Abstract
Context
Asthma is a common respiratory system disease. Louki Zupa decoction (LKZP), a traditional Chinese medicine, presents a promising efficacy against lung diseases.
Objective
To investigate the pathogenic mechanism of asthma and reveal the intervention mechanism of LKZP.
Materials and methods
Forty-eight female Balb/c mice were randomly divided into 6 groups: normal control group (NC), ovalbumin (OVA)/saline asthma model group, OVA/LL group, OVA/LM group, OVA/LH group and OVA/DEX group (n = 8 per group). The asthmatic mice were modelled through intraperitoneal injecting and neutralizing OVA. LKZP decoction was administrated by gavage at the challenge stage for seven consecutive days (2.1, 4.2 and 8.4 g/kg/day). We investigated the change in lung function, airway inflammation, mucus secretion and TH-1/TH-2-related cytokines. We further verify the activated status of the IL-33/ST2/NF-κB/GSK3β/mTOR signalling pathway.
Results
LKZP was proved to improve asthmatic symptoms, as evidenced by the down-regulated airway resistance by 36%, 58% and 53% (p < 0.01, p < 0.001 vs. OVA/saline group), up-regulated lung compliance by 102%, 114% and 111%, decreased airway inflammation and mucus secretion by 33%, 40% and 33% (p < 0.001 vs. OVA/saline group). Moreover, the content of cytokines in BALF related to airway allergy (such as IgE) and T helper 1/T helper 2 cells (like IL-2, IL-4, IL-5, IL-13, TNF-α and IFN-γ), were also markedly reduced by 13–65% on LKZP intervention groups compared with model group. Mechanistic research revealed that the IL-33/ST2-NF-κB/GSK3β/mTOR signalling pathway was activated in the OVA/saline group and LKZP significantly down-regulated this pathway.
Discussion and conclusion
LKZP improves lung function, airway inflammation, mucus secretion and correct immune imbalance by intervening with the IL-33/ST2-NF-κB/GSK3β/mTOR signalling pathway, presenting a promising therapeutic choice for asthma.
Introduction
Asthma, which affects nearly 18% of the worldwide population (Mattiuzzi and Lippi Citation2020), is a common disease characterized by airway hyperresponsiveness and airway inflammation, and the symptoms of asthma include coughing, wheezing, chest tightness and dyspnoea. Asthmatic patients could be classified according to the potential pathogenesis or variable symptoms (Kuruvilla et al. Citation2019), such as T helper 2-high (TH2-high) asthma or T helper 2-low (TH2-low) asthma (according to the expression of TH2-like cytokines). Currently, the combination of bronchodilators and glucocorticoids aimed at the phenotype is the conventional treatment for asthma, and biological agents, such as Omalizumab, aimed at the endotype have gradually become the second treatment option for asthmatic patients. Although biological agents have a certain relief effect on asthma symptoms, they have adverse side effects (McGregor et al. Citation2019). Therefore, it is one of the most important focuses currently on finding effective treatments with minimal side effects in asthmatics. Given the heterogeneity of asthma, a widely accepted method to study the pathophysiological mechanisms and interventions of asthma is genetics. Previous studies have suggested that interleukin 33 (IL-33), interleukin 1 receptor-like 1 (IL1RL1) and interleukin 4 (IL-4) are independent gene loci related to the occurrence of asthma (El-Husseini et al. Citation2020). According to previous reports, the IL-33/ST2 pathway may be one of the most important pathways in the context of genetics (El-Husseini et al. Citation2020; Saikumar Jayalatha et al. Citation2021).
IL-33, as a member of the IL-1 protein family, is initially expressed as a nuclear factor of high endothelial venules (NF-HEVs) in high endothelial venules (HEVs) of lymphoid organs, and it plays an important role in controlling the phenotype of HEV (Baekkevold et al. Citation2003). Recent research has indicated that IL-33, also known as the sirens, is a key cytokine released by bronchial structural cells (such as bronchial epithelial cells) when a stimulus acts on the airway. IL-33 then combines with its receptor [suppression of tumorigenicity 2 (ST2)] and induces the activation of downstream signalling pathways, thereby inducing a series of asthma-related symptoms (Schmitz et al. Citation2005; Bartemes et al. Citation2012; Cayrol and Girard Citation2018; Stier et al. Citation2019; Vasanthakumar and Kallies Citation2019; Finlay et al. Citation2020). ST2 is found in immune cells and bronchial structural cells (Finlay et al. Citation2020). Previous studies have reported that the IL-33 gene and ST2 gene are susceptibility genes for asthma, especially in childhood asthma and early-onset asthma (El-Husseini et al. Citation2020; Shaban et al. Citation2021). Another clinical study has suggested that the combination of ST2 and IL-33 results in continuous activation of the nuclear factor kappa β (NF-κB) signalling pathway and recruitment of eosinophils to the airway in an aggravated manner (Portelli et al. Citation2020). Additionally, the proteolytic enzymes secreted by neutrophils and mast cells can cleave IL-33 into smaller but more active fragments (Lefrancais et al. Citation2012). This cycle further aggravates asthmatic symptoms. However, in-depth research focussed on the IL-33/ST2 pathway downstream of the pathogenic mechanism has not been conducted. Therefore, one of the objectives of the presented study was to investigate the underlying pathogenic mechanism after the activation of IL-33/ST2 activation to further uncover its diverse role and provide more methods for clinical diagnosis and treatment.
Louki Zupa (LKZP), as traditional Uyghur medicine, is widely used clinically and is composed of Hyssopus officinalis L. (Lamiaceae). and Orris root. Liquid chromatography-tandem mass spectrometry (LC-MS/MS) analysis has identified rosmarinus acid as the main active ingredient of H. officinalis and luteolin, iris and apigenin as the main active ingredients of Orris root through LC-MS/MS analysis (Wuniqiemu et al. Citation2021). Both animal experiments and clinical studies have demonstrated that LKZP has significant effects on treating respiratory diseases. However, due to the complexity of the LKZP components and the diversity of its targets, the exact mechanism of action for treating diseases needs to be further studied.
In the present study, the potential downstream molecules of the IL-33/ST2 pathway were investigated in an acute asthma mouse model. We used pharmacodynamics to investigate LKZP, and the results indicated that LKZP can improve asthmatic symptoms by intervening in the IL-33/ST2-NF-kB/GSK3β/mTOR signalling pathway.
Materials and methods
Experimental animals
Female BALB/c mice (6–8 weeks, body weight of 18 ± 2 g), were purchased from Shanghai Jiesijie Laboratory Animal Co. Ltd. The mice were housed in the animal holding unit building (School of Pharmacy, Fudan University), with a pathogen-free facility and free to standard animal diet and normal drinking water. All animal experiments in this study were approved by the Ethics Committee of Animal Experiments of Fudan University (Authorization number: 2020-HSYY-JS-675).
Asthma model establishment and treatment
Forty-eight female Balb/c mice were randomly divided into 6 groups, 8 in each group, including the normal control group (NC), ovalbumin (OVA)-induced asthma model group (OVA/saline), low dose of LKZP group (OVA/LL, 2.1 g/kg), medium dose of LKZP group (OVA/LM, 4.2 g/kg), high dose of LKZP group (OVA/LH, 8.4 g/kg) (LKZP decoction, provided by Sinopharm Xinjiang Pharmaceutical Co. Ltd.), OVA-induced asthma model group + positive control dexamethasone group (OVA/DEX, 2 mg/kg). As shown in , on days 0, 7 and 14, the mice in the model group and the four intervention groups were injected intraperitoneally with 0.2 mL sterile PBS solution containing 20 µg OVA (grade V, Sigma, Taufkirchen, Germany) and 2 mg aluminium hydroxide (Sigma-Aldrich, St. Louis, MO, USA), the normal group was intraperitoneally injected with an equal dose of sterile PBS. On days 21–27, mice in the model group and intervention groups were inhaled 3% OVA solution through an ultrasonic nebulizer (402AI ultrasonic nebulizer, Yuyue, China) for 30 min each time, and the normal group was aerosolized with the same amount of PBS for 30 min at the same time. After the aerosolization challenge, the intervention of LKZP groups and the dexamethasone group were administrated with 0.2 mL corresponding drug to each mouse by gavage, and each mouse in the normal group and the model group was given an equal dose of PBS per day by gavage.
