Abstract
Context
Sodium tanshinone IIA sulphate (STS) is a product originated from Salvia miltiorrhiza Bunge [Lamiaceae], which exerts an antitumour effect. However, the role of STS on lung adenocarcinoma (LUAD) remains unexplored.
Objective
Our study explores the effect and mechanism of STS against LUAD.
Materials and methods
LUAD cells were treated with 100 μM STS for 24 h and control group cells were cultured under normal medium conditions. Functionally, the viability, migration, invasion and angiogenesis of LUAD cells were examined by MTT, wound healing, transwell and tube formation assay, respectively. Moreover, cells were transvected with different transfection plasmids. Dual luciferase reporter and RNA immunoprecipitation (RIP) assays were used to verify the relationship between miR-874 and eEF-2K.
Results
STS significantly decreased the viability (40–50% reduction), migration (migration rate of A549 cells from 0.67 to 0.28, H1299 cells from 0.71 to 0.41), invasion (invasion numbers of A549 cells from 172 to 55, H1299 cells from 188 to 35) and angiogenesis (80-90% reduction) of LUAD cells. Downregulation of miR-874 partially abolished the antitumour effect of STS. EEF-2K was identified to be the target of miR-874, and its downregulation markedly abolished the effects of miR-874 downregulation on tumourigenesis of LUAD. Moreover, silencing of TG2 abrogated eEF-2K-induced progression of LUAD.
Discussion and Conclusions
STS attenuated the tumourigenesis of LUAD through the mediation of the miR-874/eEF-2K/TG2 axis. STS is a promising drug to fight against lung cancer, which might effectively reverse drug resistance when combined with classical anticancer drugs.
Introduction
Lung cancer is a subtype of malignancy with high mortality, which accounts for about 20% of cancer deaths (Cao et al. Citation2021). In addition, non-small cell lung cancer (NSCLC) is a subtype of lung cancer, and lung adenocarcinoma (LUAD) is the major subtype of NSCLC (Huo et al. Citation2021). Although great advances have been made for the treatment of LUAD, the outcome is not ideal. Limited understanding about the pathogenesis of LUAD might contribute to poor prognosis, which blocks the therapeutic effect (Deng et al. Citation2021; Liu et al. Citation2021). Hence, it is essential to explore the pathogenesis of LUAD for discovering new therapeutic strategies against LUAD.
Sodium tanshinone IIA sulphate (STS) is one of the active constituents of Salvia miltiorrhiza Bunge [Lamiaceae] (Dan shen in Chinese, an herb identified by the Chinese Pharmacopoeia). STS is presently used for the treatment of ulcerative colitis, myocardium injury and coronary artery disease, etc. (Li et al. Citation2017; Zhang et al. Citation2019; Peng et al. Citation2021). It has been reported that STS was able to exhibit antitumour, antioxidative, anti-angiogenic and anti-inflammatory activities (Zhang et al. Citation2019; Liu et al. Citation2021; Peng et al. Citation2021). Regarding its antitumour roles, STS was documented to have therapeutic effects on colorectal cancer (Liu et al. Citation2021). Nevertheless, the mechanism underlying the function of STS in LUAD remains enigmatic.
MicroRNAs (miRNAs) are noncoding small RNAs, which are widely distributed in the human body (Re et al. Citation2021; Sun et al. Citation2022). The dysregulation of miRNAs is able to induce the occurrence of malignant tumours and inflammation (Bao et al. Citation2021; Fernandez-Figueras et al. Citation2022). In addition, miRNAs are vital modulators in the progression of lung cancer. For example, miR-199a-3p was able to aggravate the malignant behaviours of NSCLC (Dong et al. Citation2021). MiR-301a-3p can accelerate the growth of NSCLC cells (Li et al. Citation2020). Meanwhile, miR-874 acts as an inhibitor in a variety of cancers. For instance, miR-874 was able to inhibit the growth of colorectal cancer (Wang et al. Citation2021a). It was reported that STS could suppress the malignant behaviour and epithelial-mesenchymal transition process in gastric cancer by modulating the miR-874/HMGB2 axis (Yuan et al. Citation2020). Nevertheless, the relation between STS and miR-874 in lung cancer needs to be further validated.
Eukaryotic elongation factor-2 kinase (eEF-2K) is an atypical member of the α-kinase family (Comert Onder et al. Citation2020), which is involved in the progression of various diseases (including malignant tumours) (Veglia and Li Citation2016; Guan et al. Citation2020). EEF-2K was reported to be upregulated in lung cancer (Bircan et al. Citation2018). Nevertheless, the effect of eEF-2K on angiogenesis during the progression of LUAD remains unclear. Data derived from a bioinformatics tool found that eEF-2K might be the downstream target of miR-874, while the detailed correlation between miR-874 and eEF-2K in LUAD has not been reported yet.
Transglutaminase 2 (TG2) is a member of the transglutaminase superfamily which acts as a crucial mediator in the cellular process (cell proliferation, migration, invasion, etc.) (Chen et al. Citation2021; Lu et al. Citation2021). It was reported that TG2 was high expressed in lung cancer cells and TG2 deficient cells alleviated the malignant behaviour of lung cancer (Lei et al. Citation2021). Meanwhile, it was demonstrated that silencing of eEF-2K could induce apoptosis in pancreatic cancer cells through inactivation of TG2 (Ashour et al. Citation2014a), while the detailed association between eEF-2K and TG2 in LUAD remains unknown.
