Abstract
Context
Qi Teng Xiao Zhuo granule (QTXZG) is a traditional Chinese medicine (TCM) used for therapeutic effects on chronic glomerulonephritis (CGN). However, the underlying mechanism remains unclear.
Objective
To investigate the molecular mechanism of QTXZG on CGN by proteomics.
Materials and methods
The CGN model was induced in Sprague–Dawley rats by injecting adriamycin (3.5 mg/kg, Day 1; 3.0 mg/kg, Day 14) twice through the tail vein. Urine samples were collected on the 21st day; and the rats divided randomly into control, adriamycin, QTXZG administration groups. Rats in the QTXZG group received QTXZG (10.8 g/kg); control and adriamycin groups were given physiological saline once per day for 30 days. Proteomics was applied to identify the candidate proteins combined with autophagy database and verified by immunofluorescence (IF) and western blots (WB).
Results
278 differentially expressed proteins (DEPs) were identified based on proteomics and Rab7 was screened as an autophagy protein biomarker. In vitro cell experiments, we found that QTXZG (20%, IC50 = 23.47%) could decrease the expression of NLRP3, Caspase-1, IL-18, IL-1β, while increasing the expression of Pink1, Parkin, Rab7, Podocalyxin. The cell apoptosis rate increased from 6.68 ± 0.07 to 11.03 ± 0.36%. Overexpression of Rab7 resulted in an increase in autophagy relevant protein expression.
Discussion and conclusion
TCM CGN-regulating herbs (QTXZG) can exert therapeutic effects by affecting the Rab7/Pink1/Parkin pathway to promote mitochondrial autophagy. New breakthroughs in targeted Rab7 may eventually enable such applications.
Keywords:
Introduction
Globally, approximately 10% of adults have chronic kidney disease (CKD) (Lopez-Novoa et al. Citation2010). Chronic glomerulonephritis (CGN) is the most common cause of end-stage kidney disease and is the most common component of CKD. CGN is associated with immune-mediated inflammatory diseases, the main clinical symptoms are edema, hematuria, albuminuria, and hypertension (Gao et al. Citation2023). However, the molecular mechanism of its pathogenesis is unclear (Blom et al. Citation2017). Current clinical CGN treatment generally relies on Western drugs, which show insufficient efficacy, are expensive, and can lead to numerous side effects (An et al. Citation2014; Wei et al. Citation2022). Traditional Chinese medicine (TCM) has a history of thousands of years, and it is an alternative therapy for many diseases, including CKD (Dai et al. Citation2022). Multiple active phytochemical components may target multiple molecules/pathways simultaneously in TCM formulas, thus potentially having a better effect than individual compounds. This phenomenon gives TCM unique advantages in CGN prevention and treatment. In recent years, effective TCM compounds have been advanced for improving kidney function, reducing kidney injury, and preventing kidney fibrosis (Wei et al. Citation2020).
Qi Teng Xiao Zhuo granule (QTXZG) is an empirical prescription for the treatment of CGN created by a famous Chinese medicine practitioner (Enze Cao) of the First Affiliated Hospital of Anhui University of Chinese Medicine based on the theory of Xin’an medicine about ‘strengthening the spleen, replenishing water, and removing blood stasis’. Xin’an medicine is traditional Chinese medicine that has been handed down since the Song dynasty. In the process of clinical application, QTXZG has been proven by clinical practice and has a good curative effect on CGN (Liu et al. Citation2007; Hu et al. Citation2008).
In 2007, QTXZG obtained the approval number of the Anhui Provincial Medical System (Anhui province Food and Drug Administration, Z20070001); this formula consists of seven herbs: Astragalus membranaceus (Fisch.) Bunge [(Leguminosae), Huangqi in Chinese], Leonurus japonicus Houtt. [(Labiatae), Yimucao in Chinese], Coix lacryma-jobi L. [(Gramineae), Yiyiren in Chinese], Hedyotis diffusa Willd. [(Rubiaceae), Baihuasheshecao in Chinese], Imperata cylindrica Beauv. [(Gramineae), Baimaogen in Chinese], Tripterygium wilfordii Hook. f. [(Celastraceae), Leigongteng in Chinese], Cryptotympana pustulata Fabricius [(Cicadidae), Chantui in Chinese] (Chan et al. Citation2012; Li et al. Citation2016). These seven herbs have been used in the treatment of CGN in many countries.
QTXZG showed high efficacy and few side effects in CGN treatment, with a preliminary study by our group showing its efficacy in a doxorubicin-induced CGN rat model (Gao et al. Citation2016). Clinical studies have shown that QTXZG could significantly reduce urinary protein content and increase plasma albumin in patients with CGN (Liu et al. Citation2007; Hu et al. Citation2008). In pharmaceutical preparations, our previous studies examined the optimal extraction process of QTXZG by orthogonal experiments and employed different granule preparation methods to compare and screen the preparation processes of QTXZG (Gao and Zhou Citation2004; Wang et al. Citation2019). In addition, quality control research was carried out, the components in QTXZG were analyzed by thin-layer chromatography and high-performance liquid chromatography, and effective quality control data were obtained, which provided a theoretical basis for the production of QTXZG (Wei et al. Citation2010; Ge et al. Citation2017). Pharmacodynamically, we found that QTXZG could reduce blood urea nitrogen, Cr, interleukin-1β (IL-1β), interleukin-6 (IL-6), and tumor necrosis factor-α, increase interleukin-10 (IL-10), and alleviate the pathological changes of CGN, which indicated that QTXZG has a certain therapeutic effect on CGN (Wei et al. Citation2016). We also used network pharmacology to explore the effective components, potential targets, and pathways of QTXZG for the treatment of CGN (Liu et al. Citation2021). A key characteristic of QTXZG is its multicompound, multipathway, and multitarget composition, which makes it difficult to understand its mechanism. We considered applying proteomics technology to explore its molecular mechanism for the treatment of CGN based on our previous study.
Proteomics, a powerful tool for monitoring protein expression changes, can be used to identify altered proteins that may be potential drug development targets (Vasilogianni et al. Citation2022). A comprehensive analysis of protein changes is also helpful in understanding the mechanism of drug action. Proteomics has been successfully used to discover many active ingredients and their mechanisms of action in TCM (Suo et al. Citation2016).