Figure 1. (A) Experiment protocol for asthma model and treatment. Mice were grouped, sensitized, treated and challenged as described before. LKZP (2.1 g/kg; 4.2 g/kg; 8.4 g/kg) had been given by gavage from day 21 to 27. Within 24 h after the final challenge, mice were sacrificed. (B) Scheme of OVA inhalation.
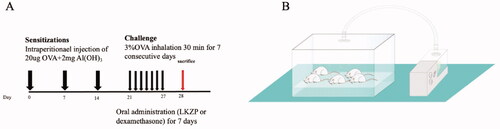
Measurement of Airway hyperresponsiveness (AHR)
Within 24 h after the last nebulization challenge, each mouse was tested for lung function by using the Fine Pointe Resistance and Compliance system (DSI Buxco Electronics, Troy, NY). Each mouse was weighed and injected with 2% pentobarbital sodium (Sigma-Aldrich, St Louis, MO, USA) at a dose of 50 mg/kg. After the mouse was successfully anaesthetized, the trachea was cut and intubated. The mouse was put into a cavity connected with a ventilator, after the breathing is stable, mice were exposed to methacholine (Mch, Sigma-Aldrich; St. Louis, MO) at the increasing dose of 0, 3.125, 6.25 and 12.5 mg/mL to detect lung function. Lung dynamic compliance (Cdyn) and airway resistance (RL) were recorded during this process.
Collection and assessment of serum and bronchoalveolar lavage fluid (BALF)
The blood was taken by removing the eyeballs, The blood volume of each mouse was between 0.3 and 0.5 mL. After standing for 2 h at room temperature, all samples were centrifuged at 2500 g for 25 min at room temperature, all supernatants were aliquoted and stored in at −80 °C for later use. The left lung was lavaged with 0.3, 0.3 and 0.2 mL with pre-cooled PBS and the lavage fluid was collected, the BALF was centrifuged at 4 °C and 500 g for 10 min. The supernatant was aliquoted and stored in at −80 °C for later use. Pellet was resuspended in 50 µL PBS, and the count of total white blood cells (WBC), eosinophils (EOS), lymphocytes (LYM), monocytes (MON), neutrophils (NEU) was measured in an automatic cell counter (Hemavet950 instrument; Drew Scientific Group, UK).
Lung histopathologic examination
The middle lobe of the right lung was taken and fixed in 4% phosphate-buffered formalin, and the remaining lung tissues were frozen at −80 °C for later protein and mRNA analysis. The middle lobe was embedded in paraffin and cut into 4 µm sections to expose the largest surface area of lung tissue in the bronchial plane. Hematoxylin-eosin (HE) staining and Periodic Acid-Schiff (PAS) staining were used to observe airway inflammation and mucus secretion. Image results were recorded under an optical microscope (×200, ×100), the semi-quantitative method was used to score the degree of inflammatory infiltration and secretion of mucus (Cho et al. Citation2001; Bao et al. Citation2018). Five views in each tissue section and three tissue sections in each group were randomly scored in a double-blind screen performed by two independent pathologists with Image-pro plus 6.0 software (Media Cybernetics Inc., Warrendale, PA, USA). In short, the degree of peribranchial inflammation was assessed according to the following histologic grading system: (1) absence of peribranchial inflammatory cells; (2) a few scattered peribranchial inflammatory cells involving less than 25% of the circumference of the bronchus; (3) focal peribranchial inflammatory cell infiltrate not completely surrounding a bronchus (i.e., involving approximately 25–75% of the circumference of the bronchus); (4) 1 definite layer of peribranchial inflammatory cells completely surrounding a bronchus; (5) 2 definite layers of peribranchial inflammatory cells completely surrounding a bronchus; (6) 3 or more layers of peribranchial inflammatory cells completely surrounding a bronchus. In each lung section, the mean peribranchial inflammatory score was calculated by adding the scores of all the individual bronchioles in the lung section and dividing this score by the number of bronchioles present in the lung section. Bule or purple goblet cells were determined as PAS-positive, percentage of PAS-positive cells in total goblet cells was calculated to reflect goblet cell metaplasia. The numbers of PAS-negative and PAS-positive cells were counted around the internal perimeter of the airway by image software. A morphometrical analysis of the volume density of PAS-positive material in lobar bronchi and segmental bronchi was performed to evaluate mucus hypersecretion. The data are expressed as a percentage of PAS-positive material area in the total epithelial area.
Detection of cytokines, IgE
Enzyme-linked immunosorbent assay was used to detect the concentration of Immunoglobulin (IgE) in serum and IL-33, Interleukin-2 (IL-2), Interleukin-4 (IL-4), Interleukin-13 (IL-13), Interleukin-5 (IL-5), tumour necrosis factor α (TNF-a), Interferon-gamma (IFN-γ) in BALF according to the experimental manual (MultiSciences, Hangzhou, China).
Detection of gene expression
Take the upper lobe of the left lung cryopreserved at −80 °C, total RNA was extracted by Trizol (BIO TNT) according to the manufacturer’s instruction, then the total RNA was reverse transcripted into the first strand of cDNA (PrimeScriptTMRT Master Mix (Perfect Real Time) Takara BIO INC) according to the instruction, the concentration and A260/280 of cDNA were checked to judge the purity of the sample extract. Real-time quantitative polymerase chain reaction (qPCR) experiment (TB Green® Premix Ex TaqTM II (Tli RNaseH Plus) Takara BIO INC) was performed to detect the expression level of IL-33, ST2, IL-4, IL-13, IL-5, TNF-a, IFN-γ (primer sequences are presented in ), and the results were analyzed as previously reported (Wei et al. Citation2016).
Table 1. Primer sequences of the genes detected by real-time quantitative polymerase chain reaction.
Immunohistochemical staining
Immunohistochemical staining was used to detect the expression of IL-33 and ST2 in lung tissues according to the experimental manual (Siding, Shanghai, China). Tissue images were captured under an optical microscope (×400), and the semi-quantitative analysis was used to score the degree of IL-33 and ST2 expression (Tang et al. Citation2021). Five brown or tawny views in each tissue section and three tissue sections in each group were randomly selected under the microscope, and integrated optical density (IOD) values of tissue sections were measured by Image-pro plus 6.0 software (Media Cybernetics Inc., Warrendale, PA, USA), and the mean IOD values were considered as the relative expression of IL-33 and ST2.
Western blot test
Lungs were mechanically disrupted to extract total protein and phosphorylated protein according to the manufacturer’s instruction (Beyotime, Beijing, China). Lung proteins were separated by SDS-PAGE and transferred to polyvinylidene fluoride membrane for immunoblotting with antibodies. Antibodies used include Phospho-GSK-3β (Ser9) (1:1000; Cell Signalling Technology), GSK-3β (1:1000; Cell Signalling Technology), NF-κB p65 (1:1000; Cell Signalling Technology), Phospho-mTOR (Ser2448) (1:1000; Cell Signalling Technology), recombinant anti-mTOR (phospho S2448) antibody (1:1000; Abcam), Anti-ST2 antibody-BSA free (1:1000; Abcam), recombinant anti-IL-33 antibody (1:1000; Abcam), Beta Actin Monoclonal Antibody (1:10,000; Proteintech group). The secondary antibody is goat anti-rabbit IgG H&L (HRP) (1:10,000; Beyotime) or goat anti-mouse (1:10,000; Proteintech group). ECL Plus (Solabao) was used to detect chemiluminescence, images were acquired by LAS4000 imaging system (Fujifilm Corporation, Tokyo, Japan). Semi-quantitative analysis was conducted by ImageJ software.