Based on the above background, it was hypothesised that STS might inhibit the tumourigenesis of LUAD by regulating the miR-874/eEF-2K/TG2 axis. Our study aimed to detect the relation between STS and miR-874 in LUAD. In addition, this research focussed on the mechanism by which miR-874 regulates the progression of LUAD. We hope this research will shed light on providing new targets in the treatment LUAD.
Materials and methods
Cell culture and treatment
LUAD cell lines (A549 and H1299), human umbilical vein endothelial cells (HUVECs) and 293 T cells were obtained from ATCC (Manassas, VA, USA). Cells were cultured in DMEM (Thermo Fisher Scientific, Waltham Mass, MA, USA) supplemented with 10% FBS (Invitrogen, Carlsbad, CA, USA), 1% streptomycin and penicillin (Thermo Fisher Scientific) at 37 °C and 5% CO2.
To investigate the function of STS in LUAD, LUAD cells were treated with 0, 10, 50, 100, and 200 μM STS (cat. no. SML2517, ≥95% in purity, Sigma-Aldrich, Milwaukee, WI, USA) for 24 h. In addition, Paclitaxel (PTX, 200 nM, cat. no. P875571, MACKLIN, Shanghai, China) was used as the positive control.
Cell transfection
LUAD cells were transfected with miR-874 inhibitor, miR-874 mimics or their negative control (inhibitor NC and mimics NC, respectively) to knockdown or overexpress miR-874. For eEF-2K overexpression, LUAD cells were transfected with oe-NC or oe-eEF-2K (Genepharma, Shanghai, China). The transfections were performed using Lipofectamine 2000 (cat.no. 11668019, Invitrogen) according to the manufacturer’s instructions and the transfection efficiency was detected by RT-qPCR 48 h later.
The lentivirus vectors containing short-hairpin RNA (sh-RNA) targeting eEF-2K/TG2 and negative control (sh-NC) were purchased from Genepharma. The lentiviral vector DNAs were then transfected into 293 T cells. After 48 h of transfection, the cells were incubated at 37 °C, and the supernatants containing shRNAs or their negative control were filtered into particles. LUAD cells were cultured in 6-well plates until the confluence reached 40–60%, and then were transfected with these constructed lentiviruses (3 × 108 TU/mL). After 48 h of incubation, stable lung cancer cells were then selected by puromycin (2.5 μg/mL, Sigma Aldrich, St. Louis, MO, USA). RT-qPCR was used to measure the transfection efficiency.
MTT assay
LUAD cells (5 × 103 per well) were seeded overnight. Subsequently, cells were treated with oe-NC or oe-eEF-2K for 48 h, and then cells were exposed to 20 μL MTT solution (Beyotime, Shanghai, China) for another 2 h. Then, supernatants were removed and DMSO (150 μL) was added to each well. Finally, the absorbance of cells (490 nm) was measured by microreader.
Transwell assay
The upper chamber was pre-treated with matrigel (100 μL, BD Bioscience, Frankin Lake, NJ, USA). LUAD cells (1.0 × 106 per chamber) were seeded into the upper chamber containing FBS (1%). The lower chamber was supplemented with DMEM (Thermo Fisher Scientific) containing 10% FBS (Gibco, Carlsbad, CA, USA). After 24 h of incubation at 37 °C, the transwell chamber was rinsed, fixed and stained with glutaraldhyde (5%) and crystal violet (0.1%) for 30 min at 4 °C. The transwell chamber was observed using microscope after washed with PBS. The cell invasion was reflected by the invaded cell number.
Wound healing assay
LUAD cells (5 × 103) were plated into a 6-well cell culture plate. Subsequently, cells were underlined perpendicular with a small pipette head when cell confluence reached 80-90%. Cells were further cultured in a serum-free medium after being washed. Then, the scratch widths were calculated using a microscope at 0 and 24 h.
In vitro angiogenesis assay
The 6-well plates were pre-coated with Matrigel (BD Biosciences, Franklin Lakes, NJ, USA). Subsequently, HUVECs (1 × 105) were seeded for 24 h. HUVECs were grown in A549 or H1299 cells with transfected tumour cell-conditioned medium. The capillary-like structure formation was photographed, and then the branch points were calculated. The tube length was analyzed by AxioVision Rel (version 4.8, Carl Zeiss AG, Germany).
Quantitative real-time polymerase chain reaction (RT-qPCR)
Total RNA that extracted from LUAD cells with TRIzol (Invitrogen) reagent. Subsequently, cDNA was synthesized by using reverse transcription kit (TaKaRa, Tokyo, Japan). RT-qPCR was performed by ABI7500 real-time PCR system was using SYBR Green kit (Takara). Afterwards, the relative gene expression levels were quantified by 2-ΔΔCt method. β-Actin and U6 were used as internal controls of mRNA and miRNA, respectively. Primer sequences were listed as follows: eEF-2K forward: 5′-ATGACCCAAGCTCGAACCAG-3′ and reverse: 5′-CCTGTGTCGAGTAGCACGTT-3′; miR-874: forward: 5′-GCCGAGCTGCCCTGGCCCGAGG-3′ and reverse: 5′-GTCG TATCCAGTGCAGGGTCCGAGGTATTCGCACTGGATACGACTCGGTC-3′; U6: forward: 5′-CTCGCTTCGGCAGCACAT-3′ and reverse: 5′-AACGCTTCACGAATTTGCGT-3′ and β-actin: forward: 5′-GTCCACCGCAAATGCTTCTA-3′ and reverse: 5′-TGCTGTCACCTTCACCGTTC-3′.
