Abstract
Context
Lucialdehyde B (LB), an effective triterpenoid isolated from Ganoderma lucidum (Leyss. ex Fr.) Karst. (Polyproraceae), exerts cytotoxic activity against nasopharyngeal carcinoma CNE2 cells.
Objective
To investigate the antiproliferative and pro-apoptotic effects of LB on CNE2 cells and explore its underlying mechanisms.
Materials and methods
LB concentrations of 5–40 μg/mL were used. Cell proliferation was determined using MTT, CFSE, and colony formation assays. LB-induced apoptosis and cell cycle arrest were measured by flow cytometry after 48-h LB treatments. Fluorescence microscopy and flow cytometry were performed to measure the alteration of MMP, mPTP opening, ROS level, and Ca2+ content in CNE2 cells. Western blotting was performed to evaluate the expression of mitochondrial apoptosis-related and Ras/ERK signaling proteins.
Results
IC50 values of LB against CNE2 cells for 24, 48, and 72 h were 25.42 ± 0.87, 14.83 ± 0.93, and 11.60 ± 0.77 μg/mL, respectively. The CFSE assay showed that the cell proliferation index was 12.70 in the LB treatment group and 31.44 in the control group. LB significantly reduced clonogenic capacity, promoted cell apoptosis and induced cell cycle arrest at the G2/M phase. Our observations also revealed that LB induced ROS and calcium aggregation, opening of mPTP, MMP reduction, upregulation of mitochondrial apoptosis-related protein expression and inhibition of Ras/ERK signaling cascades.
Discussion
LB suppresses proliferation and induces mitochondrial-dependent apoptosis in nasopharyngeal carcinoma CNE2 cells.
Conclusions
LB may have a potential use as a clinical drug candidate for nasopharyngeal carcinoma treatment.
Introduction
Nasopharyngeal carcinoma (NPC), an epithelial carcinoma originating in the nasopharyngeal mucosal lining, has become one of the major public health problems in Southern China and Southeast Asia (Chen et al. Citation2019). Radiotherapy is the primary treatment modality in early-stage NPC while concurrent chemo-radiation therapy followed by adjuvant chemotherapy is the primary treatment strategy in stages III and IV (Siak et al. Citation2021). However, radiotherapy and chemotherapy for NPC inevitably induce acute and late toxicities. Traditional Chinese medicines could provide an alternative therapy, especially since they have been widely used by the medical community because of their naturally low toxicity (Yang et al. Citation2021). Therefore, the development of effective and safe novel drugs against NPC from traditional Chinese medicine needs to be explored.
Ganoderma lucidum (Leyss. ex Fr.) Karst. (Polyproraceae), a well-known traditional Chinese medicine, has been used for thousands of years in China (Li et al. Citation2020). In recent times, G. lucidum has been used widely as a clinical drug to prevent and treat cancer, chronic hepatitis, hyperlipemia, Alzheimer’s disease, and macular degeneration, among others (Ahmad et al. Citation2021). G. lucidum contains a variety of chemical components such as triterpenoids, polysaccharides, nucleotides, sterols, fatty acids, alkaloids, polypeptides, and trace elements (Wang et al. Citation2020). As one of the main bioactive components of G. lucidum, triterpenoids are well known for their diverse pharmacological activities such as hepatoprotective, anti-inflammatory, antiviral, antibacterial, antioxidant, and antitumor properties (Ahmad Citation2018). Among them, the antitumor effect of triterpenoids of G. lucidum has attracted great interest from medical researchers. In our previous study, we found that a G. lucidum extract inhibited the proliferation of the human nasopharyngeal carcinoma cell line CNE2 and induced apoptosis of CNE2 cells through the mitochondrial pathway (Huang et al. Citation2014). Notably, the extract contains triterpenoids, but it is unclear whether the triterpenoids are responsible for the antiproliferation effect observed. It is therefore essential for us to investigate the action and mechanism of pure G. lucidum triterpenoids on nasopharyngeal carcinoma for discovering natural anti-NPC agents.
Lucialdehyde B (LB) was isolated from G. lucidum, and is an effective triterpenoid with an aldehyde group, which showed cytotoxic effects against LLC, T-47D, Sarcoma 180, and Meth-A tumor cells (Gao et al. Citation2002). LB was found to exhibit potent inhibitory activity against the herpes simplex virus (Niedermeyer et al. Citation2005). Based on early activity screening, we found that LB could inhibit the growth of the nasopharyngeal carcinoma cell line CNE2 in vitro (Yu et al. Citation2022). Therefore, we hypothesized that LB may be a promising anti-NPC agent. This study aimed to investigate the antiproliferative and pro-apoptotic effects of LB on nasopharyngeal carcinoma CNE2 cells and explore its underlying mechanisms.
Materials and methods
Reagents
3-(4,5-Dimethylthiazol-2-yl)-2,5-diphenyl tetrazolium bromide (MTT) and propidium iodide (PI) were purchased from Sigma-Aldrich (St. Louis, MO, USA). CFDA-SE and crystal violet were purchased from MedChemExpress (Monmouth Junction, NJ, USA). An annexin V-FITC/PI assay kit was purchased from Roche (Basel, Switzerland). Antibodies against Ras (14429S), c-Raf (53745S), p-c-Raf (9421S), Erk1/2 (4695S), p-Erk1/2 (4370S), GAPDH (5174S), caspase-9 (9508 T), caspase-3 (9665 T), Bcl-2 (15071 T), cleaved caspase-9 (7237 T), cleaved caspase-3 (9664 T), Bax (5023S), and Cytc (4280S) were purchased from Cell Signaling Technology (Danvers, MA, USA).