Autophagy, a lysosomal degradation pathway that can remove and reuse damaged organelles and biomolecules, is an important regulatory mechanism for maintaining intracellular homeostasis (Galluzzi and Green Citation2019; Nakatogawa Citation2020; Rubio-Tomás et al. Citation2023). Growing evidence indicates that autophagic dysfunction is associated with the pathogenesis of kidney diseases, including acute kidney injury, diabetic nephropathy, and glomerulosclerosis (Choi Citation2020; Minami et al. Citation2023). Autophagy includes macroautophagy, microautophagy, and chaperone-mediated autophagy, and mitophagy is macroautophagy that involves selective autophagic processes. As selective autophagy, mitophagy can selectively identify mitochondria and protect the kidney by removing abnormal mitochondrial aggregates (Dagar et al. Citation2022).
In this study, we conducted a proteomics analysis to identify proteins differentially expressed in CGN. Rab7, an autophagy-related protein, was chosen as a biomarker. We then investigated the role of QTXZG in regulating Rab7/Pink1/Parkin signaling pathway-mediated mitochondrial autophagy in the treatment of CGN. The study goals were to determine the anti-CGN properties of QTXZG, clarify its mechanism of action, and inspire novel research directions for developing TCM-based CGN treatments.
Materials and methods
Experimental animals
Male specific-pathogen free (SPF) Sprague–Dawley (SD) rats (weight, 200 ± 20 g; 7-weeks-old) were purchased from the Zhengzhou Huiji Huaxing experimental animal farm (SCXK(LU) 20190002). QTXZG was purchased from the First Affiliated Hospital of the Anhui University of Chinese Medicine. The experimental procedures were approved by the Animal Ethics Committee of the Anhui University of Traditional Chinese Medicine (number AHUCM - rats − 2020017).
Rats were housed at 18-22 °C with 40-60% humidity and access to food and water ad libitum with a 12 h light/dark cycle (n = 9). After 1 week of adaptation, rats were divided randomly into three groups (n = 3) (control, adriamycin model, and QTXZG administration). On Days 1 and 14, the model and QTXZG administration groups were injected with adriamycin (3.5 and 3.0 mg/kg, respectively) via the tail vein. Urine samples were collected on the 21st day of the experimental period, housing each rat individually in a metabolic cage for 24 h (urinary protein ≥ 50 mg/kg). Rats in the QTXZG administration group were given QTXZG granules (10.8 g/kg), and the control and adriamycin groups were given an equivalent solvent (physiological saline) once per day for 30 days.
Rats were anesthetized intraperitoneally with sodium pentobarbital (30 mg/kg, intraperitoneal injection, IP). Aorta blood was obtained immediately after sodium pentobarbital anesthesia was administered to rats. Glomerular tissues were harvested to store at −80 °C. Following anesthesia, the rats were sacrificed by cervical decapitation.
QTXZG serum concentration preparation
Twenty male SPF SD rats were randomly divided into the control (n = 7) or QTXZG (n = 13) group. Control group rats were administered normal saline (intragastric, 2 mL/100 g). QTXZG group rats were administered QTXZG (intragastric, 2 mL/100 g), which was 10 times the clinical dose of QTXZG (intragastric, 27 g/kg), once daily for 7 days. Rats were anesthetized with 3% pentobarbital sodium 1 h after intragastric administration on Day 7. Blood samples were obtained from rats’ abdominal aortas and allowed to coagulate for 2 h at room temperature. The supernatant was centrifuged at 4 °C (3000 rpm, 15 min) for inactivation (56 °C, 30 min). It was then filtered, bacteria were removed with a 0.22 µm microporous filter membrane, and the samples were packed in groups and frozen at −80 °C for later use. The drug concentration was prepared before use. The optimal QTXZG serum concentrations were screened using the CCK-8 assay.
Cell culture
Rat glomerular podocyte cell lines were purchased from Hunan Fenghui Biotechnology Co., Ltd., and cultured in 5% CO2 at 37 °C. A puromycin aminonucleoside (PAN) (50 µg/mL)-induced cell nephritis model was used, and the cells were randomly divided into three groups: control (glomerular podocyte + normal rat serum), model (glomerular podocyte + PAN + normal rat serum), and QTXZG (glomerular podocyte + PAN + optimal QTXZG serum concentration).
Overexpression plasmid transfection
The Rab7 overexpression plasmid (635 bp) was designed by General Biol Co., Ltd. (Anhui, China). Lipofectamine 2000 (Thermo, USA) was used to transfect the Rab7 overexpression plasmid into rat glomerular podocytes according to the manufacturer’s instructions.
CCK-8 cell activity assay to screen for optimal QTXZG serum concentration and timing
Cell viability was determined via a CCK-8 kit (Bestbio, Shanghai, China) and titrated for the optimal time and serum concentration. A 100 µL glomerular podocyte suspension was inoculated into 96 well plates at a cell density of 5 × 104 cells/well for 12 h at 37 °C in a 5% CO2-saturated humidity incubator. Cells were divided into two groups: control group (cell, PAN) and control + QTXZG serum (cell, PAN, and 1.25%, 2.5%, 5%, 10%, 20%, or 40% serum QTXZG). Cells were removed 0, 12, 24, 48, or 72 h after treatment, and 10 μL of CCK-8 reagent was added to each well, after which they were placed in an incubator for 1 h and removed, and the OD value of each well was measured using a microplate analyzer at a wavelength of 450 nm. The cell viability rate was calculated as follows:
Cell viability rate (%) = [ODdrug - ODblank]/[ODcontrol - ODblank] × 100%
Histopathological examination
Glomerular tissues were fixed in 10% formaldehyde solution, embedded in paraffin, and sliced into 4 µm thick sections. Sections were then stained with hematoxylin and eosin (HE) and Masson trichrome, after which images were observed under a × 200 optical microscope.