Western blot detection
Total proteins of LUAD cells were isolated with the ice-pre-treated RIPA Lysis Buffer. Meanwhile, the proteins were quantified by BCA protein kit (Beyotime), and then separated by 10% SDS-PAGE. Subsequently, proteins were transferred onto PVDF membranes. The membranes were blocked with 5% non-fat milk. Afterwards, the membranes were incubated with primary antibodies against eEF-2K (ab270948, 1:1000), TG2 (ab109200, 1:1000) and β-actin (ab8227, 1:1000). Then, membranes were incubated with HRP-labeled secondary antibody (ab7090, 1:5000) for 2 h. ECL kit was used to visualize the blots, and the protein band density was quantified by using ImageJ software. All antibodies were obtained from Abcam (MA, CA, USA).
Dual luciferase gene reporter assay
EEF-2K (3′-UTR) with the binding sites of miR-874 was obtained from Genepharma and cloned into vectors (pmirGLO, Promega, MA, CA, USA) for establishment of wild-type or mutate-type eEF-2K vector (WT/MUT). The WT-eEF-2K or MUT-eEF-2K was co-transfected into 293 T cells together with miR-874/NC mimics by using Lipofectamine 2000. The luciferase activity was detected by Dual-Glo Luciferase System (Promega).
RNA immunoprecipitation assay (RIP)
The relationship between eEF-2K and miR-874 was explored by RIP assay. In brief, chromatin immunoprecipitation was performed using anti-Ago2 antibody (#SAB4200085, Sigma-Aldrich, St. Louis, MA, USA), and IgG (#R9255, Sigma-Aldrich) was used as negative control. The immunoprecipitated RNA was isolated by using TRIzoL, and the level of miR-874/eEF-2K gene was assessed by RT-qPCR.
Statistical analysis
All data were expressed by mean ± standard deviation (S.D.), which was analyzed by using SPSS (IBM, USA). Student’s t-test or One-way analysis of variance (ANOVA) with Tukey’s tests was performed to analyze the differences between two groups or among multiple groups. p < 0.05 suggested a significant change.
Results
STS significantly inhibited the malignant behaviour of LUAD cells
STS is a water-soluble derivative of Tanshinone II A with a relative molecular mass of 396.39 (). Firstly, the MTT assay was used to screen the most suitable concentration of STS. The data revealed that STS dose-dependently inhibited the viability of LUAD cells (). Among them, 100 μM STS decreased cell viability by about 50%, which was selected in subsequent experiments. In addition, PTX is a natural product which had antitumour effect, and is widely used in the clinical treatment of lung cancer. To evaluate the effect of STS on LUAD cells, LUAD cells were treated with STS, and PTX was used as the positive control. As revealed in , the viability of H1299 and A549 cells was reduced by STS or PTX, and STS (or PTX) notably inhibited the migration and invasion of LUAD cells (). What’s more, STS markedly inhibited the tube formation of HUVECs, and the effect of STS was similar with PTX (). Altogether, STS significantly suppressed the viability, migration, invasion and angiogenesis of LUAD cells.
Figure 1. STS significantly inhibited the malignant behaviour of LUAD cells. (A) The structure of STS is presented. (B) LUAD cells were exposed to 0, 10, 50, 100, and 200 μM STS for 24 h. The viability of LUAD cells was tested by the MTT assay. LUAD cells were exposed to 100 μM STS or 200 nM PTX for 24 h. (C) The viability of LUAD cells was tested by the MTT assay. (D) The migration of LUAD cells was detected by wound healing assay. (E) The invasion of LUAD cells was evaluated by the transwell assay. (F) The tube formation of HUVECs was investigated by the angiogenesis assay. *p < 0.05, **p < 0.01, ***p < 0.001.
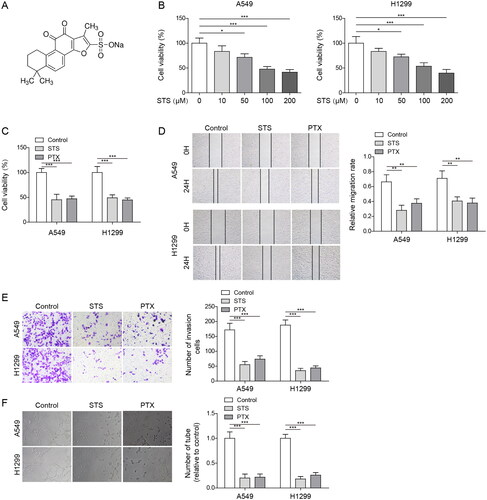
MiR-874 downregulation reversed STS-induced inhibition of angiogenesis in LUAD cells
To explore the association between STS and miR-874 in LUAD, RT-qPCR was performed. As shown in , the level of miR-874 in LUAD cells was increased by STS. Moreover, miR-874 inhibitor decreased miR-874 level in A549 and H1299 cells (). Additionally, the promoting effect of STS on miR-874 level was reversed by downregulation of miR-874 (). illustrates that STS inhibited LUAD cell viability, which was abolished by miR-874 knockdown. Consistently, STS-induced decrease of cell migration and invasion in LUAD was partially restored by miR-874 inhibitor (). Moreover, the anti-angiogenesis effect of STS on LUAD cells was greatly abolished in the presence of miR-874 inhibitor (). To sum up, STS could attenuate the angiogenesis of LUAD cells through upregulation of miR-874.