Cell culture and source of LB
The nasopharyngeal carcinoma cell lines, CNE2 and CNE1, and the mouse embryonic fibroblast cell line, NIH3T3 (Chinese Academy of Medical Sciences, Beijing, China), were cultured in RPMI1640 (Gibco, Grand Island, NY, USA) medium containing 10% fetal bovine serum, 100 IU/mL penicillin and 100 μg/mL streptomycin in an incubator with 5% CO2 saturated humidity at 37 °C. Cells in logarithmic growth phase were used for experiments. LB with a purity of more than 98% was obtained from Fujian Xianzhilou Biological Science and Technology Co., Ltd. (Fuzhou, China).
Proliferation assessment using MTT, CFSE, and colony formation assays
Cell cytotoxicity was detected using the MTT assay (Yuan et al. Citation2016). Briefly, cells (CNE1, CNE2, and NIH3T3) in the logarithmic growth phase were seeded in 96-well plates at a density of 6 × 103 cells/well, respectively. Cells were treated with LB in the concentration range of 5–80 μg/mL for 24, 48, and 72 h. Next, MTT solution (20 μL, 5 mg/mL) was added to each well and incubated for 4 h at 37 °C. Then the medium was discarded and 150 µL DMSO was added to dissolve the formazan crystals. Finally, viable cells were detected by measuring absorbance at 570 nm using a Model 550 Microplate Reader (Bio-Rad, Hercules, CA, USA). The IC50 value, the drug concentration causing 50% cell growth inhibition, was estimated from survival curves using the logit method.
The colony formation assay was determined using a previously described method (Zhang et al. Citation2022). CNE2 cells were seeded in 12-well plates at a density of 3 × 105 cells/well and left overnight in an incubator at 37 °C. When the cells became adherent, various concentrations of LB were added to the wells and further incubated for 12 h. The cells were resuspended and then seeded in 12-well plates at a density of 500 cells/well. After that, the cells were cultured in an incubator for another 14 d to form colonies. After 14 d, cells were stained with 0.5% (v/v) crystal violet in methanol. Images were captured using a stereomicroscope (model SZX16, Olympus, Tokyo, Japan) and the colony number was counted directly.
The CFSE assay was determined using a previously described method (Caballero et al. Citation2022). After culturing, CNE2 cells were washed with sterile PBS-BSA, resuspended in 1 mL CFDA-SE diluent and maintained at 37 °C for 15 min, protected from light. Then, the suspensions were washed twice with RPMI 1640 by centrifugation at 300 g for 10 min. Subsequently, CFSE-labeled cells at a density of 3 × 105 cells/well were seeded in 6-well plates, cultured, and treated with LB (10 μg/mL) for 72 h. Another 1 × 106 CFSE-labeled cells were fixed with 4% paraformaldehyde and stored at 4 °C away from light to be used as the parental cell control during detection. After treatment, cells from each well and the parental cell control were transferred to appropriate tubes and analyzed using a FACS Canto II flow cytometer (Becton-Dickinson, San Jose, CA, USA). The proliferation index was analyzed using ModFit software (Verity Software House, Topsham, USA).
Cell cycle analysis
CNE2 cells were seeded in 6-well plates at a density of 3 × 105 cells/well and left overnight in an incubator at 37 °C. When the cells became adherent, various concentrations of LB were added to the wells and they were further incubated for 48 h. The cells were then harvested and fixed in ice-cold absolute ethanol at 4 °C overnight. The fixed cells were washed three times with PBS and then stained darkly with PI. Immediately after a 30 min incubation in the dark, the stained cells were analyzed using the FACS Canto II flow cytometer.
Apoptosis assay
Apoptosis induced by LB was detected using annexin V-FITC/PI staining as previously described (Zhai et al. Citation2022). Briefly, CNE2 cells were seeded in 6-well plates at a density of 3 × 105 cells/well and left overnight in an incubator at 37 °C. When the cells became adherent, various concentrations of LB were added to the wells and they were further incubated for 48 h. The cells were harvested, washed with ice-cold PBS, and resuspended in 300 µL of binding buffer. Subsequently, 5 µL of annexin V-FITC and 5 µL of PI were added to the cell suspension. The suspended cells were then incubated for 15 min at room temperature in the dark for staining to occur. After that, the FACS Canto II flow cytometer was used to evaluate the stained cells.
Mitochondrial membrane potential (MMP, ΔΨm) detection
The alteration of ΔΨm in CNE2 cells was assessed using an MMP assay kit (Beyotime Biotechnology, Jiangsu, China) as previously described (Li et al. Citation2021). Briefly, CNE2 cells were seeded in 6-well plates at a density of 3 × 105 cells/well and left overnight in an incubator at 37 °C. When the cells became adherent, various concentrations of LB were added to the wells and they were further incubated for 24 h. The cells were then harvested and stained with JC-1 dye for 15 min. After being washed with buffer solution, the cells were observed under a Leica DM IL fluorescence microscope (Leica, Wetzlar, Germany) or analyzed using the FACS Canto II flow cytometer).
