most common forms of respiratory disease, with about 120 million new cases and 1.3 million deaths every year (Kulkarni et al. Citation2022). The number of deaths caused by severe pneumonia accounts for 8.7% of all deaths each year (Bryce et al. Citation2005). Therefore, pneumonia has become a major health problem, requiring urgent attention (Torres et al. Citation2021). Pneumonia refers to the inflammation associated with the terminal airway, alveoli and lung parenchyma. It can be caused by infection, physical and chemical factors, immune injury, allergy and drug use; however, infection is the most common cause of pneumonia (Cilloniz et al. Citation2022). Nearly, all pathogenic microorganisms and parasites can cause pneumonia (Mulholland Citation2018). The main clinical manifestations are fever, cough, dyspnoea and moist rales in the lung, and the most common pathogens include Streptococcus pneumoniae, Haemophilus influenzae, Mycoplasma pneumoniae, Chlamydia pneumoniae and Klebsiella pneumoniae (Ottosen and Evans Citation2014). In recent years, the excessive prophylactic use of antibiotics, combined use of multiple antibiotics, increased use of high-level antibiotics, empirical clinical medication and frequent replacement of antibiotics have contributed to a significant increase in drug resistance rates (Rodrigues Citation2017; Wunderink and Waterer Citation2017; Ho and Ip Citation2019). Long-term use of antibiotics can also cause side effects in patients (Mathur et al. Citation2018; Graham et al. Citation2022). Therefore, researchers have and are continuing to explore various avenues, including examining the efficacy of traditional Chinese medicine (TCM) for the treatment of pneumonia, to reduce dependence on antibiotics (Xi et al. Citation2020; Ling et al. Citation2021).
Qiangli Wuhu (QLWH) mixture is a medical concoction approved and registered by Ningxia Medical Products Administration. This mixture has been formulated by modifying the classic traditional prescriptions of Chinese medicine, ‘Ma Xing Shi Gan Decoction’ and ‘Sangbaipi Decoction.’ The following constituents of the original prescriptions are retained in QLWH mixture: Ephedrae herba, Semen armeniacae amarum, Mori cortex, Radix Scutellariae and Pinelliae Rhizoma Praeparatum Cum Alumine. In addition, the following constituents have been added to the mixture: Bombyx batryticatus, Pheretima, Stemonae Radix, Folium Eriobotryae, Descurainiae Semen Lepidii Seneb, Uncariae Ramulus Cum Uncis and Fritillariae Thunbergii Bulbus (Tian et al. Citation2016). In the past 20 years of clinical application, QLWH mixture has been successfully used to treat cough, acute and chronic bronchitis, pneumonia and other diseases caused by respiratory tract infections. The mixture can treat several types of pneumonia, especially in children with phlegm-heat sealed lung (a type of symptom recognized in TCM) pneumonia (Wang and Li Citation2020). However, its mechanisms of action remain elusive.
With a collaboration between computer network technology and life sciences and the rapid development of interdisciplinary technology, network pharmacology integrates the academic theories of systems biology and pharmacology (Wu and Wu Citation2015). Computer network technology is used to summarize and analyse the results from previous studies into molecular drug structures, target characteristics and network interactions of genes, proteins and metabolites. This is done to establish a variety of openly accessible scientific databases (Zhang et al. Citation2017). Computer network technology provides an opportunity to study the multi-target mechanisms of action of TCM. Additionally, it provides a sense of direction for further exploring potential mechanisms of action of TCM compound mixtures in the treatment of pneumonia (Li and Zhang Citation2014).
In this study, we first retrieved the effective components and action targets of QLWH mixture to interactively analyse the correlation between the mixture and the therapeutic targets of pneumonia. We then identified these therapeutic targets of QLWH mixture and verified the results through controlled experiments. This study aimed to lay a foundation for further exploration of the mechanisms of action of QLWH mixture against pneumonia, providing a basis for its extensive clinical application.
Materials and methods
Network pharmacology
The databases used for drug target screening were as follows: TCMSP database (http://tcmspw.com/tcmsp.php), TCMID database (http://119.3.41.228:8000/tcmid/), PubChem database (https://pubchem.ncbi.nlm.nih.gov/) and Swiss Target Prediction database (http://www.swisstargetprediction.ch/). Using a filter condition for OB (oral drug bioavailability) >30%; DL (class medicine) >0.18.
