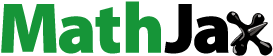
Abstract
Context
Jiang-Zhi-Ning (JZN), a traditional Chinese medicinal formula, is used to treat hyperlipidemia in clinics.
Objective
To screen the hypolipidemic “bioequivalent substance system (BSS)” of JZN and elucidate the potential hypolipidemic mechanism.
Materials and methods
In vitro, the TG content in HepG2 cells was determined after the intervention of the combination of advantageous components (CAC) by uniform design. In vivo, hyperlipidemia models were established by Triton WR-1339 (400 mg/kg; i.p.) in male ICR mice, and corresponding treatments were administered via oral administration once. The mice were divided into 12 groups (n = 5): control, hyperlipidemic model, simvastatin (positive control, 20 mg/kg), gradient doses of JZN granules (2, 4 and 8 g/kg) and the hypolipidemic effective extraction (HEE) of JZN (120, 240 and 480 mg/kg) and CAC groups (20, 40 and 160 mg/kg). Serum TC, TG, LDL-C and HDL-C were performed after 24 h. Transcriptomics and qRT–PCR technology were used to explore the mechanism of the “BSS” of JZN.
Results
In vitro, the ratio of CAC was determined. CAC could reduce the TG content in HepG2 cells (77.21%). Compared with the model group, the high dose of CAC could markedly decrease the levels of TC (61.86%), TG (105.54%) and LDL-C (39.38%) and increase the level of HDL-C (232.67%). CAC was proved to be the “BSS”. Transcriptomics and qRT–PCR analysis revealed CAC regulated non-alcoholic fatty liver disease, bile secretion, PPAR and adipocytokine signalling pathway.
Discussion and conclusions
These findings provided new feasible ideas and methods for the elucidation of the pharmacodynamic material basis.
Introduction
Hyperlipidemia is a pathological condition of lipid metabolism disorder for a variety of reasons and is also a common cause of atherosclerosis and other diseases. In recent years, many researchers explored the mechanism of traditional Chinese medicine and chemical medicine in preventing and treating hyperlipidemia. Regardless if it is traditional Chinese medicine or chemical medicine, it usually plays a role in lowering blood lipids by regulating cholesterol metabolism, triglyceride metabolism, bile acid metabolism, etc. At the same time, recent research showed that some targets play an important role in treating hyperlipidemia, such as PCSK9 and ANGPTL3 (Zhu et al. Citation2016; Rosenson et al. Citation2020; Ahamad and Bhat Citation2022; Shakir et al. Citation2022). Traditional Chinese medicine, which has the advantages of significant efficacy, diverse pathways of action and few side effects in the treatment of hyperlipidemia over a long history, has become an exciting topic in recent years in this field.
The traditional Chinese medicine (TCM) Jiang-Zhi-Ning (JZN) is composed of Polygonum multiflorum Thunb. [Polygonaceae] (the root tuber), Crataegus pinnatifida Bge. [Nymphaeaceae] (the fructus), Nelumbo nucifera Gaertn. [Rosaceae] (the leaves) and Cassia obtusifolia L. [Leguminous] (the seed), and it was recorded in Volume 13 of the Chinese Medicine Prescriptions Standard issued by the PRC health ministry. JZN, which is commonly used in the clinical treatment of hyperlipidemia, promotes blood circulation of the coronary artery, softens blood vessels, resists arrhythmia and lowers blood lipids (Chinese Pharmacopoeia Commission Citation2020). Modern pharmacological studies showed that effective extraction of JZN could significantly reduce the contents of TC, TG, LDL-C, CRI and AI in hyperlipidemic rats (Chen et al. Citation2012) and regulate cholesterol metabolism by regulating LDL receptor and Cyp7a1 mRNA expression levels (Chen et al. Citation2011). In the JZN prescription, Crataegus pinnatifida is the monarch drug, which contains flavonoids, triterpenes, phytosterols, organic acids, etc., with the effect of promoting the β-oxidation of fatty acids and inhibiting cholesterol synthesis (Lin et al. Citation2011; Niu et al. Citation2011; Kwok et al. Citation2013). Nelumbo nucifera is the minister drug and mainly contains flavonoids and alkaloids, among others, and it has the effects of lipid-lowering, antihypertensive and stopping bleeding (Li et al. Citation2017). Cassia obtusifolia is an adjuvant drug that contains anthraquinones and naphthol with the effects of reducing exogenous lipid uptake, inhibiting endogenous lipid synthesis and promoting lipid transport (Li et al. Citation2008; Gao et al. Citation2013; Wang et al. Citation2014). Polygonum multiflorum is an absorbent drug that contains flavonoids, anthraquinone, stilbene glycoside, polysaccharide, lecithin, etc., with the effect of increasing the liver lipase content and promoting the breakdown of liver lipids (Zuo et al. Citation2017; Zhang et al. Citation2019).
Commonly, TCM compound preparation shows the characteristics of a complex composition of chemical components, diverse pathways of action and ambiguous mechanisms of action (Hao et al. Citation2017). TCM compounds exert their clinical therapeutic efficacy by multiple active components acting on multiple targets cooperatively, rather than the linear addition of a few chemical components (Zhang and Feng Citation2019). TCM compounds usually contain many different types of chemical components, including several active components. These active components are combined to exert medicinal effects, with different chemical components having different pathways or mechanisms of action. Due to many factors, such as origin, harvest time and growing environment, the contents of active components in natural medicines vary, which will also affect the efficacy. At the same time, the relationship between the efficacy and concentration of active components is not an absolute linear relationship. Meanwhile, several types of effective components are combined together; in other words, the efficacy of TCM compounds, including several types of effective components combined together is the result of the synergistic effect of various components, which is not only related to the composition of the effective components but also related to the ratio and interaction of each component. Therefore, for TCM prescriptions, the basic research of pharmacodynamic substances includes not only the discovery of the active components but also the confirmation of the proportion of each active component as well as a clear explanation of its mechanism. Therefore, our group proposed the research idea of the TCM compound “bioequivalent substance system” (BSS). In other words, in TCM compounds, a group of effective components with the same pharmacodynamic effect, clear chemical composition and proportional relationship is the TCM compound “BSS”.
According to the research idea of the TCM compound “bioequivalent substance system”, our previous work demonstrated that quercetin-3-O-β-d-glucuronide, armepavine, emodin-8-O-β-d-glucoside, 2-hydroxy-1-methoxyaporphine, hyperoside and rubrofusarin-6-O-β-d-gentiobioside () (Zhang et al. Citation2021) were the hypolipidemic advantageous components of JZN, which had good hypolipidemic activity. However, the underlying mechanisms of JZN in lowering blood lipids remains to be clarified.
