Abstract
Context
Yi-Shen-Hua-Shi (YSHS) is a traditional Chinese medicine that treats chronic kidney disease (CKD). However, its efficacy in reducing proteinuria and underlying mechanisms is unknown.
Objective
This single-center randomized controlled trial explored whether YSHS could improve proteinuria and modulate the gut microbiota.
Materials and methods
120 CKD patients were enrolled and randomized to receive the renin-angiotensin-aldosterone system (RAAS) inhibitor plus YSHS (n = 56) or RAAS inhibitor (n = 47) alone for 4 months, and 103 patients completed the study. We collected baseline and follow-up fecal samples and clinical outcomes from participants. Total bacterial DNA was extracted, and the fecal microbiome was analyzed using bioinformatics.
Results
Patients in the intervention group had a significantly higher decrease in 24-h proteinuria. After 4 months of the YSHS intervention, the relative abundance of bacteria that have beneficial effects on the body, such as Faecalibacterium, Lachnospiraceae, Lachnoclostridium, and Sutterella increased significantly, while pathogenic bacteria such as the Eggerthella and Clostridium innocuum group decreased. However, we could not find these changes in the control group. Redundancy analysis showed that the decline in 24-h proteinuria during follow-up was significantly correlated with various taxa of gut bacteria, such as Lachnospiraceae and the Lachnoclostridium genus in the YSHS group. KEGG analysis also showed the potential role of YSHS in regulating glycan, lipid, and vitamin metabolism.
Discussion and conclusion
The YSHS granule reduced proteinuria associated with mitigating intestinal microbiota dysbiosis in CKD patients. The definite mechanisms of YSHS to improve proteinuria need to be further explored.
Trial registration
ChiCTR2300076136, retrospectively registered.
Introduction
Chronic kidney disease (CKD) has become a major public health problem worldwide (Global, regional, and national burden of chronic kidney disease, 1990–2017: a systematic analysis for the GBD Chronic Kidney Disease Collaboration 2020). The global prevalence of CKD was estimated to be approximately 10% of the adult population (Jha et al. Citation2013) and 10.8% in China (Zhang et al. Citation2012). The global burden of disease (GBD) analysis has shown that CKD is one of the most common causes of mortality. Various factors independently affect the kidney, such as age, sex, region, socio-economic status, and multimorbidity. Generally, chronic conditions such as hypertension and diabetes are the major cause of CKD. CKD patients regularly take medications to control blood glucose, blood pressure, lipids and a low-protein diet to reduce proteinuria. Increased proteinuria was a risk factor for renal function decline, all-cause mortality, and cardiovascular mortality (Su et al. Citation2020).
The gut microbiota can be divided into two main enterotypes according to the dominant bacterial phylotype, Firmicutes and Bacteroidetes. Among these, Clostridium genera represent 95% of the Firmicutes phyla, while Bacteroidetes are represented mainly by Bacteroides and Prevotella (Rinninella et al. Citation2019). Bacteroides predominantly metabolize protein, while Prevotella metabolizes saccharin. Gut dysbiosis has been reported in antibiotic-associated bone marrow suppression (Josefsdottir et al. Citation2017), depression (Kelly et al. Citation2019), vascular dysfunction (Karbach et al. Citation2016), and immune-mediated diseases (Rooks and Garrett Citation2016). Past studies have shown that CKD patients are prone to intestinal microecology disorders, with a decreasing abundance of Lactobacillus, Bifidobacterium, Bacteroides, and Prevotella (Huang et al. Citation2022). Gut dysbiosis, in contrast, contributes to the pathogenesis of CKD (Zaky et al. Citation2021). Increasing evidence indicates a vital role for the gut microbiota in the progression of CKD and related complications (Felizardo et al. Citation2016; Yang et al. Citation2018). Several mechanisms have been proposed, such as gut microbiome alterations, dysregulation of microbiota-derived uremic toxins (Wong et al. Citation2014), and activation of aryl hydrocarbon receptors (Ichii et al. Citation2014).