The databases used for disease target screening were as follows: DisGeNET database (https://www.disgenet.org/), GeneCards database (https://www.genecards.org/), TTD database (http://db.idrblab.net/ttd/) and STRING database (https://string-db.org/). From the information obtained from the database, the obtained target name was input into the UniProt database to obtain the gene name of the target (Gene name). Drawing and analysis software: Venny2.1 online software mapping tools (https://bioinfogp.cnb.csic.es/tools/venny/), Cytoscape3.7.2 and R3.6.1 software.
Experimental materials
Male Institute of Cancer Research (ICR) mice weighing 20 ± 2 g were provided by the Animal Experimental Center of Ningxia Medical University. Mice were adapted to a moderate environment of 21–25 °C and humidity of 50 ± 20% for three days, with 12 h of light per day. Adequate food and water were provided. All animal experiments were carried out in accordance with the recommendations of the institutional and national guidelines for animal care and use. The protocol was approved by the Ethics Committee of Animal Experiments of Ningxia Medical University, Yinchuan, China (no. IACUC-NYLAC-20, 10 January 2021).
Qiangli Wuhu mixture was produced by the Chinese Medicine Research Center of Ningxia (batch number: 20201217, crude drug content: 1.05 g/mL). Lipopolysaccharide (LPS) was purchased from Sigma-Aldrich (St. Louis, MO). Dexamethasone (DXM) was supplied by Meilunbio (Dalian, China). Enzyme-linked immunosorbent assay (ELISA) kits (TNF-α, IL-6, IL-1β, IL-4 and IL-10) were purchased from Servicebio (Wuhan, China). Antibodies against TLR4 (1:500), MyD88 (1:1000) and NLRP3 (1:1000) were purchased from Abcam (Cambridge, MA). Antibodies against IKK-beta (1:500), P-IKK-beta (1:500), IκB-α (1:1000), p-IκB-α (1:1000), NF-κB (1:1000), p-NF-κB (1:1000), GAPDH (1:2000), α-tubulin (1:1000) and HRP-anti-rabbit secondary antibody (1:5000) were purchased from ProteinTech (Wuhan, China). SUPER ECL Assay Kits and bicinchoninic acid (BCA) Protein Assay Kits were purchased from Nanjing KeyGen Biotech Co., Ltd. (Nanjing, China).
Establishment of pneumonia model, grouping and treatment of mice
The daily dose of QLWH mixture for adults weighing 60 kg is 100 mL, and the crude drug concentration supplied is 1.01 g/mL. According to the formula, the daily equivalent dose for mice was about 20 g/kg (Janhavi et al. Citation2022).
This was set as the high dose. The low dose was set at 10 g/kg. DXM was used as the positive control drug at a dose of 1.8 mg/kg.
Mice were randomly divided into five groups (n = 12), including a no-treatment control group (control), pneumonia model group (vehicle), QLWH mixture low dose group (10 g/kg), QLWH mixture high dose group (20 g/kg) and DXM positive control group (DXM). Modelling was performed by endotracheal infusion of LPS at 10 mg/kg, 5 μL at a time, for three consecutive days. After the animal model was established, various treatments were intragastrically administered twice a day for five days. Vehicle mice were administered an equal volume of 0.9% normal saline.
ELISA
Mice were anaesthetized by an intraperitoneal injection of 10% chloral hydrate; then the eyeballs of experimental mice were clipped with tweezers and quickly removed, and the blood from the orbit was extracted into anticoagulant tubes. The plasma supernatant was then extracted by centrifugation and stored at −80 °C. The serum concentrations of TNF-α, IL-1β, IL-6 and IL-10 were detected with ELISA kits according to the manufacturer’s instructions.
Haematoxylin and eosin staining
The thorax of the mice was exposed after anaesthesia. Phosphate-buffered saline was first perfused through the right ventricle, followed by a 4% paraformaldehyde fixative solution. The right lung was extracted and fixed in 10% formaldehyde solution, paraffin sections were prepared, and haematoxylin–eosin staining was performed to observe histopathological changes in the lung, such as the degree of damage to alveolar epithelial cells, inflammatory cell infiltration and alveolar wall thickening.
