Abstract
Context
Huangqi Guizhi Wuwu Decoction (HGWD) is effective in treating ischaemic stroke (IS). However, its mechanism of action is still unclear.
Objective
Network pharmacology integrated with in vivo experiments were used to clarify the underlying mechanisms of HGWD for treating IS.
Materials and methods
TCMSP, GeneCards, OMIM and STRING were used to retrieve and construct visual protein interaction networks for the key targets. The AutoDock tool was used for molecular docking between key targets and active compounds. The neuroprotective effect of HGWD were verified in a middle cerebral artery occlusion (MCAO) model rat. The Sprague-Dawley (SD) rats were divided into sham, model, low-dose (5 g/kg, i.g.), high-dose (20 g/kg, i.g.), and nimodipine (20 mg/kg, i.g.) groups once daily for 7 days. The neurological scores, brain infarct volumes, lipid peroxidation, inflammatory cytokines, Nissl bodies, apoptotic neurons, and signalling pathways were all investigated and evaluated in vivo.
Results
Network pharmacology identified 117 HGWD targets related to IS and 36 candidate compounds. GO and KEGG analyses showed that HGWD anti-IS effects were mainly associated with PI3K-Akt and HIF-1 signalling pathways. HGWD effectively reduced the cerebral infarct volumes (19.19%), the number of apoptotic neurons (16.78%), and the release of inflammatory cytokines, etc. in MCAO rats. Furthermore, HGWD decreased the levels of HIF-1A, VEGFA, Bax, cleaved caspase-3, p-MAPK1, and p-c-Jun while increasing the expression of p-PI3K, p-AKT1, and Bcl-2.
Discussion and conclusion
This study initially elucidated the mechanism of HGWD anti-IS, which contributed to the further promotion and secondary development of HGWD in clinical practice.
Introduction
Stroke is extremely damaging because of the high rate of illness, death, and disability it causes (Yang et al. Citation2021). IS and haemorrhagic stroke are two kinds of stroke that can be distinguished from one another. IS was responsible for approximately 87% of strokes in China (Wu et al. Citation2019). The occlusion of cerebrovascular or low blood perfusion is the aetiology of IS, which prevents the flow of oxygen, blood, and other nutrients, resulting in the corresponding loss of neurologic function (Benjamin et al. Citation2019). Therefore, one of the most critical therapeutic concepts for clinical treatment of stroke is to restore blood flow as soon as possible (Liu et al. Citation2010). Thrombolysis, neurotrophic factor delivery to preserve nerve cells, and symptomatic therapy are now the most common clinical therapies for acute stroke. Because of the acute phase’s short window, the majority of patients with stroke are not candidates for these therapies (George and Steinberg Citation2015; Prabhakaran et al. Citation2015). Furthermore, a therapeutic strategy like thrombolysis can restore short-term blood flow, which may exacerbate inflammation or neuroexcitatory toxicity and run the risk of causing cerebral ischaemia-reperfusion damage (Xu et al. Citation2017). Therefore, it’s vital to find a supplementary or alternative treatment for ischaemic stroke.
In the presence of hypoxia, the brain reacts in a variety of ways. One of the most notable is the production of hypoxia proteins, such as the Hypoxia-inducible factor-1 (HIF-1) (Baranova et al. Citation2007). HIF-1 activity is dependent on the potency and activity of the oxygen regulatory subunit HIF-1α (Ke and Costa Citation2006). HIF-1α achieves oxygen-stable transcriptional regulation and adaptive responses by regulating some downstream target genes, such as erythropoietin, VEGF, and glucose transporter (Chen et al. Citation2015). Furthermore, HIF-1 levels were positively linked with the severity of IS in clinical investigations. HIF-1 might be a predictor of clinical prognosis in IS patients, with higher HIF-1 levels indicating a worse outcome (Amalia et al. Citation2020). Therefore, a better knowledge of HIF-1’s involvement in IS is required to enhance therapy strategies and pharmaceutical development.
Using a “multi-component, multi-target, and multi-pathway synergistic mechanism” treatment strategy, traditional Chinese medicine (TCM) has been practiced in China for over 3000 years (Li et al. Citation2014; Zhang et al. Citation2015). By testing the neuroprotective effects of TCM on the IS models, it is possible to find new potential neuroprotective agents. HGWD was first published in Zhang Zhongjing’s “Jingui Yaolue” during the Eastern Han Dynasty. HGWD contains Astragalus membranaceus (Fisch.) Bge. (Fabaceae), Cinnamomum cassia Presl (Lauraceae), Paeonia lactiflora Pall. (Ranunculaceae), Zingiber officinale Rose. (Zingiberaceae), and Ziziphus jujuba Mill. (Rhamnaceae). In recent years, HGWD has achieved satisfactory clinical results in the treatment of “blood stasis” characterized by numbness pain and rough pulse (Zheng et al. Citation2020). As shown in the prior research, HGWD significantly enhanced neurological and motor performance in stroke patients, promoted independence in self-care, and lessened the symptoms of shoulder-hand syndrome (Ma et al. Citation2016; Jiang et al. Citation2017; Yin and Ren Citation2017). Therefore, HGWD have promising therapeutic benefits when used for the treatment of IS. However, the material foundation and mechanisms of HGWD in the treatment of IS have not yet been clarified, which is a major impediment that inhibits its broad application.
Because of major advances in bioinformatics and poly-pharmacology, network-based drug discovery is now considered as a feasible strategy for cost-effective drug development (Shen et al. Citation2020). Network pharmacology is an approach to examine formulation processes in vivo, comprehend formulation-disease relationships, and assist experimental studies (Boezio et al. Citation2017). According to TCM’s holistic viewpoint, it explores the molecular mechanism of formulation by utilizing a “disease-target-herb” network model (Kibble et al. Citation2015; Xu et al. Citation2021). It involves creating a “compound-target-pathway” network to assess the influence of bioactive substances and targets. Applying network pharmacology to find the key targets for ischaemic stroke is a good strategy. (Wang et al. Citation2022). The present research employs a network informatics technique to analyse the mechanisms of HGWD against IS and prove its effectiveness as a treatment for IS.
The chemical composition of HGWD was initially gathered from the Traditional Chinese Medicine Systems Pharmacology (TCMSP) database and literature mining in this study. The bioactive ingredients in HGWD were evaluated for pharmacokinetic properties, and then fed into TCMSP to determine their targets. Additionally, GeneCards and OMIM were used to search for IS targets. “Compound-targets-disease” and “target-pathway” networks were established. Molecular docking and experimental verification were also applied to investigate the bioactive ingredients and mechanism of HGWD against IS.
Materials and methods
Network pharmacology
Collection of active compounds and their corresponding targets
The main ingredients of each herb in the prescription of HGWD were collected by searching TCMSP (https://old.tcmsp-e.com/tcmsp.php) and literature mining (Ru et al. Citation2014). ADME analysis was performed on each component. The inclusion criteria for screening potential active components were oral bioavailability (OB) > 30% and drug-like properties (DL) > 0.18. The PubChem (https://pubchem.ncbi.nlm.nih.gov/) and ChemDraw databases were utilized to obtain the chemical formulas for bioactive substances (Kim et al. Citation2016). The files were all stored in MOL2 format. SwissTarget Prediction (http://www.swisstargetprediction.ch/) was applied to predict active component targets with a species boundary of “Homo sapiens” (Daina et al. Citation2019). An online database called UniProt (https://www.uniprot.org/) was utilized to identify the gene name and gene ID of the targets (UniProt Citation2021).
IS-Related targets
The OMIM (http://omim.org/) and GeneCards (https://www.genecards.org/) databases were utilized to find the IS-related targets for this research (Safran et al. Citation2010; Amberger et al. Citation2015). Accordingly, we chose the terms “ischaemic stroke,” “stroke,” “cerebral ischaemic stroke,” and “cerebral infarction” to screen for targets relevant to IS. All retrieved data were gathered and duplicate outcomes were deleted to create the target database for IS. The Venn platform (http://bioinformatics.psb.ugent.be/webtools/Venn/) was utilized to discover overlapping genes of active ingredients and IS, which were analysed in further investigation (Wang et al. Citation2014).