Mitochondrial permeability transition pore (mPTP) opening assay
The mPTP opening was detected using the mPTP assay kit (Bestbio Biotechnology, Shanghai, China) according to the manufacturer’s instructions. Briefly, CNE2 cells were seeded in 6-well plates at a density of 3 × 105 cells/well and left overnight in an incubator at 37 °C. When the cells became adherent, various concentrations of LB were added to the wells and the cells were further incubated for 24 h. The cells were then harvested and stained with BBcellProbe® M61 dye for 15 min. After the stained cells were washed with PBS, the fluorescence was observed using the Leica DM IL fluorescence microscope or analyzed using the FACS Canto II flow cytometer.
ROS detection
The alteration of ROS in CNE2 cells was assessed using an ROS assay kit (Beyotime Biotechnology) as previously described (Min et al. Citation2021). Briefly, CNE2 cells were seeded in 6-well plates at a density of 3 × 105 cells/well and left overnight in an incubator at 37 °C. When the cells became adherent, various concentrations of LB were added to the wells and the cells were further incubated for 24 h. The cells were harvested and stained with 2',7'-dichlorodihydrofluorescein diacetate (DCFH-DA) for 20 min. Afterwards, the stained cells were washed with PBS and the fluorescence was observed using the Leica DM IL fluorescence microscope or analyzed using the FACS Canto II flow cytometer.
Ca2+ content detection
The alteration of Ca2+ content in CNE2 cells was assessed using a calcium ion assay kit (Bestbio Biotechnology, Shanghai, China). Briefly, CNE2 cells were seeded in 6-well plates at a density of 3 × 105 cells/well and left overnight in an incubator at 37 °C. When the cells became adherent, various concentrations of LB were added to the wells and further incubated for 24 h. The cells were harvested and stained with 0.5 mL BBcellProbe® F03 dye for 30 min at 37 °C. After being washed three times with PBS, the cells were further incubated at 37 °C for 30 min. The fluorescence was observed using a Leica DM IL fluorescence microscope or analyzed using the FACS Canto II flow cytometer.
Western blotting
After 48 h of incubation with LB, CNE2 cells were harvested and lysed in RIPA lysis buffer (Beyotime Biotechnology). Protein concentrations were determined using a BCA protein assay kit (Beyotime Biotechnology). Equal amounts of protein were separated in a 12% SDS-PAGE gel and subsequently transferred to a PVDF membrane (Millipore, Billerica, MA, USA). The proteins were blocked in 5% skimmed milk in TBS-Tween 20 (TBST) for 1 h at room temperature and exposed to specific primary antibodies overnight at 4 °C. The membranes were then washed twice with TBST and probed with horseradish peroxidase-conjugated secondary antibody (Cell Signaling Technology) for 2 h at room temperature. Bound antibodies were visualized using enhanced chemiluminescence plus reagents (GE Healthycare, Pittsburgh, PA, USA) and these bands were analyzed using ImageJ software (National Institutes of Health, Bethesda, MD). All antibodies used in this study were as follows: Ras, c-Raf, p-c-Raf, Erk1/2, p-Erk1/2, GAPDH, caspase-9, caspase-3, Bcl-2, cleaved caspase-9, cleaved caspase-3, Bax and Cytc.
Statistical analysis
Statistical analysis was performed using SPSS version 22.0 software (IBM, Armonk, USA). All experiments were repeated three times and all data were expressed as mean ± standard deviation (mean ± SD). One-way ANOVA was used for statistical analysis and t-test was used for pairwise comparison. p < 0.05 was considered statistically significant (*: p < 0.05, **: p < 0.01, ***: p < 0.001).
Results
LB inhibited the proliferation of CNE2 cells
The structure of LB is shown in . The effects of LB on the cell proliferation in vitro were tested using MTT, colony formation, and CFSE stain assays. Following the treatment with 5–40 μg/mL LB for 24, 48, and 72 h, the viability of CNE2 cells significantly decreased in a dose- and time-dependent manner (). The IC50 values were 25.42 ± 0.87, 14.83 ± 0.93, and 11.60 ± 0.77 μg/mL in CNE2 cells treated with LB for 24, 48, and 72 h, respectively. In contrast, LB showed weak inhibition against CNE1 cells with an IC50 of more than 40 μg/mL. Additionally, LB showed low cytotoxic activity against normal NIH3T3 cells with an IC50 of more than 80 μg/mL (). Subsequently, we conducted a colony forming assay to further investigate the effect of LB on CNE2 cells. Compared with the control group, the number of cell colonies of CNE2 significantly decreased after LB treatment (20 and 40 μg/mL; ). Finally, the CFSE staining assay was performed using LB (10 μg/mL). Following 72 h of treatment, compared to the control, LB was significantly effective in reducing CNE2 cell proliferation (). The cell proliferation index was 12.70 ± 0.46 in the LB treatment group and 31.44 ± 1.73 in the control group. These results indicated that LB inhibited the proliferation of CNE2 cells.