Phosphorylated protein array detection
Commercial human and mouse MAPK pathway phosphorylation array C1 kit (Ray Biotech, USA) was used to semi-quantitatively detect 17 phosphorylated MAPK pathway proteins according to the manufacturer’s protocol. In short, frozen lung tissues were taken, and protease inhibitors and two phosphatase inhibitors (Ray biotech, USA) were added to RIPA Lysis Solution (Strong) (Beyotime, P0013B) to extract total phosphorylated protein. The total protein concentration was determined using the BCA detection kit and standardized to 1 mg/mL in blocking buffer. The phosphorylated array membrane was incubated in a blocking buffer (room temperature, 30 min). The total protein extract was incubated overnight at 4 °C. The membrane was washed and add HRP-anti-rabbit IgG to incubate overnight at 4 °C. The membrane was incubated with chemiluminescence detection buffer (ECL detection reagent) and observed by the LAS4000 imaging system. Phosphorylated protein signal point intensity was calculated by ImageJ software. Quantified and normalized against the positive control point for comparison.
Statistical analysis
SPSS software was used for all data analysis, GraphPad Prism 9 (GraphPad Software, La Jolla, CA, USA) was used for all chart production, and all data were expressed as mean ± standard error of mean (SEM). Comparisons between groups were performed using one-way analysis of variance (ANOVA) with a post hoc least significantly different (LSD) test to calculate the statistical significance of differences between more than two groups. A difference with a p-value < 0.05 was considered statistically significant.
Results
LKZP decoction improved lung function in OVA-induced asthma mouse model
The lung function of mice was tested within 24 h after conducting the last OVA challenge, as shown in . The basic values of airway resistance (RL) and lung compliance (Cdyn) were excited by PBS, meanwhile, Mch was applied to detect the RL and Cdyn. Compared with the NC group, the asthma mice presented an obvious increase in RL [p < 0.001 at the dose of 25 mg/mL, ] and a decrease in Cdyn [], indicating the successful establishment of OVA-induced asthma mouse model. The stimulation of Mch decreased in the three treatment groups and OVA/DEX group. The deviation of ameliorating effects on RL between the LKZP intervention groups and the model group was observed with 12.5 mg/mL of Mch, and the statistical difference was presented in 25 mg/mL [p < 0.01 or p < 0.001, ]. OVA/LM and OVA/LH groups possess a better effect on improving lung function than OVA/LL group. The Cdyn decreased among all groups while no significant difference among normal control, asthma model, LKZP treatment and OVA/DEX groups [].
Figure 2. Effects of LKZP on lung function to Mch in asthma mice. (A) Increase of RL (%); (B) Decrease of Cdyn (%); (C) Increase of RL (%) (Mch:25 mg/mL); (D) Decrease of Cdyn (%) (Mch:25 mg/mL). The data are presented as the mean ± SEM. n = 3–5 mice/group. #p < 0.05; ##p < 0.01; ###p < 0.001 vs. normal control group. *p < 0.05; **p < 0.01; ***p < 0.001 vs. OVA/saline group. Ns: not significant.
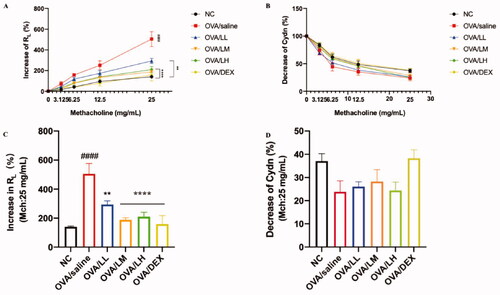
LKZP decoction diminished inflammatory cells and cytokines levels in serum and BALF of OVA-induced mouse asthma model
The excessive infiltration of inflammatory cells and elevated production of IgE are two typical features that happen in an asthma attack period. As shown in , we detected the total inflammatory cells and populations in BALF, and the content of IgE in serum was tested by ELISA. Results indicated that the number of total WBC and EOS in the model group increased significantly compared with the NC group, and the production of IgE also significantly increased in OVA/saline group [p < 0.01 or p < 0.001, ]. These inflammatory changes further indicated the allergic asthma model was established successfully. With LKZP intervention, the number of inflammatory cells in BALF was decreased than OVA/saline group, especially EOS (76, 33 and 22%), one of the indicators of allergic asthma, significant difference can be seen between OVA/LL group and OVA/saline group (p < 0.001). The content of IgE was decreased among LKZP (35, 56 and 54%) and DEX treatment groups (p < 0.05, p < 0.01 or p < 0.001). Interestingly, as opposed to the result of EOS, OVA/LM and OVA/LH groups showed markedly reduced concentration of IgE than the OVA/LL group.
Figure 3. Effects of LKZP on ameliorating inflammatory cells and cytokines. (A) Leukocytes in BALF. (B) Content of IgE in serum. (C–I) Content of IL-33, IL-2, IL-4, IL-5, IL-13, TNF-α and IFN-γ in BALF. The data are presented as the mean ± SEM. n = 4–5 mice/group. #p < 0.05; ##p < 0.01; ###p < 0.001 vs. normal control group. *p < 0.05; **p < 0.01; ***p < 0.001 vs. OVA/saline group.
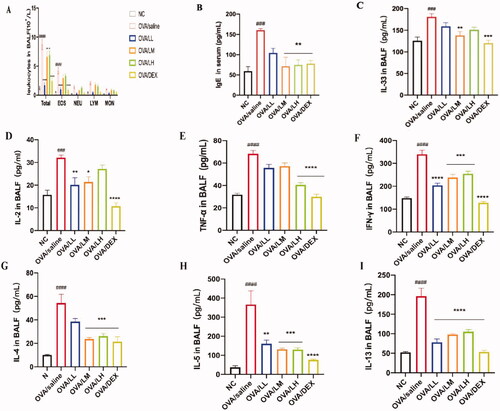
To determine the change of IL-33 in BALF, we detected the concentration of IL-33, as depicted in . The result demonstrated that the expression level of IL-33 increased sharply in OVA/saline group compared with the NC group. In addition, the expression level of IL-33 significantly decreased in treatment groups, especially in OVA/LM (25%) and OVA/DEX groups (p < 0.01 or p < 0.001). However, the concentration of IL-33 in the OVA/LL and OVA/LH groups was not significantly different from the model group.
To explore the intervention of LKZP in inhibiting the activation of both TH1/2 cells, the content of cytokines that are typically derived from these two groups of cells in BALF were tested []. The number of TH1 cell-related cytokines (e.g., IL-2, TNF-α and IFN-γ) in the OVA/saline group were significantly higher than in the NC group (p < 0.001). The statistical results of the number of TH2 cell-related cytokines, such as IL-4, IL-5 and IL-13, showed the same changes as TH1 cell-related cytokines. The results of LKZP and DEX intervention groups showed a markedly reduced effect on these two types of cytokines (p < 0.05, p < 0.01 or p < 0.001), but not in a dose-dependent manner.