Creating a PPI network
A protein-protein interaction (PPI) analysis was conducted among the common targets to identify the core genes for HGWD treatment. The STRING database (https://string-db.org/) is known to hold a large amount of experimental data as well as the results of text mining, and it can employ bioinformatics to forecast a variety of outcomes (Szklarczyk et al. Citation2017). The Cytoscape 3.9.0 and STRING database were used to perform PPI analysis in this research.
Gene ontology (GO) and Kyoto encyclopedia of genes and genomes (KEGG) pathway analyses
To investigate the functional annotation and involved pathways of core genes identified in the PPI analysis, the Metascape (http://metascape.org/gp/index.html) database was utilized to conduct GO and KEGG enrichment (Zhou et al. Citation2019).
Network construction
A “herb-ingredient-target-disease” network was constructed in Cytoscape 3.9.0 when the common targets of HGWD and IS were identified. Based on the results of KEGG enrichment, the top 20 pathways were selected to explore the relationship between core targets and pathways, and a “core target-pathway” network was established. The Network nalyzer, a plugin for Cytoscape, was used to assess the network’s topological properties.
Molecular docking
In this study, we ran molecular docking of compounds and targets with the help of AutoDock Vina 1.1.2. The RCSB PDB (http://www.rcsb.org/) was employed to obtain 3D protein structure files (Prlic et al. Citation2010). 2D structures of the ligand molecules were acquired from PubChem, optimized by Chem3D, and saved as Mol2 files. First, protein receptors and docking ligands were prepared in PyMOL2.4 by removing water, extracting ligands and ions. AutoDock Tools 1.5.6 converted protein receptors and ligands to pdbqt format. Finally, we utilized Autodock Vina to conduct molecular docking and calculate the affinity between a ligand and each protein receptor.
Chemicals and reagents
The Astragalus membranaceus, Cinnamomum cassia, Paeonia lactiflora, Zingiber officinale, and Ziziphus jujuba used in the HGWD formulation were all obtained from Guangzhou Zhixin Co., Ltd. (180,402-180,602). All herbs were complied with the 2020 Chinese Pharmacopoeia and their authenticity were verified by Professor Danyan Zhang from Guangzhou University of Chinese Medicine. The crude herbs (Astragalus membranaceus, Cinnamomum cassia, Paeonia lactiflora, Zingiber officinale, and Ziziphus jujuba) were mixed in a ratio of 1:1:1:2:1 and placed in 10 times their volume of water for 0.5 h. After that, the herbs were twice decocted for 1 h each time. After combining, filtering, and concentrating the water-based decoctions to a concentration of 2.5 g/mL, they were then frozen at −80 °C for use in the following studies. High-performance liquid chromatography (HPLC) was employed for the purpose of HGWD quality control.
Primary antibodies against AKT1(AF0836), p-AKT1(AF0016), MAPK1(BF0412), p-MAPK1(AF1015), c-Jun(AF6090), p-c-Jun(AF3095), p-PI3K(AF3242), PI3K(AF6242), Bcl-2(AF6139), Bax(AF0120), β-actin (T0022), β-tubulin (T0023), and secondary antibodies goat anti-rabbit IgG (S0001) were acquired from Affinity (Cincinnati, OH, USA). VEGFA (66828-1-Ig) and HIF-1A (66730-1-Ig) were acquired from Proteintech (Rosemont, IL, USA). Cell Signalling Technologies (Danvers, MA, USA) provided the primary antibodies against caspase-3 (9662s) for this study. The secondary antibodies were purchased from Sino Biological, Inc. (SSA004 and SSA005; Beijing, China). Nanjing Jiancheng Bioengineering Institute (Nanjing, Jiangsu, China) provided LDH (A020-2-2), MDA (A003-1-2), and SOD (A001-3-2) assay kits. TNF-α (MM-0180R1), IL-6 (MM-0190R1), and IL-1β (MM-0047R1) ELISA kits were purchased from Jiangsu Meimian Industrial Co., Ltd. (Yancheng, Jiangsu, China).
Animal experiment
Animals
Adult male SD rats, weighing 230 ± 20 g, were obtained from Beijing Huafukang Biotechnology Co., Ltd (Guangdong, China, SYXK (YUE) 2019-0202). The rats were kept at 22.5 ± 2.5 °C and 40%–70% humidity for 12 h light/dark cycles, with adequate water and food. In accordance with national and international guidelines, the animal experiments were conducted in compliance with instructions authorized by the Animal Health and Ethics Committee of the College of Chinese Materia Medica of Guangzhou University of Chinese Medicine (Guangdong, China). The ethics review form number is ZYD-2021-265.
Establishment of MCAO model and experimental design
The MCAO rat model was established as previously described (Liao et al. Citation2016). Briefly, the SD rats were anesthetized by intraperitoneal injection of 2% sodium pentobarbital (40 mg/kg) and their body temperature was maintained at 37 °C with a heating blanket. Depending on the animal’s weight and carotid diameter, a 40 mm nylon thread was delivered from the right common carotid artery to the lumen of the internal carotid artery at a depth of 22 mm to block the right middle cerebral artery under deep anaesthesia. The implanted thread was carefully removed after 1.5 h to restore blood flow. The MCAO rats were then returned to their original surroundings to await reawakening. After the surgery, the animals’ health conditions were monitored daily. The animals with higher levels of pain were housed in separate cages or given appropriate amounts of penicillin to prevent infection, thus minimizing pain and suffering. Rats that did not meet the criteria were then excluded based on the scoring criteria to minimize potential confounding factors. The sham group only performed surgical procedures without inserting thread.
Fifty male rats were randomly assigned to the sham, model, low-dose (5 g/kg), high dose (20 g/kg) of HGWD, and nimodipine groups by using a random classification. According to the weight of rats, the low-dose and high-dose groups were, respectively, treated with 5 g/kg and 20 g/kg HGWD by intragastric administration once daily. The nimodipine group received 20 mg/kg HGWD by intragastric administration. In the sham and model groups, rats were given a single dose of normal saline solution by oral gavage daily. All groups were administered continuously for 7 days.
Neurological deficit evaluation
After 24 h of operation, the MCAO rats were selected and scored using the grading criteria established by Longa et al. (Citation1989). The grading standard was listed as follows: Score 0: normal, no neurological damage; score 1: unable to stretch left forepaw; score 2: incline to the left while walking; score 3: dump to the left while walking; score 4: unconsciousness. Rats that received a score of 0 or 4 were taken out of the study after operation.
LDH, MDA, and SOD level measurement
Following the manufacturer’s protocols, biochemical assay kits were used to examine the levels of LDH, MDA, and SOD in brain homogenate supernatant from rats.
Enzyme linked immunosorbent assay (ELISA)
ELISA kits were applied to assess rat serum levels of TNF-α, IL-6, and IL-1β according to the manufacturer’s protocols.
2,3,5-Triphenyl tetrazolium chloride (TTC) staining
Rats were decapitated under deep anaesthesia after 7 days of drug administration. The brains were promptly removed and then sliced into five coronal slices with a thickness of 2 mm. Then, coronal slices were dyed with 1% TTC for 20 min at 37 °C in the dark. The infarcted area was white, and the non-infarcted area was red-purple. Cerebral infarct areas were measured by ImageJ software (NIH, Bethesda, MD, USA). To determine infarct volume in the prior research (Liao et al. Citation2016), the infarct area was multiplied by its thickness.
Hematoxylin and eosin (HE) staining
After fixing the brains in paraformaldehyde at a concentration of 4% for a period of 24 h, the samples were dehydrated, embedded in paraffin, cut into a thickness of 4 μm, and stained with HE. Pathological histomorphology was observed with an inverted microscope (Jiangsu Kaiji Biotech., China).
Nissl staining
The slices from the right hemisphere were collected for Nissl staining. All slices were dewaxed, rehydrated, immersed in toluidine blue solution, washed with distilled water, and dehydrated with ethanol and xylene. The Nissl bodies were observed under an inverted microscope (Jiangsu Kaiji Biotech., China).
Terminal transferase biotinylated-dUTP nick end labelling (TUNEL) staining
TUNEL assay was performed using a TUNEL kit according to the manufacturer’s protocols (Kaiki, China). The percentage of TUNEL-positive cells was determined by analyzing images captured at high magnification (400×) from each group.