Figure 1. Structure of each advantage component. A: quercetin-3-O-β-d-glucuronide B: hyperoside C: emodin-8-O-β-d-glucoside D: rubrofusarin-6-O-β-d-gentiobioside E: 2-hydroxy-1-methoxyaporphine F: armepavine.
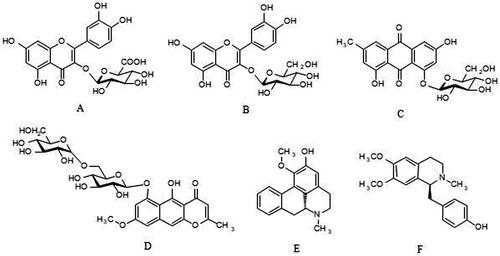
On the basis of a previous study (Zhang et al. Citation2021), the CAC and the proportion of advantageous components in JZN were determined in this study, and the pharmacodynamic substance basis and hypolipidemic mechanism of JZN were clarified. Our study provides a scientific basis for the establishment of quality control methods and the clinical application of JZN.
Materials and methods
Materials and reagents
Armepavine (batch no.: P22M10F88910), 2-hydroxy-1-methoxyaporphine (batch no.: M16N10S103163), hyperoside (batch no.: P14A11F121347), emodin-8-O-β-d-glucoside (batch no.: P17A10F86138), and sodium oleate (batch no.: J24M6Y1) were purchased from the Shanghai Yuanye Biotechnology Co., Ltd. (Shanghai, China). Quercetin 3-O-β-d-glucuronide (batch no.: Must-20070805) was purchased from the Chengdu Must Biotechnology Co., Ltd. (Chengdu, China). Rubrofusarin-6-O-β-d-gentiobioside (batch no.: 16120621) was purchased from the Shanghai Tauto Biotech Co., Ltd. (Shanghai, China). The purity of all standards was demonstrated to exceed 98.0% by HPLC analysis. Chloromethane, isopropyl alcohol and anhydrous ethanol were all from the Beijing Chemical Plant Co., Ltd. (Beijing, China) and were pure for analysis.
All herbs used in this study were commercially available dry matter. Crataegus pinnatifida (Hebei, batch no.: 20160315), Cassia obtusifolia (Anhui, batch no.: 20160501), Nelumbo nucifera (Hebei, batch no.: 20160301) and Polygonum multiflorum (Hubei, batch no.: 20160401) were purchased from the Anguo Shengtai Medicinal Materials Co. Ltd. (Hebei, China). All samples were authenticated by Professor Yuan Zhang (College of Traditional Chinese Medicine, Beijing University of Chinese Medicine). JZN granules (batch no.: 150812) were purchased from the Beijing Tongrentang (Group) Co., Ltd., and simvastatin (batch no.: P03869) was purchased from the Beijing Huawei Ruike Chemical Company.
Animals and experimental protocol
Four-week-old male ICR mice weighing 18–22 g were purchased from the SiBeiFu Biotechnology Co., Ltd. (Beijing, China) (Certificate No: SCXK (Jing) 2019-0010). The mice were maintained at 25–30 °C, 50–70% relative humidity and a 12 h light/dark cycle with free access to food and water. All animal experiments were conducted in accordance with the ethical standards of the Animal Care and Welfare Committee of Beijing University of Chinese Medicine (Certificate number: BUCM-04-2018030102-1013).
Cell culture
HepG2 cells were purchased from the Cell Resource Center (Beijing, China) and cultured in RPMI-1640 (Thermo Corporation, Waltham, MA) supplemented with 10% FBS and antibiotics (100 units/mL penicillin and 100 μg/mL streptomycin) (Thermo Corporation) in an incubator at 37 °C in humidified 5% CO2.
Preparation of the HEE of JZN
According to the formula ratio of JZN, 500 g of Crataegus pinnatifida and 25 g of Cassia obtusifolia were weighed and extracted in distilled water twice (2 h each time, the amount of the solvent was seven times the total weight of herbs), and then the extract was evaporated into paste. Meanwhile, 75 g of Nelumbo nucifera and 25 g of Polygonum multiflorum were weighed, mixed together, added to a volume of 50% ethanol (25 times) to reflux for 1.5 h, and filtered, and then the residue was refluxed in the same way twice. The ethanol extract was mixed and evaporated into a paste. The two pastes above were mixed and suspended in 5000 mL of distilled water. An AB-8 macroporous adsorption resin column (with a diameter-to-height ratio of 1:7) was used for enrichment and separation. After the adsorption was completed, the resin column was eluted with distilled water and 50% ethanol, and the 50% ethanol eluate was collected and dried under vacuum conditions. Dryness was proven to be responsible for the efficacy of JZN, and it was the HEE of JZN.
Ratio optimization of the hypolipidemic CAC of JZN
Selection of the mixed-level uniform design table
The minimum concentration at which the six advantageous components exerted hypolipidemic activity was taken as the initial concentration, and the IC50 value of the six advantageous components in inhibiting TG contents in HepG2 cells was taken as the intermediate concentration. The concentration levels of each component were uniformly designed within the safe concentration range of each advantageous component (Huang et al. Citation2016; Zhang et al. Citation2021; Chen et al. Citation2023). As the intermediate concentration of armepavine was close to the maximum safe concentration, a uniform design experiment at the optimized mixing level was chosen. The uniform design table of the optimal mixing level (Sun et al. Citation2015) was constructed using DPS software (Version 9.01, China). The advantageous components were combined into 12 combinations according to the concentration shown in .
Table 1. Concentration level table of each combination.
Establishment of hyperlipidemia model in HepG2 cells and determination of the TG content
HepG2 cells were placed in 6-well plates (1 × 105 cells/well) and incubated in 5% CO2 and 37 °C incubators for 24 h. The cells were induced by sodium oleate (SO 70 μg/mL) and cultured for 24 h. Then, the cells were divided into the control group, administration group, model group (SO 70 μg/mL) and sim group (35 μmol/mL). Each administration group was treated with 2 mL of drug-containing medium at different concentrations and incubated for 24 h. At the end of treatment, the cells were treated with Triton X-100 lysate and then centrifuged at 1000 rpm for 10 min at 4 °C, and the TG contents were determined with an assay kit (Nanjing Jiancheng Bioengineering Institute, Nanjing, China) according to the manufacturer’s instructions.
Data analysis
The effect of each combination on the TG content in lipid-laden HepG2 cells was transformed into the inhibition rate of each combination on the TG content according to the formula:
(1)
(1)
The raw data and inhibition rate of each experiment were input into DPS 9.01 software, and the input data were defined as data matrix blocks. Then, quadratic polynomial stepwise regression analysis was performed.