Western blot
Lung tissues of mice were collected and stored at −80 °C for later use. Radio-immune precipitation assay lysate was added to the lung tissue to split homogenate, and protein was extracted and determined by BCA assay. The protein was concentrated and then transferred to polyvinylidene fluoride membrane by sodium dodecyl sulphate-polyacrylamide gel electrophoresis, then sealed with 5% skim milk at room temperature for 2 h, and finally incubated at 4 °C overnight with the primary antibody. The next day, the gel was rinsed four times with Tris-buffered saline/tween 20 (TBST) for 8 min, incubated at room temperature with secondary antibody for 90 min, and then rinsed three times with TBST. The developing reaction was performed by SUPER ECL Assay Kit, and images were collected. Then, ImageJ software (Bethesda, MD) was used to analyse grey values to assess protein expression.
Statistical analysis
Data were analysed, and graphs were generated using SPSS version 23.0 (SPSS Inc., Chicago, IL) and GraphPad Prism 7 software (La Jolla, CA). Data are shown as the mean ± standard error of the mean. One-way ANOVA was used to perform statistical analysis, followed by Tukey’s post hoc pairwise test, and a p< 0.05 was considered the threshold for statistical significance.
Results
Network pharmacology
Screening of active ingredients and their targets
The compound drugs constituents were searched in the TCMSP database, and the ingredients not included in the TCMSP database were searched by TCMID as potential active ingredients. The structures of the components were obtained using the PubChem database and imported into the Swiss Target Prediction database. Targets with predicted scores greater than 0 were selected as drug targets.
OB ≥ 30% and DL ≥ 0.18 were set in the TCMSP database. The active components of Ephedrae herba, Armeniacae semen amarum, Mori cortex, Scutellariae radix, Bombyx batryticatus, Pheretima, Stemonae Radix, Eriobotryae Folium, Descurainiae Semen Lepidii Seneb, Uncariae Ramulus Cum Uncis, Fritillariae Thunbergii Bulbus and Pinelliae Rhizoma Praeparatum Cum Alumine were retrieved. Subsequently, 203 potentially active compounds and 1129 drug targets were screened using the Swiss Target Prediction database ().
Table 1. Active components and targets of Qiangli Wuhu mixture.
Screening of pathogenic targets
A total of 854 disease targets were obtained using ‘pneumonia’ as the keyword in DisGeNET, GeneCards and TTD databases.
Screening of drug-disease common targets
Screening yielded 1129 drug targets and 854 disease targets; these targets were fed to the Venny2.1 online software mapping tool platform to obtain a Venn diagram, and 135 drug-disease common targets were identified after intersection ().
Construction of network diagram
Cytoscape 3.7.2 software was used to construct a network diagram of ‘drug-component-target-disease’ and the Network Analyzer function was used to analyse the main active ingredients of TCM compounds. In total, 203 potential active ingredients and 135 drug-disease common targets in TCM compounds were input to the Cytoscape software. Isolated components without intersection with targets were deleted, and the network diagram of the ‘drug-component-target-disease’ interaction was drawn ().
Protein–protein interaction network diagram
The above 135 common targets were input into the STRING database for retrieval. The protein type was set as ‘Homo sapiens,’ and the minimum interaction threshold was 0.4. The network relationship data of target interaction were obtained and imported into Cytoscape software to draw the protein–protein interaction (PPI) network diagram ().
Core target screening based on topology analysis
The PPI network was imported into Cytoscape 3.7.2, and topology analysis was conducted using the Network Analyzer tool. Genes with values greater than average scores were selected as core targets, and bar charts were drawn for the first 30 targets using R 3.6.1 ().
Gene Ontology enrichment analysis
Bioconductor bioinformatics resources, Gene Ontology (GO) and Kyoto Encyclopedia of Genes and Genomes (KEGG), were used to conduct functional enrichment analysis of key target genes based on R software (p value <0.05, Q value <0.05), and the results were outputted in the form of a bubble graph ().