Western blotting
After the last neurological deficit evaluation, brain tissues were quickly removed from each group of rats and then homogenized. After quantifying the proteins, sodium dodecyl sulfate polyacrylamide gel electrophoresis (SDS-PAGE) with a 10% concentration gel was used to separate the proteins. A PVDF membrane (0.22 μm, Millipore, USA) was then used to transfer the proteins. Blocking was performed by incubating with a concentration of 5% fat-free dry milk for 1 h. After that, primary antibodies (1:1000), were, respectively, added to the membranes for incubation at 4 °C for overnight. Following washing with TBST 3 times, membranes were incubated with secondary antibodies (1:2000) for 1 h at 4 °C. Proteins were visualized using Tanon 5200 (Tanon Science & Technology Co., Ltd., Shanghai, China). ImageJ (NIH, Bethesda, MD, USA) was applied to calculate the integrated density of each band.
Statistical analysis
The findings of each group were shown as mean ± standard deviation (SD). Differences between two groups were analysed using the Student’s t-test, whereas multiple group differences were examined using one-way analysis of variance. The statistical work was conducted using SPSS 22.0 (SPSS, Inc., Chicago, IL, USA). p < 0.05 was deemed as statistically significant.
Results
Active compounds in HGWD
Based on the retrieval and screening criteria, 47 active ingredients in HGWD were obtained. 17 compounds were identified in Astragalus membranaceus, 6 in Cinnamomum cassia, 11 in Paeonia lactiflora, 5 in Zingiber officinale, and 19 in Ziziphus jujuba. Several herbs contain common ingredients. With the help of topological analysis, the 10 highest-degree ingredients were selected as active ingredients, including quercetin, β-sitosterol, kaempferol, etc. (). The Uniprot database was utilized to retrieve the gene names and gene IDs for 232 targets from 43 active HGWD components, whereas for 4 active components of HGWD there were no targets meeting the set criteria.
Table 1. The information of the top 10 active ingredients.
Candidate targets
Both the GeneCards and OMIM databases were utilized to collect and screen 981 genes associated with IS. Meanwhile, we collected 232 different target genes for HGWD. As shown in , the 117 overlapping targets were discovered from the intersection of HGWD and IS and classified as potential targets.
Figure 1. The primary therapeutic targets of HGWD for treating IS. (A) The Venn diagram displays shared and distinct IS and HGWD targets. (B) A network of PPI visualizations constructed with the STRING database. (C) Forty core target selections. (D) Cytoscape visualizes PPI network. (E) Cluster for the PPI network. (F) Cluster 1 (score = 5.26). (G) Cluster 2 (score = 2.61). (H) Cluster 3 (score = 2). (I) Cluster 4 (score = 1.6). (J) Cluster 5 (score = 1.5).
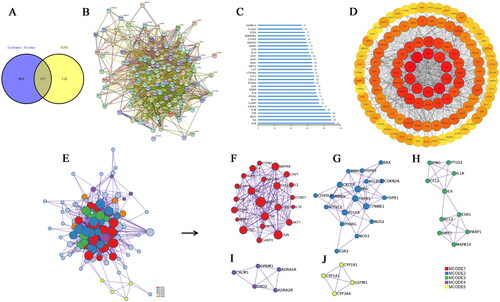
PPI network
It was used to evaluate the association between 117 intersection targets and generate a PPI visualization network using the STRING database . The PPI network was comprised of 116 nodes and 2138 edges after removing one free target. The top 30 core PPI targets were evaluated based on the network degree distribution, including ALB, AKT1, and IL-6 and so on (, ). Furthermore, the PPI network was partitioned into 5 clusters to explore the potential targets of HGWD against IS in . The nodes with bigger sizes and darker colours indicated the more significant role they played in the PPI network ().
Table 2. Key targets of HGWD acting on IS (top 30).
Network visualization
shows a “herbs-ingredients-targets-disease” network with 159 nodes and 577 edges to highlight the association among active ingredients, common targets, and IS. The size of the node in the network was positively linked to its degree value. The duplicate ingredients in several herbals and their corresponding targets were removed. Finally, 36 candidate compounds and 117 core targets were drawn from the network. A “core targets-pathways” network, seen in , includes the top 20 pathways. After a comprehensive analysis of these pathways, we concluded that the HIF-1 signalling pathway was strongly associated with the mechanism of HGWD anti-IS. The key targets are MAPK1, AKT1, IL-6, Jun, TNF, Bax, Bcl-2, caspase-3, HIF1A, IL-1β, PI3K and VEGFA.
GO and KEGG analyses
displays the results of GO analysis. The top 10 biological process (BP) terms were mainly a response to apoptosis, nitrogen compounds, hypoxia, and so on, indicating that the therapeutic effect of HGWD against IS was primarily associated with apoptosis, nitrogen compound regulation, and hypoxia (). The top 5 cellular component (CC) terms were membrane raft, endoplasmic reticulum lumen, side of membrane, extracellular matrix, and postsynaptic membrane (). It meant that HGWD mainly acted on the synaptic membrane and other organelle membranes in cellular. The top 5 molecular function (MF) terms were protein domain-specific binding, DNA-binding transcription factor binding, cytokine receptor binding, protein kinase binding, and catecholamine binding (). The top 20 pathways with statistically significant differences (p < 0.05) were identified using KEGG analysis, as shown in . Among them, IL-17, HIF-1, Foxo, cAMP and NF-κB signalling pathways were most closely related to stroke treatment. The KEGG results combined with GO analysis results and screened key targets suggested that HGWD played a role against IS mainly via the HIF-1 and apoptotic signalling pathway. These findings indicated that HGWD acted against IS through multiple targets and multiple pathways.
Figure 3. GO and KEGG enrichment analyses for HGWD. (A) Biological processes that have been enriched on common targets. (B) Molecular functions that have been enriched on common targets. (C) Cellular components that have been enriched on common targets. (D) Bar chart for KEGG pathway analysis. (E) Bubble chart for KEGG pathway analysis.
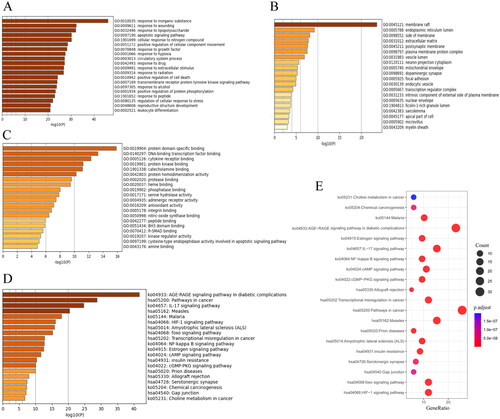
Molecular docking
We selected the key targets for molecular docking with the top 10 potentially active compounds based on the “herbs-ingredients-targets-disease” network. The key targets include MAPK1, PI3K, AKT1, c-Jun, caspase-3, IL-6, TNF-α, IL-1β, Bax, Bcl-2, HIF-1A and VEGFA. When it comes to molecular docking, the lower the affinity criteria, the stronger the binding affinity. displays the virtual docking outcomes for IL-6 (A), Bcl-2 (B), caspase-3 (C), c-Jun (D), AKT1 (E), IL-1β (F), VEGFA (G), and MAPK1 (H) with kaempferol, HIF-1A (I), TNF-α (J), and PI3K (K) with fumarine, Bax (L) with stigmasterol. The results of the heatmap in demonstrates the lowest binding energy for the docking of the key targets and active compounds. The results show that all affinity values are below −5 kcal/mol, indicating that the key targets have a relatively stable binding energy with the active compounds. PI3K, AKT1, and HIF-1A exhibit high binding affinity to all active drugs, with values ranging from −6.4 to −10 kcal/mol. Kaempferol, fumarine, and stigmasterol are observed to have lower average binding energy values to the key targets than the other compounds with values of −8.59 − 8.41, −8.25 kcal/mol. With values of −8.59, −8.41, and −8.25 kcal/mol, kaempferol, fumarine, and stigmasterol have lower average binding energies to the key targets than the other compounds.
