Abstract
Context
Brassica incana Ten. (Brassicaceae) is an edible plant with very limited available information. Previous studies have demonstrated the polyphenolic profile and the antioxidant and cytotoxic properties of the leaf and flowering top hydroalcoholic extracts.
Objective
The volatile composition and the antidiabetic and anti-obesity potential of B. incana leaf and flowering top extracts have been investigated.
Material and methods
The volatile characterization of the extracts was attained by HS-SPME-GC/MS analysis. The antidiabetic and anti-obesity potential was investigated spectrophotometrically in vitro by the ability to modulate pancreatic lipase and α-glucosidase at different concentrations using orlistat and acarbose as reference drugs. The inhibition of advanced glycation end-products (AGEs) was measured with aminoguanidine as reference and the antioxidant activity with the xanthine/xanthine oxidase system and Trolox for comparative purposes.
Results
Several volatiles belonging to different chemical classes were identified, being sulphur compounds the most abundant in both leaf and flowering top extracts (56.33% and 64.40% of all volatiles). Although the leaf extract showed lower IC50 values in most of the assays (0.968 and 1.921 mg/mL for α-glucosidase; 0.192 and 0.262 mg/mL for AGEs; 0.022 and 0.038 mg/mL for superoxide scavenging), there were no statistically significant differences between both samples. These extracts showed a similar behaviour to Trolox in the xanthine oxidase assay (IC50 values of 0.022 mg/mL for leaf extract; 0.038 mg/mL for flowering top and 0.028 for Trolox).
Conclusions
Leaves and flowering tops from B. incana can be used as sources of functional compounds that could act as antidiabetic and anti-obesogenic agents.
Introduction
Brassica incana Ten., a wild B. oleracea-related species, is an edible plant belonging to the Brassicaceae family. Brassica incana is a suffrutex up 100 cm high, woody at the base, branched, glabrous except at base. Basal leaves with petiole with two irregularly dentate wings, are pubescent to tomentose especially on the lower surface and along the veining, ovate to lanceolate, lyrate; the lamina has margin entire and irregularly crenate or 1–2 lobes in the lower half, usually obtuse; upper leaves are denticulate, with amplexicaule basal auricles, gradually smaller. The flowers are gathered in racemes many-flowered with yellow spatulate petals. The fruit is a siliqua patent, constricted at intervals, terete, gradually attenuate into beak (Heywood Citation1964). This species is native to south-eastern Europe, including Albania, Bosnia-Herzegovina, Croatia, Greece, and Italy; the plant has also been introduced in Ukraine and Crimea (Marhold Citation2011).
As far as we know, only a few studies on B. incana are present in the published literature, which focus on the glucosinolates contained in the leaves and the seeds, while no studies on its therapeutic potential are available (Horn and Vaughan Citation1983; Heaney et al. Citation1987; Velasco and Becker Citation2000).
Considering the very limited information about B. incana, our research team started a study aimed at investigating the potential of this species as a source of bioactive phytochemicals. In a previous study, some of the authors of the present work had characterized the volatile composition of B. incana fresh leaves and roots (Tripodi et al. Citation2012). In a recent work, the antioxidant properties, the cytotoxicity against human colorectal adenocarcinoma (Caco-2) cells and the absence of toxicity versus brine shrimp larvae (Artemia salina Leach) of the hydroalcoholic extracts obtained from the leaves and the flowering tops of B. incana grown wild in Sicily (Italy) were established (Miceli et al. Citation2020). Moreover, the quali-quantitative characterization of the phenolic compounds was performed, highlighting the presence of quercetin, kaempferol and isorhamnetin derivatives, whose antioxidant, anti-inflammatory properties as well as protective against metabolic disorders have been previously demonstrated (Carullo et al. Citation2017; Nasri et al. Citation2017; Liu et al. Citation2021).
