Abstract
Context
Zengye decoction (ZYD) has been considered to have a curative effect on Sjogren’s syndrome (SS). However, its therapeutic mechanisms remain obscure.
Objectives
This research explores the mechanisms of ZYD against SS.
Materials and methods
The active compounds and targets of ZYD were searched in the TCMSP and BATMAN-TCM databases. SS-related targets were obtained from the GeneCards database. The GO and KEGG enrichment analyses elucidated the molecular mechanisms. Animal experiments were performed using 8 C57BL/6 mice that served as the control group (physiological saline treatment) and 16 NOD mice randomly divided into the model group (physiological saline treatment) and the ZYD group (ZYD treatment) for 8 weeks to verify the therapeutic effects of ZYD on SS.
Results
Twenty-nine active compounds with 313 targets of ZYD and 1038 SS-related targets were screened. Thirty-two common targets were identified. β-Sitosterol and stigmasterol might be important components. GO analysis suggested that the action of ZYD against SS mainly involved oxidative stress, apoptotic processes, and tumor necrosis factor receptor superfamily binding, etc. KEGG analysis indicated the most significant signaling pathway was apoptosis-multiple species. Animal experiments showed that ZYD improved lymphocytic infiltration of the submandibular glands (SMGs), reduced the serum levels of TNF-α, IL-1β, IL-6, and IL-17, upregulated the expression of Bcl-2, and downregulated the expression of Bax and Caspase-3 in the model mice.
Discussion and conclusion
ZYD has anti-inflammatory and anti-apoptotic effects on SS, which provides a theoretical basis for the treatment of SS with ZYD.
Introduction
Sjogren’s syndrome (SS), a systemic autoimmune disorder, mainly results the destruction and dysfunction of the secretory glands due to lymphocytic infiltration of the exocrine glands. The systemic manifestation of SS involves almost any organ system, and the salivary and the lacrimal glands are mainly affected, causing mouth and eye dryness (Fox Citation2005; Brito-Zerón et al. Citation2016). The mortality of primary Sjogren’s syndrome (pSS) is about 50% higher than that of the general population (Huang et al. Citation2021). Patients with SS have a poor quality of life, which increases psychological and physiological burdens (Vivino Citation2017). Currently, the pathogenesis of SS is still unknown. However, it might be connected to heredity, infection, sex hormones, and innate or acquired immunity (Wei et al. Citation2021; Negrini et al. Citation2022). Due to a lack of effective evidence, the current treatment of SS mainly includes symptomatic management and glucocorticoids and immunosuppressive agents to improve patients’ quality of life. However, these drugs have serious side effects and poor efficacy (Fox et al. Citation2021). A current research topic of great interest is biological agents that mediate B-cell hyperactivity, T-cell costimulation, and abnormal proinflammatory cytokine release in SS (Chowdhury et al. Citation2021). However, the efficacy and safety of such agents need to be further evaluated (Mavragani and Moutsopoulos Citation2020; Chowdhury et al. Citation2021). Therefore, effective and targeted therapies are urgently needed.
Traditional Chinese medicine (TCM), a unique medical system, has a lengthy history in China. TCM has fewer side effects and is suitable for the treatment of chronic non-serious diseases (Zheng et al. Citation2011). The pathogenesis of SS in TCM involves yin deficiency, dryness, heat, and blood stasis (Zhang Citation2011; Wang et al. Citation2021). Zengye decoction (ZYD), as a traditional Chinese medicine formula, is composed of Radix Scrophulariae (Chinese name Xuanshen; the dried root of Scrophularia ningpoensis Hemsl. [Scrophulariaceae]), Rehmannia glutinosa (Chinese name Shengdi; the tuber root of Rehmannia glutinosa (Gaertn.) Libosch. [Orobanchaceae]) and Radix Ophiopogonis (Chinese name Maidong; the dried tuber root of Ophiopogon japonicus (L. f.) Ker Gawl. [Asparagaceae]) with a weight ratio of 5:4:4 (Li et al. Citation2019). The decoction exhibits multiple pharmacological activities, such as metabolism, oxidative stress, cell ion balance, anti-inflammatory, and coagulation regulation (Yuewen et al. Citation2018). In clinical treatment, ZYD is used for treating constipation, SS, diabetes, pharyngitis, laryngitis, and other diseases (Minghao et al. Citation2016; Liu D et al. Citation2019; Wang M et al. Citation2020). Related research indicated that ZYD could lower the expression of serum TNF-α and IL-1β levels in SS model rats (Sun et al. Citation2015). Other research reported that ZYD protected the structure and function of submandibular glands (SMGs) in NOD mice (Li et al. Citation2019). Nevertheless, the mechanisms of ZYD against SS have not been elucidated.
Network pharmacology, a comprehensive, systematic, and holistic approach, predicts the target profiles and pharmacological actions of TCM (Luo et al. Citation2020). As a novel research approach, TCM network pharmacology is anticipated to succeed in achieving the transformation from experience-based to evidence-based therapy (Li and Zhang Citation2013; Zhang et al. Citation2019). The NOD mice with polyautoimmunity are an excellent model for studying autoimmune diseases, such as autoimmune thyroiditis, neuropathies, and SS, except for diabetes (Aubin et al. Citation2022). We acquired the active compounds and possible targets of ZYD against SS, built a network of drug–target–disease relationship, and predicted the pharmacological mechanisms of ZYD against SS using the TCM network pharmacology method. Animal experiments on NOD mice further proved the efficacy of ZYD and the predicted results of network pharmacology. Our research offers a theoretical foundation for the pathogenesis and clinical management of SS.
Materials and methods
Screening of bioactive compounds and therapeutic targets of ZYD
In the Traditional Chinese Medicine Systems Pharmacology Database and Analysis Platform (TCMSP) (https://tcmspw.com/tcmsp.php), we set the related parameters as follows: oral bioavailability (OB) ≥ 30% and drug-likeness (DL) ≥ 0.18 according to the characteristics of absorption, distribution, metabolism, excretion, and toxicity (ADME) (Ru et al. Citation2014). The three herbs Radix Scrophulariae, Rehmannia glutinosa and Radix Ophiopogonis of ZYD were separately retrieved to collect active compounds and targets. ZYD can nourish yin, increase body fluids, and moisten dryness. We used the Bioinformatics Analysis Tool for Molecular mechANism of Traditional Chinese Medicine (BATMAN-TCM) database (http://bionet.ncpsb.org/batman-tcm/) to supplement the search (Liu et al. Citation2016). Integrating the two databases’ search results, we collected the bioactive components and related targets of ZYD.
The GeneCards database (https://www.genecards.org/) provide abundant information for researchers on human genes, diseases, and proteins (Stelzer et al. Citation2016). In the database, ‘Sjogren’s syndrome’ as a keyword was used to screen SS-related targets. Finally, the common targets were collected between SS and ZYD using Venn analysis (http://www.bioinformatics.com.cn/).