Figure 1. LB inhibited the proliferation of CNE2 cells. (A) The structure of LB. (B) CNE1 and NIH3T3 cells were treated with LB (5, 10, 15, 20, 30, 40 and 80 μg/mL) for 48 h. The cell viability was measured by MTT assay. (C) CNE2 cells were treated with LB (5, 10, 15, 20, 30 and 40 μg/mL) for 24, 48 and 72 h. The cell viability was measured by MTT assay. (D) The images and quantification results of colony formation assay after treatment with LB (10, 20, and 40 μg/mL). (E) Proliferation test using CFDA-SE probe. Cells were treated with LB (10 μg/mL) for 72 h, flow cytometry was executed for the evaluation of the proliferation index (PI). (n = 3, *p < 0.05, **p < 0.01, ***p < 0.001 compared with control group).
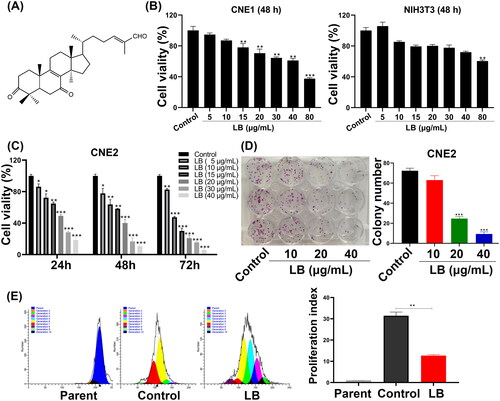
LB induced G2/M phase arrest in CNE2 cells
The impact of LB on cell cycle arrest was detected by PI staining and flow cytometry. A concentration-dependent increase in the percentage of cells arrested in the G2/M cell cycle was observed following treatment with LB (10 and 20 μg/mL) for 48 h (). Notably, the proportion of cells in the G2/M phase increased as LB concentration increased (10.00 ± 2.09% and 15.54 ± 3.04% for 10 μg/mL and 20 μg/mL LB, respectively vs. 6.39 ± 1.95% for the control). Thus, LB induces cell cycle arrest at the G2/M phase in CNE2 cells, which may be partly responsible for the inhibition of proliferation observed.
Figure 2. LB evoked cycle arrest at G2/M phase and inhibited Ras/ERK signaling pathway on CNE2 cells. (A) Cells were treated with LB (10 and 20 μg/mL) for 48 h. Flow cytometry was executed for the evaluation of the percentage of cells at G0/G1, S, and G2/M phases. (B) Cells were treated with LB (10, 20, and 40 μg/mL) for 48 h. Western blotting was implemented for the determination of Ras, c-Raf, p-c-Raf, Erk1/2, p-Erk1/2, and GAPDH expression in CNE2 cells. GAPDH was used as an internal control. (n = 3, *p < 0.05, **p < 0.01, ***p < 0.001 compared with the control group).
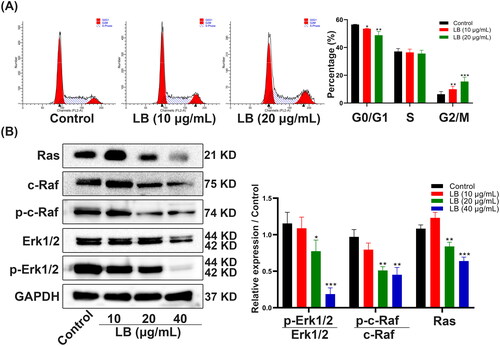
LB significantly inhibited the Ras/ERK signaling pathway
Activation of the Ras/ERK signaling pathway can promote the proliferation, invasion and metastasis of nasopharyngeal carcinoma cells (Sun et al. Citation2014). Therefore, we explored the effect of LB on the Ras/ERK signaling pathway using western blotting. We found that the protein levels of Ras, c-Raf and Erk1/2 decreased as the concentration of LB increased (20 and 40 μg/mL). Further, the phosphorylation of c-Raf and Erk1/2 also decreased with an increase in the dose (). These data implied that the inhibition of LB on CNE2 cells proliferation might be associated with inhibition of the Ras/ERK signaling pathway.
LB induced the apoptosis of CNE2 cells via the mitochondrial pathway
To investigate the action and mechanism of LB on the apoptosis of CNE2 cells, we performed an annexin V-FITC/PI double staining assay and detected the expression of cell apoptosis-related proteins using western blotting. Compared with the control group, after treatment with LB for 48 h, the percentages of early and late apoptotic cells significantly increased (). After LB treatment, the protein expression levels of caspase-3 and Bcl-2 were significantly downregulated while the protein expression levels of cleaved caspase-9, cleaved caspase-3, Bax, and Cytc were significantly upregulated (). The results indicated that LB may induce the apoptosis of CNE2 cells through the mitochondrial pathway, and to further investigate this hypothesis, we detected the changes of MMP reported in association with mitochondrial injury (Fulda Citation2015) under LB treatment. We applied the fluorescent dye JC-1 to detect the changes in MMP. After treatment with LB for 24 h, the red/green fluorescence ratio of CNE2 cells decreased from 2.47 ± 0.24 (control) to 2.11 ± 0.19 (10 μg/mL) and 1.84 ± 0.06 (20 μg/mL), suggesting that LB resulted in the loss of MMP (). Previous studies have reported that the opening of the mitochondrial permeability transition pore (mPTP) plays an important role in the mitochondria pathway of apoptosis (Baines Citation2009). In the present study, we performed BBcellProbe® M61 staining to explore whether mPTP opening occurred during LB-induced CNE2 cell apoptosis. Compared with the control group, the LB treatment resulted in a significant reduction of green fluorescence from 1.76 ± 0.15 (control) to 1.20 ± 0.04 (10 μg/mL) and 0.51 ± 0.12 (20 μg/mL), indicating that LB induced mPTP opening in CNE2 cells (). Taken together, these findings suggested that LB induced CNE2 cell apoptosis through the mitochondrial pathway.