Bioequivalence analysis in vitro
The control group, administration group, model group (SO) and sim group were set. Each administration group was treated with 2 mL of JZN granules and the HEE of JZN, and incubated for 24 h. The method was described above in “Establishment of hyperlipidemia model in HepG2 cells and determination of the TG content”.
Bioequivalence analysis in vivo
Establishment of hyperlipidemia model in mice
Four-week-old male ICR mice weighing 18–22 g were randomly divided into 12 groups, with 6 mice in each group, including the control group, hyperlipidemic model group, sim group (20 mg/kg), low, middle and high dose of JZN granules (2, 4, 8 g/kg), low, middle and high dose of the HEE of JZN (120, 240, 480 mg/kg), and low, middle and high dose of the CAC groups (20, 40, 160 mg/kg). All groups were fed a standard chow diet. Triton WR-1339 model solution (400 mg/kg) was intraperitoneally injected into all mice in the treated groups after 12 h of fasting to induce a hyperlipidemic model, and mice in the normal control group were intraperitoneally injected with an equal volume of saline. Then, the TG, TC and LDL-C contents in the serum of model mice were significantly higher than those of the control mice (p < 0.01), and the HDL-C content in the serum of model mice was significantly lower than that of the control mice (p < 0.01), indicating that the hyperlipidemia mice were successfully modelled.
Determination of lipid indices
After intraperitoneal injection with Triton WR-1339, all groups were orally administered the relevant drugs, and the control group and hyperlipidemic model group were given an equal volume of 0.2% CMC-Na solution.
After 24 h of oral administration, mice were anesthetized with ether, blood samples were collected into centrifuge tubes from the fosse orbital vein and centrifuged, and the supernatant was collected for testing. The concentrations of TC, TG, HDL-C and LDL-C in the serum of mice were measured with a commercially available detection kit (Nanjing Jiancheng Institute of Biological Engineering, Nanjing, China) according to the manufacturer’s instructions.
Assessment of bioequivalence
According to the above Formula 1 in “Data analysis”, the content determination results of TC, TG, HDL-C and LDL-C in animal experiments were converted into the inhibition rates of TC, TG and LDL-C and the promotion rate of HDL-C of each drug (i.e., efficacy value), and the content determination results of TG in cell experiments were converted into the inhibition rate of TG of each drug (i.e., efficacy value).
(1)
(1)
Bioequivalence was evaluated by calculating the 95% confidence interval of the ratio between the inhibition rates of the two drugs. If the 95% confidence interval of relative efficacy fell within the range from 70% to 140%, the two drugs were considered to be bioequivalent (Karalis et al. Citation2012; Liu et al. Citation2014).
Transcriptome sequencing analysis
Total RNA extraction, library construction and transcriptome sequencing
Total RNA of the high-fat cell model (SO) and the “bioequivalent substance system” administration (N) groups was isolated using TRIzol reagent according to the manufacturer’s protocol, and those groups were treated with DNase I reagent. The integrity and purity of total RNA were assessed. The cDNA libraries were constructed for transcriptome sequencing on Illumina HiSeq. To obtain clean reads, the raw reads were filtered by removing reads containing adapters, reads containing more than 5% N and low-quality reads with a basic mass value of Q ≤ 5. Then, all clean reads were mapped to the reference sequence with HISAT2 software, and the FRKM (expected number of fragments per kilobase of transcript sequence per million base pairs sequenced) was calculated to obtain gene expression levels for each sample. In this experiment, the correlation coefficient was greater than 0.8 for the samples of biological replicates. On the basis of the gene expression level FPKM, the differentially expressed genes between the two samples in the experiment were defined as follows: the absolute value of log2 (fold change) between two samples was greater than 1, and the value of false discovery rate (FDR) was less than or equal to 0.001. A volcano plot was used to visually represent the overall differential expression distribution of genes. Gene Ontology (GO) and Kyoto Encyclopedia of Genes and Genomes (KEGG) pathway enrichment analyses of differentially expressed genes (DEGs) were implemented by R software (clusterProfiler).
Transcriptome validation of DEGs by qRT–PCR
The RNA of the SO and N groups, which was used for quantitative analysis of DEG expression, was prepared in the same way as for transcriptome sequencing. Then, the concentration, purity and integrity of the RNA were determined. Total RNA solution was used as a template for reverse transcription into cDNA. qRT–PCR was performed using a Roche Light Cycler® 480II Real-time PCR system and the following primer sequences ().
Table 2. Primer sequence table.
Statistical analysis
SPSS 22.0 software was used for statistical analysis of experimental data, which are expressed as the mean ± standard deviation ( ± s). Statistical analysis was performed using univariate ANNOVA. GraphPad Prism 8.0 software was used for graphic rendering. The model group data are normalized. p < 0.05 was considered to indicate a statistically significant difference.
Results
Pharmacodynamic analysis of the hypolipidemic CAC of JZN based on uniform design
Ratio optimization of the hypolipidemic CAC of JZN
The effects of different combinations on the TG content in lipid-laden HepG2 cells were used to evaluate the hypolipidemic activity of each combination. The specific results are shown in . Quadratic polynomial regression analysis was performed to obtain the regression equation as follows: Y = 45.0809381 + 0.18444509496×1 − 0.8972380513×5 + 0.011237521113×5*X5 − 0.0026104358199×6*X6 − 0.0009251838990×1*X2 − 0.0010537014802×1*X4 + 0.0013152148192×2*X4 + 0.0005468619825×4*X6 + 0.003437354688×5*X6, R2 = 0.9999, significance level p = 0.0008. The Durbin–Watson statistic of this equation is 1.7106, R > Ra, indicating that the Y regression effect is significant. The results of the data processing are shown in and .
Figure 2. Effect of compositions N1-N12 on TG content in lipid-laden HepG2 cells (n = 5). Compared with SO group, *p < 0.05, **p < 0.01.
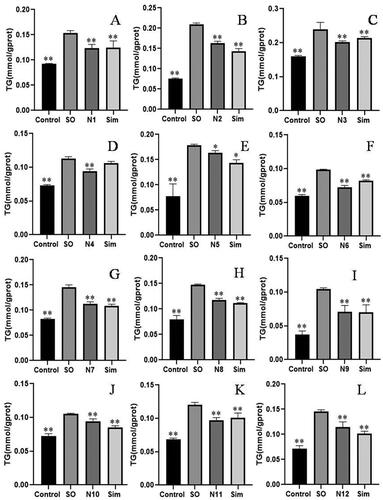
Figure 3. Interaction diagram of various factors. A-X1*X4, B-X2*X4, C-X1*X2, D-X5*X6, E-X4*X6, X1: quercetin-3-O-β-d-glucuronide, X2: hyperoside, X3: rubrofusarin-6-O-β-d-gentiobioside, X4: emodin-8-O-β-d-glucoside, X5: 2-hydroxy-1-methoxyaporphine, X6: armepavine.