Yi-Shen-Hua-Shi (YSHS) granule is a traditional Chinese medicine (developed by Guangzhou Consun Pharmaceutical Co. Ltd) consisting of 16 herbs, namely: Ginseng Redix et Rhizoma, Astragali Radix, Atractylodis Macrocephalae Rhizoma, Poria, Alismatis Rhizoma, Pinelliae Rhizoma Praeparatum Cum Alumine, Notopterygii Rhizoma et Radix, Angelicae Pubescentis Radix, Saposhnikoviae Radix, Bupleuri Radix, Copidis Rhizoma, Paeoniae Radix Alba, Citri Reticulatae Pericarpium, Glycyrrhizae Radix et Rhizoma praeparata Cum Melle, Zingiberis Rhizoma Recens and Jujubae Fructus. The drug has previously been shown to be effective in restoring renal function, reducing dampness, and improving edema. According to the instructions, chronic glomerulonephritis patients with serum creatinine < 2 mg/dL are also recommended. The granule has been reported to improve chronic glomerulonephritis in a rat model (Zhao et al. Citation2019), improve podocyte injury, and alleviate the progression of diabetic nephropathy (Liang et al. Citation2022). However, the precise mechanism by which YSHS granules reduce proteinuria remains unclear. This study explored whether the YSHS granule could improve proteinuria and clinical manifestations in CKD patients by regulating intestinal microecology.
Methods
Study design and participants
We conducted a single-center, randomized controlled trial (RCT) with 120 non-dialysis CKD patients in the Nephrology Department of Xuanwu Hospital, Capital Medical University, from March 2021 to October 2022. The inclusion criteria were patients aged >18 years with an estimated glomerular filtration rate (eGFR) ≥ 45 mL/min/1.73 m2 and 24-h proteinuria > 0.5 g. All patients had received treatment with the renin-angiotensin-aldosterone system (RAAS) inhibitor for more than 8 weeks. Exclusion criteria included patients with a history of antibiotic or probiotic use within 3 months, severe infection, systemic diseases (i.e., malignancy, autoimmune diseases, liver or heart diseases), recent surgeries, urinary tract obstruction, pregnancy, participation in other clinical trials within 3 months, known allergies to investigational medications or other inappropriate conditions.
The sample size of the intention-to-treat patients was calculated based on efficiency of YSHS (27.5% in the intention group; 23.3% in the control group) with an error level of 0.05 and 90% statistical power. Considering the potential dropout rate (10%), the number of patients enrolled in each group was increased to 60. Randomization was performed by an external statistical consultant not related to this study using a computer-generated random sequence. Eligible patients were randomized in a 1:1 ratio to receive regular RAAS inhibitors (control group) or combined with YSHS free of charge (intervention group, one bag three times daily) for 4 months. YSHS granule have been approved by the State Food and Drug Administration of China. According to the guidelines, the main side-effect and risk was explained to the patients, including thirsty, and bitter taste. Patients were also suggested to have a light diet. In addition, each participant was given 100 yuan as the transportation compensation. All subjects gave their informed written consent to participate in this study, which was conducted following the Declaration of Helsinki. The Xuanwu Hospital Medical Ethic Committee approved this study [No.2020(025)], and the protocol was adhered to during the study. After we obtained IRB approval, we were informed by our IRB that the registration was not necessary and commenced the study without registering this trial. However, once this matter was brought to our attention, we promptly registered with the Chinese Clinical Trial Registry (ChiCTR, number ChiCTR2300076136) as required by this journal. The baseline control group was denoted as C0 and, at the follow-up assessment, as C1. Similarly, the baseline for the intervention group was labeled T0, and at the 4-month follow-up, it was designated T1.