Figure 3. LB induced apoptosis in CNE2 cells. (A & B) Cells were treated with LB (10, 20, and 40 μg/mL) for 48 h. (A) The apoptotic cells were stained with Annexin V/PI and analyzed using flow cytometry. (B) Western blotting was implemented for the determination of Cytc, Bax, Bcl-2, caspase-3, cleaved caspase-3, caspase-9, cleaved caspase-9 and GAPDH expression in CNE2 cells. GAPDH was used as an internal control. (n = 3, *p < 0.05, **p < 0.01, ***p < 0.001 compared with control group).
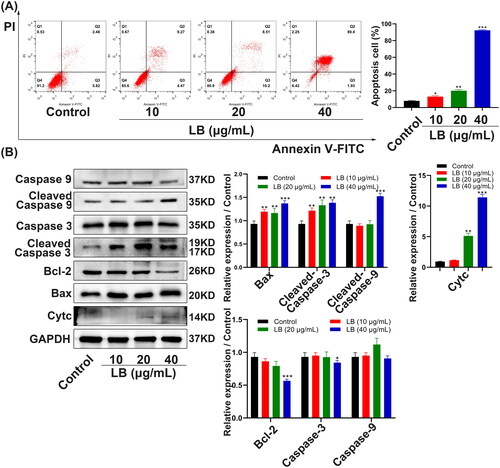
Figure 4. LB induced apoptosis via the mitochondrial pathway in CNE2 cells. (A & B) The effects of mitochondrial membrane potential (MMP) and mPTP opening in CNE2 cells were measured by flow cytometry and fluorescence microscope. (A) Cells were treated with LB (10 and 20 μg/mL) for 24 h and stained with JC-1 dye. Scale bar = 100 μm. (B) Cells were treated with LB (10 and 20 μg/mL) for 24 h and using the fluorescent probe BBcellProbe M61. Scale bar = 50 μm. MFI: mean fluorescence intensity. (n = 3, *p < 0.05, **p < 0.01, ***p < 0.001 compared with the control group).
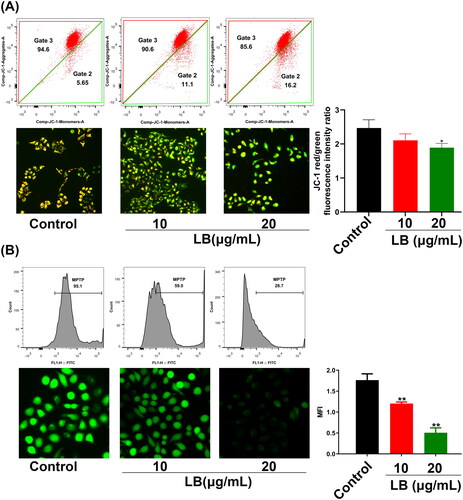
LB induced ROS and calcium aggregation in CNE2 cells
The excessive accumulation of ROS and calcium in tumor cells could trigger the mitochondrial apoptotic pathway (Huang et al. Citation2009; Moloney and Cotter Citation2018). CNE2 cells were treated with LB (10 and 20 μg/mL) for 24 h and then stained with a DCFH-DA probe for ROS detection and a BBcellProbe® F03 probe for Ca2+ detection. The fluorescence measured by flow cytometry and fluorescence microscopy reflected the ROS levels or Ca2+ content in CNE2 cells. Compared with the control group, the green fluorescence in the LB group significantly increased in both experiments, suggesting that LB induced ROS aggregation and Ca2+ overload in CNE2 cells (). Thus, these results indicated that LB significantly affected ROS levels and Ca2+ content in CNE2 cells and these effects were closely related to apoptosis.
Figure 5. LB induced ROS aggregation and calcium overload in CNE2 cells. (A & B) Cells were treated with LB (10 and 20 μg/mL) for 24 h. The fluorescence intensity measured by flow cytometry and fluorescence microscopy reflected the ROS levels or Ca2+ content in CNE2 cells. (A) Using the fluorescent probe DCFH-DA for ROS levels determination. Scale bar = 50 μm. MFI: mean fluorescence intensity. (B) Using the fluorescent probe BBcellProbe F03 for Ca2+ content determination. Scale bar = 50 μm. (n = 3, *p < 0.05, **p < 0.01, ***p < 0.001 compared with the control group).