PPI network construction
Using the STRING database, a protein–protein interaction (PPI) network with common targets was constructed (https://string-db.org/). ‘Homo sapiens’ was chosen as the species source and ‘medium confidence (0.400)’ was set as the minimum required interaction score. Simultaneously, disconnected nodes were hidden in the network, and other parameters kept the default values. Additionally, a bar graph showing the connection degree of core targets was constructed using R (ver. 4.0.2) software (Chan Citation2018).
GO and KEGG enrichment analysis
First, it is necessary to install some packages in the R 4.0.2 software, including colorspace, stringi, ggplot2, DOSE, ClusterProfiler, and enrichplot. Gene ontology (GO) and Kyoto Encyclopedia of Genes and Genomes (KEGG) pathway enrichment analyses of the common targets were carried out using R 4.0.2 software with a p-value = 0.05 and q-value = 0.05 (Chen et al. Citation2017).
Compound–target and pathway–target network construction
Cytoscape is a platform for analyzing, integrating, and visualizing complicated networks and network data. It provides basic network analysis functionality (Demchak et al. Citation2018). The compound–target and pathway–target networks of ZYD against SS were constructed to visualize their interrelationships using Cytoscape (ver. 3.8.1) software.
Molecular docking
Molecular docking was used to reflect the binding affinity of primary components and key targets. The components’ two-dimensional structures were retrieved from the PubChem database (https://pubchem.ncbi.nlm.nih.gov/) and transformed into three-dimensions using Chem3D software. After standardization of target names in the UniProt database (https://www.uniprot.org/), the target protein’s structure was obtained from the PDB database (http://www.rcsb.org/), and then removed solvent and organic of protein molecules using PyMOL software. Hydrogenated the protein molecules and found the energy pockets using AutoDock tools 1.5.6 software. Finally, AutoDock Vina 1.1.2 was used to carry out molecular docking between the component and target protein, and the docking findings were visualized using PyMOL software. The docking score reflected binding energy, and a lower docking indicates stronger binding activity. A docking score of < −5.0 kcal/mol indicated good binding activity, and < −7.0 kcal/mol indicated strong binding activity (Saikia and Bordoloi Citation2019).
Reagents and instruments
TNF-α, IL-1β, IL-6, and IL-17 enzyme-linked immunosorbent assay (ELISA) kits was purchased from Elabscience, China. Phenylmethylsulfonyl fluoride (PMSF) was purchased from Aladdin, China. A phosphatase inhibitor, radioimmunoprecipitation assay (RIPA) lysis buffer, and bicinchoninic acid (BCA) protein assay kit were ordered from Beyotime Biotechnology, China. Antibodies, including GAPDH, Bax, Bcl-2, Caspase-3 (CASP3), HRP-labeled rabbit anti-sheep IgG, and HRP-labeled rabbit anti-rats IgG, were purchased from Wuhan Sanyin Biotechnology Co., Ltd., China, and Wuhan Doctor De Biological Engineering Co., Ltd., China. An ECL substrate fluid was obtained from Beijing Plilai Gene Technology Co., Ltd., China. An X-ray photograph was purchased from Ruike Medical Equipment Co., Ltd., China. A developing and fixing kit was purchased from Tianjin Hanzhong Photography Materials Factory, China.
Preparation of ZYD
The following were purchased from the traditional Chinese Medicine Pharmacy of the Second Xiangya Hospital of Central South University (Changsha China): the dispensing granules of Radix Scrophulariae (lot. number: 200825), Rehmannia glutinosa (lot. number: 200867), and Radix Ophiopogonis (lot. number: 201186) in the proportion of 5:4:4. The plant materials were verified by Associate Professor of Fang Tang. The numbers of voucher specimen are YBZ-PFKL-2021131 (Radix Scrophulariae), YBZ-PFKL-2021115 (Rehmannia glutinosa), and Xiang-PF2021134 (Radix Ophiopogonis). These dispensing granules were mixed, dissolved, and diluted with boiling water to the required concentration (1.5 g/mL) (Sun et al. Citation2015).
Animals and administration
Sixteen NOD mice and 8 C57BL/6 mice aged 7 weeks and weighing 18–20 g (female, specific pathogen-free) were purchased from Changzhou Cavens Experimental Animal Co., Ltd., Changzhou, China. The 16 NOD mice were randomly allocated to two groups: the model group and the ZYD group, with eight mice in each group. The 8 C57BL/6 mice served as a control group. The standard feeding conditions for all animals included alternating 12 h light/dark cycle, with a constant temperature of 22 °C and humidity of 50%. After a week of adaptive feeding, ZYD was continuously administered for 8 weeks to the mice in the ZYD group by intragastric injection (20 mL/kg/d). The mice in the model and normal control groups received the same amount of physiological saline (20 mL/kg/d) (Sun et al. Citation2015). Peripheral blood was drawn at the end of the 8th week. The mice were then sacrificed by dislocation of the cervical vertebra, and the SMGs were removed for subsequent research.
The research was conducted in accordance with the principles of laboratory animal use and care as found in the European Community guidelines (EEC Directive of 1986; 86/609/EEC). The animal use protocol has been reviewed and approved by the Institutional Animal Care and Use Committee (IACUC), The Second Xiangya Hospital, Central South University, China (Approval Number: 2022002).
Determination of water consumption and salivary secretion
The mice in each group were provided with sufficient drinking water. The daily water consumption in each group was calculated as follows: the amount of water remaining on the previous day minus the amount remaining on that day. The weekly water consumption for each group was calculated.
The salivary secretions of mice were measured according to the wet weight method at 5, 6, 7, and 8 weeks after administration. First, the dry weight of the small cotton ball was measured on the electronic balance, and the weight was recorded as M0. After pentobarbital sodium was intraperitoneally injected to render the mice fully anesthetized, the small cotton ball was put into the opening of the duct of the SMGs of the mice. After 5 min, the cotton balls were removed and weighed again, and the weight was recorded as M1. Finally, the salivary secretions of mice were calculated using M1 and M0.
HE staining
The mice SMG tissues were embedded in paraffin after being fixed in 10% neutral formalin. The sections were then stained with hematoxylin and eosin (HE) and evaluated using a light microscope. The labial salivary gland biopsy criteria put out by Chisholm and Mason were used to score the SMG sections (Chisholm and Mason Citation1968). According to the lymphocytic infiltration and the number of lymphocytic foci per 4 mm2 of SMG tissue, the SMG sections were divided into grades 0 to 4. Grade 0 indicated no lymphocytic infiltration, which is recorded as 0 point. Grade 1 indicated slight lymphocytic infiltration but no lymphocytic foci, recorded as 1 point. Grade 2 indicated moderate infiltrate but no lymphocytic foci, recorded as 2 points. Grade 3 indicated 1 lymphocyte foci, recorded as 3 points. Grade 4 indicated more than 1 lymphocytic focus, recorded as 4 points (Lymphocytic foci were defined as an aggregate of 50 or more lymphocytes).