Proteomics analysis
For extraction of proteins, renal cortex tissues were homogenized with SDT buffer containing a protease inhibitor cocktail (150 mM Tris-HCl, pH 8.0, 4% SDS, 100 mM dithiothreitol). The homogenate was then boiled for 5 min and centrifuged at 40,000 g for 30 min. The supernatant was collected to measure protein concentrations by a bicinchoninic acid assay (BCA, Pierce, USA). For protein digestion, trypsin solution with triethylamine borane (150 µL, 50 mM) was added to the proteins at 1:50 (M:M) overnight at 37 °C. Peptides from each sample were labeled by tandem mass tags. Peptides were fractionated using the Agilent 1200 LC system (Agilent Technologies, Santa Clara, CA, USA). They were reconstituted with buffer A (20 mM ammonium acetate in H2O, pH 10) and loaded onto a C18 column. Elution was performed via 5-35% buffer B (20 mM ammonium acetate, 90% ACN/H2O, pH 10) for 35 min, 35-90% buffer B for 5 min, and 90% buffer B for 5 min. Elution was monitored by measuring the absorbance at 214 nm, and fractions were collected every min. The eluted peptides were pooled into 10 fractions and vacuum dried. A Q Exactive Mass Spectrometer (Thermo Fisher Scientific, Waltham, MA, USA) was run in positive ion mode in a data-dependent manner: full MS scan from 350 to 2000 m/z; full scan resolution at 70,000; MS/MS scan resolution at 17,500; normalized collision energy 28% and stepped 20%.
Protein identification and data analysis
Differentially expressed proteins were filtered based on fold change > 1.5 and p < 0.05. OmicShare tools (https://www.omicshare.com/tools/) were used for Gene Ontology (GO) and Kyoto Encyclopedia of Genes and Genomes (KEGG) analyses of the differentially expressed proteins. Metascape (https://metascape.org/) was used to analyze the protein–protein interaction network. The intersection of proteins with the autophagy database (http://hamdb.scbdd.com/home/index/) was identified.
Transmission electron microscopy
Cells were collected from each group, fixed with 2.5% glutaraldehyde and 1% ozone acid, dehydrated with acetone and injected with epoxy resin embedding agent. The samples were then sliced and stained, and the cell ultrastructure was observed via transmission electron microscopy.
Enzyme-linked immunosorbent assay
Cellular IL-18 and IL-1β levels were measured using commercial enzyme-linked immunosorbent assay (ELISA) kits (RuiXin Biotech, Quanzhou, China) according to the manufacturers’ instructions. All measurements were performed in duplicate and are expressed as pg/mL. IL-18 and IL-1β levels were measured using an enzyme-labeled instrument.
Immunofluorescence analysis
Collected cells were washed twice with PBS and fixed in 4% paraformaldehyde for 20 min. Then, 0.3% Triton X-100 was used to permeate for 20 min at room temperature, and the sections were blocked with goat serum for 30 min at 37 °C. Finally, the primary antibody was added and incubated for 60 min at 37 °C. The antibodies were diluted as follows: Pink1, 1:100; Rab7, 1:100; Podocalyxin (Podxl), 1:100; and IL-1β, 1:100. The primary antibody was removed, and the appropriate secondary antibody was added and incubated for 30 min at 37 °C. Nuclei were stained with DAPI solution. All images were photographed by fluorescence microscopy.
Cytometry analysis
An Annexin V-FITC/PI apoptosis kit (Solarbio, Beijing, China) was used according to the manufacturer’s instructions. Cells were collected and washed twice with precooled PBS buffer containing 5 µL of Annexin V-FITC for 5 min at room temperature in the dark. Then, 5 µL of propidium iodide was added to each sample for 5 min at room temperature in the dark. Finally, flow cytometry was performed.
Western blot analysis
Each cell group was collected and lysed in RIPA buffer (Biosharp, HeFei, China) to complete protein extraction. The collected protein samples were added to a sodium dodecyl sulfate polyacrylamide gel (Activagen Bio, Hefei, China) at a ratio of 1:4 to separate the proteins. Then, the gels were electrotransferred to polyvinylidene difluoride membranes (Millipore, USA). The membranes were blocked using 5% skim milk powder. Then, the following primary antibodies were added overnight at 4 °C: anti-β-Actin (Zs-BIO, USA, 1:1,000); anti-Pink1 (Affinity, USA, 1:1,000); anti-Parkin (Affinity, USA, 1:1,000); anti-Rab7 (ZENBIO, ChengDu, China, 1:1,000); anti-NLRP3 (Affinity, USA, 1:1,000); anti-Caspase-1 (Affinity, USA, 1:1,000); anti-Podxl (ABclonal, WuHan, China, 1:1,000); anti-Beclin1 (Affinity, USA, 1:500); anti-LC3 II/LC3 I (Affinity, USA, 1:500); and anti-P62 (CST, USA, 1:1000). The membranes were washed with PBS buffer and incubated with the secondary antibody for 2 h at room temperature, after which the membrane signal was observed via an enhanced chemiluminescence kit (Thermo, USA). Film strips were analyzed using ImageJ software 1.8.0.
Statistical analysis
Spectral data were screened using Mascot version 2.3.0 (Matrix Science) with taxonomy set to Homo sapiens (UniProt_human_9606, containing 41,917 sequences) using Proteome Discoverer 1.4 (Thermo Fisher Scientific). Quantitative protein ratios were normalized to correct for systematic error/bias. Data execution preventions (DEPs) were indicated when p < 0.05, and fold changes were > 1.5 or < 0.83 for the two sample batches. Statistical analyses were performed with SPSS version 21.0. Experimental data are presented as the mean ± standard deviation.
Results
Histopathology findings
HE staining revealed that the glomerulus was atrophic and deformed, the glomerular basement membrane was thickened significantly, and inflammatory cell infiltration was observed around the glomerulus in the model group compared with the normal group. However, compared with those of the model group, the glomerulus tended to be normal, basement membrane thickening was reduced, and inflammatory cell infiltration was significantly reduced in the QTXZG group. Masson trichrome staining indicated that in the model group, the glomerulus was significantly atrophic and deformed, the glomerular basement membrane thickened, and collagen deposition increased compared to those of the control group. In the QTXZG group, the glomerulus tended to be normal, and basement membrane thickening and collagen deposition were reduced compared with those in the model group ().
Figure 1. Proteomic analysis of glomerular tissue in adriamycin-induced rats. (A). HE and Masson’s staining were used to observe pathological changes in glomerular tissue (200 ×). a: Glomerular basement membrane thickening; b: glomerular atrophy and deformation; c: inflammatory cell infiltration. a1: collagen deposition; b1: glomerular basement membrane thickening; c1: glomerular atrophy and deformation. (B). Venn diagram of differentially expressed proteins (DEPs) coregulated in the model (M) and QTXZG (Q) groups.