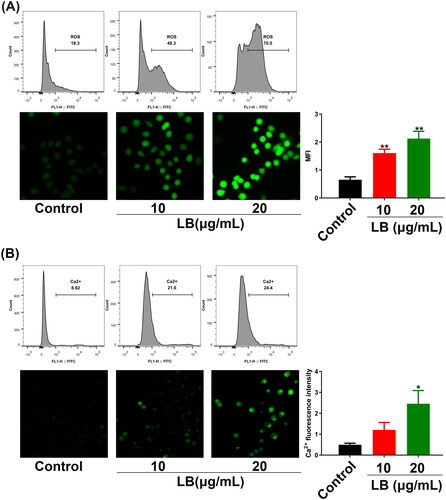
Discussion
Triterpenoids, a class of terpenoids, play an important role in the bioactivity of G. lucidum. To date, more than 150 triterpenoids have been identified from the fruiting bodies, spores, and mycelia of G. lucidum (Zhao et al. Citation2016). The antiproliferative effects of G. lucidum triterpenoids have been demonstrated in various human tumor cells such as leukemia, bladder cancer, breast cancer, cervical cancer, colorectal cancer, gastric cancer, and hepatoblastoma cells (Liang et al. Citation2019). G. lucidum triterpenoids also showed inhibitory effects on nasopharyngeal carcinoma. CNE1 and CNE2 are two nasopharyngeal carcinoma cell lines with distinct degrees of differentiation, which are often used in the research of nasopharyngeal carcinoma. CNE1 is a highly differentiated human nasopharyngeal carcinoma cell line, and CNE2 is a poorly differentiated human nasopharyngeal carcinoma cell line (Chen et al. Citation2019). In the present study, LB, a triterpenoid from G. lucidum, inhibited the nasopharyngeal carcinoma CNE2 cells in a dose- and time-dependent manner as detected using the MTT assay. Subsequently, the CFSE and colony formation assays further confirmed that LB had an antiproliferation effect on nasopharyngeal carcinoma CNE2 cells. In contrast, LB showed weak inhibitory activity against CNE1 cells.
G. lucidum triterpenoids exhibit a wide variety of antitumor activities by blocking the cell cycle, inducing apoptosis and autophagy, and inhibiting tumor metastasis and angiogenesis (Liu et al. Citation2020). The Ras/ERK signaling pathway is an important signaling system mediating cell responses in mammals and plays an important role in the survival, proliferation, differentiation, and apoptosis of tumor cells (Ali et al. Citation2022). Activation of the Ras/ERK signaling pathway can promote proliferation, invasion, and metastasis as well as inhibit the apoptosis of nasopharyngeal carcinoma cells (Ooft et al. Citation2015). In the present study, LB showed a dose-dependent reduction in the protein expression of Ras, c-Raf, Erk1/2 and their phosphorylated products, p-c-Raf and p-Erk1/2, suggesting that LB could inhibit the proliferation of nasopharyngeal carcinoma CNE2 cells by inhibiting the Ras/ERK signaling pathway.
Mitochondria play a very important role in the endogenous apoptosis pathway, also known as the mitochondrial pathway (Roca-Agujetas et al. Citation2019). The mitochondrial permeability transition pore (mPTP) is a nonspecific channel composed of protein complexes between the inner and outer membranes of the mitochondria. The opening and closing of mPTP at a normal level maintains the permeability of the mitochondrial membrane. Many ions, water, and other substances freely diffuse into mitochondria, causing mitochondrial swelling and outer membrane rupture, which results in the release of Cytc and AIF into the cytoplasm (Izzo et al. Citation2016). Subsequently, Cytc interacts with apoptotic protease activating factor-1 (Apaf-1) to activate caspase-9. Cleaved caspase-9 activates caspase-3 and cleaved caspase-3 is an essential indicator of apoptosis. Next, cleaved caspase-3 activates PARP and cleaved PARP induces apoptosis by inhibiting DNA repair (Simbulan-Rosenthal et al. Citation1999; Xu et al. Citation2020). In this study, the annexin V-FITC/PI double staining assay first demonstrated the pro-apoptotic effect of LB and then western blotting found that LB significantly downregulated Bcl-2 and caspase-3 and upregulated Cytc, Bax, cleaved caspase-9, and cleaved caspase-3 in CNE2 cells in vitro. Therefore, these results indicated that LB induced CNE2 cell apoptosis through the mitochondrial pathway.
ROS mainly originate from the mitochondria and the mitochondria are the main site of ROS-induced damage (Farías et al. Citation2017). The presence of a large number of ROS oxidizes the thiol group of adenine nucleotide translocase (ANT) and causes the excessive opening of mPTP, which leads to an increase in the permeability of the mitochondrial membrane and a decrease in MMP (Boyman et al. Citation2019). As a multifunctional second messenger, Ca2+ plays an essential role in the production of ROS (Sabharwal and Schumacker Citation2014). The excessive accumulation of mitochondrial Ca2+ may also trigger mPTP opening, thereby decreasing the MMP (Wang et al. Citation2019). As cell-death inducers, Ca2+ and ROS can activate the mitochondrial pathway (Dong et al. Citation2017). The results of the present study showed that LB significantly increased the ROS level and Ca2+ content in CNE2 cells, suggesting that LB activated the mitochondrial pathway to induce CNE2 cells apoptosis through the accumulation of ROS and calcium.
In general, we explored the antiproliferative and apoptosis-inducing effects and mechanisms of LB on CNE2 cells in vitro. However, the effects and mechanisms of LB on CNE2 cells in vivo need to be further explored. This is the limitation of our research and also the intended direction of our future work.