Figure 4. Partial results of molecular docking. Kaempferol was, respectively, docked to the surface of IL-6 (PDB ID: 1ALU) (a), Bcl-2 (PDB ID: 1G5M) (B), caspase-3 (PDB ID: 3DEH) (C), c-Jun (PDB ID: 1JNM) (D), AKT1 (PDB ID: 4EJN) (E), IL-1β (PDB ID: 4GAF) (F), VEGFA (PDB ID: 1VPP) (G), MAPK1 (PDB ID: 1PME) (H); fumarine was, respectively, docked to the surface of HIF-1A (PDB ID: 1LM8) (I), TNF-α (PDB ID: 1AM8) (J), and PI3K (PDB ID: 3DBS) (K); Bax (PDB ID: 3WZE) was docked to the surface of stigmasterol (L), and the binding relationship between compounds and the proteins. A heatmap depicting the findings of molecular docking (M) and the docking score (kcal/mol) reflects the lowest binding energy of compounds-target molecule docking.
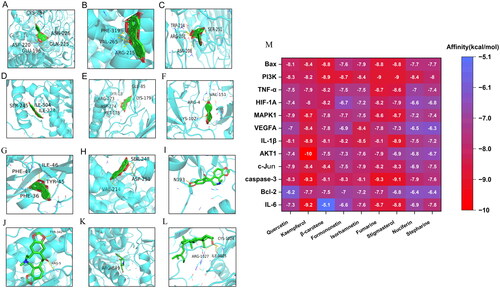
HGWD quality control
The primary components of HGWD were analyzed using HPLC. A Waters C18 column (4.6 mm, 250 μm, 5 μm) was applied for chromatographic separation. The column’s temperature was set to 30 °C. The injection volume for each sample was set to 10 μL. The detecting wavelength was 278 nm. The analytical mobile phase was made up of acetonitrile (solvent A) and water containing 0.025% formic acid (solvent B), and it had a flow rate of 1 mL/min. The running time was 76 min. The gradient elution procedure was listed in . The chromatograms of HPLC were as shown in . Five compounds of HGWD including calycosin-7-O-glucoside, calycosin, paeoniflorin, cinnamaldehyde, and 6-gingerol were identified by authentic standards. The contents of these ingredients in HGWD were 75.37, 10.02, 4561.6, 48.93, and 142.65 μg/mL, respectively.
Figure 5. Pharmacological effects of HGWD anti-IS. (A)The extracted HPLC chromatograms of HGWD. Calycosin 7-O-glucoside (a), calycosin (b), paeoniflorin (c), cinnamaldehyde (d), 6-gingerol (e), HGWD (f). (B) Neurobehavioral score. All data were shown as mean ± SD. (n = 10). (C) TTC staining. (D) The cerebral infarction rate was used to compare the changes among the five groups. (n = 3). (E) LDH level in the brain. (F) SOD activity in the brain. (G) MDA level in the brain. (H) IL-6 level in the blood. (I) IL-1β level in the blood. (J) TNF-α level in the blood. (n = 3), *p < 0.05, **p < 0.01 compared with the model group.
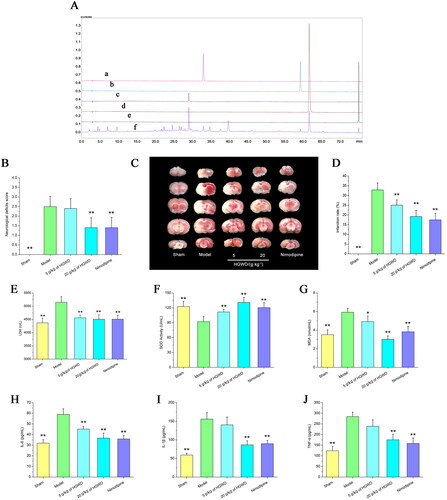
Table 3. Gradient elution procedure of HGWD.
HGWD decreased infarct volume and neurological impairments
The neurological function scores of animals in all groups were within the accepted criteria. The neurobehavioral scores of the model group were obviously greater than the 20 g/kg of HGWD and nimodipine groups (p <0.01) in . Compared with 5 g/kg of HGWD group, 20 g/kg of HGWD group was more effective in ameliorating neurological deficits. shows that the MCAO model increased cerebral infarct volume relative to the sham group (p < 0.01). When compared to the model group, the HGWD (5, 20 g/kg) and nimodipine groups reduced cerebral infarct volume in MCAO rats (p < 0.01). The findings revealed that HGWD not only had a degree of anti-cerebral infarction effect but also ameliorated neurological deficits.
HGWD alleviated cerebral ischaemia/reperfusion (I/R) induced lipid peroxidation in MCAO rats
Oxidative stress causes cerebral I/R damage (Chen et al. Citation2017). Compared to the sham-operated group, the model group had much higher levels of MDA, LDH and less SOD activity (p < 0.01, ). Nevertheless, the changes were reversed after the HGWD administration. HGWD significantly reduced MDA and LDH production while up-regulated SOD expression in the model rats. These results indicated that HGWD significantly inhibited lipid peroxidation caused by cerebral I/R injury.
HGWD reduced inflammatory cytokines in MCAO rats
According to the findings of the ELISA test, the model group’s TNF-α, IL-1β, and IL-6 levels were significantly higher than the sham-operated group’s (p < 0.01, ). However, when compared to the model group, the TNF-α, IL-1β, and IL-6 levels were considerably lower in the 20 g/kg HGWD group (p < 0.01). The findings indicated that HGWD had a certain degree of anti-inflammatory effect.
HGWD alleviated neuronal injury
As shown in , the neocortex and hippocampal neurons of the sham-operated group appeared to be healthy with abundant cytoplasm, clear nuclei, and orderly arrangement. In the model group, the ischaemic neocortex and hippocampal CA3 had necrotic areas, and the brain matrix was loose and oedematous. Moreover, a large number of neurons were missing, and the remaining neurons were pyknotic. Compared with other groups, the model group showed that the brain matrix in the hippocampus DG area seemed loose and oedematous, with an increased perivascular space. Additionally, some of the neurons were also clumped together in a disorganized manner, and parts of them were pyknotic, ruptured, and swollen. Most cytoplasm of neurons in the ischaemic neocortex and hippocampus CA3 area was shrunk with an enlarged intercellular space and deeply stained in the 5 g/kg of HGWD group. When compared to the model group, the structures of neocortical and hippocampal neurons were enhanced with well-defined cell boundaries and nuclei in the 20 g/kg of HGWD and nimodipine groups. Furthermore, there were more intact and numerous cells in the neocortex and hippocampus area in the 20 g/kg of HGWD or nimodipine-treated rats compared to the model group.
HGWD reduced Nissl body loss in neurons
Light blue cytoplasm and purple-blue nuclei were identified in the stained cells in the cortex as well as the hippocampus. shows the sham-operated group as having dark blue nucleoli of neurons with many purple-blue Nissl bodies around the nucleus. More model rats had pyknotic cells and darkly coloured Nissl bodies than the other groups in the neocortex, and many of these cells were indistinct or completely missing. Large numbers of vacuolated neurons were discovered in the hippocampus CA3 area in the 5 g/kg of HGWD group, while in the model group most had disappeared. Neurons were arranged closely and orderly, with only a few vacuolated neurons in the hippocampus CA3 area in the 20 g/kg of HGWD group and nimodipine group. Besides, the model group’s hippocampal DG neurons were dispersed compared to other groups. Furthermore, the model group had considerably fewer Nissl-positive cells in the neocortex and hippocampus than the 20 g/kg HGWD group, indicating that high dosages of HGWD decreased neuronal damage and Nissl body loss during cerebral I/R.
HGWD alleviated cell apoptosis via the PI3K/AKT signalling pathway during cerebral I/R
A few TUNEL-positive neurons were discovered in the sham-operated group, but there were more of these neurons in the operated groups’ ischaemic penumbra region (). demonstrated that TUNEL-positive neurons in the 20 g/kg of HGWD and nimodipine groups were considerably reduced relative to the model group (p < 0.05). This indicated that high dosages of HGWD alleviated cell apoptosis during cerebral I/R. ) shows that the protein levels for the PI3K/AKT signalling pathways were altered following HGWD stimulation. Comparing the model group to the sham group, we found that the expression of cleaved caspase-3 and Bax were considerably higher, whereas the expression of Bcl-2, p-PI3K and p-AKT1 were lower. Nonetheless, the outcomes were reversed with the administration of HGWD and nimodipine. Comparing the 20 g/kg of HGWD group to the model group, the expression of cleaved caspase-3 and Bax went down, while the expression of Bcl-2, p-PI3K and p-AKT1 went up. Therefore, when compared to the model group, the ratios of Bcl-2/Bax, p-PI3K/PI3K and p-AKT1/AKT1 were also increased in the 20 g/kg of HGWD group, while the ratios of Bax/β-actin and cleaved caspase-3/β-actin were decreased. The results demonstrated that HGWD reduced cell apoptosis via the PI3K/AKT signalling pathway.