Figure 5. Gene Ontology enrichment analysis of Qiangli Wuhu mixture used to treat pneumonia. BP: biological processes; CC: cell component; MF: molecular function.
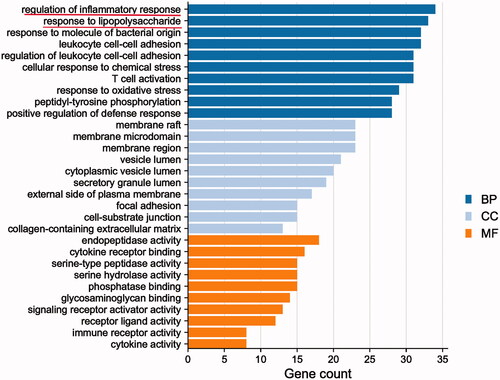
The biological process, cell component and molecular function of 135 common targets were selected by GO analysis with an R language algorithm. GO results showed that the intersection gene set was enriched in 2387 biological process pathways, including bacterial infection, LPS response, inflammatory response and T cell activation; the intersection gene set was enriched in 62 cell components, and the set of intersected genes was enriched in 130 molecular function-related processes, mainly including cytokine receptor and primary lysosomal sac function.
KEGG enrichment analysis
A total of 158 KEGG pathways were obtained by running 135 common targets with an algorithm in the R programming language. The first 20 results formed a bar graph with KEGG functional enrichment (). The results showed that the involved pathways highly correlated with the TNF, TLR, phosphoinositide 3-kinases (PI3K) and the mitogen-activated protein kinase (MAPK) signalling pathways. Considering that the biological processes involved in treating pneumonia by QLWH mixture are highly correlated with inflammatory response, LPS reaction and bacterial infection, it is intuitive that TNF-α, TLR and NF-κB signalling pathways were highly related to the involved signalling pathways.
Effect of Qiangli Wuhu mixture on serum inflammatory factors of mice with LPS-induced pneumonia
Mice were administered tracheal drops of LPS for three consecutive days, and intragastric administration of a strong Wuhu mixture (10 and 20 g/kg) was conducted to detect its effects on serum inflammatory factors namely, TNF-α, IL-1β, IL-6 and anti-inflammatory factor IL-10. The results showed that serum levels of TNF-α, IL-1β and IL-6 in model mice were significantly increased. In contrast, TNF-α, IL-1β and IL-6 levels were significantly decreased by intragastric treatment with different dosages of QLWH mixture (). At the same time, QLWH mixture effectively increased the serum level of the anti-inflammatory factor IL-10 ().
Haematoxylin and eosin staining
The lung tissues of control mice were stained, revealing that cells were uniform in size without inflammatory cell infiltration (). The vehicle group had a few intact alveolar structures with uneven alveolar cell size and narrowed airways; inflammatory infiltration, vascular hyperplasia and fibrosis with small blood vessels, lymphocytes and a few macrophages could be observed (). The lung tissue lesions of mice in the high and low dose QLWH mixture groups were slight, with interstitial lung thickening and the presence of individual inflammatory cells, which had been significantly improved compared with the pathological vehicle group (). The DXM group was used as the positive control ().
Effect of Qiangli Wuhu mixture on inflammatory factors and NLRP3/TLR4/NF-κB signalling pathway in mice with LPS-induced pneumonia
According to the results of network pharmacology analysis, NLRP3 is an important protein in the lung inflammation process. In addition, TLR4 is the LPS recognition receptor, while MyD88 is the connector protein of this signalling pathway. As shown in , the expressions of NLRP3, TLR4 and MyD88 were significantly upregulated in the vehicle group compared to the control group; however, the levels of NLRP3, TLR4 and MyD88 were effectively inhibited by the administration of QLWH mixture. Considering that MyD88 signal transduction mediates the NF-κB pathway (Oeckinghaus and Ghosh Citation2009), we evaluated the effect of QLWH mixture on the NF-κB pathway by Western blot analysis. As shown in the results (), LPS stimulation significantly increased the level of p-IκB-α/IκB-α, p-IκB-α/IκB-α and p-NF-κB/NF-κB. However, the levels of p-IκB-α/IκB-α, p-IκB-α/IκB-α and p-NF-κB/NF-κB in mice were effectively reduced by intragastric administration of different doses of QLWH mixture.