Figure 2. MiR-874 downregulation reversed STS-induced inhibition of angiogenesis in LUAD cells. LUAD cells were exposed to 100 μM STS for 24 h. Then, (A) The level of miR-874 in LUAD cells was determined by RT-qPCR. LUAD cells were transfected with inhibitor NC or miR-874 inhibitor for 24 h. (B) The level of miR-874 in LUAD cells was evaluated by RT-qPCR. LUAD cells were transfected with inhibitor NC or miR-874 inhibitor for 24 h, and then treated with 100 μM STS for 24 h. (C) The expression of miR-874 in LUAD cells was investigated by RT-qPCR. (D) The viability of LUAD cells was tested by the MTT assay. (E) The migration of LUAD cells was detected by the wound healing assay. (F) The invasion of LUAD cells was evaluated by the transwell assay. (G) The tube formation of HUVECs was tested by the angiogenesis assay. *p < 0.05, **p < 0.01, ***p < 0.001.
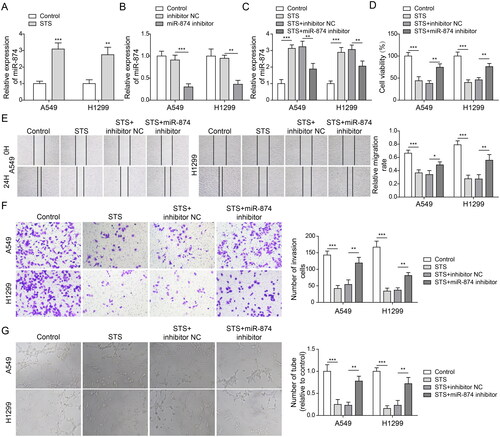
MiR-874 directly targeted eEF-2K
Next we explored the relation between miR-874 and eEF-2K. As demonstrated in , STS obviously reduced the level of eEF-2K in LUAD cells. In contrast, the expression of eEF-2K in LUAD cells was notably upregulated by downregulation of miR-874 (. Meanwhile, the prediction of Starbase database found that miR-874 and eEF-2K had mutual binding sites (). The dual luciferase gene reporter assay showed that the luciferase activity in WT-eEF-2K was inhibited by miR-874 upregulation (). Furthermore, miR-874 was demonstrated to interact with eEF-2K by the RIP assay (). Taken together, the results implied that eEF-2K was the downstream mRNA of miR-874.
Figure 3. MiR-874 directly targeted eEF-2K. LUAD cells were exposed to 100 μM STS for 24 h. Then, (A, B) the level of eEF-2K in LUAD cells was evaluated by western blots. LUAD cells were transfected with inhibitor NC or miR-874 inhibitor for 24 h. (C, D) The level of eEF-2K in LUAD cells was investigated by western blots. (E) The downstream mRNA of miR-874 was predicted by the Starbase database. Cells transfected with WT/MUT-eEF-2K/mimics NC/miR-874 mimics for 48 h. (F) The relative luciferase assay in WT/MT-eEF-2K was measured by a dual luciferase report assay. (G) The enrichment of miR-874 and eEF-2K were investigated by the RIP assay. **p < 0.01, ***p < 0.001.
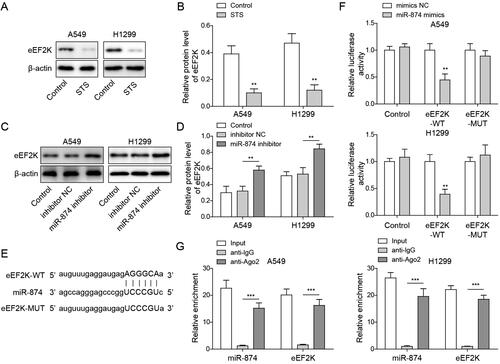
MiR-874 mediated the antitumour effect of STS by inhibiting eEF-2K in LUAD cells
To confirm the effect of eEF-2K on LUAD progression, LUAD cells were transfected with sh-eEF-2K. As indicated in , the expression of eEF-2K in LUAD cells was markedly inhibited in the presence of sh-eEF-2K. STS-induced inactivation of eEF-2K was abolished by miR-874 inhibitor, while the effect of miR-874 inhibitor was hindered by downregulation of eEF-2K (). MiR-874 inhibitor notably enhanced the viability of LUAD cells in STS-treated cells, whereas this effect was restored by silencing eEF-2K (). Consistently, STS-inhibited cell migration and invasion in LUAD were rescued by miR-874 inhibitor, while this phenomenon was partially declined by eEF-2K downregulation (. Furthermore, miR-874 inhibitor reversed the anti-angiogenesis effect of STS, while the knockdown of eEF-2K offset the effect of miR-874 inhibitor (). In summary, STS decreased the malignant behaviour of LUAD cells by regulating miR-874/eEF-2K axis.
Figure 4. MiR-874 mediated the antitumour effect of STS by inhibiting eEF-2K in LUAD cells. LUAD cells were transfected with sh-NC or sh-eEF-2K. Then, (A, B) the level of eEF-2K in LUAD cells was tested by western blots. LUAD cells were transfected with inhibitor NC + sh-NC, miR-874 inhibitor + sh-NC or miR-874 inhibitor + sh-eEF-2K, and then treated with 100 μM STS for 24 h (C, D) The level of eEF-2K in LUAD cells was tested by western blots. (E) The viability of LUAD cells was tested by the MTT assay. (F) The migration of LUAD cells was evaluated by the wound healing assay. (G) The invasion of LUAD cells was examined by the transwell assay. (H) The tube formation of HUVECs was tested by the angiogenesis assay. *p < 0.05, **p < 0.01, ***p < 0.001.