LKZP decoction attenuated airway inflammation in OVA-induced mouse asthma model
In order to determine the effects of LKZP on attenuating airway inflammation and mucus secretion, pathological changes in lung tissues were detected by Hematoxylin-eosin (HE) and Periodic Acid-Schiff (PAS) staining, results were shown in , significant inflammatory cell aggregation and mucus secretion were found around the blood vessels and small airways in the model group. However, the degrees of inflammation and mucus secretion were relieved in the LKZP and DEX administration groups []. The OVA/LL and OVA/LM groups possessed better effects than OVA/LH group. The inflammatory pathology score suggested that the change level of inflammatory infiltration between the model group and intervention groups had a statistical difference [p < 0.001, ]. The mucus secretion level and percentage of abnormal goblet cells were remarkably improved in mice treated with LKZP and DEX compared with OVA/saline group [p < 0.001, ].
Figure 4. LKZP attenuated airway inflammation and mucus secretion in OVA-induced asthma mouse. (A) HE staining in lung tissue (×100, ×200), Bar = 100 µm. (B) Periodic Acid-Schiff (PAS) staining in lung tissue (×100, ×200), Bar = 100 µm. (C) Scores of airway inflammation. (D) Percentage of PAS (+) epithelial cells. The data are presented as the mean ± SEM. n = 4–5 mice/group. #p < 0.05; ##p < 0.01; ###p < 0.001 vs. control group. *p < 0.05; **p < 0.01; ***p < 0.001 vs. OVA/saline group.
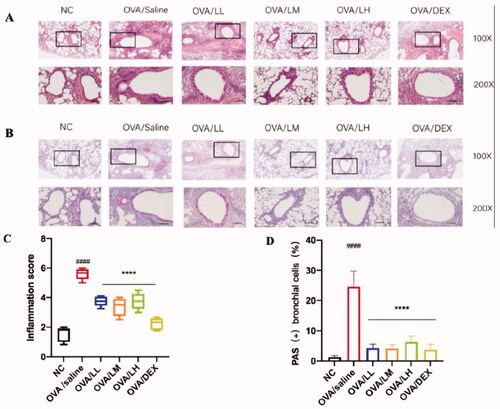
LKZP decoction suppressed the protein expression level of IL-33 and ST2 in OVA-induced mouse asthma model
To further explore the regulatory mechanism of LKZP in asthma, based on the preliminary research result, we selected OVA/LM group to confirm its treatment mechanism for asthma. To research the pathogenic mechanism of the IL-33/ST2 pathway in OVA-induced asthma attacks, we performed an immunohistochemical experiment and western blot experiment to detect the protein expression level of IL-33 and ST2 in mouse lung tissues. The results are presented in . The result of the immunohistochemical experiment revealed that IL-33 and ST2 were almost not expressed in the NC group. However, markedly upregulated expression of them presented around lung tissues and small airways in the OVA/saline group, and significant downregulation of IL-33 and ST2 was also observed in the LKZP treatment group [], significant statistical difference was found between the OVA/LM group and the model group through semi-quantitative analysis [p < 0.001, ]. The expression levels of IL-33 and ST2 were further verified by a western blot experiment; this experiment result demonstrated consistency with the result of the immunohistochemistry test (as shown in , p < 0.01 or p < 0.001). The above results revealed that the IL-33/ST2 pathway is markedly activated in OVA-induced asthmatic mice. We also predict the obvious improvement effectiveness of LKZP on asthma may be due to the inhibition of IL-33/ST2 pathway.
Figure 5. Effects of LKZP on the IL-33/ST2 signalling pathway detected by immunohistochemical (A, B) and western blot (E, F). (A, C) Immunohistochemical results of IL-33 in lung (×400), Bar = 100 µm. (B, D) Immunohistochemical results of ST2 in lung (×400), Bar = 100 µm. (G, H) The relative density quantifications of IL-33 and ST2, respectively. The results were expressed as the ratio of IL-33 and ST2 relative to β-actin, respectively. The data are presented as the mean ± SEM. n = 4 mice/group. #p < 0.05; ##p < 0.01; ###p < 0.001 vs. control group. *p < 0.05; **p < 0.01; ***p < 0.001 vs. OVA/saline group.
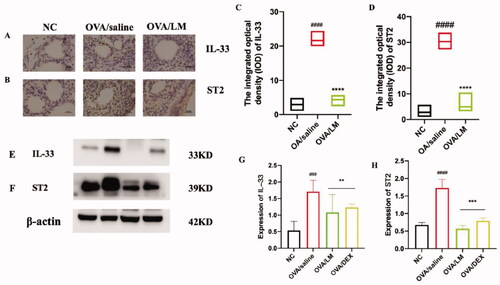
LKZP affects the inflammatory response mediated by the IL-33/ST2-NF-kB/GSK3β/mTOR signalling pathway
To further verify the downstream activated mechanism after the activation of the IL-33/ST2 pathway, based on the related literature, the expression level of total proteins and phosphorylated status of key proteins in the NF-κB/MAPK signalling pathway were detected through a western blot experiment (). Obviously increased expression level of phosphor-NF-κB p65 (pp65) was presented in the OVA/saline group. The expression was effectively suppressed after the intervention of LKZP and DEX []. No significant deviation in the expression of total protein p65 was found no among the NC group, OVA/saline group and intervention group [].
Figure 6. Effects on NF-κB signalling pathway of LKZP. (A) The protein expression of pp65 and p65 in lung tissues were detected by western blot. (B, C) The relative density quantifications of pp65 and p65 respectively. The results were expressed as the ratio of pp65 and p65 relative to β-actin, respectively. The data are presented as the mean ± SEM. n = 4 mice/group. #p < 0.05; ##p < 0.01; ###p < 0.001 vs. control group. *p < 0.05; **p < 0.01; ***p < 0.001 vs. OVA/saline group.
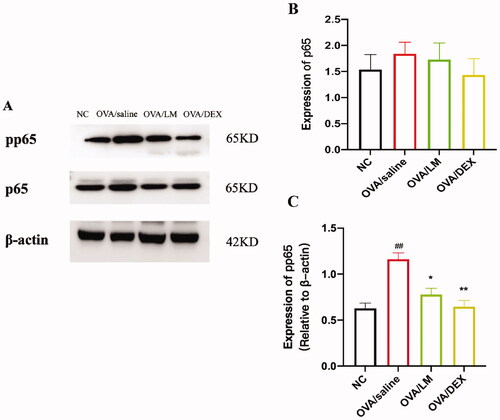
The detailed phosphorylated status of the protein in the MAPK signalling pathway was further analyzed by a commercial human/mouse MAPK protein phosphorylation array. As shown in and , a 1.09-fold increase in the signal intensity of GSK3β in NC array vs. OVA/saline array; a 30% decrease in the signal intensity of GSK3β in OVA/LM array vs. OVA/saline array. A 1.32-fold increase in the signal intensity of mTOR in NC array vs. OVA/saline array; 29% decrease in the signal intensity of mTOR in OVA/LM array vs. OVA/saline array (as shown in and ). compared with the NC group, increased phosphorylation levels of GSK3β (P-S9) and mTOR (P-S2448) were presented in the OVA/saline group, while the inhibited phosphorylated status of GSK3β (P-S9) and mTOR (P-S2448) were demonstrated in the LKZP treatment group []. The above-filtered results were also confirmed by the western blot experiment []. The results displayed that GSK3β and mTOR phosphorylation were remarkably increased in asthmatic mice (p < 0.01), while significantly suppressed by LKZP (p < 0.01). However, different from previous literature reports, our results showed that DEX appeared to be ineffective in inhibiting phosphorylation of GSK3β and mTOR [].