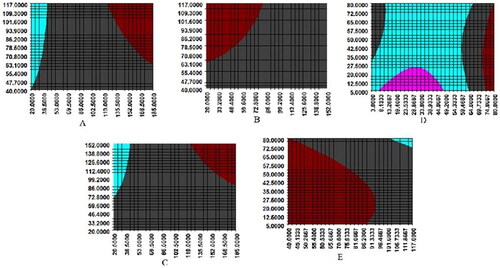
Table 3. Data processing results.
According to the data shown in , the hypolipidemic activity of the combination N6 was the most significant, and its inhibition rate of the TG content in lipid-laden HepG2 cells was the highest (66.15%). Then, the optimal proportion of each advantageous component in the combination was obtained when the Y value reached the maximum. The results showed that the maximum inhibition rate of the advantageous combination was 77.21%, and the best proportion was as follows: quercetin-3-O-β-d-glucuronide: hyperoside: rubrofusarin-6-O-β-d-gentiobioside: emodin-8-O-β-d-glucoside: 2-hydroxy-1-methoxyaporphine: armepavine = 9.32:1:4.45:1.85:2.41:1.92.
Table 4. Inhibitory rate of each combination on TG content in lipid-laden HepG2 cells.
Efficacy verification of the CAC
The efficacy of the CAC, which was composed of six advantageous components with the proportion stated above in “Ratio optimization of the hypolipidemic CAC of JZN” (named Nz), was verified on the TG content in lipid-laden HepG2 cells. The TG content was significantly inhibited with Nz treatment in HepG2 cells, and the inhibition rate was 74.03% (), which was close to the maximum Y (77.21%).
Figure 4. Effect of Nz on TG content in lipid-laden HepG2 cells (n = 5). Influence of each administration group on the TG content in lipid-laden HepG2 cells (n = 5). SO: hyperlipidemic model group Nz: CAC administration group. G: JZN granules group HEE: HEE of JZN group. Compared with the model group, *p < 0.05, **p < 0.01.
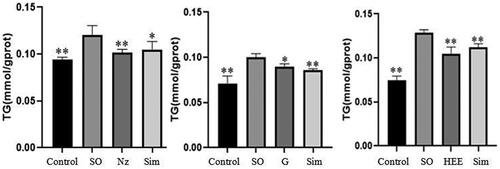
Bioequivalence analysis in vivo
Effect of each administration group on the TC content in the serum of hyperlipidemia mice
As shown in , the TC content in the serum of model mice was significantly higher than that of control mice. JZN granules, the HEE of JZN and the CAC had inhibitory effects on the TC content in the serum of high-fat mice. Compared with the model group (SO), the inhibition rates of the low-, middle- and high-dose JZN granule groups, the low-, middle- and high-dose HEE JZN groups, and the low-, middle- and high-dose CAC groups on the TC content in the serum of high-fat mice were 45.32, 24.82, 49.08, 30.46, 74.05, 59.95, 11.40, 45.29 and 61.86%, respectively, wherein the low-, and high-dose JZN granule groups (p < 0.01, p < 0.01), the middle-, and high-dose HEE of JZN groups (p < 0.01, p < 0.01), and the low-, middle- and high-dose CAC groups (p < 0.05, p < 0.01, p < 0.01) significantly reduced the TC content in the serum of hyperlipidemia mice.
Effect of each administration group on the TG content in the serum of hyperlipidemia mice
As shown in , the TG content in the serum of model mice was significantly higher than that of control mice (p < 0.01). Compared with the model group, the inhibition rates of the low-, middle- and high-dose JZN granule groups, the low-, middle- and high-dose HEE of JZN groups, and the low-, middle- and high-dose CAC groups on the TG content in the serum of high-fat mice were 34.48, 56.70, 65.61, 53.11, 63.56, 85.26, 55.20, 83.21 and 105.54%, respectively, wherein the low-, middle- and high-dose JZN granule groups (p < 0.05, p < 0.01, p < 0.01), the low-, middle- and high-dose HEE of JZN groups (p < 0.01, p < 0.01, p < 0.01), and the low-, middle- and high-dose CAC groups (p < 0.01, p < 0.01, p < 0.01) significantly reduced the TG content in the serum of hyperlipidemic mice.
Effect of each administration group on the LDL-C content in the serum of hyperlipidemia mice
As shown in , the LDL-C content in the serum of model mice was significantly higher than that of control mice. Compared with the model group, the inhibition rates of the low-, middle- and high-dose JZN granule groups, the low-, middle- and high-dose HEE of JZN groups, and the low-, middle- and high-dose CAC groups on the LDL-C content in the serum of high-fat mice were 10.71, 28.32, 38.09, 53.50, 30.91, 29.01, 29.39, 36.57, 39.38%, respectively, wherein the middle-, and high-dose JZN granule groups (p < 0.01, p < 0.01), the low-, middle- and high-dose HEE of JZN groups (p < 0.01, p < 0.01, p < 0.01), and the low-, middle- and high-dose CAC groups (p < 0.01, p < 0.01, p < 0.01) significantly reduced the LDL-C content in the serum of hyperlipidemia mice.
Effect of each administration group on the HDL-C content in the serum of hyperlipidemia mice
As shown in , the HDL-C content in the serum of model mice was significantly lower than that of control mice (p < 0.01). Compared with the model group, the increased rates of the low-, middle- and high-dose JZN granule groups, the low-, middle- and high-dose HEE of JZN groups, and the low-, middle- and high-dose CAC groups on the HDL-C content in the serum of high-fat mice were 0, 85.34, 88.54, 87.66, 112.95, 166.72, 95.12, 94.65 and 232.67%, respectively, wherein the middle-, and high-dose JZN granule groups (p < 0.05, p < 0.05), the low-, middle- and high-dose HEE of JZN groups (p < 0.05, p < 0.01, p < 0.01), and the low-, middle- and high-dose CAC groups (p < 0.05, p < 0.05, p < 0.01) significantly increased the HDL-C content in the serum of hyperlipidemia mice.
Bioequivalence analysis
The bioequivalence analysis was conducted with the high-dose group of each drug as the main indicator, and the results are shown in .
Table 5. Bioequivalence analysis of CAC, JZN granules and HEE of JZN in vivo.