Enzyme-Linked immunosorbent assay
After 8 weeks of administration, peripheral blood was collected to determine the level of serum TNF-α, IL-1β, IL-6, and IL-17 using ELISA. The experiment was conducted in strict conformity with the instructions of the ELISA kit.
Western blot assay
After homogenizing the SMG tissue samples in RIPA lysis buffer containing PMSF and a phosphatase inhibitor, they were fully lysed on ice for 30 min to extract total protein. Protein concentrations were identified using BCA. Protein samples (40 μg) were separated using 10% SDS-polyacrylamide gels and transferred to polyvinylidene difluoride (PVDF) membranes, then blocked the membranes with 5% nonfat dry milk in Tris-buffered saline containing 0.05% Tween-20 (TBST) buffer. Subsequently, the membranes were incubated at 4 °C overnight with primary antibodies: GAPDH (internal reference; 1:1000; rabbit; AB-P-R001), Bax (1:5000; mouse; 60267-1-ig), Bcl-2 (1:1500; rabbit; 26593-1-ap), and CASP3 (1:3000; mouse; 66470-2-AP). Rabbit anti-goat and rabbit anti-mouse (1:1000; BA1060/BA1058) secondary antibodies with HRP-labeled were used to incubate these membranes for 2 h at room temperature. Then, these membranes with proteins were developed and fixed on film with an ECL reagent. Eventually, the film was scanned, and we analyzed the gray value with BandScan.
TUNEL staining
To evaluate the apoptosis rate in mice SMGs, terminal deoxynucleotidyl transferase dUTP nick-end labeling (TUNEL) staining was utilized. Sections of SMG tissue were digested with proteinase K and were incubated with terminal deoxynucleotidyl transferase (TdT) and dUTP at 37 °C for 1 h. After the sections were washed with phosphate buffered saline (PBS), a DAPI (4′,6-diamidino-2-phenylindole) staining solution was added to stain the nucleus. After the sections were thoroughly washed and slightly dried, then sealed with anti-fluorescence quenching tablets. Under a fluorescence microscope (Nikon, Japan), the sections were examined and pictures were collected. Finally, the fluorescence quantification was calculated using Image J software from the National Institutes of Health in the USA.
Statistical analysis
GraphPad Prism version 9.0 was used to analyze all data, and the results were shown as the mean ± standard deviation (SD). One-way analysis of variance (ANOVA) was carried out to compare the differences of the 2 groups, and p < 0.05 was regarded as statistically significant.
Results
Compounds, targets, and network construction
Based on the BATMAN-TCM database and TCMSP database, 47 active compounds were selected from the three herbs of ZYD. After removing the components without targets, the following 29 active compounds and 390 related targets were obtained: 8 compounds and 216 related targets in Radix Scrophulariae, 18 compounds and 121 related targets in Radix Ophiopogonis, and 3 compounds and 53 related targets in Rehmannia glutinosa (). Relevant targets (313) were obtained by combining and eliminating the duplicate targets in the 29 active components. 1038 disease-related targets were screened with ‘Sjogren’s syndrome’ as the keyword in the GeneCards database. Using Venn analysis, we obtained 32 common targets in ZYD and SS: ALOX5, GRIN3B, LYZ, TLR4, FXN, RXRB, TNF, CHRM3, CHRM1, CA2, PTGS1, SLC6A4, BCL2, BAX, CASP9, JUN, CASP3, CASP8, PON1, NR3C2, ESR1, DNMT1, PRKAB1, KCNC3, TPO, CAT, CYP17A1, NR3C1, VDR, MPO, XDH, and PRKCD ().
Figure 1. (A) Venn Diagram of common targets of ZYD and SS. (B) Compound-target network of ZYD. The network consists of 12 compounds (oval) and 32 hub genes (triangle), including 7 compounds in Xuanshen (sky-blue oval), 2 components in Maidong (yellow oval), 3 compounds in Shengdi (red oval) and 32 targets (purple triangle).
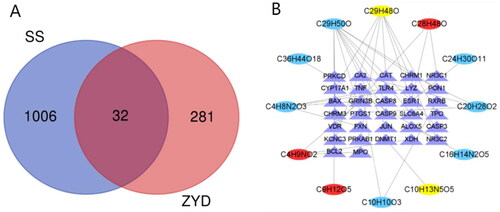
Table 1. 29 active compounds of ZYD.
The compound–target network containing the 32 common targets was constructed using Cytoscape software (). The network consisted of 44 nodes and 38 edges. Twelve compounds corresponded to these genes, including 7 compounds [14-deoxy-12 (R)-sulfoandrographolide, (−)-nissolin, p-methoxycinnamic acid, sugiol, β-sitosterol, harpagoside, and l-asparagine] in Radix Scrophulariae, 2 compounds (guanosine and stigmasterol) in Radix Ophiopogonis, and 3 compounds (rehmaglutin C, campesterol, and γ-aminobutyric acid) in Rehmannia glutinosa; of these compounds, the degree values of β-sitosterol and stigmasterol are higher, 11 and 7, respectively. β-Sitosterol and stigmasterol may be important components involved in the mechanisms of action of ZYD against SS. In the network, each compound had one or more targets. Similarly, each target could interact with one or more compounds. These reflected the features of TCM’s multiple compound and multiple target mechanisms of action.
Protein-protein interaction network construction
The 32 common targets were inputted into the STRING database. After deleting 3 unlinked targets (GRIN3B, PRKAB1, and KCNC3), the remaining 29 targets formed the PPI network (). The PPI network consists of 29 nodes and 92 edges. Targets and protein–protein associations are represented by the network’s nodes and edges, respectively. The top 20 core targets in the PPI network have a higher connection degree value (): TNF, CASP3, CAT, JUN, TLR4, ESR1, MPO, CASP8, CASP9, NR3C1, BAX, BCL2, DNMT1, ALOX5, PRKCD, PTGS1, XDH, CYP17A1, VDR, and LYZ.