As a continuation of the ongoing research, this work was designed to further investigate the phytochemical volatile profile and the biological potential of the same extracts obtained from edible parts of this species. To achieve a comprehensive view of the volatile composition of the extracts the headspace solid-phase microextraction (HS-SPME) coupled to gas chromatography-mass spectrometry (GC-MS) was utilized. Besides, B. incana extracts were investigated in vitro as candidates with antidiabetic and anti-obesity potential. For this purpose, the ability to modulate lipase and α-glucosidase together with inhibition of advanced glycation end-products (AGEs) and free radicals was examined (Sriramavaratharajan and Murugan Citation2018; Mustafa et al. Citation2022).
Materials and methods
Reagents and chemicals
All enzymes for bioassays such as lipase, α-glucosidase and xanthine oxidase were obtained from Sigma-Aldrich (Madrid, Spain). Orlistat as drug reference was also acquired from Sigma-Aldrich (Madrid, Spain) while acarbose was bought in Cymit Quimica (Barcelona, Spain). All other reagents, unless indicated, were purchased from Sigma (St. Louis, MO).
Plant material and extraction procedure
The plant material was collected around Capo d’Orlando (Messina, Italy). The leaves of Brassica incana were harvested in November 2018 and the flowering tops in May 2019. The taxonomic identification was confirmed by Prof. S. Ragusa, Department of Health Sciences, University Magna Graecia of Catanzaro. A voucher specimen (1108/18) was deposited in the same department.
After harvesting, the plant material was washed, blended, frozen, and lyophilized. The extraction was carried out as reported in our previous work (Miceli et al. Citation2020). The yields of the leaf and flowering top hydroalcoholic (70% MeOH) extracts, compared to 100 g of lyophilized plant material, were 26.47% and 33.16%, respectively.
Characterization of volatile compounds by SPME-GC/MS
Extraction (HS-SPME)
The hydroalcoholic extracts of both the leaves and the flowering tops of B. incana were analyzed for their volatile composition by HS-SPME-GC/MS.
The dried extracts were solubilized in saturated sodium chloride solution to a final concentration of 10 mg/mL; then 3 ± 0.1 mL of each extract solution were transferred to a 7 mL vial closed with a ‘mininert’ valve (Supelco, Bellefonte, PA). For the volatile extraction, the sample was equilibrated for 15 min at 40 °C, and a DVB/CAR/PDMS fibre, 50/30 μm film thickness (Supelco, Bellefonte, PA), was exposed for 15 min to the headspace of the sample maintained at 40 °C under continuous magnetic stirring. Finally, the SPME fibre was placed for 3 min into the injector port of the GC/MS, held at 260 °C, for the thermal desorption of the analytes onto the capillary GC column.
Analysis (GC/MS)
The volatiles were analyzed by a Shimadzu GC 2010 Plus gas chromatograph coupled to a TQMS 8040 triple quadrupole mass spectrometer (Shimadzu, Milan, Italy). Two capillary columns of different polarity were used: (1) VF-WAXms, 60 m, 0.25 mm i.d., 0.25 μm film thickness polar column (Agilent Technologies Italia S.p.A., Milan, Italy); (2) DB-5ms, 30 m, 0.25 mm i.d., 0.25 μm film thickness apolar column (Agilent Technologies Italia S.p.A., Milan, Italy).
The conditions were as follows. Injection mode: splitless. Oven temperature: (1) 45 °C held for 5 min, then increased to 80 °C at a rate of 10 °C/min and to 240 °C at 2 °C/min, held at 240 °C for 5 min, for VF-WAXms column; (2) 45 °C increased to 160 °C at a rate of 3 °C/min and to 260 °C at 10 °C/min, held at 260 °C for 5 min, for DB-5ms column. Carrier gas: helium at a constant flow of 1 mL/min. Transfer line temperature: 250 °C. Acquisition range: 40–360 m/z; scan speed of 1250. For the identification of the volatiles, mass spectral data, NIST’ 14 (NIST/EPA/NIH Mass Spectra Library, version 2.0, USA) and FFNSC 3.0 database, linear retention indices (LRI), literature data and injection of the available standards were used (Cincotta et al. Citation2018).