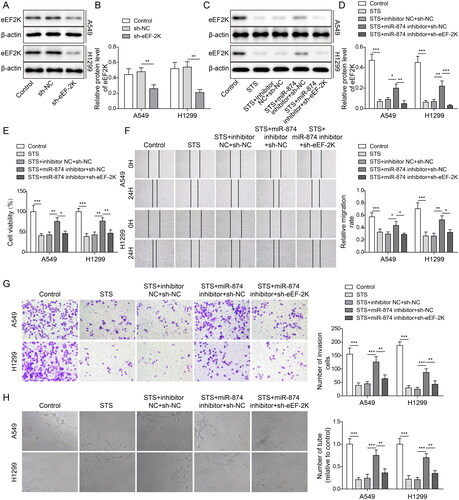
STS attenuated the tumourigenesis of LUAD through modulating the eEF-2K/TG2 axis
To further verify the mechanism underlying the function of STS in LUAD, A549 and H1299 cells were transfected with oe-eEF-2K and sh-TG2. As illustrated in , STS significantly inhibited the protein level of TG2 in LUAD cells, and the expressions of eEF-2K and TG2 in LUAD cells were notably increased by eEF-2K upregulation (). Meanwhile, the level of TG2 in LUAD cells was notably decreased by silencing of TG2 (). The expression of TG2 in LUAD cells was reduced by STS, which was reversed by upregulation of eEF-2K. However, the effect of eEF-2K upregulation on the level of TG2 was abolished by knockdown of TG2 (). Moreover, STS-induced downregulation of cell viability, migration and invasion in LUAD were significantly rescued by oe-eEF-2K, while this phenomenon was partially restored by TG2 downregulation (). EEF-2K-induced angiogenesis in HUVECs was obviously alleviated by sh-TG2 (). To sum up, STS inhibited the LUAD cell viability, migration, invasion and HUVEC angiogenesis via inactivating eEF-2K/TG2 signalling.
Figure 5. STS attenuated the tumourigenesis of LUAD through modulating the eEF-2K/TG2 axis. LUAD cells were treated with 100 μM STS for 24 h. Then, (A, B) the level of TG2 in LUAD cells was tested by western blots. β-Actin was used for normalization. LUAD cells were transfected with pcDNA3.1 (oe-NC) or pcDNA3,1-eEF-2K (oe-eEF-2K). (C, D) The levels of eEF-2K and TG2 in LUAD cells were detected by western blots. (E, F) LUAD cells were treated with sh-NC or sh-TG2. The level of TG2 in LUAD cells was evaluated by western blots. LUAD cells were transfected with oe-NC + sh-NC, oe-eEF-2K + sh-NC or oe-eEF-2K + sh-TG2, and then treated with 100 μM STS for 24 h. (G, H) The level of TG2 in LUAD cells was investigated by western blots. (I) The viability of LUAD cells was tested by MTT assay. (J) The migration of LUAD cells was evaluated by the wound healing assay. (K) The invasion of LUAD cells was determined by the transwell assay. (L) The tube formation of HUVECs was evaluated by the angiogenesis assay. **p < 0.01, ***p < 0.001.
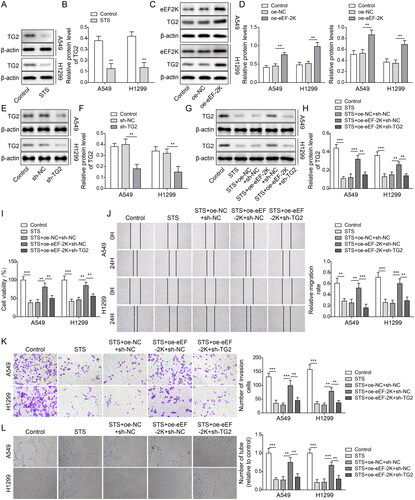
Discussion
There are more than 1.7 million new cases of lung cancer every year (Wang et al. Citation2021), and LUAD accounts for the majority of lung cancers. Nowadays, the pathogenesis of LUAD has not been completely understood yet (Chen et al. Citation2021; Yao et al. Citation2021). Meanwhile, it has been reported that STS could relieve angiogenesis during cancer progression (Huang et al. Citation2021; Zeng et al. Citation2021). Our study firstly discovered the function of STS in angiogenesis during the progression of LUAD, and the results confirmed that STS was able to attenuate the tumourigenesis of LUAD through regulating the miR-874/eEF-2K/TG2 axis.
STS functions as a tumour therapeutic agent in various human tumour cells. It was found that STS inhibited angiogenesis in colon cancer (Zou et al. Citation2021). Moreover, STS was able to attenuate the tumourigenesis of lung cancer (Li et al. Citation2021). Our research found that STS could increase the level of miR-874 and suppress the viability, migration, invasion and angiogenesis of LUAD cells. Moreover, miR-874 inhibitor reversed the antitumour effect of STS on LUAD. Herein, our research firstly explored the relation between STS and miR-874. Meanwhile, STS was confirmed to be a suppressor in cancer development by modulating miRNAs (miR-125b, miR-16-5p, etc.) (You et al. Citation2020; Wang et al. Citation2021b). Indeed, some other miRNAs which could be regulated by STS in LUAD, need to be further explored.