CAC and JZN granules
As shown in , within the 90% confidence interval, the CAC was as effective as JZN granules in reducing the TC and LDL-C contents in the serum of hyperlipidemia mice, and the two were bioequivalent. At the same time, combined with the analysis of the data in , it can be seen that the efficacy of CAC in decreasing the TG content and increasing the HDL-C content in the serum of hyperlipidemic mice is superior to that of JZN granules, and the bioequivalence relationship between the two is not significant.
CAC and HEE of JZN
In the 90% confidence interval, the efficacy of the CAC in reducing the TC and TG contents in the serum of hyperlipidemia mice was comparable to that of the HEE of JZN, and the two were bioequivalent. Then, the efficacy of the CAC in reducing the LDL-C content and increasing the HDL-C content in the serum of hyperlipidemia mice was significantly better than that of the HEE of JZN, and the bioequivalence relationship between them was not significant.
Bioequivalence analysis in vitro
Effects of each administration group on the TG content in lipid-laden HepG2 cells
The results shown in illustrate that JZN granules and the HEE of JZN can inhibit the TG content in lipid-laden HepG2 cells, and the differences were significant when compared to the model group.
Bioequivalence analysis
According to the hypolipidemic activity results of CAC above in “Efficacy verification of the CAC”, the 90% confidence interval of the relative efficacy value of JZN granules and the HEE of JZN was calculated. The results in show that the 90% confidence intervals of the relative efficacy of the CAC and JZN granules fell outside the range from 70% to 143%, which, combined with the analysis of the data in , indicated that the inhibition rate of the CAC on the TG content in the lipid-laden HepG2 cells was significantly higher than that of JZN granules, and the hypolipidemic activity of the CAC was better. The 90% confidence interval of the relative efficacy of the CAC and the HEE of JZN only partially fell within the range from 70% to 143%, indicating that the bioequivalence relationship between the CAC and the HEE of JZN was not significant, and the hypolipidemic activity of the CAC was significantly better than that of the HEE of JZN.
Table 6. Bioequivalence analysis of CAC, JZN granules and HEE of JZN in vitro.
Transcriptome sequencing analysis
Transcriptome sequencing and gene expression analysis
To confirm the hypolipidemic mechanisms of the “BSS”, we performed six cDNA libraries: SO (SO-1, SO-2 and SO-3) and N (N-1, N-2 and N-3) groups and sequenced them on the Illumina HiSeq platform.
The FPKM value of gene expression was used to calculate the correlation between three biological replicates in the SO and N groups. The results showed that the squares of Pearson’s coefficients were all higher than 0.95, indicating that the repeatability of the three groups of samples was good and that the transcriptome data obtained in this experiment can accurately and reliably display the situation of differentially expressed genes.
Identification of DEGs
The Possion Dis algorithm was used to identify DEGs with a false discovery rate (FDR) ≤ 0.001 and |log2 (fold change)| > 1 in the high-fat model group of HepG2 cells treated with the “BSS” of JZN. Then, 164 DEGs were defined in the “BSS” administration vs. SO groups, containing 43 up-regulated genes and 121 down-regulated genes. The volcano plot and MA plot analysis of DEGs are shown in .
Figure 6. Volcano plot of the differentially expressed genes. Genes upregulated by “bioequivalent substance system” of JZN are shown in red, those downregulated by “bioequivalent substance system” of JZN are in green. (A) was Volcano plot of the differentially expressed genes. Genes upregulated by “bioequivalent substance system” of JZN are shown in red, those downregulated by “bioequivalent substance system” of JZN are in green. (B) was GO analysis of DEGs. A: Biology process B: Molecular function C: Cellular component. (C) was KEGG analysis of DEGs. .
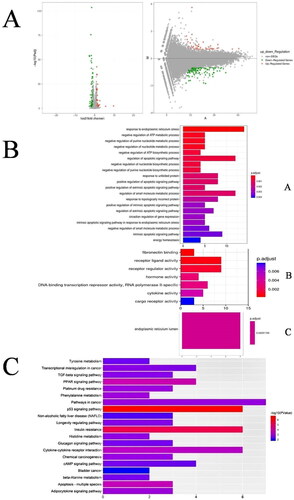
GO and KEGG enrichment analysis
To investigate the biological functions of DEGs after intervention with the hypolipidemic “BSS” of JZN in lipid-laden HepG2 cells, the DEGs were analyzed by GO and KEGG. In the GO enrichment analysis, 137 DEGs, including 129 biological processes, 7 molecular functions and 1 cellular component, were obtained. Ordered by the p value, the top 20 items in each module were selected. The results of GO enrichment are shown in . In addition, KEGG enrichment analysis showed that 164 differentially expressed genes were involved in 121 pathways. The results were sorted according to the p value from small to large, and the top 20 pathways were selected for mapping, as shown in . The enrichment pathways related to the lipid-lowering effect included the PPAR signalling pathway (Ppara, Olr1 and Acsbg1), bile secretion pathway (Ldlr and Abcc3), nonalcoholic fatty liver disease pathway (Bid, Traf2 and Ppara) and adipocytokine signalling pathway (Ppara, Acsbg1 and Ppargc1a). These four pathways are shown in .
The pathways related to hypolipidemia
PPAR signalling pathway
The DEGs involved in this signalling pathway were Ppara, Olr1 and Acsbg1. After intervention with the “BSS” in lipid-laden HepG2 cells, the expression of the Olr1 gene in the PPAR signalling pathway was down-regulated, and the expression of the Ppara and Acsbg1 genes was up-regulated. The hypolipidemic mechanism of the “BSS” may be concluded as follows: (1) the expression of the Olr1 gene in the PPAR signalling pathway was down-regulated, then the uptake of free fatty acids and the content of TG was reduced (Chui et al. Citation2005; Guo et al. Citation2019; Qiao et al. Citation2020), thereby exerting a hypolipidemic effect and delaying the progression of atherosclerosis; (2) up-regulation of Acsbg1 gene expression promoted the β-oxidation of fatty acids and provided energy to the body, while reducing the accumulation of fatty acids and lipid production to regulate lipid metabolism (Steinberg et al. Citation2000; Pei et al. Citation2003; Qing et al. Citation2018) and exert a hypolipidemic effect and (3) up-regulation of the expression of the Ppara gene promoted β-oxidation of fatty acids and cholesterol metabolism, exerting a hypolipidemic effect by reducing the content of cholesterol and fatty acids.