Bioactivity of B. incana extracts
All in vitro bioactivity tests were performed as previously described in Taviano et al. (Citation2020) using control wells with all reagents except for extract and sample wells in order to check the inhibitory profile. Blank wells were also measured in order to eliminate interferences. A wide range of concentrations (0.0001–10 mg/mL for enzymatic assays and 0.03–0.5 mg/mL for AGES) was tested in the assays.
Pancreatic lipase inhibition
Lipase inhibition was measured as previously reported by Taviano et al. (Citation2020) in 96-well microplates. Briefly, 40 µL of enzyme (2.5 mg/mL in 0.1 M phosphate buffer, pH 7.0), previously centrifugated at 2000 × g for 7 min was mixed with 40 µL of extract and 20 µL of 10 mM p-nitrophenyl butyrate (p-NPB). After 10 min incubation, absorbance was recorded at 405 nm using also orlistat as drug reference.
α-Glucosidase inhibition
α-Glucosidase inhibition was also investigated as reported by Taviano et al. (Citation2020). 100 µL of enzyme (1 U/mL) dissolved in buffer (12.5 mM Na2HPO4, 3.3 mM NaH2PO4; pH = 6.9) was mixed with 50 µL of extract and then incubated at room temperature for 10 min. Then, 50 µL of 3 mM p-nitrophenyl-α-d-glucopyranoside (pNPG) were added. After 15 min at 37 °C, absorbance was recorded at 405 nm using acarbose as drug reference.
Advanced glycation end-products inhibition
Advanced glycation end-products (AGEs) inhibition was measured in 96-black well-plates according to Spínola and Castilho (Citation2017). 10 mg/mL Bovine serum albumin solution (50 μL) was mixed with 80 μL of 0.1 M phosphate buffer (containing sodium azide 3 mM, pH =7.4), 50 μL of 0.5 M fructose solution (0.5 M) and 20 μL of extracts. After 24 h incubation at 37 °C in the dark, fluorescence was measured (355 nm excitation wavelength and 460 nm emission wavelength) using aminoguanidine (AMG) as drug reference.
Antiradical activity
Free radical scavenging activity was evaluated by calculating the percentage of inhibition of superoxide radicals generated by xanthine oxidase (Mustafa et al. Citation2022) using trolox as reference substance. Briefly, 240 μL of the reaction mixture [90 μM xanthine, 16 mM Na2CO3, and 22.8 μM nitroblue tetrazolium chloride (NBT) in phosphate buffer pH 7.0] was mixed with 30 μL of extract solution at different concentrations; then, xanthine oxidase (XO) was added, and absorbance was read at 560 nm after 2 min incubation at 37 °C.
Data and statistical analyses
Results about bioactivity are presented as mean values and standard error of mean (SEM) of at least three independent experiments in different days. All bioactivity assays were performed at between 5 and 9 different concentrations for non-linear regression. GraphPad Prism v.7.0 (GraphPad Software, La Jolla, CA) was used for formal analyses. IC50 values were obtained by non-linear regression and one-way ANOVA with Tukey multiple comparison test was used in order to detect differences between the samples.
Results and discussion
Characterization of volatile compounds by SPME-GC/MS
The volatile composition of the hydroalcoholic extracts of the B. incana leaves and flowering tops are reported in and , respectively. A large number of compounds belonging to the chemical classes of esters, alcohols, acids, ketones, aldehydes, terpenes, hydrocarbons, sulphur compounds and nitriles were detected in the headspace of leaf and flowering top extracts.
Table 1. Composition as volatile constituents and classes of substances of B. incana leaf hydroalcoholic extract.
Table 2. Composition as volatile constituents and classes of substances of B. incana flowering top hydroalcoholic extract.
Regarding the leaf extract, its volatile fraction was constituted mainly of sulphur compounds (sulphides and isothiocyanates) which accounted for over 56% of all volatiles. Among the other chemical classes, alcohols, aldehydes and acids were the most represented with a percentage close to 10% for each one. 3-Butenyl isothiocyanate (43.10%), dimethyl trisulphide (10.66%) and 1-dodecanol (7.61%) were the compounds quantitatively most represented.