Figure 7. Effects on MAPK signalling pathway of LKZP. (A) 1.09-fold increase in the signal intensity of GSK3β in NC array VS OVA/saline array; 30% decrease of in the signal intensity of GSK3β in OVA/LM array vs. OVA/saline array. (B, ) 1.32-fold increase in the signal intensity of mTOR in NC array vs. OVA/saline array; 29% decrease of in the signal intensity of mTOR in OVA/LM array vs. OVA/saline array. (C) Detailed phosphorylation status of the 17 proteins in MAPK signalling pathway were filtered by Commercial human/mouse MAPK protein phosphorylation array. (D) The protein expression GSK3β, p-GSK3β, mTOR, p-mTOR in lung tissue were detected by western blot. (E–H) The relative density quantifications of GSK3β, p-GSK3β, mTOR, p-mTOR, respectively. Results were expressed as the ratio of pp65 and p65 relative to β-actin, respectively. The data are presented as the mean ± SEM. n = 4 mice/group. #p < 0.05; ##p < 0.01; ###p < 0.001 vs. control group. *p < 0.05; **p < 0.01; ***p < 0.001 vs. OVA/saline group.
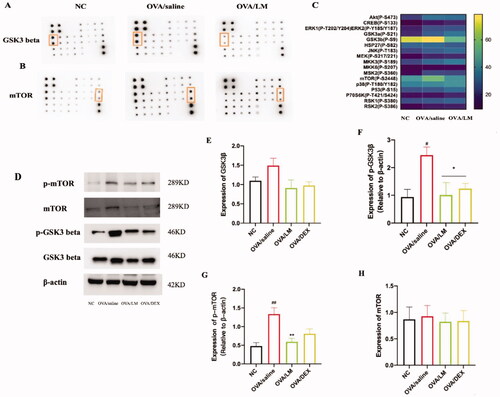
Table 2. Difference fold of GSK3β and mTOR detected by phosphorylated protein array.
LKZP regulates the expression level of inflammation-related genes
We performed a qPCR experiment to detect the gene level of IL-33, ST2 and inflammatory-related cytokines (e.g., IL-4, IL-5, IL-13, TNF-a and IFN-r). The results are shown in .Gene expression levels of IL-33 and ST2 are presented as increased in the OVA/saline group, while their expression levels are greatly downregulated after the administration of LKZP and DEX []. The elevated levels of inflammation-related indicators (e.g., IL-4, IL-5, IL-13, TNF-a and IFN-r) were found in the model group and markedly decreased expression levels of them could be found after the administration of LKZP and DEX []. Among the three LKZP intervention groups, OVA/LM showed the best anti-inflammatory effect, followed by the OVA/LL group. This may suggest that 4.2 g/kg/day is the optimal intervention concentration of LKZP.
Figure 8. LKZP regulated the expression level of inflammation-related genes. The mRNA expression of IL-33, ST2, IL-4, IL-5, IL-13, TNF-α and IFN-γ in the lung tissues were detected by qPCR. (A) mRNA expression of IL-33; (B) mRNA expression of ST2; (C) mRNA expression of IFN-γ; (D) mRNA expression of TNF-α; (E) mRNA expression of IL-4; (F) mRNA expression of IL-5; (G) mRNA expression of IL-13. The data are presented as the mean ± SEM. n = 5 mice/group. #p < 0.05; ##p < 0.01; ###p < 0.001 vs. control group. *p < 0.05; **p < 0.01; ***p < 0.001 vs. OVA/saline group.
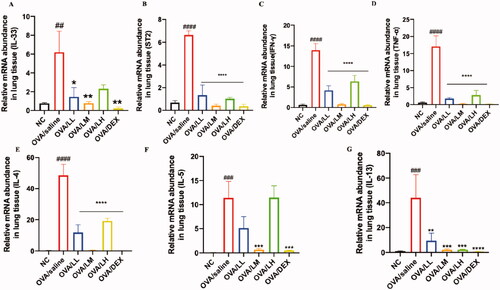
Discussion
The disruption of the epithelial cell barrier caused by allergens is the initial step of asthma attacks. IL-33 has been demonstrated to be the key cytokine released by damaged epithelial cells, and it plays an important role in AHR, inflammatory infiltration, airway contraction and other pathological changes in asthma (Barlow et al. Citation2013). IL-33 has been demonstrated to be more important than IL-25 and TSLP during the onset of asthma, which are released at the same time (Bartemes et al. Citation2012). The initial purpose of IL-33/ST2 pathway activation is to help the body eliminate exogenous pathogenic factors (Artru et al. Citation2020). However, excessive, and continuous activation may induce an excessive cascade of downstream pathways, then causing long-term inflammatory diseases such as bronchial asthma.
Current drugs to treat asthma mainly include glucocorticoids and β2-receptor agonists, which are classic but do not meet the needs of clinical patients. Several antibodies against the IL-33/ST2 pathway and other cytokines (such as IL-4) are under development. Although a number of clinical trials of these antibodies have been conducted, the side effects that are as clear as the efficacy prevent them from being approved by the FDA and becoming an option for asthmatic patients (Heck et al. Citation2015; Leung and Mitchell Citation2018; Roan et al. Citation2019; Agache et al. Citation2020; Pelaia et al. Citation2021).
LKZP has been demonstrated to have a reliable effect on respiratory diseases. Our previous study demonstrated that LKZP significantly improves asthmatic symptoms in OVA-induced acute asthma Balb/c mice (Wei et al. Citation2016). The same efficiency of LKZP has also been confirmed in a multicenter, randomized double-blind, placebo-controlled clinical study on chronic asthma patients, which also showed that LKZP treatment significantly ameliorates lung function, decreases asthma control test (ACT) scores, and reduces the onset of daytime symptoms within two weeks compared to placebo control. However, there is no significant difference in the number of night awakenings due to asthma attacks and the improvement of inflammatory-related cytokines compared with the placebo group (Lv et al. Citation2018). In a chronic asthma mouse model, the targeted proteins treated with LKZP have been investigated using proteomics technology, which demonstrated that in addition to improving lung function and airway inflammation, LKZP also has a significant effect on improving collagen deposition and mucus secretion by regulating the RMB 10 and COL6A6 activated proteins (Wuniqiemu et al. Citation2021). More in-depth research to clarify the precise mechanism and targeted proteins in acute asthma is urgently needed. In another common disease of the respiratory system, COPD, LKZP improves lung function and airway inflammation by inhibiting the IL6/pla2g2a signalling pathway, and it alleviates lung tissue fibrosis in COPD rats by intervening in the TGF-β/smad2/3 signalling pathway (Mohammadtursun et al. Citation2020). To explore the efficacy and mechanism of LKZP in the entire process of asthma attacks, an acute bronchial asthma model in Balb/c mice was created by OVA, and LKZP was administrated to asthmatic mice in the present study. Compared to the normal group, IL-33 and ST2 were found significantly increased at the cytokine, protein and gene expression levels in the model group, which was consistent with the previously reported results (Doherty et al. Citation2015; Yu et al. Citation2018). However, the activation status of the IL-33/ST2 pathway was significantly inhibited after the administration of LKZP. Thus, we hypothesized that LKZP may prevent airway epithelial cells from being damaged during the process of OVA sensitization and challenge.
IgE, which is mainly released from B cells, is a recognized elevated indicator in allergic asthma, and it is also the main mediator of airway hyperresponsiveness and airway inflammatory changes. An increased expression level of IgE was observed in the OVA/saline group, and the expression level was decreased after LKZP intervention of LKZP, suggesting that LKZP may regulate humoral immunity.