Figure 9. Effect of Qiangli Wuhu mixture on NLRP3/TLR4/MyD88 pathway in mice with LPS-induced pneumonia. (A) Protein levels of NLRP3, MyD88 and TLR4 were detected by Western blot. (B–D) Protein levels of NF-kappa B, p-NF-kappa B, IκB-α, p-IκB-α, IKK-β and p-IKK-β were detected by Western blot. #p< 0.05 compared with the control group, *p< 0.05 compared with the vehicle group.
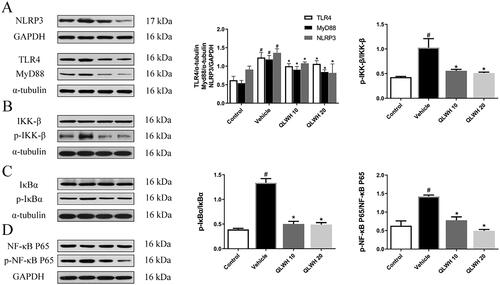
Discussion
The British Thoracic Society guidelines recommend that a clinical diagnosis of pneumonia should be explicitly treated with antibiotics, as bacterial and viral pneumonia cannot be reliably distinguished (Cardinale et al. Citation2013). Some reports show that viral and bacterial co-infection of pneumonia accounted for 30% of the cases. In addition, in the clinical treatment of pneumonia, targeted antibacterial treatment is difficult due to the lag in identifying and diagnosing pathogenic bacteria (Wallihan and Ramilo Citation2014). Therefore, the use of antibiotics is mainly based on the age of patients, the most likely pathogens, and the experience of clinicians (Das and Singh Citation2013): penicillin, cephalosporin and macrolides are the most frequently used antibiotics (Mandell et al. Citation2007). The increase in bacterial resistance renders the treatment of pneumonia difficult, and the abuse of antibiotics also implies various side effects, such as liver and kidney damage, gastrointestinal symptoms, double infection and allergy (Chen et al. Citation2021).
In the treatment of pneumonia, TCM has many functions, such as inhibiting virus proliferation, promoting the expression of interferons, inhibiting inflammation and enhancing immunity. Viruses with RNA as genetic material, such as influenza and coronaviruses, are more prone to mismatches and mutations during replication than DNA viruses. Their high variability makes it harder to develop vaccines and easier for the viruses to develop resistance to single chemicals (Woolhouse et al. Citation2016). Chinese herbal medicine and compound medicine have the characteristics of a multi-component and multi-pathway complex network. Therefore, drug resistance is relatively rare in TCM clinical practice. Increasingly, evidence suggests that Chinese medicine exerts definite therapeutic activity with few adverse reactions (Huang et al. Citation2021). Thus, Chinese medicine offers good curative effects and is significantly safe in treating pneumonia (Ma et al. Citation2018). In nearly 20 years of clinical use, QLWH mixture yielded significant curative effects in children with bronchopneumonia with an effective rate of 97.30%; however, its mechanism of action remains unclear.
The systematic view of network pharmacology resembles the holistic view of TCM, which embodies the viewpoint of system theory. Information about drug targets enables the effective treatment of a disease using drugs. In this study, 994 active ingredients were found through network pharmacology, corresponding to 135 targets for the treatment of pneumonia, reflecting the characteristics of TCM compound mixture with multiple targets. Topological analysis of the PPI network revealed that the primary core target genes affected were TNF-α, IL-6, IL-1β, NF-κB, NLRP3 and other inflammatory factors. KEGG enrichment analysis showed that the TLR, the NF-κB, T cell receptor and NLR signalling pathways highly correlate with the treatment of pneumonia by QLWH mixture.