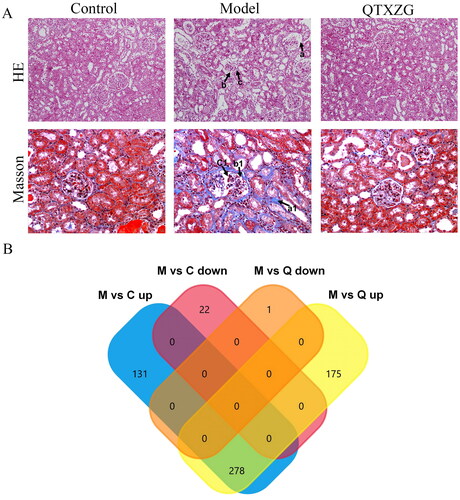
Proteomics findings
Differentially expressed proteins (DEPs) findings
There were 1714 total proteins identified in the rat renal cortex. We found that 409 proteins were upregulated and 22 were downregulated in the model group compared with the control group (FC > 1.5, p < 0.05). Moreover, 453 proteins were upregulated and one was downregulated in the model group compared with the QTXZG group (FC > 1.5, p < 0.05) ().
GO enrichment findings
GO enrichment analysis describes protein biological functions, which were divided into cellular components (CC), biological processes (BP), and molecular functions (MF). As shown in , the top 10 BPs, CCs, and MFs were screened according to the number of enriched genes. The top 20 GO enrichment results are shown in . In particular, these DEPs were involved in important BPs, mainly the nucleotide metabolic process, nucleotide biosynthetic process, cellular amino acid metabolic process, and other amino acid metabolic processes. We found that these BPs mainly occur in the mitochondria; thus, we preliminarily speculated whether the pathogenesis of CGN is related to mitochondria.
Figure 2. GO enrichment analysis of DEPs. There were 278 DEPs for the top 20 GO annotations. The outer circle is the GO name, the middle circle is the difference (up and down), and the inner circle is the z score.
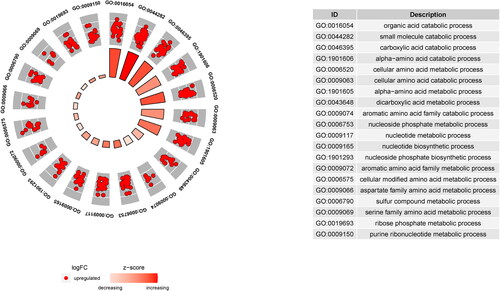
Table 1. Screened top 10 BP, MF, and CC according to number of GO-enriched genes.
KEGG pathway enrichment analysis
KEGG analysis clarified the DEP pathways. The top 17 pathway enrichment annotations are shown in . The main enrichment-derived KEGG pathways were phagosome, endocytosis, glutathione metabolism, and citrate cycle (tricarboxylic acid cycle, TCA cycle). These pathways also mainly occur in the mitochondria, which further confirms our speculation.
Protein-protein interaction network findings
Protein-protein interaction (PPI) networks describe relations among proteins. The PPI network among the DEPs is shown in and consists of 278 protein nodes. The PPI network was composed of six central proteins (RAB7A, CCT3, RPS20, RPS30, GART, RPSA). shows the PPI network of Rab7a as the hub protein. Surprisingly, Rab7a plays a role in autophagosome-lysosome fusion as well as autolysosome maturation; in addition, Rab7a plays an important role in pink/parkin-mediated mitophagy (Tang et al. Citation2023). Therefore, we speculated that the pathogenesis of CGN is related to mitophagy (Rab7 was equivalent to Rab7a unless otherwise mentioned below).
Intersection with the autophagy database
There were 278 DEPs that intersected with the autophagy database (). Eight intersections with autophagy-related proteins and their diseases or functions are shown in , among which the primary proteins were Rab7a, ctnnb1, and lamp1. shows that in the eight-protein PPI network based on the degree values, the Rab7a degree value was the highest and constituted the central protein in the PPI network. The top 20 GO enrichment analyses are shown in . We found that especially important biological processes were macroautophagy and autophagy. With the eight autophagy-related proteins, KEGG analysis was used to clarify the pathways. The top 25 KEGG pathways are shown in . The most representative pathways were autophagy-animal, mitophagy-animal, phagosome, and ferroptosis. Rab7 was an autophagy hub protein among the 8 autophagy proteins and autophagy, which was the vital pathway. Based on the above 278 DEPs GO and KEGG analysis speculation, we considered whether Rab7 is involved in mitophagy and affects the pathogenesis of CGN.
Figure 6. Identification and analysis of autophagy-related proteins. (A). UpSet Venn diagram of the intersection between DEPs and autophagy database proteins. The green and yellow horizontal histograms on the left represent the statistical value of the DEPs and autophagy database proteins, respectively. A single point in the middle matrix represents a specific protein of DEPs or autophagy database proteins, the red connection between the point and the point represents the unique intersection of DEPs and autophagy database proteins, and the vertical histogram represents the corresponding intersection protein value. (B). the interaction network of eight intersecting autophagy proteins.
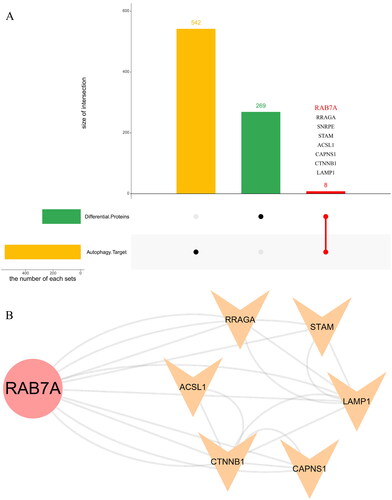
Figure 7. Z score Bubble plot of the top 20 significantly enriched differential pathways. The X-axis represents up-down normalization, and the Y-axis represents the -log10 P value. Blue indicates biological process, green indicates cellular component, and the table on the right shows the 20 GO terms with the smallest P value.
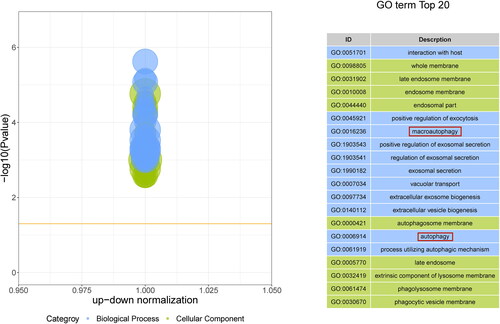
Figure 8. KEGG enrichment pathway analysis of autophagy-related proteins. Top 30 KEGG enrichment analyses of eight DEPs, X-axis represents enrichment factor, Y-axis represents pathway, and color represents P value (blue is high; red is low); lower P values indicate more significant degree of enrichment. Dot size indicates the number of DEPs, with larger dots indicating a larger number.