Figure 8. HGWD alleviated apoptosis after cerebral I/R. (A) TUNEL staining images of five groups. (Scale bar = 50 μm, 400×). (B) HGWD reduced cell apoptosis through PI3K/AKT signalling pathway. Western blotting was utilized to examine the levels of Bcl-2, Bax, caspase-3, cleaved caspase-3, AKT1, p-AKT1, PI3K, and p-PI3K. (C) Percentages of TUNEL positive cells were utilized to evaluate the differences in the five groups. Integrated density data were quantified (D-J). the results (D-H), respectively, showed the levels of Bax, Bcl-2, the ratio of Bcl-2/Bax, caspase-3, and cleaved caspase-3 in the brains of rats for five groups, with β-actin as the internal control. The results of I and J, respectively, showed the ratios of p-AKT1/AKT1 and p-PI3K/PI3K in the brains of rats for five groups. All data were shown as mean ± SD. (n = 3, *p < 0.05, **p < 0.01 compared with the model group).
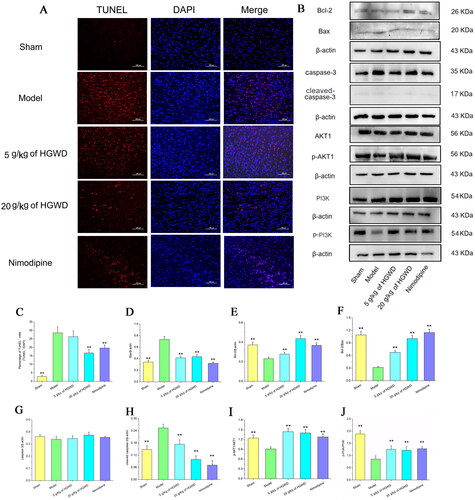
HGWD alleviated cerebral I/R damage via the HIF-1 signalling pathway
After cerebral infarction occlusion, the HIF-1 signalling pathway was tightly associated with disease progress. As shown in , when compared to the sham group, the expression of HIF-1A, VEGFA, p-MAPK1, and p-c-Jun were higher in the model group. However, comparing the 20 g/kg of HGWD and nimodipine groups to the model group, the expression of HIF-1A, VEGFA, p-MAPK1, and p-c-Jun went down. These results indicated that HGWD alleviated damage to cerebral I/R to MCAO rats via the HIF-1A-mediated MAPK signalling pathways.
Figure 9. HGWD reduced neuronal damage in MCAO rats through HIF-1 signalling pathway. (A) Western blotting was utilized to examine the levels of HIF-1A, VEGFA, MAPK1, p-MAPK1, c-Jun, and p-c-Jun. Integrated density data were quantified (B-H). the results (B-F), respectively, showed the expression of HIF-1A, VEGFA, the ratios of p-MAPK1/MAPK1, and p-c-Jun/c-Jun in the brains of rats for five groups. All data were shown as mean ± SD. (n = 3, *p < 0.05, **p < 0.01 compared with the model group).
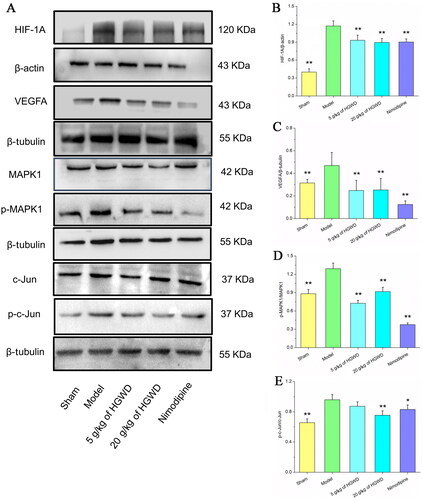
Discussion
Rather than focussing on a single chemical component, network pharmacology examines the intricate linkages in biological systems as a whole. It offers substantial advantages in terms of identifying alternate targets for TCM, developing multi-targeted medications, and bringing unique insights into TCM research (Zhang et al. Citation2019). In this research, the prospective targets and material foundation of HGWD for treating IS were analyzed utilizing network pharmacology. Most of the screened compounds have good biological activities. For example, stigmasterol, a natural steroid that exists in Zingiber officinale. and Ziziphus jujuba, has a potent immunomodulatory action. It was found that stigmasterol reduced the innate immune response and protected against organ damage caused by LPS in mouse models (Antwi et al. Citation2017). Paeoniflorin, the principal active compound in Paeonia lactiflora, possesses anti-inflammatory and immune-regulating properties. Paeoniflorin inhibited cell apoptosis and reduced inflammation via the MAPK/NF-κB pathway in an animal model of ulcerative colitis (Gu et al. Citation2017). Quercetin, a natural flavonoid screened from Astragalus membranaceus and Ziziphus jujuba, exhibits anti-coagulant, anti-inflammatory, and free radical scavenging capabilities (Ahmad et al. Citation2011; Zhang et al. Citation2011). We screened six neuroprotective components of HGWD by cell membrane solid-phase chromatography coupled with HPLC-MS/MS, including calycosin-7-O-glucoside, calycosin, paeoniflorin, 4-hydroxycinnamic acid, wogonin, and formononetin (He et al. Citation2021). The results were consistent with the high-ranking scores of the network pharmacology-filtered components, indicating that network pharmacology was a useful tool for the analysis and screening of chemical components in TCM. However, the current study also had some limitations. Network pharmacology inevitably had a certain computer algorithm, and it might miss some critical components and pathways. Therefore, we need to combine more literature mining and experimental evidence to discover more potential active ingredients in the future.
GO and KEGG were then used to greatly enrich the PPI network. After an in-depth analysis of GO and KEGG, we found that the mechanism of HGWD’s anti-IS effect was directly connected to inflammation, apoptosis and hypoxia. Besides, hypoxia-related proteins (HIF-1A, MAPK1, and c-Jun, AKT1), inflammation-related proteins (IL-6, IL-1β, TNF-α), and apoptotic-related proteins (caspase-3, Bax, Bcl-2) had high degree values in the PPI network, indicating that they were likely to be the core targets for HGWD treatment of IS. And these proteins can be regulated by the HIF-1 signalling pathway, as shown in . In summary, the findings of network pharmacology show the direction of the material basis and mechanism of action of HGWD against stroke. We assumed that HGWD exerted its anti-IS effect through the HIF-1 signalling pathway. This assumption was also verified by the following molecular-docking tests and in vivo experiments.
Figure 10. PI3K-AKT and HIF-1 signalling pathway analysis of Differential expression genes by the KEGG database. The fonts marked in red represent targets in the PI3K-AKT (A) and HIF-1 (B) signalling pathways closely related to the effect of HGWD anti-IS.
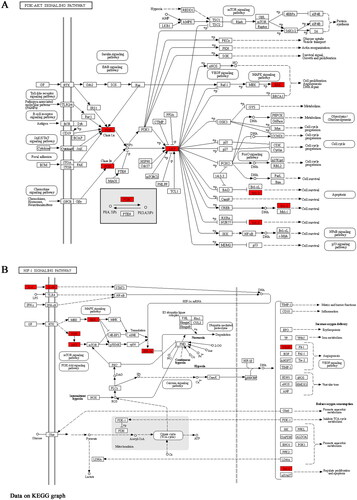
The establishment of a “drug-target-pathway” visual network is useful for further exploring the mechanism of TCM formulations. As shown in , a number of targets and important signalling pathways were discovered to be related to a variety of compounds from different herbs in HGWD. The results showed that these active compounds might have synergistic effects in the treatment of IS. Inflammation, oxidative stress, hypoxia, and apoptosis are the primary causes of IS damage (Soares et al. Citation2019). According to the comprehensive analysis of the network pharmacology findings, the HIF-1 signalling pathway was more significant than most others. Network pharmacology combined with molecular docking experiments for further in-depth verification, it is of great significance to find that the key drug targets for the treatment of stroke. Furthermore, the molecular-docking tests showed that the active compounds in HGWD had a considerable binding affinity for HIF-1 signalling pathway targets (affinity < −5), suggesting they were the primary active ingredients (). Therefore, we conjectured HGWD played a therapeutic role in IS through the HIF-1 signalling pathway. In light of this conjecture, we decided to conduct in vivo experiments to verify its validity.