NF-κB is mainly involved in immune and inflammatory responses and can induce the expression of downstream inflammatory cytokines such as TNF-α and IL-6 to regulate the inflammatory response in pathological conditions (Koch et al. Citation2014). TNF-α and TNF receptor 2 are expressed on immune cells and regulate T cell survival, activation and proliferation. One of the main causes of lung infection by Gram-negative bacteria is the ability of LPS present in the cell wall outer membrane to stimulate immune and structural cells effectively (Tang et al. Citation2021). The introduction of LPS into the systemic circulation may lead to harmful, systemic inflammatory immune responses (Coimbra et al. Citation2006). Therefore, LPS is often used to establish animal inflammatory models (Sender and Stamme Citation2014; Whitfield and Trent Citation2014).
Reliable evidence indicates that LPS tracheal drip can establish a pneumonia model in mice (Niu et al. Citation2017; Chang et al. Citation2018; Tao et al. Citation2019). The NLRP3 inflammasome is mainly expressed in neutrophils, macrophages, monocytes and dendritic cells and induces a primary immune response against pathogenic invasion. When TLR and NLR are over activated, it can lead to excessive release of IL-1β and IL-18, which can cause a continuous and massive inflammatory response, further aggravating tissue damage. TLR4 recognizes and binds to Gram-negative bacterial LPSs, leading to the activation of nuclear transcription factor-κB and expression of inflammatory factors such as IL-6 and TNF-α through MyD88-dependent and independent signalling pathways (Oeckinghaus and Ghosh Citation2009). It has been shown that TLR2 and TLR4 expression in patients with pneumonia is significantly higher than in healthy subjects, suggesting that the increase is related to pneumonia (Hauber et al. Citation2005). Therefore, TLR4 can be considered an indicator for evaluating pneumonia (Aderem and Ulevitch Citation2000), and the TLR4/NF-κB/NLRP3 axis can be used as a key pathway to studying pneumonia.
We used an LPS tracheal drip method to establish a pneumonia model in mice and conducted confirmatory experiments based on bioinformatics predictions and network pharmacology analysis. The results showed that QLWH mixture significantly improved the lung tissue of mice with LPS-induced pneumonia and reduced the expression of serum inflammatory factors. Furthermore, results of the Western blot analysis showed that the therapeutic effect of QLWH mixture might be mediated by the inhibition of the TLR4/NF-κB/NLRP3 axis. In addition, network pharmacology results predicted that the effects of QLWH mixture against pneumonia might be associated with the MAPK signalling pathway, apoptosis, intestinal immune network for IgA production, and inflammatory bowel disease. This suggests that the effect of QLWH mixture against pneumonia may also involve intestinal mucosal immunity, energy regulation and apoptosis. In follow-up studies, we will further explore other related targets and pathways. Although network pharmacology reveals the drug–gene–disease relationships by analysing network regulatory effects and PPI networks, its principle is similar to the overall concept underlying TCM (Huang et al. Citation2021). However, network pharmacology also has some limitations. First, sufficient data for TCM clinical and basic research are currently lacking, and only a few dedicated pharmacological databases exist. Second, the weights of each specific mixture component are uncertain, and the results are often redundant and not specific. Therefore, there is scope for improving the analytic algorithm.
Conclusions
In the current study, the network pharmacology approach involving data integration, network construction and enrichment analysis was used to predict the mechanism of QLWH mixture in treating pneumonia and animal experiments have validated the results of bioinformatics analysis. QLWH mixture has a strong protective effect on LPS-induced pneumonia mice through inhibiting TLR4/NF-κB/NLRP3 axis. Taken together, this study provides an exact basis for the clinical use of QLWH mixture and is of great significance for the secondary development.
Author contributions
Jie Tian and Long-Cheng Wang developed hypotheses and conceived the project; Xiao-Long Wang and Fei Chen designed the experiments and performed animal experiments; Yun Tian and Xiao-Long Wang wrote the manuscript; Li Ma and Chao-Yun Pan analysed the data and validated it; Yan-Ping Wang was involved in the administration of the project and in acquiring funding. All authors read and approved the final manuscript.
Disclosure statement
The authors report that there are no competing interests to declare.
Data availability statement
The datasets used and/or analysed during the current study are available from the corresponding author on reasonable request.
Additional information
Funding
References
- Aderem A, Ulevitch RJ. 2000. Toll-like receptors in the induction of the innate immune response. Nature. 406(6797):782–787.