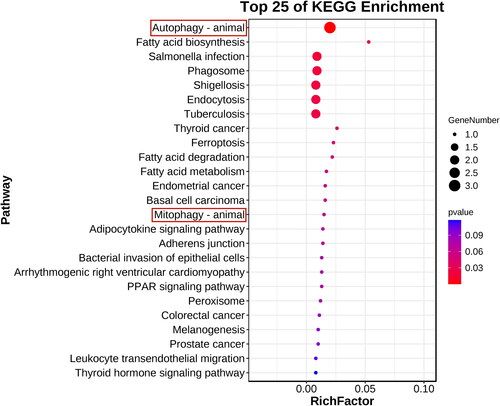
Table 2. 8 proteins selected from the intersection of 278 differential proteins and autophagy database.
Optimum QTXZG serum conditions
shows that the optimal condition was the cell median inhibitory concentration (IC50). At concentrations of 20% and 24 h, the cell viability rate (i.e., death rate) was ∼ 50%.
Transmission electron microscopy findings
shows normal mitochondria, a complete structure, orderly arranged mitochondrial cristae, and autophagosomes in the control group. Compared with the control group, the model group had abnormal mitochondrial cristae, mitochondrial damage, and decreased autophagosomes. Electron microscopy data revealed increased autophagosomes and diminished mitochondrial damage with QTXZG administration.
Immunofluorescence findings
Immunofluorescence (IF) analysis illustrated that Pink1, Rab7, and Podxl levels were decreased, and IL-1β levels were increased in the model group compared with the control group. However, Pink1, Rab7, and Podxl levels were increased, and IL-1β levels were decreased in the QTXZG group compared with the model group ().
Figure 10. Immunofluorescence analysis of glomerular podocytes. (A-D). IF analyses of Pink1, Rab7, Podxl, and IL-1β expression. Blue spots represent the Nucleus, and red spots represent various protein expression levels. (E). the column diagram shows protein expression. **p < 0.01, compared with the control group. ##p < 0.01, compared with the model group.
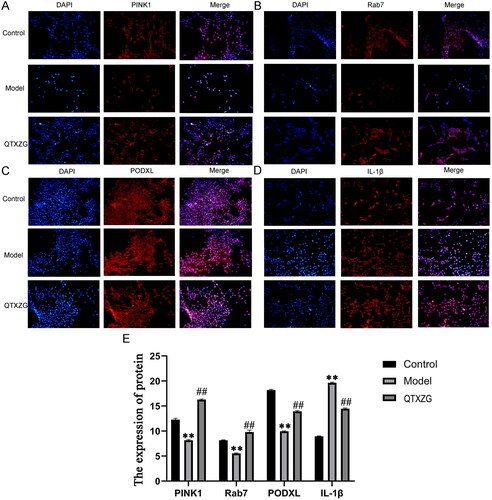
ELISA findings
ELISAs showed that IL-18 and IL-1β expression was elevated in the model group compared with the control group. In the QTXZG group, IL-18 and IL-1β expression was reduced compared with that in the model group ().
Figure 11. Effect of QTXZG on autophagy-related signaling proteins in PAN-induced glomerular podocytes. (A). ELISAs of IL-18 and IL-1β expression. (B). apoptosis was detected by flow cytometry, and the column shows the total apoptosis rate. **p < 0.01, compared with the control group. ##p < 0.01, compared with the model group. (C). caspase-1, Podxl, NLRP3, Pink1, and Rab7 protein expression was determined by Western blotting analysis. The column shows protein expression. **p < 0.01, compared with the control group. ##p < 0.01, compared with the model group.
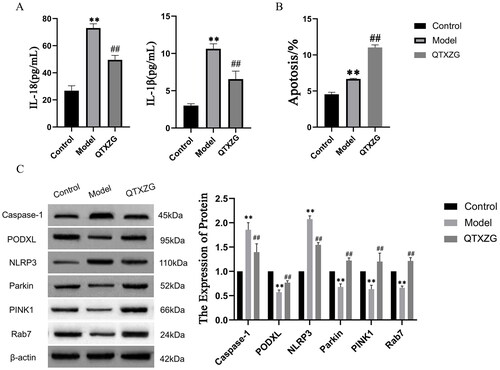
Flow cytometry findings
Flow cytometry () showed that apoptosis rates in the model group were increased compared with those in the control group. The apoptosis rates in the QTXZG group were increased compared with those in the model group.
Western blot findings
Western blot (WB) analysis was performed to identify the cellular expression of cysteine protease 1 (caspase-1), nucleotide-binding oligomerization domain-like receptor protein 3 (NLRP3), Podxl, Parkin, Pink1, and Rab7 after QTXZG administration. In the model group, Caspase-1 and NLRP3 levels were increased, and Podxl, Parkin, Pink1, and Rab7 levels were decreased compared with those in the control group. QTXZG administration significantly altered Caspase-1, NLRP3, Podxl, Parkin, Pink1, and Rab7 levels ().
A combination of Rab7 and P62, Beclin 1, and LC3 II/LC3 I was tested to determine the effect of Rab7 expression and whether Rab7 interacts with autophagy. WB analysis showed that compared with that of the model group, the expression level of P62 was decreased and Beclin 1 and LC3 II/LC3 I were increased when Rab7 was overexpressed (). These results confirmed that Rab7 plays a crucial role in autophagy.
Discussion
CGN is the most common cause of glomerulosclerosis and end-stage renal disease, which reduces patients’ quality of life and increases the economic pressures on their families and society (Liu et al. Citation2023). However, the current clinical approach is medical management aimed at improving CGN symptoms with antihypertensive, anticoagulant, hormonal, and cytotoxic drugs (Wei et al. Citation2022). QTXZG, a traditional Chinese compound preparation, has shown excellent clinical effects for CGN treatment. However, the molecular mechanism of QTXZG in CGN treatment has until now been unclear; thus, its therapeutic mechanism was studied herein.