Microglia are rapidly activated and transformed into phagocytic cells during IS, releasing a range of cytotoxic chemicals and resulting in inflammation and brain tissue necrosis (Denes et al. Citation2007; Santos Samary et al. Citation2016). The results demonstrated that HGWD ameliorated neurological deficits scores, infarct volume, Nissl body loss, inflammatory response as well as apoptosis (), indicating the neuroprotective properties of HGWD on ischaemic stroke. Cerebral I/R may cause brain injury from an oxygen free radical imbalance. The amounts of LDH and MDA during cerebral I/R can reflect ischaemic severity and lipid peroxidation. As a potent free radical scavenger, SOD can help to minimize oxidative stress (Liu et al. Citation2020). In addition, previous studies showed that oxidative stress was strongly associated with the clinical prognosis of IS (Allen and Bayraktutan Citation2009; Nanetti et al. Citation2011). The findings in this study revealed that HGWD decreased cerebral I/R-induced LDH and MDA generation while increasing SOD activity, indicating that HGWD protected against cerebral I/R damage via antioxidative stress ().
PI3K/AKT signalling is a crucial pathway regulating neuroinflammation, oxidant stress, and apoptosis in neurons (Lv et al. Citation2017; Chen et al. Citation2020). It is noteworthy that Bcl-2, p-PI3Kand p-AKT1 were significantly upregulated in the HGWD groups while Bax and cleaved caspase-3 were down-regulated in this study, indicating that neuronal cell apoptosis mediated by the PI3K/AKT signalling pathway (). Moreover, the ratio of Bcl-2/Bax is an indicator of apoptotic susceptibility (Zhang et al. Citation2019). HGWD was observed to increase the ratio of Bcl-2/Bax in this study.
The HIF signalling pathway is critical for hypoxia sensing and adaptation in the brain (Ziello et al. Citation2007). HIF-1 is activated and stabilized in neurons by high levels of ROS in mitochondria in a state of cerebral hypoxia, and thus plays a role in stimulating angiogenesis and neuroprotection, while accumulation in endothelial cells may induce blood brain barrier (BBB) damage (Qutub and Popel Citation2008; Liao et al. Citation2009; Engelhardt et al. Citation2015). Previous have revealed that HIF-1 can participate in the apoptotic cascade by activating pro-apoptotic molecules that cause mitochondrial dysfunction and neuronal cell death (Shi et al. Citation2009). Recent studies have found that mitochondrial autophagy activation can be mediated through the Hif1α/BNIP3 pathway. Autophagy regulates PGC-1α, SOD signalling pathway and attenuates ischaemia and hypoxia-induced oxidative stress (Xie et al. Citation2022). In addition, inflammation is an inevitable event after stroke, and inflammation is one of the mechanisms leading to BBB leakage. During the acute stroke, activation of HIF-1α increases the expression of downstream target VEGF and triggers BBB leakage (Chen et al. Citation2019). In addition, the accumulated ROS further activate the intracellular MAPK signalling pathway (Sarkar et al. Citation2019). Therefore, decreasing cell death, suppressing inflammation, and reducing BBB leakage become good strategies for stroke treatment. We found that the neuroprotective impact of HGWD was associated with the HIF-1 signalling pathway by downregulating the levels of HIF-1 and VEGFA as well as decreasing the ratios of p-MAPK1/MAPK1 and p-c-Jun/c-Jun in this study (). The outcomes of in vivo experiments were compatible with the findings of network pharmacology, confirming the reliability of network pharmacological prediction and the mechanism of HGWD in treating IS.
Conclusions
Network pharmacology, molecular docking, and in vivo experiments were first applied to investigate the HGWD mechanism for treating IS in this study. Simultaneously, we confirmed that HGWD inhibited inflammation, restrained oxidative stress, reduced cell apoptosis, and activated the PI3K/AKT and HIF-1 signalling pathway, thereby exerting a cerebral neuroprotective effect in MCAO rats.
Authors’ contributions
WG Liao, LS Wang, and MC Wang coordinated and directed the project. WG Liao, Y Wu, Z Xie and MY Gong performed the network pharmacology and molecular docking. YX Li, JY Du, AY Su, and LY Zhong carried out the in vivo experiments; JH Liang, Z Liu and PC Wang collated data. All authors were involved in the writing of the article. All authors reviewed and approved the final version of the manuscript.
Disclosure statement
No potential conflict of interest was reported by the author(s).
Additional information
Funding
Notes on contributors
Weiguo Liao
WG Liao is a Ph.D. student at Guangzhou University of Chinese Medicine, mainly engaged in scientific research in pharmacology and pharmacy. He has published 3 SCI articles as the first author and 1 SCI article as the corresponding author, involving the development of stroke and cancer drugs.
Minchun Wang
MC Wang, Y Wu, JY Du, YX Li, AY Su, PC Wang, JH Liang, and LY Zhong are current graduate students of Guangzhou University of Chinese Medicine, focussing on pharmacology and modern molecular biology.
Ying Wu
MC Wang, Y Wu, JY Du, YX Li, AY Su, PC Wang, JH Liang, and LY Zhong are current graduate students of Guangzhou University of Chinese Medicine, focussing on pharmacology and modern molecular biology.
Jinyan Du
MC Wang, Y Wu, JY Du, YX Li, AY Su, PC Wang, JH Liang, and LY Zhong are current graduate students of Guangzhou University of Chinese Medicine, focussing on pharmacology and modern molecular biology.
Yaxin Li
MC Wang, Y Wu, JY Du, YX Li, AY Su, PC Wang, JH Liang, and LY Zhong are current graduate students of Guangzhou University of Chinese Medicine, focussing on pharmacology and modern molecular biology.
Anyu Su
MC Wang, Y Wu, JY Du, YX Li, AY Su, PC Wang, JH Liang, and LY Zhong are current graduate students of Guangzhou University of Chinese Medicine, focussing on pharmacology and modern molecular biology.
Lanying Zhong
MC Wang, Y Wu, JY Du, YX Li, AY Su, PC Wang, JH Liang, and LY Zhong are current graduate students of Guangzhou University of Chinese Medicine, focussing on pharmacology and modern molecular biology.
Zi Xie
Z Xie and MY Gong are undergraduate students of Guangzhou University of Chinese Medicine majoring in TCM.
Mingyu Gong
Z Xie and MY Gong are undergraduate students of Guangzhou University of Chinese Medicine majoring in TCM.
Junhui Liang
MC Wang, Y Wu, JY Du, YX Li, AY Su, PC Wang, JH Liang, and LY Zhong are current graduate students of Guangzhou University of Chinese Medicine, focussing on pharmacology and modern molecular biology.
Pengcheng Wang
MC Wang, Y Wu, JY Du, YX Li, AY Su, PC Wang, JH Liang, and LY Zhong are current graduate students of Guangzhou University of Chinese Medicine, focussing on pharmacology and modern molecular biology.
Zai Liu
Z Liu is a chief pharmacist at Dongguan Hospital of Traditional Chinese Medicine, with deep academic attainment in the field of TCM.
Lisheng Wang
LS Wang is a professor at Guangzhou University of Chinese Medicine, mainly engaged in pharmacy and pharmacology research, and has achieved fruitful results in TCM against stroke. He has published more than 10 SCI papers as the corresponding author.
References
- Ahmad A, Khan MM, Hoda MN, Raza SS, Khan MB, Javed H, Ishrat T, Ashafaq M, Ahmad ME, Safhi MM, et al. 2011. Quercetin protects against oxidative stress associated damages in a rat model of transient focal cerebral ischemia and reperfusion. Neurochem Res. 36(8):1360–1371. doi: 10.1007/s11064-011-0458-6.
- Allen CL, Bayraktutan U. 2009. Oxidative stress and its role in the pathogenesis of ischaemic stroke. Int J Stroke. 4(6):461–470. doi: 10.1111/j.1747-4949.2009.00387.x.