These results are quite different from those reported in our previous study on the volatiles of B. incana leaves (Tripodi et al. Citation2012); this can be explained considering that previously the SPME extraction technique was directly applied to the fresh plant leaves, and the characteristic “green leaf” volatiles, such as (E)-2-hexanal (leaf aldehyde), (Z)-3-hexenol (leaf alcohol) and, in general, C6 aldehydes and alcohols, resulted the main constituents of the leaf headspace; instead isothiocyanates were the main volatiles of the hydroalcoholic extract of B. incana leaves. Isothiocyanates arise from the glucosinolate hydrolysis after plant cell rupture, and in case of the hydroalcoholic extract, the procedure for sample preparation certainly favoured their formation (Fenwick et al. Citation1983). However, in both cases the class of isothiocyanates was constituted mostly of 3-butenyl isothiocyanate.
The volatile fraction of the flowering top extract was composed mostly of sulphur compounds and nitriles. These two classes of compounds constituted about the 82% of the whole volatile fraction. The main constituents were dimethyl trisulphide (36.22%), dimethyl disulphide (18.51%), 3-methyl-3-butenenitrile (16.06%) and dimethyl tetrasulfide (8.16%). Isobutyl isothiocyanate and 3-butenyl isothiocyanate were the only isothiocyanates detected but they were present at very low levels representing only the 0.08% and 0.57% of the whole volatile fraction, respectively. The remaining compounds were present as minor constituents (<1%), except for 1-penten-3-one (1.95%), hexahydrofarnesyl acetone (1.32%), 1-octen-3-ol (1.07%), 1-octanol (1.11%) and limonene (2.20%).
The volatile profiles of the B. incana extracts showed significant differences. In particular, among sulphur compounds, isothiocyanates prevailed in the leaf extract, whereas sulphides in the flowering top one; similarly, Robertson et al. (Citation1993) analyzing five different varieties of Brassica napus found that organic sulphides were among the major volatile compounds released from the flowers, whereas no isothiocyanates were detected.
Moreover, the headspace of the flowering top extract was very rich in nitriles while aldehydes, alcohols and acids were quantitatively less represented than in the leaf extract headspace.
Like isothiocyanates, nitriles are hydrolysis products of glucosinolates by the action of the myrosinase. The enzymatic cleavage can lead to different products depending on the glucosinolate structure and the presence of factors which modify the action of the enzyme. It has been demonstrated that ferrous ions and acidic conditions favour nitrile formation; moreover, nitriles are also favoured by the aglycone autolysis (Fenwick et al. Citation1983).
Bioactivity of B. incana extracts
Brassica incana extracts were able to inhibit pancreatic lipase and α-glucosidase in a dose-dependent manner, as reported in . Although the observed inhibitions were not superior to the drug references used in the bioassays, orlistat and acarbose, it is the first time that these activities are reported for this plant species. In the case of pancreatic lipase, the IC50 value was lower for the flowering top extract whereas in the glucosidase assay the best results were obtained for the leaves extract (). Both enzymes, pancreatic lipase and α-glucosidase, are key physiological and pharmacological targets for the treatment and prevention of metabolic disorders such as obesity, diabetes or the metabolic syndrome and therefore widely studied as pharmacological targets for phytochemicals (Ahmad et al. Citation2020; El-Nashar et al. Citation2021). Cruciferous plants (Brassicaceae family) have been presented several times as healthy food plants due to their content in bioactive compounds; particularly because they are rich in glucosinolates and their derived volatile sulphur compounds known as isothiocyanates, which are in relation with the prevention of certain cancers and disorders such as the metabolic syndrome (Esteve Citation2020; Melim et al. Citation2022). Although is it not clear in the literature that isothiocyanates act as pancreatic lipase or alpha-glucosidase inhibitors, other cruciferous plants have also demonstrated this kind of in vitro activity (Taviano et al. Citation2020). In previous work, several polyphenols, such as phenolic acids or flavonoids, have been detected in both B. incana leaf and flowering top extracts utilized in this study (Miceli et al. Citation2020). It was reported that extracts rich in polyphenols have a great capacity to inhibit enzymes involved in glucose and fat metabolism, such as alpha-glucosidase and lipase (Les et al. Citation2020). Thus, it can be assumed that the polyphenolic compounds are involved in the lipase or glucosidase inhibiting activity highlighted for B. incana extracts.