Enrichment analysis and pathway–target network
To explore the potential mechanisms of ZYD against SS, GO and KEGG pathway enrichment analyses of the 32 common targets were performed with the aid of R software (p-value < 0.05). Using GO enrichment analysis, the functions of these targets were annotated from three aspects: the biological process (BP), the cellular component (CC), and the molecular function (MF). The results were visualized using three bar graphs (). The GO enrichment analysis results were as follows: (A) the BP was related to oxidative stress, positive regulation of neuron death, response to steroid hormones, response to reactive oxygen species, and cellular response to chemical stress; (B) the CC was mainly enriched in the presynaptic membrane, secretory granule lumen, and cytoplasmic vesicle lumen; (C) the MF was mainly associated with a steroid hormone receptor activity, nuclear receptor activity, ligand-activated transcription factor activity, peroxidase activity, apoptotic process, oxidoreductase activity, tumor necrosis factor receptor superfamily binding, and neurotransmitter binding. shows the top 20 KEGG pathways, which include apoptosis-multiple species signaling pathway, hepatitis B (HBV), toxoplasmosis, tuberculosis, apoptosis, measles, human immunodeficiency virus 1 infection (HIV-1), AGE-RAGE signaling pathway in diabetic complications, legionellosis, pathogenic Escherichia coli, p53 signaling pathway, Epstein-Barr virus (EBV), etc. The results of the KEGG enrichment analysis revealed that the mechanisms of action of ZYD against SS mainly involved the apoptosis pathway and the infection of multiple pathogenic microorganisms, among which the apoptosis-multiple species signaling pathway had a higher biological significance ().
Figure 3. GO enrichment analysis for 32 common genes in BP (A), CC (B), and MF (C). (D)KEGG pathway enrichment analysis. The longitudinal-axis of these bar graphs represents GO terms (A–C) or KEGG pathway name (D), and horizontal-axis represents the number of genes enriched in each GO terms. The color of the column represents the significance of enrichment: the redder the color, the more significant the enrichment of these genes.
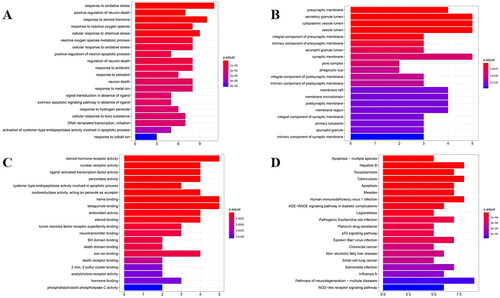
The targets enriched in each pathway are displayed in . The pathway–target network of ZYD against SS was constructed using Cytoscape3.8.1 software (). Sixteen genes were enriched in the top 20 pathways, including BCL2, BAX, CASP9, CASP3, CASP8, TLR4, TNF, JUN, ALOX5, VDR, PRKCD, PRKAB1, RXRB, CHRM3, CHRM1, and CAT. Each pathway had multiple targets, and every target appeared in multiple pathways, which revealed the network pharmacology characteristics of multiple targets and multiple pathways.
Figure 5. The pathway-target network of ZYD anti-SS. Pathways are shown as red fusiform, and target genes are shown as orange rectangle. The size of a node is proportional to the value of a degree.
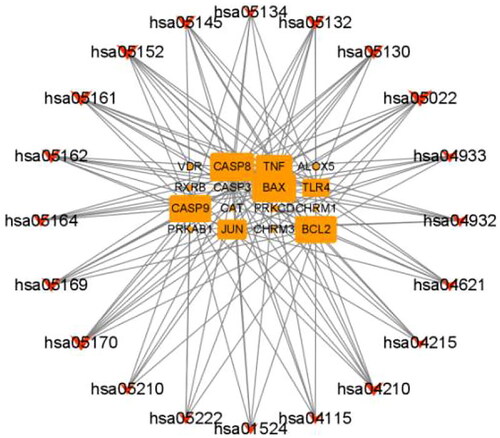
Table 2. KEGG pathway and targets enriched in each pathway.
Molecular docking analysis
Primary components (β-sitosterol and stigmasterol) and key targets (TNF, BCL2, BAX, CASP3) were selected for molecular simulation docking. The results revealed that the primary components had an effective binding activity with these key targets. Except for TNF, the binding energy between the other three targets (BCL2, BAX, CASP3) and the primary components was less than −7.0 kcal/mol, demonstrating strong binding activity (, ).
Figure 6. The molecular docking Models. (A) β-sitosterol and BAX. (B) β-sitosterol and CASP3. (C) stigmasterol and BAX. (D)stigmasterol and CASP3.
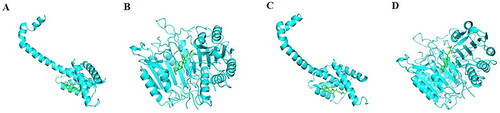
Table 3. The molecular docking Scores of primary components and key targets (kcal/mol).
ZYD decreases water consumption and increases salivary secretion
To verify the therapeutic effects of ZYD on SS, we designed an animal experiment. The model group’s weekly water consumption increased significantly compared with the control group (p < 0.01). From the 2nd week, the weekly water consumption in the ZYD group decreased significantly as compared to the model group (p < 0.01) (). From the 5th week, the weekly salivary secretion in the model group decreased significantly compared to that in the control group, while it increased significantly after ZYD administration (p < 0.01) (). These results suggested that ZYD could improve the thirst symptom and increase the salivary secretion of NOD mice.
Histopathological findings
The extent of damage and lymphocytic infiltration in the SMGs was evaluated using HE stain. As shown in , in the control group, the SMGs were undamaged, and the inner walls of the glands were smooth, with few or no lymphocytic infiltration. Whereas, in the model group, the SMG’s structure was destroyed and infiltrated by lymphocytes. In comparison to the model group, the ZYD group showed less SMG damage and lymphocytic infiltration. These differences were statistically significant based on the histological score of SMGs (p < 0.01) ().
ZYD decreased the levels of serum TNF-α, IL-1β, IL-6, and IL-17
In the compound–target network, we found that β-sitosterol and stigmasterol were the primary components involved in the mechanisms of action of ZYD against SS. β-Sitosterol and stigmasterol were considered to have anti-inflammatory effects and could inhibit the production of inflammatory cytokines, including TNF-α, IL-1β, IL-6, and IL-17 (Yang et al. Citation2019; Ahmad Khan et al. Citation2020). TNF-α, IL-1β, IL-6, and IL-17 levels rise in the serum of patients with SS (Pollard et al. Citation2013). Based on previous research, we determined the levels of TNF-α, IL-1β, IL-6, and IL-17 in mice serum using the ELISA method. According to the findings of our experimental, the model group’s serum levels of these inflammatory cytokines were significantly higher than those of the control group (p < 0.0001). After ZYD administration, the ZYD group showed significantly decreased serum levels of these inflammatory cytokines as compared to the model group (p < 0.0001) ().