- Amalia L, Sadeli HA, Parwati I, Rizal A, Panigoro R. 2020. Hypoxia-inducible factor-1alpha in acute ischemic stroke: neuroprotection for better clinical outcome. Heliyon. 6(6):e04286. doi: 10.1016/j.heliyon.2020.e04286.
- Amberger JS, Bocchini CA, Schiettecatte F, Scott AF, Hamosh A. 2015. OMIM.org: online Mendelian inheritance in man (OMIM(R)), an online catalog of human genes and genetic disorders. Nucleic Acids Res. 43(Database issue):D789–798. doi: 10.1093/nar/gku1205.
- Antwi AO, Obiri DD, Osafo N, Forkuo AD, Essel LB. 2017. Stigmasterol inhibits lipopolysaccharide-induced innate immune responses in murine models. Int Immunopharmacol. 53:105–113. doi: 10.1016/j.intimp.2017.10.018.
- Baranova O, Miranda LF, Pichiule P, Dragatsis I, Johnson RS, Chavez JC. 2007. Neuron-specific inactivation of the hypoxia inducible factor 1 alpha increases brain injury in a mouse model of transient focal cerebral ischemia. J Neurosci. 27(23):6320–6332. doi: 10.1523/JNEUROSCI.0449-07.2007.
- Benjamin EJ, Muntner P, Alonso A, Bittencourt MS, Callaway CW, Carson AP, Chamberlain AM, Chang AR, Cheng S, Das SR, et al. 2019. Heart disease and stroke statistics-2019 update: a report from the American heart association. Circulation. 139(10):e56–e528. doi: 10.1161/CIR.0000000000000659.
- Boezio B, Audouze K, Ducrot P, Taboureau O. 2017. Network-based approaches in pharmacology. Mol Inf. 36(10):1700048. doi: 10.1002/minf.201700048.
- Chen MC, Hsu WL, Hwang PA, Chou TC. 2015. Low molecular weight fucoidan inhibits tumor angiogenesis through downregulation of HIF-1/VEGF signaling under hypoxia. Mar Drugs. 13(7):4436–4451. doi: 10.3390/md13074436.
- Chen S, Peng J, Sherchan P, Ma Y, Xiang S, Yan F, Zhao H, Jiang Y, Wang N, Zhang JH, et al. 2020. TREM2 activation attenuates neuroinflammation and neuronal apoptosis via PI3K/Akt pathway after intracerebral hemorrhage in mice. J Neuroinflammation. 17(1):168. doi: 10.1186/s12974-020-01853-x.
- Chen Y, Li Y, Xu H, Li G, Ma Y, Pang YJ. 2017. Morin mitigates oxidative stress, apoptosis and inflammation in cerebral ischemic rats. Afr J Tradit Complement Altern Med. 14(2):348–355. doi: 10.21010/ajtcam.v14i2.36.
- Chen ZZ, Gong X, Guo Q, Zhao H, Wang L. 2019. Bu Yang Huan Wu Decoction prevents reperfusion injury following ischemic stroke in rats via inhibition of HIF-1 alpha, VEGF and promotion beta-ENaC expression. J Ethnopharmacol. 228:70–81. doi: 10.1016/j.jep.2018.09.017.
- Daina A, Michielin O, Zoete V. 2019. SwissTargetPrediction: updated data and new features for efficient prediction of protein targets of small molecules. Nucleic Acids Res. 47(W1):W357–W364. doi: 10.1093/nar/gkz382.
- Denes A, Vidyasagar R, Feng J, Narvainen J, McColl BW, Kauppinen RA, Allan SM. 2007. Proliferating resident microglia after focal cerebral ischaemia in mice. J Cereb Blood Flow Metab. 27(12):1941–1953. doi: 10.1038/sj.jcbfm.9600495.
- Engelhardt S, Huang SF, Patkar S, Gassmann M, Ogunshola OO. 2015. Differential responses of blood-brain barrier associated cells to hypoxia and ischemia: a comparative study. Fluids Barriers CNS. 12:4. doi: 10.1186/2045-8118-12-4.
- George PM, Steinberg GK. 2015. Novel stroke therapeutics: unraveling stroke pathophysiology and its impact on clinical treatments. Neuron. 87(2):297–309. doi: 10.1016/j.neuron.2015.05.041.
- Gu P, Zhu L, Liu Y, Zhang L, Liu J, Shen H. 2017. Protective effects of paeoniflorin on TNBS-induced ulcerative colitis through inhibiting NF-kappaB pathway and apoptosis in mice. Int Immunopharmacol. 50:152–160. doi: 10.1016/j.intimp.2017.06.022.
- He Y, Zheng H, Zhong L, Zhong N, Wen G, Wang L, Zhang Y. 2021. Identification of active ingredients of Huangqi Guizhi Wuwu decoction for promoting nerve function recovery after ischemic stroke using HT22 live-cell-based affinity chromatography combined with HPLC-MS/MS. Drug Des Devel Ther. 15:5165–5178. doi: 10.2147/DDDT.S333418.
- Jiang W, Zhang F, Zao B. 2017. Effect of Huangqi Guizhi Wuwu decoction on patients with convalescent cerebral infarction. World J Tradit Chin Med. 12:1555–1559.
- Ke Q, Costa M. 2006. Hypoxia-inducible factor-1 (HIF-1). Mol Pharmacol. 70(5):1469–1480. doi: 10.1124/mol.106.027029.
- Kibble M, Saarinen N, Tang J, Wennerberg K, Makela S, Aittokallio T. 2015. Network pharmacology applications to map the unexplored target space and therapeutic potential of natural products. Nat Prod Rep. 32(8):1249–1266. doi: 10.1039/c5np00005j.
- Kim S, Thiessen PA, Bolton EE, Chen J, Fu G, Gindulyte A, Han L, He J, He S, Shoemaker BA, et al. 2016. PubChem substance and compound databases. Nucleic Acids Res. 44(D1):D1202–1213. doi: 10.1093/nar/gkv951.
- Li H, Zhao L, Zhang B, Jiang Y, Wang X, Guo Y, Liu H, Li S, Tong X. 2014. A network pharmacology approach to determine active compounds and action mechanisms of ge-gen-qin-lian decoction for treatment of type 2 diabetes. Evid Based Complement Alternat Med. 2014:495840. doi: 10.1155/2014/495840.
- Liao SJ, Lin JW, Pei Z, Liu CL, Zeng JS, Huang RX. 2009. Enhanced angiogenesis with dl-3N-butylphthalide treatment after focal cerebral ischemia in RHRSP. Brain Res. 1289:69–78. doi: 10.1016/j.brainres.2009.06.018.
- Liao W, Yu J, Guo Z, Ba W, Wang D, Li Z, Fan W, Liao F, Wu Y, Wang L. 2016. Microdialysis combined with UPLC-MS/MS method for determination of tetramethylpyrazine and ferulic acid in striatum of awake and anesthetic rats subjected to cerebral ischemia. J Pharm Biomed Anal. 128:510–518. doi: 10.1016/j.jpba.2016.06.030.
- Liu D, Wang H, Zhang Y, Zhang Z. 2020. Protective effects of chlorogenic acid on cerebral ischemia/reperfusion injury rats by regulating oxidative stress-related Nrf2 pathway. Drug Des Devel Ther. 14:51–60. doi: 10.2147/DDDT.S228751.
- Liu S, Levine SR, Winn HR. 2010. Targeting ischemic penumbra: part I - from pathophysiology to therapeutic strategy. J Exp Stroke Transl Med. 3(1):47–55. doi: 10.6030/1939-067x-3.1.47.
- Longa EZ, Weinstein PR, Carlson S, Cummins R. 1989. Reversible middle cerebral artery occlusion without craniectomy in rats. Stroke. 20(1):84–91. doi: 10.1161/01.str.20.1.84.
- Lv MR, Li B, Wang MG, Meng FG, Yu JJ, Guo F, Li Y. 2017. Activation of the PI3K-Akt pathway promotes neuroprotection of the delta-opioid receptor agonist against cerebral ischemia-reperfusion injury in rat models. Biomed Pharmacother. 93:230–237. doi: 10.1016/j.biopha.2017.05.121.
- Ma Y, Wang H, Yang P. 2016. Treatment of 80 cases of shoulder-hand syndrome after stroke with Huangqi Guizhi Wuwu Decoction combined with rehabilitation training. Chin Med Mod Distance Educ China. 14:76–80.