The CGN rat model was established by adriamycin injection via the tail vein. Using proteomics, we identified 278 differentially expressed proteins in the model group compared with the QTXZG and control groups. Both GO and KEGG pathway analyses revealed interesting mitochondrial function results, such as the tricarboxylic acid cycle, NAD metabolism, and biosynthesis processes. These data suggested that biological changes occurred in mitochondria both before and after QTXZG administration. Next, PPI analysis showed DEPs that intersected with the autophagy database, including the autophagy protein Rab7a, suggesting that its mitochondrial activity may be the mechanism by which QTXZG affects CGN.
Mitochondria are important eukaryotic cell organelles; they produce ATP via oxidative phosphorylation and provide 95% of the cell’s energy requirements. They are also involved in metabolic signal transduction, inflammation, and apoptosis regulation. The kidney is rich in mitochondria, which play a key role in its function, and mitochondrial damage and dysfunction are major factors in many chronic and acute kidney diseases (Tang et al. Citation2021). Maintaining mitochondrial homeostasis and metabolic balance is crucial for kidney function (Bhargava and Schnellmann Citation2017). When mitochondrial damage and dysfunction occur, mitophagy is induced to maintain cell homeostasis, removing damaged or excess mitochondria (Su et al. Citation2023). Transmission electron microscopy showed that abnormal mitochondrial cristae and decreased autophagosomes were apparent in the model group. Interestingly, we also found that mitochondrial damage was reduced after QTXZG treatment.
Serine-threonine kinase (Pink1) and E3 ubiquitin ligase (Parkin) are important proteins that induce mitophagy, and the Pink1/Parkin signaling pathway induces mitophagy (Zhou et al. Citation2023). When mitochondria are damaged, the membrane potential changes and depolarizes, pink1 in the outer mitochondrial membrane is autophosphorylated, and parkin binding to ubiquitin is activated. Then, parkin is recruited by pink1 and aggregates outside the damaged mitochondria. Parkin can lead to ubiquitination of mitochondrial outer membrane proteins. Other autophagy-related proteins are then recruited to the mitochondria and mediate mitophagy (Ding et al. Citation2022). Zhang et al. (Citation2020) reported that resveratrol reversed Pink1/Parkin-mediated mitochondrial autophagy to reduce cadmium-induced kidney toxicity and thereby played a protective role in renal injury. Li et al. (Citation2017) reported that Pink1/Parkin pathway activation alone improves impaired podocytes in diabetic nephropathy.
Mitophagy is the formation of autophagosomes, after which fusion with lysosomes forms autophagolysosomes, and then, proteolytic enzymes in lysosomes degrade mitochondria. Therefore, lysosome degradation is another important step in mitophagy. Rab7, a member of the RAB GTPase family (Rab7a and Rab7b are Rab7 subtypes, sharing ∼50% homology), is a key small GTP enzyme that promotes lysosome-autophagosome fusion and is related to autophagosome maturation (Hyttinen et al. Citation2013; Li et al. Citation2023).
In our in vitro cell assays, cell apoptosis was increased in the model group, and QTXZG treatment may have increased excessive mitophagy, which further increased cell apoptosis. We found that the expression of P62 was decreased and the levels of Beclin 1 and LC3 II/LC3 I were increased when Rab7 was overexpressed. These results indicated that Rab7 overexpression could activate autophagy. Moreover, IF and WB results confirmed that pink1, parkin, and rab7 levels were increased after QTXZG treatment. The above results confirmed that QTXZG could regulate pink1- and parkin-level-induced mitophagy, improve lysosomal degradation function, and enhance damaged mitochondria clearance in the CGN, thus exerting a protective effect on the kidney.
CGN characteristics include glomerular inflammation, with infiltrating mononuclear phagocytes producing typical NLRP3 inflammasome signaling and promoting NLRP3 inflammasome activation (Conley et al. Citation2017). The NLRP3 inflammasome is composed of NLRP3 and precursor cysteine protease 1 (pro-caspase-1), the adapter protein apoptosis-associated speck-like protein. It is the main component of the inflammatory immune response. NLRP3 inflammasome activation can activate pro-caspase-1, which further promotes the maturation and secretion of the inflammatory cytokines IL-1β and IL-18, leading to amplification of the inflammatory response (Huang et al. Citation2021). However, excessive active caspase-1 and IL-1β, or other inflammasome products, may reduce podocyte-specific protein levels, including nephrin and podocalyxin (Yi et al. Citation2006; Abais et al. Citation2013).
Podxl is a sialomucin CD34, podocyte marker protein, and major protein on glomerular podocyte surfaces (Ramdin et al. Citation2023). Podocytes form the main glomerular filtration membrane component. In glomerular diseases such as CGN, podocyl damage leads to increased filtration membrane permeability (Doyonnas et al. Citation2001; Refaeli et al. Citation2020). Podxl is released from vesicles or microvilli and, when increased in urine, results in proteinuria. Therefore, in CGN, inflammatory product increases lead to increased podxl levels in urine and thus urinary protein. As such, we suspected that inflammatory products and urinary protein podxl would be decreased after QTXZG treatment. In our in vitro cell experiments, IF and WB analyses showed that NLRP3, caspase-1, IL-18, and IL-1β levels were increased in the model group and that they were decreased significantly after QTXZG treatment. Moreover, podxl expression was increased significantly before and after QTXZG treatment, which suggested that the degree of inflammation it caused decreased and that proteinuria was reduced. This phenomenon may also improve podocyte injury and protect against CGN, consistent with our expectations.
Conclusions
These cumulative findings indicated that a novel CGN treatment may be mediated by targeting the Pink1-Parkin-Rab7 axis, which regulates autophagy and CGN progression. Our results suggested that QTXZG treatment may reduce inflammation and proteinuria podxl levels; enhance Pink1/Parkin-induced mitochondrial autophagy by increasing Pink1, Parkin, and Rab7 levels; improve lysosomal degradation; and remove damaged mitochondria to protect the kidney. Our findings herein provide new insights into the mechanism by which QTXZG acts as a cytoprotective autophagy therapy and identify novel potential therapeutic targets for CGN treatment.
Author contributions
JG: conceptualization, supervision; XQ: wrote the original draft preparation, project administration, and funding acquisition; HC: methodology, validation, formal analysis, reviewing and editing, experiment; XX: conceptualization, supervision; XZ: software.
Acknowledgments
We are grateful to Mr. Qiang Fan (Ao Ji Bio-tech Co., Ltd., Shanghai, China) for providing help in proteomics analysis and Mr. Fei Gao (Hefei Yuanen Biotechnology Co., Ltd.) for providing help in data analysis.