Figure 1. Inhibition of pancreatic lipase (A) and α-glucosidase (B) by B. incana leaf and flowering top hydroalcoholic extracts. Orlistat and acarbose were used as positive control substances.
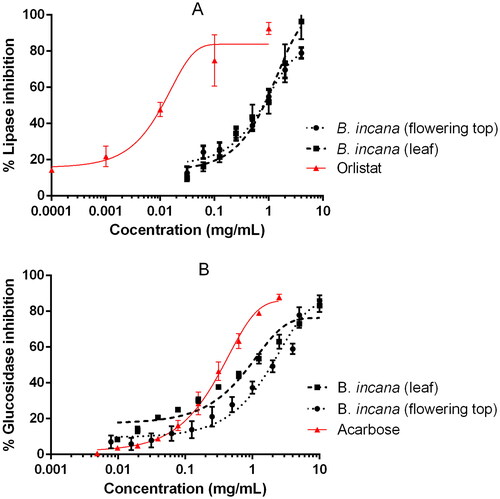
Table 3. IC50 values for B. incana leaf and flowering top hydroalcoholic extracts and drug compounds used as references.
In relation with antiobesogenic and antidiabetic activity, these extracts have also revealed AGEs inhibitory properties. The inhibitory activity is also better for the leaves than for the flowering top extracts (); the level of fluorescence of BSA alone, and BSA + fructose, and BSA + fructose + treatments is included as Supplementary material. AGEs production is implicated in these metabolic diseases because of bad hyperglycaemia control; elevated glucose blood concentration leads to increased protein glycation generating a proinflammatory state (Garay-Sevilla et al. Citation2021).
Figure 2. Inhibition of advanced glycation end-products (AGEs) by B. incana leaf and flowering top hydroalcoholic extracts (A) compared to aminoguanidine (B), used as positive control substance.
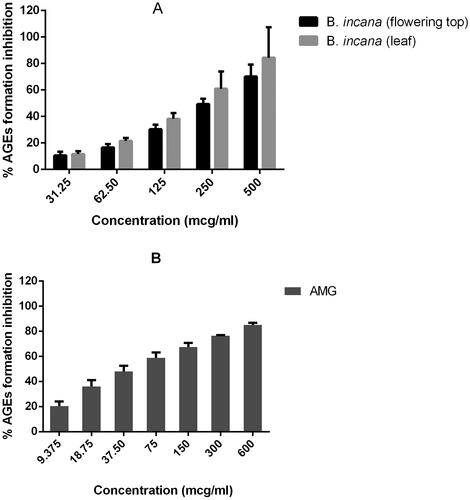
Nevertheless, hyperglycaemia not only contributes to AGEs production but also to oxidative stress and free radical release, inducing cellular ageing and disfunction (Silveira Rossi et al. Citation2022). For this reason, it is also important that α-glucosidase inhibitors may also act as anti-AGEs and antioxidant agents. shows the activity of leaf and flowering top extracts of B. incana against superoxide radicals in the xanthine/xanthine oxidase system. As it can be observed, the antiradical activity is slightly better for the leaf extract, whose IC50 values are lower than the values obtained for the flowering top one (). The antioxidant activity of B. incana has already been published (Miceli et al. Citation2020; Picchi et al. Citation2020) but this is the first time that is performed against superoxide radicals generated by xanthine oxidase and compared with trolox using non-linear regression analysis. Our results are in accordance with the previous work made by the authors as the leaf extract is better as radical scavenger than the flowering top (Miceli et al. Citation2020); nevertheless, it is surprising that the capacity of our extracts to inhibit superoxide radicals is even better than the activity displayed by Trolox ( and ). Previous work, as by Miceli et al. (Citation2020), have also dealt with the presence of phenolics and have demonstrated the absence of toxicity against A. salina nauplii, which is also important to recommend a plant matrix as a healthy functional food. Considering that these extracts act as enzyme inhibitors of pancreatic lipase and α-glucosidase and as antioxidant and anti-AGEs agents, they could represent an interesting source of bioactive molecules.