- Bhutta ZA, Das JK. 2013. Global burden of childhood diarrhea and pneumonia: what can and should be done? Pediatrics. 131(4):634–636.
- Bryce J, Boschi-Pinto C, Shibuya K, Black RE. 2005. WHO estimates of the causes of death in children. Lancet. 365(9465):1147–1152.
- Cardinale F, Cappiello AR, Mastrototaro MF, Pignatelli M, Esposito S. 2013. Community-acquired pneumonia in children. Early Hum Dev. 89(Suppl. 3):S49–S52.
- Chang JS, Lin HJ, Deng JS, Wu WT, Huang SS, Huang GJ. 2018. Preventive effects of velvet antler against lipopolysaccharide-induced acute lung injury in mice by inhibiting MAPK/NF-kappaB activation and inducing AMPK/Nrf2 pathways. Evid Based Complement Alternat Med. 2018:2870503.
- Chen X, Pan D, Chen Y. 2021. The drug resistance of multidrug-resistant bacterial organisms in pediatric pneumonia patients. Am J Transl Res. 13:3309–3315.
- Cilloniz C, Pericas JM, Rojas JR, Torres A. 2022. Severe infections due to respiratory viruses. Semin Respir Crit Care Med. 43(1):60–74.
- Coimbra R, Melbostad H, Loomis W, Porcides RD, Wolf P, Tobar M, Hoyt DB. 2006. LPS-induced acute lung injury is attenuated by phosphodiesterase inhibition: effects on proinflammatory mediators, metalloproteinases, NF-kappaB, and ICAM-1 expression. J Trauma. 60(1):115–125.
- Das RR, Singh M. 2013. Treatment of severe community-acquired pneumonia with oral amoxicillin in under-five children in developing country: a systematic review. PLOS One. 8(6):e66232.
- Graham FF, Finn N, White P, Hales S, Baker MG. 2022. Global perspective of Legionella infection in community-acquired pneumonia: a systematic review and meta-analysis of observational studies. Int J Environ Res Public Health. 19(3):1907.
- Hauber HP, Tulic MK, Tsicopoulos A, Wallaert B, Olivenstein R, Daigneault P, Hamid Q. 2005. Toll-like receptors 4 and 2 expression in the bronchial mucosa of patients with cystic fibrosis. Can Respir J. 12(1):13–18.
- Ho J, Ip M. 2019. Antibiotic-resistant community-acquired bacterial pneumonia. Infect Dis Clin North Am. 33(4):1087–1103.
- Huang K, Zhang P, Zhang Z, Youn JY, Wang C, Zhang H, Cai H. 2021. Traditional Chinese medicine (TCM) in the treatment of COVID-19 and other viral infections: efficacies and mechanisms. Pharmacol Ther. 225:107843.
- Janhavi P, Divyashree S, Sanjailal KP, Muthukumar SP. 2022. DoseCal: a virtual calculator for dosage conversion between human and different animal species. Arch Physiol Biochem. 128(2):426–430.
- Koch L, Frommhold D, Buschmann K, Kuss N, Poeschl J, Ruef P. 2014. LPS- and LTA-induced expression of IL-6 and TNF-alpha in neonatal and adult blood: role of MAPKs and NF-kappaB. Mediators Inflamm. 2014:283126.
- Kulkarni D, Wang X, Sharland E, Stansfield D, Campbell H, Nair H. 2022. The global burden of hospitalisation due to pneumonia caused by Staphylococcus aureus in the under-5 years children: a systematic review and meta-analysis. EClinicalMedicine. 44:101267.
- Li S, Zhang B. 2014. Traditional Chinese medicine network pharmacology: theory, methodology and application. Chin J Nat Med. 11(2):110–120.
- Ling X, Sun X, Kong H, Peng S, Yu Z, Wen J, Yuan B. 2021. Chinese herbal medicine for the treatment of children and adolescents with refractory mycoplasma pneumoniae pneumonia: a systematic review and a meta-analysis. Front Pharmacol. 12:678631.
- Ma X, Hu M, Wang H, Li J. 2018. Discovery of traditional Chinese medicine monomers and their synthetic intermediates, analogs or derivatives for battling P-gp-mediated multi-drug resistance. Eur J Med Chem. 159:381–392.