ZYD has an anti-apoptosis effect on SMGs in NOD mice
To confirm the anti-apoptosis effect of ZYD, the expression of Bcl-2, Bax and CASP3 proteins was identified through a western blot (WB) assay, and the SMGs were stained with TUNEL. In accordance with the results of WB analysis, when compared to the control group, the relative expression of Bcl-2 protein was dramatically downregulated (p < 0.01) and the expression of Bax and CASP3 proteins were significantly upregulated (p < 0.001) in the model group (). After ZYD administration, the relative expression of Bcl-2 protein was significantly upregulated (p < 0.01) and the expression of Bax and CASP3 proteins was significantly downregulated (p < 0.05 and p < 0.01) in the ZYD group compared to the model group. The TUNEL staining results were shown in . The apoptotic SMG epithelial cells were marked with a green fluorescent protein (GFP). In comparison to the control group, there were more apoptotic cells in the model group (p < 0.01). The ZYD group showed fewer apoptotic cells than the model group (p < 0.01), which reflected the anti-apoptotic impact of ZYD in treating SS.
Figure 10. (A) Western blotting was used to detect the expression of Bcl-2, Bax and Caspase3 proteins in mice. (B–D) The level of relative expression of Bcl-2, Bax, and Caspase3. ##p < .01 and ###p < .001 compared with the control group. *p < .05 and **p < .01 compared with the model group.
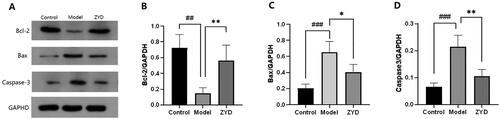
Figure 11. (A) Representative TUNNEL staining images of SMG epithelial cells sections in mice magnified 200 times, with green fluorescence indicating the apoptotic cells. (B) The fluorescence quantification of TUNNEL staining. ##p < .01 compared with the control group. **p < .01 compared with the model group.
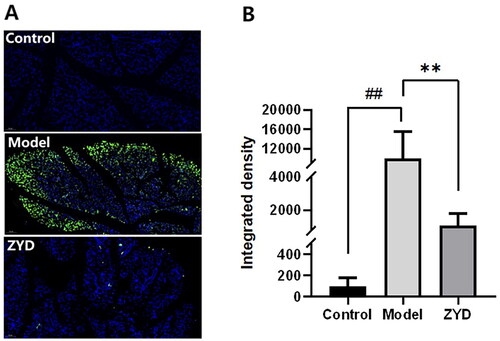
Discussion
In this study, 29 active compounds and 313 putative targets of ZYD were identified in the BATMAN-TCM and TCMSP databases, and 1038 SS-related targets were searched in the GeneCards database. Then, 32 common targets of ZYD and SS were screened via Venn analysis, which may be key targets when studying the mechanisms of action of ZYD against SS. The 32 common targets were employed for subsequent network construction and functional and pathway enrichment analysis. The compound–target network indicated that β-sitosterol and stigmasterol were crucial components in treating SS with ZYD. Twenty core targets were identified in the PPI network. Retrieving the 20 core targets in the GeneCards database, we found that most of them are related to apoptosis, such as TNF, CASP3, CASP8, CASP9, BAX, BCL2, and PRKCD. Additionally, TNF was involved in inflammatory and immune responses. β-Sitosterol and stigmasterol had good docking activity with TNF, BCL2, BAX, CASP3, respectively. The GO enrichment analysis showed that the mechanisms of action of ZYD against SS involved oxidative stress, neuron death, response to steroid hormone and other biological processes, and various molecular functions, such as steroid hormone receptor activity, apoptotic process, tumor necrosis factor receptor superfamily binding, and neurotransmitter binding. Oxidative stress often occurs in immune activation and inflammatory states (Norheim et al. Citation2012). Previous studies reported that oxidative stress may be related to the etiology of SS, and increased levels of oxidative stress markers (protein carbonyl, advanced oxidation protein products, 8-hydroxy-2′-deoxyguanosine, and hexanoyl-lysine) were found in the saliva, conjunctiva, and plasma of patients with SS (Ryo et al. Citation2006; Norheim et al. Citation2012; Wakamatsu et al. Citation2013). SS usually occurs in menopausal women, which was related to the lack of estrogen levels and the increase of apoptosis of exocrine gland cells, while, in men, the gonads produce testosterone and convert it into dihydrotestosterone (DHT), which protects against acinar cell apoptosis (Konttinen et al. Citation2012). The neurologic manifestations of SS were common extraglandular manifestations. Some studies have found that the neurological manifestations of patients with SS were related to the loss of nerve fibers and degeneration of neuron (Mori et al. Citation2005; Birnbaum et al. Citation2019). KEGG enrichment analysis indicated that the mechanisms of action of ZYD against SS mainly involved the apoptosis pathway, and other mechanisms were involved with viral infections (HBV, measles, HIV-1, and EBV) and bacterial infections (Mycobacterium tuberculosis (MT), legionellosis, pathogenic E. coli, and Salmonella). Viral and bacterial infections may cause immune system dysregulation and trigger SS (Sipsas et al. Citation2011; Yanagisawa et al. Citation2018; Bartoloni et al. Citation2019). A bioinformatics study on the progression of pSS found that upregulated differentially expressed genes (DEGs) in pSS patients compared to controls were enriched in the infection-related pathway such as EBV infection (Li N et al. Citation2021). However, whether ZYD has antiviral and antibacterial effects has not been reported yet, and this needs to be further explored. According to the KEGG results in this study, we speculated that ZYD might treat SS triggered by infectious agents.
Phytosterols β-sitosterol and stigmasterol may have a range of biological effects, such as antiviral, anti-inflammatory, immunosuppressive and anticancer effects (Awad and Fink Citation2000; Le et al. Citation2017; Paniagua-Perez et al. Citation2017). It was reported that the immunomodulatory effects of β-sitosterol and stigmasterol inhibited the growth of T cells that induced by Concanavalin A (ConA) in murine cells (Le et al. Citation2017). β-Sitosterol, especially, could reduce the production of T helper 2 (Th2) cytokines (IL-4 and IL-10) and significantly decrease the proliferation of both CD4+ CD25+ Th cells and CD8+CD25+cytotoxic T cells. It was discovered that SS patients had considerably more CD4+CD25high T cells in the peripheral blood (Gottenberg et al. Citation2005). In the intracranial aneurysm (IA) rat model, β-sitosterol could reduce the expression of TNF-α, IL-1β, IL-6, and IL-17 in the circle of Willis (Yang et al. Citation2019). Stigmasterol inhibited the expression of proinflammatory cytokines TNF-α, IL-6, IL-1β by downregulating the expression of NF-kBp65 and p38MAPK in the joint tissues of rats with collagen-induced arthritis (CIA) (Ahmad Khan et al. Citation2020). This study’s animal experiment indicated that ZYD could decrease water consumption, increase salivary secretion, improve SMG damage, and reduce SMG lymphocytic infiltration in NOD mice. Additionally, the serum levels of TNF-α, IL-1β, IL-6, and IL-17 were considerably higher in the model group than those of the control group, whereas the ZYD group’s serum levels of these cytokines were significantly decreased as compared to the model group. The histological characteristic of SS is the infiltration of immune cells in the lacrimal and salivary glands, including CD4+ Th cells, CD8+ cytotoxic T (Tc) cells, B cells, plasma cells. The CD4+ T cells predominate in the early stage of SS, while B cells or plasma cells predominate as the disease progresses (Psianou et al. Citation2018; Saito et al. Citation2018). Previous research found that Th1 and Th17 cells appeared in the initial stage of SS, and Th2 and follicular helper T (Tfh) cells predominate as the disease progresses (Maehara et al. Citation2012). Abnormal cytokine secretion plays a major part in the pathogenesis of SS, including the overexpression of pro-inflammatory cytokines (TNF-α, IFN-γ, IL-1β, IL-6, and IL-17) and the low expression of anti-inflammatory cytokines (TGF-β) (Boumba et al. Citation1995; Roescher et al. Citation2010; Psianou et al. Citation2018). Studies have reported that ZYD might lower the serum levels of TNF-α, IL-1β, and IFN-γ in SS model mice (Sun et al. Citation2015; Zhou et al. Citation2020). A study showed significantly decreased the serum IFN-γ-to-IL-10 ratio and lymphocytic infiltration of SMGs in NOD mice after ZYD administration. Thus, the study speculated that ZYD protected SMGs in NOD mice by correcting the cytokine imbalance of the Th1 (e.g. IFN-γ) and Th2 (e.g. IL-10) (Li et al. Citation2019). The findings of our study demonstrated that the mechanism of action of ZYD against SS may be related to inhibiting the production of inflammatory cytokines.