Conclusions
Herein, the volatile profile and the antidiabetic and anti-obesity potential of leaves and flowering tops from Brassica incana grown wild in Sicily (Italy) are reported. Significant differences in the volatile composition of the leaf and flowering top hydroalcoholic extracts have been highlighted. In particular, among sulphur compounds, isothiocyanates prevailed in the former, being 3-butenyl isothiocyanate the main component. Both extracts have been shown for the first time to inhibit pancreatic lipase, α-glucosidase, advanced glycation end-products and superoxide radicals in the xanthine/xanthine oxidase system, although the flowering top extract displayed better pancreatic lipase inhibiting activity, there were not significant differences between the leaf extract and the flowering top. The present findings indicate that leaves and flowering tops from B. incana are a source of functional ingredients that could act as antidiabetic and anti-obesogenic agents.
Supplemental Material
Download PDF (257 KB)Acknowledgments
The authors gratefully thank Prof. Salvatore Ragusa for plant collection and identification. Universidad San Jorge and Gobierno de Aragón are also thanked for financial support (Phyto-Pharm Group B44_20D). Sonia Núñez received a PhD grant from Industrias Químicas del Ebro.
Disclosure statement
No potential conflict of interest was reported by the author(s).
Correction Statement
This article has been republished with minor changes. These changes do not impact the academic content of the article.
References
- Ahmad B, Friar EP, Vohra MS, Garrett MD, Serpell CJ, Fong IL, Wong EH. 2020. Mechanisms of action for the anti-obesogenic activities of phytochemicals. Phytochemistry. 180:112513.
- Carullo G, Cappello AR, Frattaruolo L, Badolato M, Armentano B, Aiello F. 2017. Quercetin and derivatives: useful tools in inflammation and pain management. Future Med Chem. 9(1):79–93.
- Cincotta F, Verzera A, Tripodi G, Condurso C. 2018. Non-intentionally added substances in PET bottled mineral water during the shelf-life. Eur Food Res Technol. 244(3):433–439.
- El-Nashar HAS, Mostafa NM, El-Shazly M, Eldahshan OA. 2021. The role of plant-derived compounds in managing diabetes mellitus: a review of literature from 2014 To 2019. Curr Med Chem. 28(23):4694–4730.
- Esteve M. 2020. Mechanisms underlying biological effects of cruciferous glucosinolate-derived isothiocyanates/indoles: a focus on metabolic syndrome. Front Nutr. 7:111.
- Fenwick GR, Heaney RK, Mullin WJ, VanEtten CH. 1983. Glucosinolates and their breakdown products in food and food plants. Crit Rev Food Sci Nutr. 18(2):123–201.
- Garay-Sevilla ME, Rojas A, Portero-Otin M, Uribarri J. 2021. Dietary AGEs as exogenous boosters of inflammation. Nutrients. 13(8):2802.
- Heaney RK, Fenwick GR, Mithen RF, Lewis BG. 1987. Glucosinolates of wild and cultivated Brassica species. Phytochemistry. 26(7):1969–1973.
- Heywood VH. 1964. Brassica L. In: Tutin TG, Heywood VH, Burges NA, Moore DM, Valentine DH, Walters SM, Webb DA, editors. Flora Europaea. Cambridge: Cambridge University Press; p. 335–339.
- Horn PJ, Vaughan JG. 1983. Seed glucosinolates of fourteen wild Brassica species. Phytochemistry. 22(2):465–470.
- Les F, Cásedas G, Valero MS, Arbonés-Mainar JM, López V. 2020. Rock tea (Jasonia glutinosa (L.) DC.) polyphenolic extract inhibits triglyceride accumulation in 3T3-L1 adipocyte-like cells and obesity related enzymes in vitro. Food Funct. 11(10):8931–8938.