Nonalcoholic fatty liver disease pathway
Nonalcoholic fatty liver disease (NAFLD) is a spectrum of diseases caused by the accumulation of large amounts of fat in the liver, ranging from simple steatosis to more severe steatohepatitis with liver inflammation as well as fibrosis (called nonalcoholic steatohepatitis (NASH)). The hypolipidemic “BSS” of JZN could down-regulate the expression of Bid and Traf2 genes in the NAFLD pathway and up-regulate the expression of Ppara genes after intervention in lipid-laden HepG2 cells. The hypolipidemic mechanism may be as follows: (1) gene Bid was reduced, which inhibits the expression of downstream Casp3 and Casp7 genes and inhibits apoptosis, thereby reducing hepatocyte damage (Molpeceres et al. Citation2007); (2) down-regulation of the gene Traf2 could reduce the production of multiple downstream inflammatory factors, thereby alleviating the development of steatohepatitis and protecting the liver and (3) up-regulation of the expression of the Ppara gene reduced the content of fatty acids in the liver and alleviated the symptoms of NAFLD.
Bile secretion pathway
Bile is an important secretion for the digestion and absorption of fats and fat-soluble vitamins in the small intestine. In addition, bile is an important means of eliminating superfluous cholesterol and many waste products, bilirubin, drugs and toxic compounds. After intervention with the “BSS” in lipid-laden HepG2 cells, the expression of the Ldlr and Abcc3 genes in the bile secretion pathway was up-regulated. The hypolipidemic mechanism may be concluded as follows: (1) the expression of the Ldlr gene was increased, which simultaneously enhanced the binding to LDL-C and then promoted bile acid metabolism and accelerated the metabolism of cholesterol and (2) the gene Abcc3 was up-regulated, which alleviated the symptoms of cholestasis by removing excess bile acid from hepatocytes.
Adipocytokine signalling pathway
Adipose tissue has a broad secretion profile and can secrete a large number of cytokines called adipocytokines. Common cytokines include adiponectin (ADPN), leptin (LEP), TNFα, IL6 and angiogenin-like protein 4 (ANGPT4) (Gong et al. Citation2007). These factors play an important role in insulin signal transduction, lipid metabolism disorders and cardiovascular diseases. The hypolipidemic “BSS” of JZN can have a hypolipidemic effect by interfering with the adipocytokine signalling pathway after intervention in HepG2 cells. The mechanism may be concluded as follows: (1) the expression of the Traf2 gene was down-regulated, and the expression of downstream proteins, including mTOR, JNK and IKK, was reduced, thereby down-regulating the inhibition of IRS1 tyrosine phosphorylation by serine phosphorylation and reducing insulin resistance and (2) the Ppara and Ppargc1a genes were up-regulated, which up-regulated the expression of CPT1 protein, promoted β-oxidation of fatty acids and reduced lipid accumulation.
Confirmation of DEGs by qRT–PCR
To validate the expression of DEGs sourced from the transcriptome, five special DEGs that repeatedly recurred in several pathways were selected. After intervention with the hypolipidemic “BSS” of JZN, the expression of these genes was determined by qRT–PCR. The results () show that the expression of DEGs from qRT–PCR was consistent with the results of transcriptome sequencing, indicating that the transcriptome sequencing results were reliable.
Discussion
Hyperlipidemia is a pathological condition that when blood lipids increase, a large amount of lipid is deposited on the blood vessel wall, which can directly cause a series of diseases, especially atherosclerosis, that endanger the well-being of patients. TCM compound has unique advantages in the treatment of hyperlipidemia: abundant resources, definite curative effects, flexible formula, less adverse effects and a variety of lipid-lowering pathways, so it has a good application prospect (Zhang et al. Citation2015). The selection of research ideas and the application of experimental models are very diverse when studying the mechanism of hypolipidemic action of drugs.
In the present study, the research idea of the TCM compound “bioequivalent substance system” was proposed. Bioequivalence assessment was initially applied to determine whether two drugs are bioequivalent by calculating the 90% confidence interval range of the ratio of their pharmacodynamic parameters (Karalis et al. Citation2012). Subsequently, it was applied to the evaluation of the efficacy of TCM (compound) and combination of advantageous components which screened from TCM (compound). If the 90% confidence interval of relative efficacy fell within the range from 70% to 140%, the combination of advantageous components and the TCM compound was considered to be bioequivalent (Liu et al. Citation2014).
According to the research idea of TCM compound “bioequivalent substance system”, the ratio of the hypolipidemic CAC of JZN was determined. Then, a pharmacodynamic study of the CAC of JZN was carried out using animal experiments and cell experiments. In this study, Triton WR-1339 was used as an acute model for preliminary and rapid screening of hypolipidemic agents. The hyperlipidemia model induced by Triton WR-1339 could cause endogenous lipid disorders and liver inflammation in mice with a short modelling time (Schurr et al. Citation1972; Li et al. Citation2019; Eimad Dine et al. Citation2020). At the same time, compared with the control group, TC, TG and LDL-C levels in plasma of hyperlipidemia rats were significantly increased, HDL-C levels in plasma of hyperlipidemia rats were significantly decreased, which were consistent with the results reported previously (Eimad Dine et al. Citation2020). Our pharmacological data showed that CAC can significantly reduce the content of TG, TC and LDL-C in hyperlipidemia rats’ plasma, and the bioequivalence relationship between the CAC, JZN granules and the HEE of JZN was carried out using the contents of TC, TG and LDL-C in the serum of mice as indicators. The results confirmed that the three have a certain bioequivalent relationship. Second, in cell experiments, SO was used to induce a hyperlipidemia model of HepG2 cells, and the bioequivalence relationship between the CAC, JZN granules and the HEE of JZN was studied with the TG content as an index. The results showed that the hypolipidemic activity of the CAC was better than those of JZN granules and the HEE of JZN, and the three did not have an absolute bioequivalence relationship. There is no absolute bioequivalence according to the evaluation standard, so it is speculated that “BSS” has some advantages in lowering TG than JZN granules, perhaps because of the result after purification.
Meanwhile, the composition of chemical components of TCM compound was complex, the clinical therapeutic efficacy of TCM compounds was the result of the whole and synergistic effect of several active components, rather than the linear addition of a few chemical components (Zhang and Feng Citation2019). Nowadays, the concept of phytochemical synergy plays an important role in promoting human health and preventing hyperlipidemia (Liu et al. Citation2022). On the basis of the complexity of the regulatory network of lipid metabolism, synergistic therapy has shown to be superior to monotherapy (Efferth and Koch Citation2011). For example, a combination of quercetin, hyperoside, rutin and chlorogenic acid (6:9:2:1) could increase the inhibitory activity of HMG-CoA reductase by 58.9% (Gao et al. Citation2021). The mixture of quercetin and kaempferol (1:1) could improve LDL-C uptake more effectively in HepG2 cells (Yusof et al. Citation2018).