IL-5, which is mainly released from TH2 cells, is an important regulator of the proliferation and differentiation of B cells and eosinophils. The increased expression level of IL-5 indirectly indicates the aggravation of TH2 cell responses. Consistently, the present study found that OVA sensitizations and inhalation promoted airway hyperresponsiveness and increased eosinophils in BALF. Severe eosinophil infiltration around the airway and perivasculature was also demonstrated in the model group, which may have been caused by the massive secretion of IL-5. The above inflammatory changes were significantly improved in the treatment groups, indicating that reducing the secretion of IL-5 may be a mechanism by which LKZP improves asthma-related symptoms. IL-4 is the gold standard of TH2 cell activation, and it acts on many immune cells and structural cells. The expression level of IL-4 was sharply increased in the model group but decreased after LKZP intervention. Both ELISA and qPCR results suggested that LKZP had a remarkable anti-inflammatory effect on asthma by interfering with the expression of IL-4 and IL-5. Although the precise mechanism remains unknown, these results suggested that LKZP can act directly and/or indirectly on TH2-type cells. Moreover, the present study only demonstrated that LKZP reduced the secretion of IL-4 and IL-5 from TH2-type cells, but it remains unknown whether LKZP inhibits the effect of IL-4 and IL-5. Thus, additional studies are warranted, which may help to discover whether LKZP has similar effects as mepolizumab and other biological agents, and they may help to define the target of LKZP clearly. Asthma is an inflammatory disease that is imbalanced in the activation of TH1-/2-type cells. Representative cytokines secreted from TH1-type cells were evaluated by ELISA and qPCR. Compared to the normal group, the model group had significantly elevated expression levels of IL-2, TNF-α and IFN-γ found in the model group, which indicated that the imbalanced state in asthma is not only the weakening of TH1 cell function but also the increase of TH2 cells. TH1-type cells participate in the entire process of asthma and exert an inflammatory effect on eliminating allergens. Interestingly, there was no significant difference between the expression levels of type 1 and type 2 cytokines, which may be attributed to the acute asthma model, in which TH2 cells were not yet fully functioning. The expression levels of TH1/2 cell-related cytokines were found reduced after LKZP intervention, indicating that LKZP has a comprehensive anti-inflammatory effect on asthma. Thus, these findings suggested that LKZP weakens the function of the two types of cells and adjusts them to a relatively balanced state to exert anti-inflammatory effects.
IL-13 is mainly secreted from activated TH2-type cells. IL-13 has been shown to be associated with changes in airway resistance, airway hyperresponsiveness, and mucus secretion in an asthma model (Kurowska-Stolarska et al. Citation2009). In the present study, the number of metaplastic goblet cells and the degree of mucus secretion were significantly increased in the OVA/saline group but markedly decreased in LKZP treatment groups, which may have been due to suppression of the function of IL-13. IL-13 acts as a bridge between IL-33 and the innate immune response. Most innate immune cells express the receptor of ST2 on their surface and are activated by direct binding to IL-33. However, macrophages are the main functional innate immune cells, and they rarely express ST2. The secretion of IL-13 from macrophages can be strengthened once IL-33 binds to ST2 on the surface of macrophages, and macrophages are further activated and differentiated into M2-type macrophages by IL-13 in an autocrine manner, which then participates in the inflammatory process of asthma and, in turn, secrete IL-33 (Kurowska-Stolarska et al. Citation2009). Therefore, macrophages become the activated target and secretion source of IL-33 (Portelli et al. Citation2020). In the present study, the expression level of IL-13 was significantly decreased by LKZP. These findings suggested that the infiltration of inflammatory cells and the secretion of inflammatory cytokines are directly inhibited by LKZP at both the occurrence and development stages of asthma, and the cross-linking between cells after the disruption of the epithelial barrier is also regulated.
As an Uyghur medicine compound, LKZP has many pleiotropic characteristics to treat diseases. ST2 is widely distributed in the immune and nonimmune cells. Thus, it is important to investigate pathways other than the IL-33/ST2 pathway to fully understand the mechanisms of LKZP. Some scholars have reported that the downstream NF-κB and p38/ERK/JNK-MAPK signalling pathways are activated after the binding of IL-33 and ST2 (Schmitz et al. Citation2005). Inflammatory changes induced by the activation of the IL33/ST2-NF-κB/MAPK signalling pathway have been verified in many subsequent experiments (Kato Citation2019; Zhang et al. Citation2019; Ochayon et al. Citation2020; Zhang et al. Citation2020).
The NF-κB signalling pathway, a well-known inflammation-related pathway, plays an important role in the inflammatory changes of asthma. Some scholars have reported that the recruitment of eosinophils is promoted by the activated IL-33/ST2 signalling pathway and regulated by the downstream activated NF-κB nuclear factor (Chow et al. Citation2010). In the present study, the expression levels of the key proteins p65, and pp65 in the NF-κB signalling pathway were found to increase in the model group and inhibited after LKZP intervention. These data indicated that LKZP exerts an anti-inflammatory effect on asthmatic mice by inhibiting the activation of the IL-33/ST2-NF-κB signalling pathway.
The activation status of the MAPK signalling pathway was evaluated by detecting seventeen phosphorylated proteins in the MAPK signalling pathway using a commercial human and mouse MAPK pathway phosphorylation array C1 kit. Glycogen synthase kinase-3β (GSK3β; P-S9) and mammalian target of rapamycin (mTOR; P-S2448) were identified as the most significantly differentially phosphorylated proteins, and these results were verified by western blot analyses.
GSK3β regulates the activity of glycogen synthase and is the main regulator of many signal proteins, transcription factors, and structural protein signal transduction. At present, there are relatively few studies on GSK3β in asthma that have mainly focussed on the barrier function of epithelial cells and the related pathological mechanisms of airway smooth muscle. GSK3β is a negative regulator of β-catenin. After the phosphorylation and inactivation of GSK3β, β-catenin is activated continuously as the downstream target, which leads to the degradation of E-cadherin on the epithelial cell membrane, inducing the proliferation and hypertrophy of airway smooth muscle cells (Bentley et al. Citation2009; McCubrey et al. Citation2014; Yao et al. Citation2019). In addition, GSK3β can positively regulate the activation of the NF-κB signalling pathway, thereby playing an important role in the inflammatory changes in asthmatic mice (Bao et al. Citation2007). In the present study, the total protein expression level of GSK3β and p-GSK3β were found significantly increased in the model group but decreased in the LKZP intervention group. These results suggested that airway smooth muscle cells, which are of great significance in bronchial asthma, may also be one of the targets of LKZP. Follow-up experiments should be conducted in the mouse chronic asthma model to verify this mechanism.
mTOR is an important regulator of cell growth and proliferation. Some scholars have reported that the intracellular mucin particles in the airway epithelial cells are reduced by inhibiting the activation of mTOR, and further studies have verified this result in patients with asthma and COPD (Sweeter et al. Citation2021). In the asthmatic mice in the present study, PAS staining identified mucus secretion around the small airway, which may be consistent with the increased expression levels of mTOR and p-mTOR. Previous reports have found that upregulated levels of mTOR in serum-induced an imbalance in immune responses, and mTOR inhibitors attenuate asthmatic markers and restore the balance of TH17/Treg and TH1/TH2 cells (Fredriksson et al. Citation2012; Zhang et al. Citation2017). The present study demonstrated that the p-mTOR expression was increased in the OVA/saline group but decreased after LKZP intervention. These findings suggested that LKZP restores the imbalance of the adaptive immune response in asthma by inhibiting the phosphorylation of p-mTOR. Based on the above results, we speculated that after IL-33 binds to the immune and structural cells in the lung tissue, hypertrophy and metaplasia of goblet cells and the increased secretion of mucus are promoted by the activated mTOR signalling pathway. This process may be reduced by directly inhibiting the phosphorylation of mTOR in mast cells (mainly resources of mucus secretion) by LKZP. The excessive secretion of IL-13 and IL-5 by TH2 cells stimulated by IL-33 acts as a key element in OVA-induced asthmatic mice. Studies have found that the expression process of IL-13 and IL-5 are significantly inhibited by mTOR inhibitors (Salmond et al. Citation2012). Therefore, the degree of inflammatory infiltration around the airway and AHR in asthma may be improved by LKZP by inhibiting the function of mTOR.