Disclosure statement
No potential conflict of interest was reported by the authors.
Additional information
Funding
References
- Abais JM, Zhang C, Xia M, Liu Q, Gehr TW, Boini KM, Li PL. 2013. NADPH oxidase-mediated triggering of inflammasome activation in mouse podocytes and glomeruli during hyperhomocysteinemia. Antioxid Redox Signal. 18(13):1537–1548. doi:10.1089/ars.2012.4666.
- An P, Dang HM, Shi XM, Ye BY, Wu XL. 2014. “Qufeng Tongluo” acupuncture prevents the progression of glomerulonephritis by decreasing renal sympathetic nerve activity. J Ethnopharmacol. 155(1):277–284. doi:10.1016/j.jep.2014.05.019.
- Bhargava P, Schnellmann RG. 2017. Mitochondrial energetics in the kidney. Nat Rev Nephrol. 13(10):629–646. doi:10.1038/nrneph.2017.107.
- Blom K, Odutayo A, Bramham K, Hladunewich MA. 2017. Pregnancy and glomerular disease: a systematic review of the literature with management guidelines. Clin J Am Soc Nephrol. 12(11):1862–1872. doi:10.2215/CJN.00130117.
- Chan K, Shaw D, Simmonds MSJ, Leon CJ, Xu QH, Lu AP, Sutherland I, Ignatova S, Zhu YP, Verpoorte R, et al. 2012. Good practice in reviewing and publishing studies on herbal medicine, with special emphasis on traditional Chinese medicine and Chinese materia medica. J Ethnopharmacol. 140(3):469–475. doi:10.1016/j.jep.2012.01.038.
- Choi ME. 2020. Autophagy in kidney disease. Annu Rev Physiol. 82:297–322. doi:10.1146/annurev-physiol-021119-034658.
- Conley SM, Abais JM, Boini KM, Li PL. 2017. Inflammasome activation in chronic glomerular diseases. Curr Drug Targets. 18(9):1019–1029. doi:10.2174/1389450117666160817103435.
- Dagar N, Kale A, Steiger S, Anders HJ, Gaikwad AB. 2022. Receptor-mediated mitophagy: an emerging therapeutic target in acute kidney injury. Mitochondrion. 66:82–91. doi:10.1016/j.mito.2022.08.004.
- Dai Y, Chen XX, Yang H, Yang JY, Hu QC, Xiao XL, Guo XC, Zeng JH, Ma X. 2022. Evidence construction of Huangkui capsule against chronic glomerulonephritis: a systematic review and network pharmacology. Phytomedicine. 102:154189. doi:10.1016/j.phymed.2022.154189.
- Ding WX, Dong YB, Zhang XL. 2022. Globular adiponectin protects hepatocytes against intermittent hypoxia-induced injury via pink1/parkin-mediated mitophagy induction. Sleep Breath. 26(3):1389–1397. doi:10.1007/s11325-021-02508-8.
- Doyonnas R, Kershaw DB, Duhme C, Merkens H, Chelliah S, Graf T, Mcnagny KM. 2001. Anuria, omphalocele, and perinatal lethality in mice lacking the CD34-related protein podocalyxin. J Exp Med. 194(1):13–27. doi:10.1084/jem.194.1.13.
- Galluzzi L, Green DR. 2019. Autophagy-independent functions of the autophagy machinery. Cell. 177(7):1682–1699. doi:10.1016/j.cell.2019.05.026.
- Gao JR, Qin XJ, Jiang H, Wang T, Song JM, Xu SZ. 2016. The effects of Qi Teng Xiao Zhuo granules, traditional Chinese medicine, on the expression of genes in chronic glomerulonephritis rats. J Ethnopharmacol. 193:140–149. doi:10.1016/j.jep.2016.08.011.
- Gao JR, Zhou CD. 2004. Orthogonal experiment on the extraction process of Senkang granules. J Anhui Univ Chin Med. 23:44–46. In Chinese.
- Gao YC, Jiang NN, Qin XJ, Jiang H, Wei LB, Gao JR. 2023. High-throughput data on circular RNA reveal novel insights into chronic glomerulonephritis. Genes Genomics. 45(4):475–490. doi:10.1007/s13258-022-01320-2.
- Ge DD, Gao JR, Wu X, Yang L, Wei LB, Qin XJ, Song JM. 2017. Study on improving the quality standard of Qi Teng Xiao Zhuo granules. Chin J Tradit Clin Med. 29:650–655. In Chinese.
- Huang Y, Xu W, Zhou RB. 2021. NLRP3 inflammasome activation and cell death. Cell Mol Immunol. 18(9):2114–2127. doi:10.1038/s41423-021-00740-6.
- Hu SJ, Cao EZ, Wang YP, Fang Q, Liu JS, Mao YP. 2008. Clinical study on the therapeutic effect of shenkang granule on chronic nephritis. Chin J Tradit Clin Med. 20:140–141. In Chinese.
- Hyttinen JM, Niittykoski M, Salminen A, Kaarniranta K. 2013. Maturation of autophagosomes and endosomes: a key role for rab7. Biochim Biophys Acta. 1833(3):503–510. doi:10.1016/j.bbamcr.2012.11.018.
- Liu T, Zhuang XX, Qin XJ, Wei LB, Gao JR. 2023. The potential role of N6-methyladenosine modification of LncRNAs in contributing to the pathogenesis of chronic glomerulonephritis. Inflamm Res. 72(3):623–638. doi:10.1007/s00011-023-01695-2.
- Liu JS, Fang Q, Hu SJ, Wang YP, Lv Y, Zhao L. 2007. Clinical study of shenkang granules in the treatment of chronic glomerulonephritis. Liaoning J Tradit Chin Med. 34(2):171–172. In Chinese.
- Liu T, Gao YC, Qin XJ, Gao JR. 2021. Exploring the mechanism of Jianpi Qushi Huayu Formula in the treatment of chronic glomerulonephritis based on network pharmacology. Naunyn Schmiedebergs Arch Pharmacol. 394(12):2451–2470. doi:10.1007/s00210-021-02159-2.
- Li W, Du MM, Wang QZ, Ma XJ, Wu LN, Guo F, Ji HF, Huang FJ, Qin GJ. 2017. FoxO1 promotes mitophagy in the podocytes of diabetic male mice via the PINK1/Parkin pathway. Endocrinology. 158(7):2155–2167. doi:10.1210/en.2016-1970.