MiR-874 is a crucial antagonist in cancer progression. MiR-874 was reported to attenuate the invasion of papillary thyroid cancer cells by targeting FAM84A (Ding et al. Citation2021). Our finding suggested that eEF-2K was the target mRNA of miR-874 in LUAD cells, and eEF-2K knockdown rescued the antitumour effect of miR-874 downregulation on LUAD. EEF-2K was known to be an oncogene in different cancers (Yu et al. Citation2019; Guan et al. Citation2020). In addition, eEF-2K was identified to be positively associated with the poor prognosis of patients with LUAD (Bircan et al. Citation2018). TG2 was positively regulated by eEF-2K, and eEF-2K silencing was able to reduce the invasion and migration of pancreatic cancer cells via downregulation of TG2 (Ashour et al. Citation2014a, Citation2014b). This research found that overexpression of eEF-2K aggravated tumour progression of LUAD cells, and TG2 silencing could reverse this phenomenon. MiR-874 mediated the antitumour effect of STS by inhibiting the eEF-2K/TG2 pathway.
Our study found that STS had similar anti-LUAD effects as PTX in vitro. PTX is a subtype of chemotherapy drug which has cytotoxicity. Although PTX has been widely used in the clinic, the toxicity of PTX limits its further application. PTX was able to induce neuronal pain (Staff et al. Citation2020), cardiovascular toxicity (Khaled et al. Citation2022) and pulmonary toxicity (Bielopolski et al. Citation2017) during cancer treatment. In addition, long-term use of PTX can induce resistance. At present, the extraction of antitumour drugs from Chinese medicine has attracted more and more attention. STS is a diterpene quinone compound derived from Salvia miltiorrhiza. A previous study found that STS could protect alveolar epithelial cells from mitochondrial dysfunction, oxidative stress and apoptosis (Guan et al. Citation2021). Moreover, STS was able to prevent chronic obstructive pulmonary disease and alleviate silico-fibrosis in rats (Zhu et al. Citation2020; Tian et al. Citation2022). Of note, STS could also reverse chemotherapeutic drug-induced neuropathic pain and attenuate the malignancy of glioma cells (Di Cesare Mannelli et al. Citation2018). The above results suggest that STS might reduce the damage to normal cells during the anti-LUAD process. However, comparison of the toxic effects of STS and PTX on normal lung cells remains unclear. The regulation effect of STS on LUAD in vivo and its comparison with PTX are worthy of further study. In addition, the reduction of PTX resistance by STS combined with PTX therapy in LUAD cells remains to be further investigated.
Conclusions
STS inhibited angiogenesis in LUAD via increasing miR-874. Mechanistically, miR-874 inhibited TG2 via targeting eEF-2K, and the effect of STS on LUAD process was achieved by increasing miR-874. STS is expected to be a safe and effective drug for the treatment of lung cancer. The exploration of the antitumour mechanism of STS is of great significance for finding alternative drugs against lung cancer or adjuvant to resist chemoresistance.
Author contributions
Guarantor of integrity of the entire study: Zhi-Hua Zhang, Bu Wang. Study concepts: Bu Wang, Fang Zou, Gu Xin. Study design: Bao-Li Xiang, Jian-Qing Zhao, Sheng-Fang Yuan. Definition of intellectual content: Bu Wang, Zhi-hua Zhang, Xiu-Long Zhang. Literature research: Bu Wang, Fang Zou, Jian-Qing Zhao. Clinical studies: Sheng-Fang Yuan, Xiu-Long Zhang. Experimental studies: Gu Xin, Sheng-Fang Yuan. Data acquisition: Bu Wang, Gu Xin. Data analysis: Bao-Li Xiang, Jian-Qing Zhao. Statistical analysis: Sheng-Fang Yuan, Xiu-Long Zhang. Manuscript preparation: Fang Zou, Bao-Li Xiang. Manuscript editing: Sheng-Fang Yuan, Zhi-hua Zhang. Manuscript review: Bao-Li Xiang, Bu Wang.
Disclosure statement
The authors declared no competing interests in this research.
Data availability statement
All data generated or analyzed during this study are included in this published article.
Additional information
Funding
References
- Ashour AA, Abdel-Aziz AA, Mansour AM, Alpay SN, Huo L, Ozpolat B. 2014a. Targeting elongation factor-2 kinase (eEF-2K) induces apoptosis in human pancreatic cancer cells. Apoptosis. 19(1):241–258.
- Ashour AA, Gurbuz N, Alpay SN, Abdel-Aziz AA, Mansour AM, Huo L, Ozpolat B. 2014b. Elongation factor-2 kinase regulates TG2/beta1 integrin/Src/uPAR pathway and epithelial-mesenchymal transition mediating pancreatic cancer cells invasion. J Cell Mol Med. 18(11):2235–2251.
- Bao J, Lin C, Zhou X, Ma D, Ge L, Xu K, Moqbel SAA, He Y, Ma C, Ran J, et al. 2021. CircFAM160A2 promotes mitochondrial stabilization and apoptosis reduction in osteoarthritis chondrocytes by targeting miR-505-3p and SIRT3. Oxid Med Cell Longev. 2021:5712280.
- Bielopolski D, Evron E, Moreh-Rahav O, Landes M, Stemmer SM, Salamon F. 2017. Paclitaxel-induced pneumonitis in patients with breast cancer: case series and review of the literature. J Chemother. 29(2):113–117.
- Bircan HA, Gurbuz N, Pataer A, Caner A, Kahraman N, Bayraktar E, Bayraktar R, Erdogan MA, Kabil N, Ozpolat B. 2018. Elongation factor-2 kinase (eEF-2K) expression is associated with poor patient survival and promotes proliferation, invasion and tumor growth of lung cancer. Lung Cancer. 124:31–39.
- Cao F, Xiao Z, Chen S, Zhao C, Chen D, Haisma HJ, Dekker FJ. 2021. HDAC/MIF dual inhibitor inhibits NSCLC cell survival and proliferation by blocking the AKT pathway. Bioorg Chem. 117:105396.