The activation status of the NF-κB/MAPK signalling pathway was evaluated to determine the downstream functional roles of the IL-33/ST2 pathway. Many studies on the classic NF-κB/MAPK signalling pathway have been conducted in inflammatory diseases, such as asthma, but there are few reports focussing on targeting GSK-3β and mTOR, especially rare in respiratory diseases. Research on GSK-3β currently is focussed on glycogen synthesis and metabolism-related functions. The present study demonstrated that in the OVA-induced acute asthma mouse model, the first step is initiated by the activated IL-33/ST2 pathway in the broken epithelial barrier, and the NF-κB/GSK-3β/mTOR signalling pathway is presented as the downstream target. The NF-κB/MAPK/GSK-3β/mTOR signalling pathway is directly involved in pathological changes in asthma. In contrast, these signal transduction regulatory molecules also bridge the function of the inflammatory-related IL-33/ST2 pathway with the immune response. Our results suggested that during the onset of asthma, LKZP participates in every stage and that the effects of LKZP on regulating TH1/2 immune imbalance and reducing the AHR, airway inflammation and mucus secretion are especially important. In the acute asthma mouse model, our results suggested that LKZP may have an effect on anti-airway remodelling, which was consistent with previous research results of our team (Wuniqiemu et al. Citation2021).
However, limited to the characteristics of the compound, further research is needed to define the phenotype and precise target of LKZP in the treatment of asthma, which will develop new treatment strategies for asthma.
Conclusions
LKZP can improve the pathological changes such as the damage to the epithelial barrier, airway inflammatory infiltration and mucus secretion in the OVA-induced acute mouse asthma model and control the symptoms of asthma (such as airway hyperresponsiveness) by regulating activation of the IL-33/ST2-NF-κB/MAPK/GSK-3β/mTOR signalling pathway, as shown in .
Author contributions
X Huang: Methodology, Formal analysis, Investigation, Writing – original draft. H Yu: Methodology, Formal analysis, Investigation. C Xie: Formal analysis. YL Zhou: Formal analysis. MM Chen: Formal analysis. HL Shi: Formal analysis. WF Tang: Formal analysis. JC Dong: Visualisation, Supervision, Funding acquisition. QL Luo: Visualisation, Supervision, Funding acquisition.
Acknowledgements
The authors thanks Fudan University School of Medicine and Sinopharm Xinjiang Pharmaceutical Co. Ltd. for kindly supplementing with LKZP solution.
Disclosure statement
No potential conflict of interest was reported by the author(s).
Additional information
Funding
References
- Agache I, Rocha C, Beltran J, Song Y, Posso M, Sola I, Alonso-Coello P, Akdis C, Akdis M, Canonica GW, et al. 2020. Efficacy and safety of treatment with biologicals (benralizumab, dupilumab and omalizumab) for severe allergic asthma: a systematic review for the EAACI guidelines - recommendations on the use of biologicals in severe asthma. Allergy. 75(5):1043–1057.
- Artru F, Saleh MB, Maggiotto F, Lassailly G, Ningarhari M, Demaret J, Ntandja-Wandji LC, de Barros JPP, Labreuche J, Drumez E, et al. 2020. Il-33/st2 pathway regulates neutrophil migration and predicts outcome in patients with severe alcoholic hepatitis. J Hepatol. 72(6):1052–1061.
- Baekkevold ES, Roussigne M, Yamanaka T, Johansen FE, Jahnsen FL, Amalric F, Brandtzaeg P, Erard M, Haraldsen G, Girard JP. 2003. Molecular characterization of nf-hev, a nuclear factor preferentially expressed in human high endothelial venules. Am J Pathol. 163(1):69–79.
- Bao Z, Lim S, Liao W, Lin Y, Thiemermann C, Leung BP, Wong WSF. 2007. Glycogen synthase kinase-3 beta inhibition attenuates asthma in mice. Am J Respir Crit Care Med. 176(5):431–438.
- Bao WP, Zhang YY, Zhang M, Bao AH, Fei X, Zhang X, Zhou X. 2018. Effects of ozone repeated short exposures on the airway/lung inflammation, airway hyperresponsiveness and mucus production in a mouse model of ovalbumin-induced asthma. Biomed Pharmacother. 101:293–303.
- Barlow JL, Peel S, Fox J, Panova V, Hardman CS, Camelo A, Bucks C, Wu XY, Kane CM, Neill DR, et al. 2013. Il-33 is more potent than IL-25 in provoking IL-13-producing nuocytes (type 2 innate lymphoid cells) and airway contraction. J Allergy Clin Immunol. 132(4):933–941.
- Bartemes KR, Iijima K, Kobayashi T, Kephart GM, McKenzie AN, Kita H. 2012. Il-33-responsive lineage- cd25+ cd44(hi) lymphoid cells mediate innate type 2 immunity and allergic inflammation in the lungs. J Immunol. 188(3):1503–1513.
- Bentley JK, Deng H, Linn MJ, Lei J, Dokshin GA, Fingar DC, Bitar KN, Henderson WR Jr, Hershenson MB. 2009. Airway smooth muscle hyperplasia and hypertrophy correlate with glycogen synthase kinase-3 beta phosphorylation in a mouse model of asthma. Am J Physiol Lung Cell Mol Physiol. 296(2):L176–L184.
- Cayrol C, Girard JP. 2018. Interleukin-33 (IL-33): a nuclear cytokine from the IL-1 family. Immunol Rev. 281(1):154–168.
- Cho JY, Miller M, Baek KJ, Castaneda D, Nayar J, Roman M, Raz E, Broide DH. 2001. Immunostimulatory DNA sequences inhibit respiratory syncytial viral load, airway inflammation, and mucus secretion. J Allergy Clin Immunol. 108(5):697–702.
- Chow JYS, Wong CK, Cheung PFY, Lam CWK. 2010. Intracellular signaling mechanisms regulating the activation of human eosinophils by the novel th2 cytokine IL-33: implications for allergic inflammation. Cell Mol Immunol. 7(1):26–34.
- Doherty TA, Baum R, Newbury RO, Yang T, Dohil R, Aquino M, Doshi A, Walford HH, Kurten RC, Broide DH, et al. 2015. Group 2 innate lymphocytes (ilc2) are enriched in active eosinophilic esophagitis. J Allergy Clin Immunol. 136(3):792–794.
- El-Husseini ZW, Gosens R, Dekker F, Koppelman GH. 2020. The genetics of asthma and the promise of genomics-guided drug target discovery. Lancet Respir Med. 8(10):1045–1056.
- Finlay CM, Cunningham KT, Doyle B, Mills KHG. 2020. Il-33-stimulated murine mast cells polarize alternatively activated macrophages, which suppress t cells that mediate experimental autoimmune encephalomyelitis. J Immunol. 205(7):1909–1919.
- Fredriksson K, Fielhaber JA, Lam JK, Yao XL, Meyer KS, Keeran KJ, Zywicke GJ, Qu X, Yu ZX, Moss J, et al. 2012. Paradoxical effects of rapamycin on experimental house dust mite-induced asthma. PLoS One. 7(5):e33984.
- Heck S, Nguyen J, Le DD, Bals R, Dinh QT. 2015. Pharmacological therapy of bronchial asthma: the role of biologicals. Int Arch Allergy Immunol. 168(4):241–252.
- Kato A. 2019. Group 2 innate lymphoid cells in airway diseases. Chest. 156(1):141–149.