Conclusions
In this study, we demonstrated that LB suppresses proliferation of CNE2 cells through inhibition of the Ras/ERK signaling pathway and induces apoptosis through activation of the mitochondrial pathway. Our data provided experimental evidence for the potential use of LB as a clinical drug candidate for nasopharyngeal carcinoma treatment.
Author contributions
Peng Li conceived and designed the study. Lingxue Liu and Zhangning Yu performed the experiments. Lingxue Liu, Peng Li, and Benchen Liu wrote the manuscript. Jing Chen analyzed the data. Changhui Wu collected the samples. Jianhua Xu and Ye Li coordinated technical support.
Disclosure statement
No potential conflict of interest was reported by the author(s).
Additional information
Funding
References
- Ahmad MF. 2018. Ganoderma lucidum: ersuasive biologically active constituents and their health endorsement. Biomed Pharmacother. 107:507–519.
- Ahmad R, Riaz M, Khan A, Aljamea A, Algheryafi M, Sewaket D, Alqathama A. 2021. Ganoderma lucidum (Reishi) an edible mushroom; a comprehensive and critical review of its nutritional, cosmeceutical, mycochemical, pharmacological, clinical, and toxicological properties. Phytother Res. 35(11):6030–6062.
- Ali ES, Akter S, Ramproshad S, Mondal B, Riaz TA, Islam MT, Khan IN, Docea AO, Calina D, Sharifi-Rad J, et al. 2022. Targeting Ras-ERK cascade by bioactive natural products for potential treatment of cancer: an updated overview. Cancer Cell Int. 22(1):246.
- Baines CP. 2009. The molecular composition of the mitochondrial permeability transition pore. J Mol Cell Cardiol. 46(6):850–857.
- Boyman L, Coleman AK, Zhao G, Wescott AP, Joca HC, Greiser BM, Karbowski M, Ward CW, Lederer WJ. 2019. Dynamics of the mitochondrial permeability transition pore: Transient and permanent opening events. Arch Biochem Biophys. 666:31–39.
- Caballero M, Barreto N, Bonfanti AP, Munhoz J, Rocha E, Silva T, Sutti R, Verinaud L, Pinheiro de Mato FC, Lanfredi GP. 2022. Isolated components from spider venom targeting human glioblastoma cells and its potential combined therapy with rapamycin. Front Mol Biosci. 9:752668.
- Chen Y, Chen Z, Su Y, Lin DH, Chen M, Feng SY, Zou CY. 2019. Metabolic characteristics revealing cell differentiation of nasopharyngeal carcinoma by combining NMR spectroscopy with Raman spectroscopy. Cancer Cell Int. 19:37.
- Chen YP, Chan ATC, Le QT, Blanchard P, Sun Y, Ma J. 2019. Nasopharyngeal carcinoma. Lancet. 394(10192):64–80.
- Dong ZW, Shanmughapriya S, Tomar D, Siddiqui N, Lynch S, Nemani N, Breves SL, Zhang XQ, Tripathi A, Palaniappan P, et al. 2017. Mitochondrial Ca2+ uniporter is a mitochondrial luminal redox sensor that augments MCU channel activity. Mol Cell. 65(6):1014–1028.e7.
- Farías JG, Molina VM, Carrasco RA, Zepeda AB, Figueroa E, Letelier P, Castillo RL. 2017. Antioxidant therapeutic strategies for cardiovascular conditions associated with oxidative stress. Nutrients. 9(9):966.
- Fulda S. 2015. Targeting apoptosis for anticancer therapy. Semin Cancer Biol. 31:84–88.
- Gao JJ, Min BS, Ahn EM, Nakamura N, Lee HK, Hattori M. 2002. New triterpenoid aldehydes, lucialdehydes A-C, from Ganoderma lucidum and their cytotoxicity against murine and human tumor cells. Chem Pharm Bull (Tokyo). 50(6):837–840.
- Huang CC, Cheng HH, Lin KL, Cheng JS, Tsai JY, Liao WC, Fang YC, Jan CR. 2009. Tamoxifen-induced [Ca2+]i rise and apoptosis in corneal epithelial cells. Toxicology. 255(1-2):58–64.
- Huang XW, Zhang XP, Liu F, Li P, Xu JH, Li MM. 2014. [ Effect of triterpenoid GLE from Ganoderma lucidum on apoptosis induction via mitochondrial pathway in CNE2 cells]. Chin J Hosp Pharm. 34:867–869. Chinese
- Izzo V, Bravo-San Pedro JM, Sica V, Kroemer G, Galluzzi L. 2016. Mitochondrial permeability transition: new findings and persisting uncertainties. Trends Cell Biol. 26(9):655–667.
- Li P, Liu LX, Huang S, Zhang YH, Xu JH, Zhang ZQ. 2020. Anti-cancer effects of a neutral triterpenoid fraction from Ganoderma lucidum and its active constituents on SW620 human colorectal cancer cells. Anticancer Agents Med Chem. 20(2):237–244.
- Li YB, He PZ, Liu YH, Qi MM, Dong WG. 2021. Combining sodium butyrate with cisplatin increases the apoptosis of gastric cancer in vivo and in vitro via the mitochondrial apoptosis pathway. Front Pharmacol. 12:708093.
- Liang CY, Tian DN, Liu YZ, Li H, Zhu JL, Li M, Xin MH, Xia J. 2019. Review of the molecular mechanisms of Ganoderma lucidum triterpenoids: Ganoderic acids A, C2, D, F, DM, X and Y. Eur J Med Chem. 174:130–141.