- Chen X, Adhikary G, Shrestha S, Xu W, Keillor JW, Naselsky W, Eckert RL. 2021. Transglutaminase 2 maintains hepatocyte growth factor signaling to enhance the cancer cell phenotype. Mol Cancer Res. 19(12):2026–2035.
- Chen Z, Huang Y, Hu Z, Zhao M, Li M, Bi G, Zheng Y, Liang J, Lu T, Jiang W, et al. 2021. Landscape and dynamics of single tumor and immune cells in early and advanced-stage lung adenocarcinoma. Clin Transl Med. 11:e350.
- Comert Onder F, Durdagi S, Sahin K, Ozpolat B, Ay M. 2020. Design, synthesis, and molecular modeling studies of novel coumarin carboxamide derivatives as eEF-2K inhibitors. J Chem Inf Model. 60(3):1766–1778.
- Deng M, Tong R, Zhang Z, Wang T, Liang C, Zhou X, Hou G. 2021. EFNA3 as a predictor of clinical prognosis and immune checkpoint therapy efficacy in patients with lung adenocarcinoma. Cancer Cell Int. 21(1):535.
- Di Cesare Mannelli L, Piccolo M, Maione F, Ferraro MG, Irace C, De Feo V, Ghelardini C, Mascolo N. 2018. Tanshinones from Salvia miltiorrhiza Bunge revert chemotherapy-induced neuropathic pain and reduce glioblastoma cells malignancy. Biomed Pharmacother. 105:1042–1049.
- Ding Y, Wu L, Zhuang X, Cai J, Tong H, Si Y, Zhang H, Wang X, Shen M. 2021. The direct miR-874-3p-target FAM84A promotes tumor development in papillary thyroid cancer. Mol Oncol. 15(5):1597–1614.
- Dong C, Cao H, Liu Z, Xi L, Shi Y, Yang R. 2021. CHML targeted by miR-199a-3p promotes non-small cell lung cancer cell growth via binding to Rab5A. Pathol Res Pract. 227:153626.
- Fernandez-Figueras MT, Carrato C, Saenz-Sarda X, Musulen E, Fuente MJ, Puig L. 2022. MicroRNA31 and MMP-1 contribute to the differentiated pathway of invasion - with enhanced epithelial-to-mesenchymal transition - in squamous cell carcinoma of the skin. Arch Dermatol Res. 314(8):767–775.
- Guan Y, Jiang S, Ye W, Ren X, Wang X, Zhang Y, Yin M, Wang K, Tao Y, Yang J, et al. 2020. Combined treatment of mitoxantrone sensitizes breast cancer cells to rapalogs through blocking eEF-2K-mediated activation of Akt and autophagy. Cell Death Dis. 11(11):948–951.
- Guan R, Yao H, Li Z, Qian J, Yuan L, Cai Z, Ding M, Liu W, Xu J, Li Y, et al. 2021. Sodium tanshinone IIA sulfonate attenuates cigarette smoke extract-induced mitochondrial dysfunction, oxidative stress, and apoptosis in alveolar epithelial cells by enhancing SIRT1 pathway. Toxicol Sci. 183(2):352–362.
- Huang H, Yao Y, Hou G, Zhao C, Qin J, Zhang Y, Duan Y, Song C, Chang J. 2021. Design, synthesis and biological evaluation of tanshinone IIA-based analogues: potent inhibitors of microtubule formation and angiogenesis. Eur J Med Chem. 224:113708.
- Huo KG, Notsuda H, Fang Z, Liu NF, Gebregiworgis T, Li Q, Pham NA, Li M, Liu N, Shepherd FA, et al. 2021. Lung cancer driven by BRAF(G469V) mutation is targetable by EGFR kinase inhibitors. J Thorac Oncol. 110:1000–1004.
- Khaled SS, Soliman HA, Abdel-Gabbar M, Ahmed NA, Attia K, Mahran HA, El-Nahass ES, Ahmed OM. 2022. The preventive effects of naringin and naringenin against paclitaxel-induced nephrotoxicity and cardiotoxicity in male Wistar rats. Evid Based Complement Alternat Med. 2022:8739815.
- Lei X, Cao K, Chen Y, Shen H, Liu Z, Qin H, Cai J, Gao F, Yang Y. 2021. Nuclear Transglutaminase 2 interacts with topoisomerase II to promote DNA damage repair in lung cancer cells. J Exp Clin Cancer Res. 40(1):224.
- Li S, Jiao Y, Wang H, Shang Q, Lu F, Huang L, Liu J, Xu H, Chen K. 2017. Sodium tanshinone IIA sulfate adjunct therapy reduces high-sensitivity C-reactive protein level in coronary artery disease patients: a randomized controlled trial. Sci Rep. 7(1):17451.
- Li Z, Zhang Y, Zhou Y, Wang F, Yin C, Ding L, Zhang S. 2021. Tanshinone IIA suppresses the progression of lung adenocarcinoma through regulating CCNA2-CDK2 complex and AURKA/PLK1 pathway. Sci Rep. 11(1):23681.
- Li L, Zhao D, Cheng G, Li Q, Chu Y, Chu H, Ding Y, Li C. 2020. beta-Elemene suppresses Warburg effect in NCI-H1650 non-small-cell lung cancer cells by regulating the miR-301a-3p/AMPKalpha axis. Biosci Rep. 40:141–144.
- Liu L, Gao H, Wen T, Gu T, Zhang S, Yuan Z. 2021. Tanshinone IIA attenuates AOM/DSS-induced colorectal tumorigenesis in mice via inhibition of intestinal inflammation. Pharm Biol. 59:89–96.