Additionally, apoptosis damages the salivary and lacrimal glands in patients with SS (Manganelli and Fietta Citation2003). The increased apoptosis rate of epithelial cells in SS may be related to the downregulation of BCL2 (an apoptosis inhibitor) and upregulation of Bax (an apoptosis inducer) (Manganelli and Fietta Citation2003). The 2016 ACR/EULAR criteria reflect that the lymphocytic focus score of the salivary glands and anti-SSA/Ro account for the highest weights in the classification criteria of pSS (Shiboski et al. Citation2017). Anti-SSA/Ro has a pro-apoptotic effect. TRIM21- (anti-SSA/Ro-) targeting microRNAs downregulated not only the expression of anti-SSA/Ro but also the expression of pro-apoptotic genes BAX, CASP8, and CASP9 and upregulated the expression of antiapoptotic gene BCL2 (Yang et al. Citation2020). One study found that significant activation of CASP3 and a high ratio of Bax/Bcl-2 were detected in the SMGs of SS model mice (Lin et al. Citation2011). The outcomes of GO and KEGG enrichment analyses revealed that the underlying mechanism of action of ZYD against SS may be associated with apoptosis. The most significant KEGG pathway was apoptosis-multiple species, which involved target proteins in the apoptosis-mammals signaling pathway, including BCL2, BAX, CASP9, CASP3, and CASP8 (). Moreover, our animal experiment showed that the model group had a higher Bax and CASP3 expression and a lower Bcl-2 expression than those of the control group, while the ZYD group showed a lower Bax and CASP3 expression and a higher Bcl-2 expression than those in the model group. Finally, TUNEL staining confirmed that ZYD had an anti-apoptotic effect in treating SS.
Conclusion
Network pharmacology predicted that β-sitosterol and stigmasterol (identified to have anti-inflammatory effects) were the most important compounds, and apoptosis was the main pathway involved in the mechanisms of action of ZYD against SS. Our animal experiment verified that ZYD exerted an anti-inflammatory impact by inhibiting the serum levels of inflammatory cytokines, including TNF-α, IL-1β, IL-6, and IL-17. Moreover, ZYD exerted an anti-apoptotic effect by downregulating the expression of Bax and CASP3 and upregulating the expression of Bcl-2 in NOD mice. Therefore, the potential mechanisms of action ZYD against SS may be associated with anti-inflammation and anti-apoptosis. This research provides a theoretical basis for treating SS with ZYD and increases the selectivity for subsequent SS clinical treatment. However, further trials are necessary to confirm the effective dosage and adverse reactions of ZYD in the treatment of SS.
Author contributions
JY and JT conceived and designed the study; JY searched the databases and performed the data analysis, wrote the manuscript. JY, SW, JY, WH and BT conducted animal experiment and statistical analysis. JT supervised the study, performed the data analysis and revised the manuscript. WP supervised the study and revised the manuscript.
Acknowledgments
We thank Bullet Edits Limited for the linguistic editing and proofreading of the manuscript.
Disclosure statement
The authors declare that the research was conducted in the absence of any commercial or financial relationships that could be construed as a potential declaration of interest.
Additional information
Funding
References
- Ahmad Khan M, Sarwar A, Rahat R, Ahmed RS, Umar S. 2020. Stigmasterol protects rats from collagen induced arthritis by inhibiting proinflammatory cytokines. Int Immunopharmacol. 85:106642. doi: 10.1016/j.intimp.2020.106642.
- Aubin AM, Lombard-Vadnais F, Collin R, Aliesky HA, McLachlan SM, Lesage S. 2022. The NOD mouse beyond autoimmune diabetes. Front Immunol. 13:874769. doi: 10.3389/fimmu.2022.874769.
- Awad A, Fink C. 2000. Phytosterols as anticancer dietary components: evidence and mechanism of action. J Nutr. 130(9):2127–2130. doi: 10.1093/jn/130.9.2127.
- Bartoloni E, Alunno A, Gerli R. 2019. The dark side of Sjogren’s syndrome: the possible pathogenic role of infections. Curr Opin Rheumatol. 31(5):505–511. doi: 10.1097/BOR.0000000000000631.
- Birnbaum J, Lalji A, Saed A, Baer AN. 2019. Biopsy-proven small-fiber neuropathy in primary Sjogren’s syndrome: neuropathic pain characteristics, autoantibody findings, and histopathologic features. Arthritis Care Res. 71(7):936–948. doi: 10.1002/acr.23762.
- Boumba D, Skopouli F, Moutsopoulos H. 1995. Cytokine mRNA expression in the labial salivary gland tissues from patients with primary Sjögren’s syndrome. Br J Rheumatol. 34(4):326–333. doi: 10.1093/rheumatology/34.4.326.
- Brito-Zerón P, Baldini C, Bootsma H, Bowman SJ, Jonsson R, Mariette X, Sivils K, Theander E, Tzioufas A, Ramos-Casals M. 2016. Sjogren syndrome. Nat Rev Dis Primers. 2:16047. doi: 10.1038/nrdp.2016.47.
- Chan, BKC. 2018. Data analysis using R programming. Adv Exp Med Biol. 1082:47–122.