- Liu S, Xiao P, Kuang Y, Hao J, Huang T, Liu E. 2021. Flavonoids from sea buckthorn: a review on phytochemistry, pharmacokinetics and role in metabolic diseases. J Food Biochem. 45(5):e13724.
- Marhold KB. 2011. Euro + Med Plantbase – the Information Resource for Euro-Mediterranean Plant Diversity [accessed on 1 February 2020]. http://www.emplantbase.org/home.html.
- Melim C, Lauro MR, Pires IM, Oliveira PJ, Cabral C. 2022. The role of glucosinolates from cruciferous vegetables (Brassicaceae) in gastrointestinal cancers: from prevention to therapeutics. Pharmaceutics. 14(1):190.
- Miceli N, Cavò E, Ragusa M, Cacciola F, Mondello L, Dugo L, Acquaviva R, Malfa GA, Marino A, D’Arrigo M, et al. 2020. Brassica incana Ten. (Brassicaceae): phenolic constituents, antioxidant and cytotoxic properties of the leaf and flowering top extracts. Molecules. 25(6):1461.
- Mustafa AM, Mazzara E, Abouelenein D, Angeloni S, Nunez S, Sagratini G, López V, Cespi M, Vittori S, Caprioli G, et al. 2022. Optimization of solvent-free microwave-assisted hydrodiffusion and gravity extraction of Morus nigra L. fruits maximizing polyphenols, sugar content, and biological activities using central composite design. Pharmaceuticals. 15(1):99.
- Nasri I, Chawech R, Girardi C, Mas E, Ferrand A, Vergnolle N, Fabre N, Mezghani-Jarraya R, Racaud-Sultan C. 2017. Anti-inflammatory and anticancer effects of flavonol glycosides from Diplotaxis harra through GSK3β regulation in intestinal cells. Pharm Biol. 55(1):124–131.
- Picchi V, Lo Scalzo R, Tava A, Doria F, Argento S, Toscano S, Treccarichi S, Branca F. 2020. Phytochemical characterization and in vitro antioxidant properties of four Brassica wild species from Italy. Molecules. 25(15):3495.
- Robertson GW, Griffiths DW, Smith WM, Butcher RD. 1993. The application of thermal desorption‐gas chromatography‐mass spectrometry to the analyses of flower volatiles from five varieties of oilseed rape (Brassica napus spp. oleifera. Phytochem Anal. 4(4):152–157. )
- Silveira Rossi JL, Barbalho SM, Reverete de Araujo R, Bechara MD, Sloan KP, Sloan LA. 2022. Metabolic syndrome and cardiovascular diseases: going beyond traditional risk factors. Diabetes Metab Res Rev. 38(3):e3502.
- Spínola V, Castilho PC. 2017. Evaluation of Asteraceae herbal extracts in the management of diabetes and obesity. Contribution of caffeoylquinic acids on the inhibition of digestive enzymes activity and formation of advanced glycation end-products (in vitro). Phytochemistry. 143:29–35.
- Sriramavaratharajan V, Murugan R. 2018. Cumin scented leaf essential oil of Cinnamomum chemungianum: compositions and their in vitro antioxidant, α-amylase, α-glucosidase and lipase inhibitory activities. Nat Prod Res. 32(17):2081–2084.
- Taviano MF, Miceli N, Acquaviva R, Malfa GA, Ragusa S, Giordano D, Cásedas G, Les F, López V. 2020. Cytotoxic, antioxidant, and enzyme inhibitory properties of the traditional medicinal plant Matthiola incana (L.) R. Br. Biology. 9(7):163.
- Tripodi G, Verzera A, Dima G, Condurso C, Ragusa S. 2012. Brassica fruticulosa Cyr. and Brassica incana Ten. (Brassicaceae) as Mediterranean traditional wild vegetables: a valuable source of bioactive compounds. J Essent Oil Res. 24(6):539–545.
- Velasco L, Becker HC. 2000. Variability for seed glucosinolates in a germplasm collection of the genus Brassica. Genet Resour Crop Evol. 47(3):231–238.