- Liu Y, Liang L, Ji L, Zhang F, Chen D, Duan S, Shen H, Liang Y, Chen Y. 2021. Potentiated lung adenocarcinoma (LUAD) cell growth, migration and invasion by lncRNA DARS-AS1 via miR-188-5p/KLF12 axis. Aging . 13(19):23376–23392.
- Lu N, Wang X, Shi W, Bian L, Zhang X, Yang G, Tang X, Wang J, Zou Y, Weng Y. 2021. Black phosphorus nanoparticles promote osteogenic differentiation of EMSCs through upregulated TG2 expression. Nanoscale Res Lett. 16(1):154–159.
- Peng KY, Gu JF, Su SL, Zhu Y, Guo JM, Qian DW, Duan JA. 2021. Salvia miltiorrhiza stems and leaves total phenolic acids combination with tanshinone protect against DSS-induced ulcerative colitis through inhibiting TLR4/PI3K/AKT/mTOR signaling pathway in mice. J Ethnopharmacol. 264:113052.
- Re M, Tomasetti M, Monaco F, Amati M, Rubini C, Sollini G, Bajraktari A, Gioacchini FM, Santarelli L, Pasquini E. 2021. MiRNome analysis identifying miR-205 and miR-449a as biomarkers of disease progression in intestinal-type sinonasal adenocarcinoma. Head Neck. 12:1113–1116.
- Staff NP, Fehrenbacher JC, Caillaud M, Damaj MI, Segal RA, Rieger S. 2020. Pathogenesis of paclitaxel-induced peripheral neuropathy: a current review of in vitro and in vivo findings using rodent and human model systems. Exp Neurol. 324:113121.
- Sun ZP, Tan ZG, Peng C, Yi WM. 2022. LncRNA SNHG3 facilitates the malignant phenotype of cholangiocarcinoma cells via the miR-3173-5p/ERG axis. J Gastrointest Surg. 26(4):802–812.
- Tian D, Miao Y, Hao W, Yang N, Wang P, Ge Q, Zhang C. 2022. Tanshinone IIA protects against chronic obstructive pulmonary disease via exosomeshuttled miR4865p. Int J Mol Med. 50(1):97.
- Veglia G, Li G. 2016. Tunable liaisons: eEF-2K, CaM, and calcium. Structure. 24(9):1438–1439.
- Wang Y, Chen H, Wei X. 2021a. Circ_0007142 downregulates miR-874-3p-mediated GDPD5 on colorectal cancer cells. Eur J Clin Invest. 51(7):e13541.
- Wang Y, Jin W, Wang J. 2021b. Tanshinone IIA regulates microRNA125b/foxp3/caspase1 signaling and inhibits cell viability of nasopharyngeal carcinoma. Mol Med Rep. 23(5):700–702.
- Wang H, Wang X, Xu L, Cao H, Zhang J. 2021. Nonnegative matrix factorization-based bioinformatics analysis reveals that TPX2 and SELENBP1 are two predictors of the inner sub-consensuses of lung adenocarcinoma. Cancer Med. 10(24):9058–9077.
- Yao Y, Zhou Y, Hua Q. 2021. CircRNA hsa_circ_0018414 inhibits the progression of LUAD by sponging miR-6807-3p and upregulating DKK1. Mol Ther Nucleic Acids. 23:783–796.
- You S, He X, Wang M, Mao L, Zhang L. 2020. Tanshinone IIA suppresses glioma cell proliferation, migration and invasion both in vitro and in vivo partially through miR-16-5p/Talin-1 (TLN1) axis. Cancer Manag Res. 12:11309–11320.
- Yu P, Wang HY, Tian M, Li AX, Chen XS, Wang XL, Zhang Y, Cheng Y. 2019. Eukaryotic elongation factor-2 kinase regulates the cross-talk between autophagy and pyroptosis in doxorubicin-treated human melanoma cells in vitro. Acta Pharmacol Sin. 40(9):1237–1244.
- Yuan F, Zhao ZT, Jia B, Wang YP, Lei W. 2020. TSN inhibits cell proliferation, migration, invasion, and EMT through regulating miR-874/HMGB2/beta-catenin pathway in gastric cancer. Neoplasma. 67(5):1012–1021.
- Zeng L, Wu Q, Wang T, Li LP, Zhao X, Chen K, Qian J, Yuan L, Xu H, Mei WJ. 2021. Selective stabilization of multiple promoter G-quadruplex DNA by using 2-phenyl-1H-imidazole-based tanshinone IIA derivatives and their potential suppressing function in the metastatic breast cancer. Bioorg Chem. 106:104433.
- Zhang WX, Xiao XY, Peng CG, Chen WL, Xie S, Wang DW. 2019. Sodium tanshinone IIA sulfate protects myocardium against paraquat-induced toxicity through activating the Nrf2 signaling pathway in rats. Hum Exp Toxicol. 38(2):247–254.
- Zhu Z, Li Q, Xu C, Zhao J, Li S, Wang Y, Tian L. 2020. Sodium tanshinone IIA sulfonate attenuates silica-induced pulmonary fibrosis in rats via activation of the Nrf2 and thioredoxin system. Environ Toxicol Pharmacol. 80:103461.
- Zou W, Qian C, Zhang S, Wan X, Wei Z, Li X, Wu Y, Chen W, Wang A, Zhao Y, et al. 2021. Targeting the Ang2/Tie2 axis with tanshinone IIA elicits vascular normalization in ischemic injury and colon cancer. Oxid Med Cell Longev. 2021:7037786.