- Chen L, Zhang YH, Wang S, Zhang Y, Huang T, Cai YD. 2017. Prediction and analysis of essential genes using the enrichments of gene ontology and KEGG pathways. PLOS One. 12(9):e0184129. doi: 10.1371/journal.pone.0184129.
- Chisholm D, Mason D. 1968. Labial salivary gland biopsy in Sjögren’s disease. J Clin Pathol. 21(5):656–660. doi: 10.1136/jcp.21.5.656.
- Chowdhury F, Tappuni A, Bombardieri M. 2021. Biological therapy in primary Sjogren’s syndrome: effect on salivary gland function and inflammation. Front Med. 8:707104. doi: 10.3389/fmed.2021.707104.
- Demchak B, Otasek D, Pico AR, Bader GD, Ono K, Settle B, Sage E, Morris JH, Longabaugh W, Lopes C, et al. 2018. The cytoscape automation app article collection. F1000Res. 7:800. doi: 10.12688/f1000research.15355.1.
- Fox RI. 2005. Sjögren’s syndrome. Lancet. 366(9482):321–331. doi: 10.1016/S0140-6736(05)66990-5.
- Fox RI, Fox CM, Gottenberg JE, Dorner T. 2021. Treatment of Sjogren’s syndrome: current therapy and future directions. Rheumatology. 60(5):2066–2074. doi: 10.1093/rheumatology/kez142.
- Gottenberg JE, Lavie F, Abbed K, Gasnault J, Le Nevot E, Delfraissy JF, Taoufik Y, Mariette X. 2005. CD4 CD25high regulatory T cells are not impaired in patients with primary Sjögren’s syndrome. J Autoimmun. 24(3):235–242. doi: 10.1016/j.jaut.2005.01.015.
- Huang H, Xie W, Geng Y, Fan Y, Zhang Z. 2021. Mortality in patients with primary Sjogren’s syndrome: a systematic review and meta-analysis. Rheumatology. 60(9):4029–4038. doi: 10.1093/rheumatology/keab364.
- Konttinen YT, Fuellen G, Bing Y, Porola P, Stegaev V, Trokovic N, Falk SS, Liu Y, Szodoray P, Takakubo Y. 2012. Sex steroids in Sjogren’s syndrome. J Autoimmun. 39(1–2):49–56. doi: 10.1016/j.jaut.2012.01.004.
- Le CF, Kailaivasan TH, Chow SC, Abdullah Z, Ling SK, Fang CM. 2017. Phytosterols isolated from Clinacanthus nutans induce immunosuppressive activity in murine cells. Int Immunopharmacol. 44:203–210. doi: 10.1016/j.intimp.2017.01.013.
- Li S, Zhang B. 2013. Traditional Chinese medicine network pharmacology: theory, methodology and application. Chin J Nat Med. 11(2):110–120. doi: 10.1016/S1875-5364(13)60037-0.
- Li CY, Wu SL, Sun LX, Yan TT, Wang Y. 2019. Protective effect of zengye decoction on submandibular glands in nonobese diabetic mice. Chin J Integr Med. 25(1):45–50. doi: 10.1007/s11655-014-1981-5.
- Li N, Li L, Wu M, Li Y, Yang J, Wu Y, Xu H, Luo D, Gao Y, Fei X. 2021. Integrated bioinformatics and validation reveal potential biomarkers associated with progression of primary Sjogren’s syndrome. Front Immunol. 12:697157. doi: 10.3389/fimmu.2021.697157.
- Lin X, Song JX, Shaw PC, Ng TB, Wong RN, Sze SC, Tong Y, Lee KF, Zhang KY. 2011. An autoimmunized mouse model recapitulates key features in the pathogenesis of Sjogren’s syndrome. Int Immunol. 23(10):613–624. doi: 10.1093/intimm/dxr066.
- Liu D, Lin L, Lin Y, Zhong Y, Zhang S, Liu W, Zou B, Liao Q, Xie Z. 2019. Zengye decoction induces alterations to metabolically active gut microbiota in aged constipated rats. Biomed Pharmacother. 109:1361–1371. doi: 10.1016/j.biopha.2018.11.013.
- Liu Z, Guo F, Wang Y, Li C, Zhang X, Li H, Diao L, Gu J, Wang W, Li D, et al. 2016. BATMAN-TCM: a bioinformatics analysis tool for molecular mechanism of traditional Chinese medicine. Sci Rep. 6:21146. doi: 10.1038/srep21146.
- Luo TT, Lu Y, Yan SK, Xiao X, Rong XL, Guo J. 2020. Network pharmacology in research of Chinese medicine formula: methodology, application and prospective. Chin J Integr Med. 26(1):72–80. doi: 10.1007/s11655-019-3064-0.
- Maehara T, Moriyama M, Hayashida JN, Tanaka A, Shinozaki S, Kubo Y, Matsumura K, Nakamura S. 2012. Selective localization of T helper subsets in labial salivary glands from primary Sjögren’s syndrome patients. Clin Exp Immunol. 169(2):89–99. doi: 10.1111/j.1365-2249.2012.04606.x.
- Manganelli P, Fietta P. 2003. Apoptosis and Sjogren syndrome. Semin Arthritis Rheum. 33(1):49–65. doi: 10.1053/sarh.2003.50019.
- Mavragani CP, Moutsopoulos HM. 2020. Sjogren’s syndrome: old and new therapeutic targets. J Autoimmun. 110:102364. doi: 10.1016/j.jaut.2019.102364.
- Minghao L, Weina D, Zhenling Z. 2016. Research progress in clinical application of zengyetang. Acta Chinese Med. 31:1525–1528.
- Mori K, Iijima M, Koike H, Hattori N, Tanaka F, Watanabe H, Katsuno M, Fujita A, Aiba I, Ogata A, et al. 2005. The wide spectrum of clinical manifestations in Sjogren’s syndrome-associated neuropathy. Brain. 128(Pt 11):2518–2534. doi: 10.1093/brain/awh605.
- Negrini S, Emmi G, Greco M, Borro M, Sardanelli F, Murdaca G, Indiveri F, Puppo F. 2022. Sjogren’s syndrome: a systemic autoimmune disease. Clin Exp Med. 22(1):9–25. doi: 10.1007/s10238-021-00728-6.
- Norheim KB, Jonsson G, Harboe E, Hanasand M, Goransson L, Omdal R. 2012. Oxidative stress, as measured by protein oxidation, is increased in primary Sjogren’s syndrome. Free Radic Res. 46(2):141–146. doi: 10.3109/10715762.2011.645206.
- Paniagua-Perez R, Flores-Mondragon G, Reyes-Legorreta C, Herrera-Lopez B, Cervantes-Hernandez I, Madrigal-Santillan O, Morales-Gonzalez JA, Alvarez-Gonzalez I, Madrigal-Bujaidar E. 2017. Evaluation of the anti-inflammatory capacity of beta-sitosterol in rodent assays. Afr J Tradit Complement Altern Med. 14(1):123–130. doi: 10.21010/ajtcam.v14i1.13.