- Nanetti L, Raffaelli F, Vignini A, Perozzi C, Silvestrini M, Bartolini M, Provinciali L, Mazzanti L. 2011. Oxidative stress in ischaemic stroke. Eur J Clin Invest. 41(12):1318–1322. doi: 10.1111/j.1365-2362.2011.02546.x.
- Prabhakaran S, Ruff I, Bernstein RA. 2015. Acute stroke intervention: a systematic review. JAMA. 313(14):1451–1462. doi: 10.1001/jama.2015.3058.
- Prlic A, Bliven S, Rose PW, Bluhm WF, Bizon C, Godzik A, Bourne PE. 2010. Pre-calculated protein structure alignments at the RCSB PDB website. Bioinformatics. 26(23):2983–2985. doi: 10.1093/bioinformatics/btq572.
- Qutub AA, Popel AS. 2008. Reactive oxygen species regulate hypoxia-inducible factor 1alpha differentially in cancer and ischemia. Mol Cell Biol. 28(16):5106–5119. doi: 10.1128/MCB.00060-08.
- Ru J, Li P, Wang J, Zhou W, Li B, Huang C, Li P, Guo Z, Tao W, Yang Y, et al. 2014. TCMSP: a database of systems pharmacology for drug discovery from herbal medicines. J Cheminform. 6:13. doi: 10.1186/1758-2946-6-13.
- Safran M, Dalah I, Alexander J, Rosen N, Iny Stein T, Shmoish M, Nativ N, Bahir I, Doniger T, Krug H, et al. 2010. GeneCards Version 3: the human gene integrator. Database. 2010:baq020. doi: 10.1093/database/baq020.
- Santos Samary C, Pelosi P, Leme Silva P, Rieken Macedo Rocco P. 2016. Immunomodulation after ischemic stroke: potential mechanisms and implications for therapy. Crit Care. 20(1):391. doi: 10.1186/s13054-016-1573-1.
- Sarkar S, Chakraborty D, Bhowmik A, Ghosh MK. 2019. Cerebral ischemic stroke: cellular fate and therapeutic opportunities. Front Biosci. 24(3):435–450. doi: 10.2741/4727.
- Shen Y, Zhang B, Pang X, Yang R, Chen M, Zhao J, Wang J, Wang Z, Yu Z, Wang Y, et al. 2020. Network pharmacology-based analysis of Xiao-Xu-Ming decoction on the treatment of Alzheimer’s disease. Front Pharmacol. 11:595254. doi: 10.3389/fphar.2020.595254.
- Shi Q, Zhang P, Zhang J, Chen X, Lu H, Tian Y, Parker TL, Liu Y. 2009. Adenovirus-mediated brain-derived neurotrophic factor expression regulated by hypoxia response element protects brain from injury of transient middle cerebral artery occlusion in mice. Neurosci Lett. 465(3):220–225. doi: 10.1016/j.neulet.2009.08.049.
- Soares ROS, Losada DM, Jordani MC, Evora P, Castro ESO. 2019. Ischemia/reperfusion injury revisited: an overview of the latest pharmacological strategies. IJMS. 20(20):5034. doi: 10.3390/ijms20205034.
- Szklarczyk D, Morris JH, Cook H, Kuhn M, Wyder S, Simonovic M, Santos A, Doncheva NT, Roth A, Bork P, et al. 2017. The STRING database in 2017: quality-controlled protein-protein association networks, made broadly accessible. Nucleic Acids Res. 45(D1):D362–D368. doi: 10.1093/nar/gkw937.
- UniProt C. 2021. UniProt: the universal protein knowledgebase in 2021. Nucleic Acids Res. 49(D1):D480–D489.
- Wang L, Wang L, Wang H, Zhu T. 2022. Investigation into the potential mechanism and molecular targets of Fufang Xueshuantong capsule for the treatment of ischemic stroke based on network pharmacology and molecular docking. Front Pharmacol. 13:949644. doi: 10.3389/fphar.2022.949644.
- Wang Y, Thilmony R, Gu YQ. 2014. NetVenn: an integrated network analysis web platform for gene lists. Nucleic Acids Res. 42(Web Server issue):W161–166. doi: 10.1093/nar/gku331.
- Wu S, Wu B, Liu M, Chen Z, Wang W, Anderson CS, Sandercock P, Wang Y, Huang Y, Cui L, et al. 2019. Stroke in China: advances and challenges in epidemiology, prevention, and management. Lancet Neurol. 18(4):394–405. doi: 10.1016/S1474-4422(18)30500-3.
- Xie W, Zhu T, Zhang S, Sun X. 2022. Protective effects of Gypenoside XVII against cerebral ischemia/reperfusion injury via SIRT1-FOXO3A- and Hif1a-BNIP3-mediated mitochondrial autophagy. J Transl Med. 20(1):622. doi: 10.1186/s12967-022-03830-9.
- Xu J, Guan Z, Wang X, Sun D, Li Y, Pei B, Lu Y, Yuan L, Zhang X. 2021. Network pharmacology and experimental evidence identify the mechanism of astragaloside IV in oxaliplatin neurotoxicity. Drug Des Devel Ther. 15:99–110. doi: 10.2147/DDDT.S262818.
- Xu W, Zheng J, Gao L, Li T, Zhang J, Shao A. 2017. Neuroprotective effects of stem cells in ischemic stroke. Stem Cells Int. 2017:4653936. doi: 10.1155/2017/4653936.
- Yang T, Chen X, Mei Z, Liu X, Feng Z, Liao J, Deng Y, Ge J. 2021. An integrated analysis of network pharmacology and experimental validation to reveal the mechanism of Chinese medicine formula Naotaifang in treating cerebral ischemia-reperfusion injury. Drug Des Devel Ther. 15:3783–3808. doi: 10.2147/DDDT.S328837.
- Yin Y, Ren H. 2017. Treatment of 46 cases of sequelae of cerebral infarction with Huangqi Guizhi Wuwu decoction. Inner Mongolia Tradit Chin Med. 36:11–14.
- Zhang H, Dong R, Zhang P, Wang Y. 2019. Songorine suppresses cell growth and metastasis in epithelial ovarian cancer via the Bcl2/Bax and GSK3beta/betacatenin signaling pathways. Oncol Rep. 41(5):3069–3079. doi: 10.3892/or.2019.7070.
- Zhang R, Zhu X, Bai H, Ning K. 2019. Network pharmacology databases for traditional Chinese medicine: review and assessment. Front Pharmacol. 10:123. doi: 10.3389/fphar.2019.00123.
- Zhang Y, Bai M, Zhang B, Liu C, Guo Q, Sun Y, Wang D, Wang C, Jiang Y, Lin N, et al. 2015. Uncovering pharmacological mechanisms of Wu-tou decoction acting on rheumatoid arthritis through systems approaches: drug-target prediction, network analysis and experimental validation. Sci Rep. 5:9463. doi: 10.1038/srep09463.
- Zhang ZJ, Cheang LC, Wang MW, Lee SM. 2011. Quercetin exerts a neuroprotective effect through inhibition of the iNOS/NO system and pro-inflammation gene expression in PC12 cells and in zebrafish. Int J Mol Med. 27(2):195–203. doi: 10.3892/ijmm.2010.571.
- Zheng HZ, Shen X, He YY, Yan XL, Wang SX, Yu AM, Wang LS. 2020. Pharmacokinetic analysis of Huangqi Guizhi Wuwu decoction on blood and brain tissue in rats with normal and cerebral ischemia-reperfusion injury by microdialysis with HPLC-MS/MS. Drug Des Devel Ther. 14:2877–2888. doi: 10.2147/DDDT.S257020.
- Zhou Y, Zhou B, Pache L, Chang M, Khodabakhshi AH, Tanaseichuk O, Benner C, Chanda SK. 2019. Metascape provides a biologist-oriented resource for the analysis of systems-level datasets. Nat Commun. 10(1):1523. doi: 10.1038/s41467-019-09234-6.
- Ziello JE, Jovin IS, Huang Y. 2007. Hypoxia-Inducible Factor (HIF)-1 regulatory pathway and its potential for therapeutic intervention in malignancy and ischemia. Yale J Biol Med. 80(2):51–60.