- Li W, Jiang H, Ablat N, Wang C, Guo Y, Sun Y, Zhao X, Xu J, Zhang K, Ren R, et al. 2016. Evaluation of the acute and sub-chronic oral toxicity of the herbal formula Xiaoer Chaigui Tuire oral liquid. J Ethnopharmacol. 189:290–299. doi:10.1016/j.jep.2016.05.050.
- Li ZP, Lai MY, Li J, Yang DM, Zhao MY, Wang DD, Sun ZX, Wen P, Gou FT, Dai YX, et al. 2023. Rab7a GTPase is involved in mitophagosome formation and autophagosome-lysosome fusion in n2a cells treated with the prion protein fragment 106-126. Mol Neurobiol. 60(3):1391–1407. doi:10.1007/s12035-022-03118-5.
- Lopez-Novoa JM, Martinez-Salgado C, Rodriguez-Pena AB, Lopez-Hernandez FJ. 2010. Common pathophysiological mechanisms of chronic kidney disease: therapeutic perspectives. Pharmacol Ther. 128(1):61–81. doi:10.1016/j.pharmthera.2010.05.006.
- Minami S, Yamamoto T, Yamamoto-Imoto H, Isaka Y, Hamasaki M. 2023. Autophagy and kidney aging. Prog Biophys Mol Biol. 179:10–15. doi:10.1016/j.pbiomolbio.2023.02.005.
- Nakatogawa H. 2020. Mechanisms governing autophagosome biogenesis. Nat Rev Mol Cell Biol. 21(8):439–458. doi:10.1038/s41580-020-0241-0.
- Ramdin S, Naicker T, Baijnath S, Govender N. 2023. Kidney injury molecule-1 and podocalyxin dysregulation in an arginine vasopressin induced rodent model of preeclampsia. Eur J Obstet Gynecol Reprod Biol. 284:58–65. doi:10.1016/j.ejogrb.2023.03.012.
- Refaeli I, Hughes MR, Wong AK, Bissonnette MLZ, Roskelley CD, Wayne Vogl A, Barbour SJ, Freedman BS, Mcnagny KM. 2020. Distinct functional requirements for podocalyxin in immature and mature podocytes reveal mechanisms of human kidney disease. Sci Rep. 10(1):9419. doi:10.1038/s41598-020-64907-3.
- Rubio-Tomás T, Sotiriou A, Tavernarakis N. 2023. The interplay between selective types of (macro)autophagy: mitophagy and xenophagy. Int Rev Cell Mol Biol. 374:129–157. doi:10.1016/bs.ircmb.2022.10.003.
- Su LJ, Zhang JH, Gomez H, Kellum JA, Peng ZY. 2023. Mitochondria ROS and mitophagy in acute kidney injury. Autophagy. 19(2):401–414. doi:10.1080/15548627.2022.2084862.
- Suo TC, Wang HX, Li Z. 2016. Application of proteomics in research on traditional Chinese medicine. Expert Rev Proteomics. 13(9):873–881. doi:10.1080/14789450.2016.1220837.
- Tang CY, Cai J, Yin XM, Weinberg JM, Venkatachalam MA, Dong Z. 2021. Mitochondrial quality control in kidney injury and repair. Nat Rev Nephrol. 17(5):299–318. doi:10.1038/s41581-020-00369-0.
- Tang CZ, Deng JL, Xu RG, Li JP, Yin CC, Yang Y, Zhou YH, Deng FL. 2023. Micro/nano-modified titanium surfaces accelerate osseointegration via rab7-dependent mitophagy. Biomater Sci. 11(2):666–677. doi:10.1039/d2bm01528e.
- Vasilogianni AM, Al-Majdoub ZM, Achour B, Annie Peters S, Barber J, Rostami-Hodjegan A. 2022. Quantitative proteomics of hepatic drug-metabolizing enzymes and transporters in patients with colorectal cancer metastasis. Clin Pharmacol Ther. 112(3):699–710. doi:10.1002/cpt.2633.
- Wang Y, Wang J, Gao JR, Liu XC, Wei LB, Zhang JR. 2019. Comparative study on spray drying and wet granulation of Qi Teng Xiao Zhuo granules. Chin Med J Res Pract. 33:50–53. In Chinese.
- Wei LB, Gao JR, Gao YC, Liu XC, Jiang H, Qin XJ. 2020. Effect of the traditional Chinese medicine Qi Teng Xiao Zhuo granules on chronic glomerulonephritis rats studied by using long noncoding RNAs expression profiling. Gene. 728:144279. doi:10.1016/j.gene.2019.144279.
- Wei LB, Gao JR, Shan LL, Zhuang XX, Song JM. 2016. Effect of Qi Teng Xiao Zhuo granule on the treatment and pathomorphology of rats with adriamycin chronic nephritis. Pharm Clin Chin Mater Med. 32:149–151. In Chinese.
- Wei LB, Wu X, Wang XY, Wang YP, Meng M. 2010. Study on quality control of Shenkang granules. Chin J Inf Tradit Chin Med. 19:50–51. In Chinese.
- Wei MJ, Qiu ZM, Li H, Lu B, Wang CH, Ji LL. 2022. Integrating network pharmacology approach and experimental validation to reveal the alleviation of Shenkangning capsule on chronic nephritis. J Ethnopharmacol. 299:115676. doi:10.1016/j.jep.2022.115676.
- Yi F, Zhang AY, Li N, Muh RW, Fillet M, Renert AF, Li PL. 2006. Inhibition of ceramide-redox signaling pathway blocks glomerular injury in hyperhomocysteinemic rats. Kidney Int. 70(1):88–96. doi:10.1038/sj.ki.5001517.
- Zhang Q, Zhang C, Ge J, Lv MW, Talukder M, Guo K, Li YH, Li JL. 2020. Ameliorative effects of resveratrol against cadmium-induced nephrotoxicity via modulating nuclear xenobiotic receptor response and pink1/parkin-mediated mitophagy. Food Funct. 11(2):1856–1868. doi:10.1039/c9fo02287b.
- Zhou X, Liu KJ, Li JJ, Cui LY, Dong JS, Li J, Meng X, Zhu GQ, Wang H. 2023. Pink1/parkin-mediated mitophagy enhances the survival of Staphylococcus aureus in bovine macrophages. J Cell Mol Med. 27(3):412–421. doi:10.1111/jcmm.17664.