- Pollard RP, Abdulahad WH, Bootsma H, Meiners PM, Spijkervet FK, Huitema MG, Burgerhof JG, Vissink A, Kroese FG. 2013. Predominantly proinflammatory cytokines decrease after B cell depletion therapy in patients with primary Sjogren’s syndrome. Ann Rheum Dis. 72(12):2048–2050. doi: 10.1136/annrheumdis-2013-203447.
- Psianou K, Panagoulias I, Papanastasiou AD, de Lastic AL, Rodi M, Spantidea PI, Degn SE, Georgiou P, Mouzaki A. 2018. Clinical and immunological parameters of Sjögren’s syndrome. Autoimmun Rev. 17(10):1053–1064. doi: 10.1016/j.autrev.2018.05.005.
- Roescher N, Tak PP, Illei GG. 2010. Cytokines in Sjögren’s syndrome: potential therapeutic targets. Ann Rheum Dis. 69(6):945–948. doi: 10.1136/ard.2009.115378.
- Ru J, Li P, Wang J, Zhou W, Li B, Huang C, Li P, Guo Z, Tao W, Yang Y, et al. 2014. TCMSP: a database of systems pharmacology for drug discovery from herbal medicines. J Cheminform. 6:13. doi: 10.1186/1758-2946-6-13.
- Ryo K, Yamada H, Nakagawa Y, Tai Y, Obara K, Inoue H, Mishima K, Saito I. 2006. Possible involvement of oxidative stress in salivary gland of patients with Sjogren’s syndrome. Pathobiology. 73(5):252–260. doi: 10.1159/000098211.
- Saikia S, Bordoloi M. 2019. Molecular docking: challenges, advances and its use in drug discovery perspective. CDT. 20(5):501–521. doi: 10.2174/1389450119666181022153016.
- Saito M, Otsuka K, Ushio A, Yamada A, Arakaki R, Kudo Y, Ishimaru N. 2018. Unique phenotypes and functions of follicular helper T cells and regulatory T cells in Sjögren’s syndrome. Curr Rheumatol Rev. 14(3):239–245. doi: 10.2174/1573397113666170125122858.
- Shiboski CH, Shiboski SC, Seror R, Criswell LA, Labetoulle M, Lietman TM, Rasmussen A, Scofield H, Vitali C, Bowman SJ, et al. 2017. 2016 American College of Rheumatology/European League Against Rheumatism classification criteria for primary Sjögren’s syndrome: a consensus and data-driven methodology involving three international patient cohorts. Arthritis Rheumatol. 69(1):35–45. doi: 10.1002/art.39859.
- Sipsas NV, Gamaletsou MN, Moutsopoulos HM. 2011. Is Sjögren’s syndrome a retroviral disease? Arthritis Res Ther. 13(2):212. doi: 10.1186/ar3262.
- Stelzer G, Rosen N, Plaschkes I, Zimmerman S, Twik M, Fishilevich S, Stein T, Nudel R, Lieder I, Mazor Y, et al. 2016. The GeneCards suite: from gene data mining to disease genome sequence analyses. Curr Protoc Bioinformatics. 54:1.30.31–31.30.33.
- Sun L, Qin P, Zhang L, Sun H. 2015. Researches on the zengye tang in the influences of TNF-α and IL-1β over the SS rats. Western J Trad Chinese Med. 28:13–14.
- Vivino FB. 2017. Sjogren’s syndrome: clinical aspects. Clin Immunol. 182:48–54. doi: 10.1016/j.clim.2017.04.005.
- Wakamatsu TH, Dogru M, Matsumoto Y, Kojima T, Kaido M, Ibrahim OM, Sato EA, Igarashi A, Ichihashi Y, Satake Y, et al. 2013. Evaluation of lipid oxidative stress status in Sjogren syndrome patients. Invest Ophthalmol Vis Sci. 54(1):201–210. doi: 10.1167/iovs.12-10325.
- Wang G, Qi WX, Yan YF, Wang T, Zou FX. 2021. Mechanism of Chinese medicine against Sjögren syndrome: a review. Chinese J of Exper Trad Med Formulae. 27(20):227–235.
- Wang M, Chang SQ, Tian YS, Zhang GQ, Qi J. 2020. Zengye decoction ameliorates insulin resistance by promoting glucose uptake. Rejuvenation Res. 23(5):367–376. doi: 10.1089/rej.2019.2228.
- Wei S, He Q, Zhang Q, Fu K, Li R, Peng W, Gao Y. 2021. Traditional Chinese medicine is a useful and promising alternative strategy for treatment of Sjogren’s syndrome: a review. J Integr Med. 19(3):191–202. doi: 10.1016/j.joim.2021.01.008.
- Yanagisawa N, Ueshiba H, Abe Y, Kato H, Higuchi T, Yagi J. 2018. Outer membrane protein of gut commensal microorganism induces autoantibody production and extra-intestinal gland inflammation in mice. Int J Mol Sci. 19:3241. doi: 10.3390/ijms19103241.
- Yang Q, Yu D, Zhang Y. 2019. Beta-sitosterol attenuates the intracranial aneurysm growth by suppressing TNF-alpha-mediated mechanism. Pharmacology. 104(5-6):303–311. doi: 10.1159/000502221.
- Yang Y, Hou Y, Li J, Zhang F, Du Q. 2020. Characterization of antiapoptotic microRNAs in primary Sjogren’s syndrome. Cell Biochem Funct. 38(8):1111–1118. doi: 10.1002/cbf.3569.
- Yuewen L, Zhiqiang, L, Bolong W. 2018. Study on network target prediction and action mechanism of Zengye decoction. Chin J Mod Appl Pharm. 35:1842–1848.
- Zhang R, Zhu X, Bai H, Ning K. 2019. Network pharmacology databases for traditional Chinese medicine: review and assessment. Front Pharmacol. 10:123. doi: 10.3389/fphar.2019.00123.
- Zhang S. 2011. The TCM etiology, pathogenesy and differential treatment for Sjogren’s syndrome. J Tradit Chin Med. 31(1):73–78. doi: 10.1016/s0254-6272(11)60017-4.
- Zheng LW, Hua H, Cheung LK. 2011. Traditional Chinese medicine and oral diseases: today and tomorrow. Oral Dis. 17(1):7–12. doi: 10.1111/j.1601-0825.2010.01706.x.
- Zhou J, Zhou Q, Li JG, Liu RL. 2020. Effect of zengye runzao decoction on INF-γ, TNF-α and aquaporin in submandibular gland of Sjogren’s syndrome model mice. Acta Chinese Med. 35:2407–2410.