- Kurowska-Stolarska M, Stolarski B, Kewin P, Murphy G, Corrigan CJ, Ying S, Pitman N, Mirchandani A, Rana B, van Rooijen N, et al. 2009. Il-33 amplifies the polarization of alternatively activated macrophages that contribute to airway inflammation. J Immunol. 183(10):6469–6477.
- Kuruvilla ME, Lee FEH, Lee GB. 2019. Understanding asthma phenotypes, endotypes, and mechanisms of disease. Clin Rev Allergy Immunol. 56(2):219–233.
- Lefrancais E, Roga S, Gautier V, Gonzalez-de-Peredo A, Monsarrat B, Girard JP, Cayrol C. 2012. Il-33 is processed into mature bioactive forms by neutrophil elastase and cathepsin G. Proc Natl Acad Sci USA. 109(5):1673–1678.
- Leung E, Mitchell PD. 2018. Emerging treatment options in severe asthma. Curr Respir Med Rev. 14(2):64–76.
- Lv YB, Wei Y, Abduwaki M, Jurat T, Li FS, Wang HZ, Wu YH, Li Z, Liu B, Yin HJ, et al. 2018. A multicenter, randomized, double-blind, placebo-controlled study of the effects of loki zupa in patients with chronic asthma. Front Pharmacol. 9:351.
- Mattiuzzi C, Lippi G. 2020. Worldwide asthma epidemiology: insights from the global health data exchange database. Int Forum Allergy Rhinol. 10(1):75–80.
- McCubrey JA, Steelman LS, Bertrand FE, Davis NM, Abrams SL, Montalto G, D’Assoro AB, Libra M, Nicoletti F, Maestro R, et al. 2014. Multifaceted roles of GSK-3 and Wnt/β-catenin in hematopoiesis and leukemogenesis: opportunities for therapeutic intervention. Leukemia. 28(1):15–33.
- McGregor MC, Krings JG, Nair P, Castro M. 2019. Role of biologics in asthma. Am J Respir Crit Care Med. 199(4):433–445.
- Mohammadtursun N, Li QP, Abuduwaki M, Jiang S, Zhang H, Sun J, Dong JC. 2020. Loki zupa alleviates inflammatory and fibrotic responses in cigarette smoke induced rat model of chronic obstructive pulmonary disease. Chin Med. 15:92.
- Ochayon DE, Ali A, Alarcon PC, Krishnamurthy D, Kottyan LC, Borchers MT, Waggoner SN. 2020. Il-33 promotes type 1 cytokine expression via p38 mapk in human NK cells. J Leukoc Biol. 107(4):663–671.
- Pelaia C, Pelaia G, Longhini F, Crimi C, Calabrese C, Gallelli L, Sciacqua A, Vatrella A. 2021. Monoclonal antibodies targeting alarmins: a new perspective for biological therapies of severe asthma. Biomedicines. 9(9):1108.
- Portelli MA, Dijk FN, Ketelaar ME, Shrine N, Hankinson J, Bhaker S, Grotenboer NS, Obeidat M, Henry AP, Billington CK, et al. 2020. Phenotypic and functional translation of IL1rl1 locus polymorphisms in lung tissue and asthmatic airway epithelium. JCI Insight. 5(8):e132446.
- Roan F, Obata-Ninomiya K, Ziegler SF. 2019. Epithelial cell derived cytokines: more than just signaling the alarm. J Clin Invest. 129(4):1441–1451.
- Saikumar Jayalatha AK, Hesse L, Ketelaar ME, Koppelman GH, Nawijn MC. 2021. The central role of IL-33/IL-1rl1 pathway in asthma: from pathogenesis to intervention. Pharmacol Ther. 225:107847.
- Salmond RJ, Mirchandani AS, Besnard AG, Bain CC, Thomson NC, Liew FY. 2012. Il-33 induces innate lymphoid cell-mediated airway inflammation by activating mammalian target of rapamycin. J Allergy Clin Immunol. 130(5):1159.e6–1166.e6.
- Schmitz J, Owyang A, Oldham E, Song Y, Murphy E, McClanahan TK, Zurawski G, Moshrefi M, Qin J, Li X, et al. 2005. Il-33, an interleukin-1-like cytokine that signals via the IL-1 receptor-related protein st2 and induces t helper type 2-associated cytokines. Immunity. 23(5):479–490.
- Shaban SA, Brakhas SA, Ad’hiah AH. 2021. Interleukin-33 gene variants (rs928413, rs16924159 and rs7037276) and susceptibility to asthma among Iraqi adult patients. Meta Gene. 29:100907.
- Stier MT, Mitra R, Nyhoff LE, Goleniewska K, Zhang J, Puccetti MV, Casanova HC, Seegmiller AC, Newcomb DC, Kendall PL, et al. 2019. IL-33 is a cell-intrinsic regulator of fitness during early β cell development. J Immunol. 203(6):1457–1467.
- Sweeter JM, Kudrna K, Hunt K, Thomes P, Dickey BF, Brody SL, Dickinson JD. 2021. Autophagy of mucin granules contributes to resolution of airway mucous metaplasia. Sci Rep. 11(1):13037.
- Tang WF, Dong M, Teng FZ, Cui J, Zhu XY, Wang WQ, Wuniqiemu T, Qin JJ, Yi L, Wang SY, et al. 2021. Tmt-based quantitative proteomics reveals suppression of slc3a2 and atp1a3 expression contributes to the inhibitory role of acupuncture on airway inflammation in an ova-induced mouse asthma model. Biomed Pharmacother. 134:111001.
- Vasanthakumar A, Kallies A. 2019. Interleukin (IL)-33 and the IL-1 family of cytokines-regulators of inflammation and tissue homeostasis. Cold Spring Harb Perspect Biol. 11(3):a028506.
- Wei Y, Abduwaki M, Li MH, Luo QL, Sun J, Lv YB, Nurahmat M, Dong JC. 2016. Loki zupa (luooukezupa) decoction reduced airway inflammation in an ova-induced asthma mouse model. Chin Med. 11(1):22.
- Wuniqiemu T, Qin JJ, Teng FZ, Nabijan M, Cui J, Yi L, Tang WF, Zhu XY, Abduwaki M, Nurahmat M, et al. 2021. Quantitative proteomic profiling of targeted proteins associated with loki zupa decoction treatment in ova-induced asthmatic mice. J Ethnopharmacol. 266:113343.
- Yao L, Chen S, Tang H, Huang P, Wei S, Liang Z, Chen X, Wang H, Tao A, Chen R, et al. 2019. Transient receptor potential ion channels mediate adherens junctions dysfunction in a toluene diisocyanate-induced murine asthma model. Toxicol Sci. 168(1):160–170.
- Yu QN, Guo YB, Li X, Li CL, Tan WP, Fan XL, Qin ZL, Chen D, Wen WP, Zheng SG, et al. 2018. Ilc2 frequency and activity are inhibited by glucocorticoid treatment via stat pathway in patients with asthma. Allergy. 73(9):1860–1870.
- Zhang WZ, Borcherding N, Kolb R. 2020. IL-1 signaling in tumor microenvironment. Tumor microenvironment. Adv Exp ed Biol. 1240:1–23.
- Zhang YL, Jing Y, Qiao JY, Luan B, Wang XF, Wang L, Song Z. 2017. Activation of the mtor signaling pathway is required for asthma onset. Sci Rep. 7(1):4532.
- Zhang YY, Li S, Huang SY, Cao LZ, Liu T, Zhao JP, Wu JX, Wang JF, Cao LL, Xu JW, et al. 2019. Il33/st2 contributes to airway remodeling via p-JNK Mapk/Stat3 signaling pathway in ova-induced allergic airway inflammation in mice. Exp Lung Res. 45(3–4):65–75.