Therefore, in order to clarify the mechanism of TCM compounds, it is necessary to further analyze and study the synergistic effect among active components. In this study, in order to clarify how the six advantageous components, exert the hypolipidemic effects synergistically, further research and explanation from the aspects of the affinity between components and the synergism of pharmacodynamic characterization in vivo is needed. In addition, based on the previous research of our group, the six advantageous components could all enter the blood as prototypes, and there are also aglycone metabolites and aglycone secondary metabolites of glycoside components such as quercetin-3-O-β-d-glucuronide, hyperoside, etc. (Zhang et al. Citation2021).
Despite the advances, our study had several limitations, therefore, it is necessary to further investigate the effects of glycogen metabolites of advantageous components on the hypolipidemic in combination with other methods such as metabolomics and proteomics approaches.
Conclusions
A systematic pharmacodynamic study and evaluation of the hypolipidemic “bioequivalent substance system” of JZN was carried out, and finally the substance basis of JZN lowering blood lipid was initially clarified. Together, these findings provided new feasible research ideas and methods for the elucidation of the pharmacodynamic material basis and the study of the mechanism of natural medicine with complex components.
Author contributions
Yu-miao Li: Conceptualization, Methodology, Investigation, Software. Yan Zhang: Data curation, Investigation. Yu Zhang: Investigation, Writing-Original draft preparation. Tian-feng Lin: Formal analysis, Supervision. Yan-yan Gao: Data curation, Formal analysis. Yuan Cai, Chang Zhou and Le-Yi Yang: Writing- Reviewing and Editing. Bin Liu: Project administration. Shi-fen Dong and Yan-yan Jiang: Project administration, designing the study.
Disclosure statement
No potential conflict of interest was reported by the authors.
Additional information
Funding
References
- Ahamad S, Bhat SA. 2022. Recent update on the development of PCSK9 inhibitors for hypercholesterolemia treatment. J Med Chem. 65(23):15513–15539. doi: 10.1021/acs.jmedchem.2c01290.
- Chen LB, Ren LB, Yan HF, Deng CQ. 2023. [Effect of dose compatibility of main chemical components from Astragali Radix-Angelicae Sinensis Radix on proliferation of VSMCs based on uniform design]. Chin J Exp Tradit Med Form. 29:143–151.
- Chen JX, Zhao HH, Ma XL, Han X, Luo LT, Wang LY, Han J, Liu B, Wang W. 2012. The effects of Jiang-Zhi-Ning and its main components on cholesterol metabolism. Evid Based Complement Alternat Med. 2012:928234. doi: 10.1155/2012/928234.
- Chen JX, Zhao HH, Yang Y, Liu B, Ni J, Wang W. 2011. Lipid-lowering and antioxidant activities of Jiang-Zhi-Ning in traditional Chinese medicine. J Ethnopharmacol. 134(3):919–930. doi: 10.1016/j.jep.2011.01.048.
- Chinese Pharmacopoeia Commission. 2020. Pharmacopoeia of the People’s Republic of China (Vol I). Beijing: China Medical Science Press.
- Chui PC, Guan HP, Lehrke M, Lazar MA. 2005. PPARγ regulates adipocyte cholesterol metabolism via oxidized LDL receptor 1. J Clin Invest. 115(8):2244–2256. doi: 10.1172/JCI24130.
- Efferth T, Koch E. 2011. Complex interactions between phytochemicals. The multi-target therapeutic concept of phytotherapy. Curr Drug Targets. 12(1):122–132. doi: 10.2174/138945011793591626.
- Eimad Dine TB, Abdelbasset H, Bouchra B, Tarik K, Hicham H, Younes FZ, Chakib A. 2020. Effect of Phoenix dactylifera seeds (dates) extract in Triton wr-1339 and high fat diet induced hyperlipidaemia in rats: a comparison with simvastatin. J Ethnopharmacol. 259:112961.
- Gao J, Zhang MQ, Niu RX, Gu X, Hao EW, Hou XT, Deng JG, Bai G. 2021. The combination of cinnamaldehyde and kaempferol ameliorates glucose and lipid metabolism disorders by enhancing lipid metabolism via AMPK activation. J Funct Foods. 83:104556. doi: 10.1016/j.jff.2021.104556.
- Gao L, Zhou WJ, Ma YM, Wang YH. 2013. [Effects of ethanol extraction from Cassiae semen on leptin and NPY in hyperlipidemia rats]. Chin J Exp Tradit Med Form. 19:235–238.
- Gong DW, Li P, Zhu DL. 2007. [Research progress of adipocytokines]. J Diabetes. 15:637–638.
- Guo HF, Ning Y, Cheng G, Zan LS. 2019. [The effect of Krüppel-Like Factor 3 (KLF3) gene on bovine fat deposition]. Sci Agric Sin. 52:1272–1281.
- Hao PP, Jiang F, Cheng J, Ma LY, Zhang Y, Zhao YX. 2017. Traditional Chinese medicine for cardiovascular disease: evidence and potential mechanisms. J Am Coll Cardiol. 69(24):2952–2966. doi: 10.1016/j.jacc.2017.04.041.
- Huang JF, Li XY, Huang XQ, Wu TT, Xu W, Wu SS. 2016. [Research on effective components compatibility of Alismatis Rhizoma resisting calcium oxalate calculus in vitro based on uniform design]. Chin Tradit Herbal Drugs. 47:4160–4165.
- Karalis V, Symillides M, Macheras P. 2012. Bioequivalence of highly variable drugs: a comparison of the newly proposed regulatory approaches by FDA and EMA. Pharm Res. 29(4):1066–1077. doi: 10.1007/s11095-011-0651-y.
- Kwok CY, Li C, Cheng HL, Ng YF, Chan TY, Kwan YW, Leung GPH, Lee SMY, Mok DKW, Yu PHF, et al. 2013. Cholesterol lowering and vascular protective effects of ethanolic extract of dried fruit of Crataegus pinnatifida, hawthorn (ShanZha), in diet-induced hypercholesterolaemic rat model. J Funct Foods. 5(3):1326–1335. doi: 10.1016/j.jff.2013.04.020.
- Li YT, Cai DK, Zhan XY, Zheng LY, Yang JM, Huang XJ, Huang DE, Chen YX, Gan HN. 2019. Lipid metabolism and inflammatory changes in mice with hyperlipidemia induced by triton wr-1339. J Guangdong Pharmaceut Univ. 35:793–797.
- Li MM, Huang XY, Liang QK, Li YX, Yan Y, Tian ZF, Qiu XQ, Zhang XW, Song Q, Li HF. 2017. [Effects and mechanisms of lotus leaf water extract on lipid metabolism of adult experimental obesity rats]. Chin J Physiol. 33:476–480.