- Liu W, Yuan RY, Hou A, Tan S, Liu X, Tan PC, Huang XM, Wang JG. 2020. Ganoderma triterpenoids attenuate tumor angiogenesis in lung cancer tumor-bearing nude mice. Pharm Biol. 58(1):1070–1077.
- Min FH, Liu X, Li Y, Dong MY, Qu YD, Liu WW. 2021. Carnosic acid suppresses the development of oral squamous cell carcinoma via mitochondrial-mediated apoptosis. Front Oncol. 11:760861.
- Moloney JN, Cotter TG. 2018. ROS signalling in the biology of cancer. Semin Cell Dev Biol. 80:50–64.
- Niedermeyer TH, Lindequist U, Mentel R, Gördes D, Schmidt E, Thurow K, Lalk M. 2005. Antiviral terpenoid constituents of Ganoderma pfeifferi. J Nat Prod. 68(12):1728–1731.
- Ooft ML, Braunius WW, Heus P, Stegeman I, van Diest PJ, Grolman W, Zuur CI, Willems SM. 2015. Prognostic significance of the EGFR pathway in nasopharyngeal carcinoma: a systematic review and meta-analysis. Biomark Med. 9(10):997–1010.
- Roca-Agujetas V, de Dios C, Lestón L, Marí M, Morales A, Colell A. 2019. Recent insights into the mitochondrial role in autophagy and its regulation by oxidative stress. Oxid Med Cell Longev. 2019:3809308.
- Yuan F, Bai GG, Miao YJ, Chen Y, Li X, Chen JW. 2016. Annosquacin B induces mitochondrial apoptosis in multidrug resistant human breast cancer cell line MCF-7/ADR through selectively modulating MAPKs pathways. Pharm Biol. 54(12):3040–3045.
- Sabharwal SS, Schumacker PT. 2014. Mitochondrial ROS in cancer: initiators, amplifiers or an Achilles’ heel? Nat Rev Cancer. 14(11):709–721.
- Siak PY, Khoo ASB, Leong CO, Hoh BP, Cheah SC. 2021. Current status and future perspectives about molecular biomarkers of nasopharyngeal carcinoma. Cancers (Basel). 13(14):3490.
- Simbulan-Rosenthal CM, Rosenthal DS, Iyer S, Boulares H, Smulson ME. 1999. Involvement of PARP and poly(ADP-ribosyl)ation in the early stages of apoptosis and DNA replication. Mol Cell Biochem. 193(1-2):137–148.
- Sun W, Long GX, Wang JF, Mei Q, Liu DB, Hu GQ. 2014. Prognostic role of epidermal growth factor receptor in nasopharyngeal carcinoma: a meta-analysis. Head Neck. 36(10):1508–1516.
- Wang K, Chen BN, Yin T, Zhan YJ, Lu YH, Zhang YL, Chen JW, Wu WJ, Zhou SK, Mao WL, et al. 2019. N-Methylparoxetine blocked autophagic flux and induced apoptosis by activating ROS-MAPK pathway in non-small cell lung cancer cells. IJMS. 20(14):3415.
- Wang L, Li JQ, Zhang J, Li ZM, Liu HG, Wang YZ. 2020. Traditional uses, chemical components and pharmacological activities of the genus Ganoderma P. Karst.: a review. RSC Adv. 10(69):42084–42097.
- Xu YM, Li Z, Zhang S, Zhang HF, Teng XH. 2020. miR-187-5p/Apaf-1 axis was involved in oxidative stress-mediated apoptosis caused by ammonia via mitochondrial pathway in chicken livers. Toxicol Appl Pharmacol. 388:114869.
- Yang ZM, Zhang QH, Yu LH, Zhu JY, Cao Y, Gao XF. 2021. The signaling pathways and targets of traditional Chinese medicine and natural medicine in triple-negative breast cancer. J Ethnopharmacol. 264:113249.
- Yu ZN, Li P, Huang YY. 2022. [Chemical constituents of Ganoderma lucidum and their cytotoxic activities against human nasopharyngeal carcinoma CNE2 cells]. Shenzhen J Integr Tradit Chin West Med. 32:7–10. (Chinese).
- Zhai YF, Sun J, Sun CT, Zhao H, Li X, Yao JX, Su JJ, Xu XQ, Xu XK, Hu JN, et al. 2022. Total flavonoids from the dried root of Tetrastigma hemsleyanum Diels et Gilg inhibit colorectal cancer growth through PI3K/AKT/mTOR signaling pathway. Phytother Res. 36(11):4263–4277.
- Zhang K, Ying HY, Zhao RP, Chen YY, Deng QH. 2022. Capilliposide from Lysimachia capillipes promotes terminal differentiations and reverses paclitaxel resistance in A2780T cells of human ovarian cancer by regulating Fos/Jun pathway. Chin Herb Med. 14(1):111–116.
- Zhao XR, Zhang BJ, Deng S, Zhang HL, Huang SS, Huo XK, Wang C, Liu F, Ma XC. 2016. Isolation and identification of oxygenated lanostane-type triterpenoids from the fungus Ganoderma lucidum. Phytochem Lett. 16:87–91.