- Mandell LA, Wunderink RG, Anzueto A, Bartlett JG, Campbell GD, Dean NC, Dowell SF, File TM Jr, Musher DM, Niederman MS, et al. 2007. Infectious Diseases Society of America/American Thoracic Society consensus guidelines on the management of community-acquired pneumonia in adults. Clin Infect Dis. 44(Suppl. 2):S27–S72.
- Mathur S, Fuchs A, Bielicki J, Van Den Anker J, Sharland M. 2018. Antibiotic use for community-acquired pneumonia in neonates and children: WHO evidence review. Paediatr Int Child Health. 38(Suppl. 1):S66–S75.
- Mulholland K. 2018. Problems with the WHO guidelines for management of childhood pneumonia. Lancet Glob Health. 6(1):e8–e9.
- Niu X, Liu F, Li W, Zhi W, Zhang H, Wang X, He Z. 2017. Cavidine ameliorates lipopolysaccharide-induced acute lung injury via NF-kappaB signaling pathway in vivo and in vitro. Inflammation. 40(4):1111–1122.
- Oeckinghaus A, Ghosh S. 2009. The NF-kappaB family of transcription factors and its regulation. Cold Spring Harb Perspect Biol. 1(4):a000034.
- Ottosen J, Evans H. 2014. Pneumonia: challenges in the definition, diagnosis, and management of disease. Surg Clin North Am. 94(6):1305–1317.
- Rodrigues CMC. 2017. Challenges of empirical antibiotic therapy for community-acquired pneumonia in children. Curr Ther Res Clin Exp. 84:e7–e11.
- Sender V, Stamme C. 2014. Lung cell-specific modulation of LPS-induced TLR4 receptor and adaptor localization. Commun Integr Biol. 7:e29053.
- Tang J, Xu L, Zeng Y, Gong F. 2021. Effect of gut microbiota on LPS-induced acute lung injury by regulating the TLR4/NF-kB signaling pathway. Int Immunopharmacol. 91:107272.
- Tao H, Li N, Zhang Z, Mu H, Meng C, Xia H, Fu L, Xu Y, Zhang S. 2019. Erlotinib protects LPS-induced acute lung injury in mice by inhibiting EGFR/TLR4 signaling pathway. Shock. 51(1):131–138.
- Tian J, Wang Y-P, Ning Y, Wang Z-Y, Xu J. 2016. Optimization of water extraction process of Qiangli Wuhu mixture by orthogonal test. Mod Chin Med. 18:1933–1940.
- Torres A, Cilloniz C, Niederman MS, Menendez R, Chalmers JD, Wunderink RG, van der Poll T. 2021. Pneumonia. Nat Rev Dis Primers. 7(1):25.
- Wallihan R, Ramilo O. 2014. Community-acquired pneumonia in children: current challenges and future directions. J Infect. 69(Suppl. 1):S87–S90.
- Wang H-M, Li Q-R. 2020. Clinical study of Qiangli Wuhu mixture in treating children bronchopneumonia with phlegm-heat sealed lung. Ningxia Med J. 42(2):41–51.
- Whitfield C, Trent MS. 2014. Biosynthesis and export of bacterial lipopolysaccharides. Annu Rev Biochem. 83:99–128.
- Woolhouse ME, Brierley L, McCaffery C, Lycett S. 2016. Assessing the epidemic potential of RNA and DNA viruses. Emerg Infect Dis. 22(12):2037–2044.
- Wu X-M, Wu C-F. 2015. Network pharmacology: a new approach to unveiling traditional Chinese medicine. Chin J Nat Med. 13(1):1–2.
- Wunderink RG, Waterer G. 2017. Advances in the causes and management of community acquired pneumonia in adults. BMJ. 358:j2471.
- Xi S, Li Y, Yue L, Gong Y, Qian L, Liang T, Ye Y. 2020. Role of traditional Chinese medicine in the management of viral pneumonia. Front Pharmacol. 11:582322.
- Zhang RZ, Yu SJ, Bai H, Ning K. 2017. TCM-Mesh: the database and analytical system for network pharmacology analysis for TCM preparations. Sci Rep. 7(1):2821.