- Li CH, Li X, Fang KY, Guo BJ. 2008. Effects of anthraquinones from Cassia obtusifolia L. on cholesterol biosynthesis in cells. J Clin Rehabil Tis Engin Res. 12:6593–6596.
- Lin Y, Vermeer MA, Trautwein EA. 2011. Triterpenic acids present in hawthorn lower plasma cholesterol by inhibiting intestinal ACAT activity in hamsters. Evid Based Complement Alternat Med. 2011:801272. doi: 10.1093/ecam/nep007.
- Liu Y, Liu C, Kou X, Wang Y, Yu Y, Zhen N, Jiang J, Zhaxi P, Xue Z. 2022. Synergistic hypolipidemic effects and mechanisms of phytochemicals: a Review. Foods. 11:2774. doi: 10.3390/foods11182774.
- Liu P, Yang H, Long F, Hao H-P, Xu X, Liu Y, Shi X-W, Zhang D-D, Zheng H-C, Wen Q-Y, et al. 2014. Bioactive equivalence of combinatorial components identified in screening of an herbal medicine. Pharm Res. 31(7):1788–1800. doi: 10.1007/s11095-013-1283-1.
- Molpeceres V, Mauriz JL, Garcia-Mediavilla MV, Gonzalez P, Barrio JP, Gallego JG. 2007. Melatonin is able to reduce the apoptotic liver changes induced by aging via inhibition of the intrinsic pathway of apoptosis. J Gerontol A Biol Sci Med Sci. 62(7):687–695. doi: 10.1093/gerona/62.7.687.
- Niu CS, Chen CT, Chen LJ, Cheng KC, Yeh CH, Cheng JT. 2011. Decrease of blood lipids induced by Shan-Zha (fruit of Crataegus pinnatifida) is mainly related to an increase of PPAR α in liver of mice fed high-fat diet. Horm Metab Res. 43(9):625–630. doi: 10.1055/s-0031-1283147.
- Pei ZT, Oey NA, Zuidervaart MM, Jia ZZ, Li YY, Steinberg SJ, Smith KD, Watkins PA. 2003. The Acyl-CoA Synthetase “Bubblegum” (Lipidosin): further characterization and role in neuronal fatty acid-oxidation. J Biol Chem. 278(47):47070–47078. doi: 10.1074/jbc.M310075200.
- Qiao M, Huang CY, Wu JJ, Wu HY, Wan XL, Zhou JW, Liu GS, Mei SQ, Peng XW. 2020. [Effect of porcine OLR1 gene on intramuscular preadipocytes differentiation]. Acta Agric Zhejiangensis. 32:2147–2153.
- Qing XD, Zeng D, Wang HS, Ni XQ, Lai J, Liu L, Khalique A, Pan KC, Jing B. 2018. Analysis of hepatic transcriptome demonstrates altered lipid metabolism following Lactobacillus johnsonii BS15 prevention in chickens with subclinical necrotic enteritis. Lipids Health Dis. 17(1):93. doi: 10.1186/s12944-018-0741-5.
- Rosenson RS, Burgess LJ, Ebenbichler CF, Baum SJ, Stroes ESG, Ali S, Khilla N, Hamlin R, Pordy R, Dong YP, et al. 2020. Evinacumab in patients with refractory hypercholesterolemia. N Engl J Med. 383(24):2307–2319. doi: 10.1056/NEJMoa2031049.
- Schurr PE, Schultz JR, Parkinson TM. 1972. Triton-induced hyperlipidemia in rats as an animal model for screening hypolipidemic drugs. Lipids. 7(1):68–74. doi: 10.1007/BF02531272.
- Shakir A, Shintu M, Waqas AK, Kishor M. 2022. Development of small-molecule PCSK9 inhibitors for the treatment of hypercholesterolemia. Drug Discov Today. 27:1332–1349.
- Steinberg SJ, Morgenthaler J, Heinzer AK, Smith KD, Watkins PA. 2000. Very long-chain Acyl-CoA synthetases: human “Bubblegun” represents a new family of proteins capable of activating very long-chain fatty acids. J Biol Chem. 275(45):35162–35169. doi: 10.1074/jbc.M006403200.
- Sun DN, Wang QQ, Wang C, Liu CF, Liu WW, Lin N. 2015. [Expectorant and antitussive effects of combined administration of Trichosanthis fructus and Aconiti Radix Cocta based on uniform design]. Chin J Exp Tradit Med Form. 21:55–59.
- Wang YH, Gao L, Zhou WJ, Ma YM. 2014. [Effects of ethanol extraction from Cassiae semen on serum IL-6 and TNF-α in hyperlipidemia rats]. Chin J Exp Tradit Med Form. 20:178–181.
- Yusof HM, Sarah NML, Lam TW, Kassim MNI. 2018. Hypolipidemic effects of quercetin and kaempferol in human hepatocellular carcinoma (HepG2) cells. Int Food Res J. 25:241–245.
- Zhang QQ, Feng F. 2019. The effects of different varieties of Aurantii Fructus Immaturus on the potential toxicity of Zhi-Zi-Hou-Po decoction based on spectrum-toxicity correlation analysis. Molecules. 24: 4254. doi: 10.3390/molecules24234254.
- Zhang HJ, Liu YN, Wang M, Wang YF, Deng YR, Cui ML, Ren XL, Qi AD. 2015. One-pot β-cyclodextrin-assisted extraction of active ingredients from Xue-Zhi-Ning basing its encapsulated ability. Carbohydr Polym. 132:437–443. doi: 10.1016/j.carbpol.2015.06.072.
- Zhang Y, Li LH, Zhang JH, Lin TF, Jiang YY, Lin B. 2021. Screening of hypolipidemic active components in Jiang-Zhi-Ning and its preliminary mechanism research based on "active contribution value" study. J Ethnopharmacol. 272:113926. doi: 10.1016/j.jep.2021.113926.
- Zhang L, Wang SJ, Wang HS, Yang CF, Lai CC, Li MJ, Li X. 2019. [Study on the compatibility of effective components of Polygonum multiflorum on regulating cholesterol metabolism]. Lishizhen Med Mater Med Res. 30:37–39.
- Zhu JR, Gao RL, Zhao SP, Lu GP, Zhao D, Li JJ. 2016. Chinese guidelines for the prevention and treatment of dyslipidemia in adults (2016 revision). Chin Circ J. 31:937–953.
- Zuo YM, Wu Q, Li X, Pan MX, Liu GQ, Pan W. 2017. [Determination of three constituents in Polygoni multiflori Radix Praeparata in plasma of atherosclerosis rats]. Chin Tradit Patent Med. 39:116–120.