Outcome
We collected clinical information, including baseline characteristics, such as sex, age, CKD stage, weight, and primary renal disease, and laboratory values, such as serum albumin, creatinine, uric acid, urea, and 24-h proteinuria levels. The primary endpoint was the effect of YSHS on changes in 24 h proteinuria, while the secondary endpoint was changes in microbiota composition. During follow-up, 4 patients in the YSHS intervention group withdrew from the study: 1 had acute cerebral infarction, 1 had gastralgia, and 2 could not tolerate the bitter taste of herbs. Thirteen patients in the control group did not complete the study: 7 did not provide a follow-up fecal sample, 1 had acute myocardial infarction, 2 had diarrhea with antibiotic therapy, and 3 were unwilling to continue participating in the research. Finally, 103 patients completed the trial ().
Fecal DNA extraction and 16S rRNA sequencing
Each participant provided approximately 1 g of fresh stool sample in a 5mL sterile tube at baseline and after 4 months of follow-up, which was stored at −80 °C until extraction and sequencing of fecal DNA. Each DNA sample obtained was cleaned with the EZNA® soil DNA Kit (Omega Bio-tek, Norcross, GA, USA) according to the manufacturer’s instructions. The isolated DNA was preserved at −80 °C until it was ready for subsequent analysis. The hypervariable region V3-V4 of the bacterial 16S rRNA gene was amplified with primer pairs 338 F (5′-ACTCCTACGGGAGGCAGCAG-3′) and 806 R (5′-GGACTACHVGGGTWTCTAAT-3′) using an ABI GeneAmp® 9700 PCR thermocycler (ABI, CA).
The PCR amplification cycle conditions were as follows: initial denaturation at 95 °C for 3 min, followed by 27 cycles of denaturation at 95 °C for 30 s, annealing at 55 °C for 30 s, extension at 72 °C for 45 s, and single extension at 72 °C for 10 min, and end at 10 °C. All samples were amplified in triplicate. The PCR product was extracted from 2% agarose gel and purified using the AxyPrep DNA Gel Extraction Kit (Axygen Biosciences, Union City, CA) according to the manufacturer’s instructions and quantified using the Quantus™ Fluorometer (Promega, USA).
Data processing
The purified amplicons were combined in equimolar quantities and subjected to paired-end sequencing on an Illumina MiSeq PE300 platform (Illumina, San Diego, CA), following the standard protocols of Majorbio Bio-Pharm Technology Co. Ltd. (Shanghai, China). The raw FASTQ files were filtered by Fastp (version 0.19.6) and merged by FLASH (version 1.2.7). Optimized sequences were clustered into operational taxonomic units (OTUs) using UPARSE 7.1 with a 97% sequence similarity level. The most abundant sequence for each OTU was selected as a representative sequence. The taxonomy of each representative OTU sequence was analyzed using RDP Classifier version 2.2 against the 16S rRNA gene database (e.g., Silva v138). The metagenomic function was predicted by PICRUSt2 (Phylogenetic Investigation of Communities by Reconstruction of Unobserved States) based on OTU representative sequences. The work was registered under the NCBI Bioproject PRJNA888345.
Statistical analysis
Data are expressed as the mean and standard deviation for the normally distributed or median and interquartile range for the non-normally distributed data, respectively. Blood chemistry tests and statistical taxonomic differences were compared using either Student’s t-test for normally distributed data or Mann-Whitney test for not normally distributed data between groups. Bioinformatic gut microbiota analysis was carried out using the Majorbio Cloud platform (https://cloud.majorbio.com). Based on the OTUs information, rarefaction curves and alpha diversity indices, including observed OTUs, the Sob index, and the Shannon index were calculated with Mothur v1.30.1. The principal coordinate analysis (PCoA) based on Bray-Curtis dissimilarity using the Vegan v2.5-3 package determined the similarity between the microbial communities in different samples. Linear discriminant analysis (LDA) effect size (LEfSe) (http://huttenhower.sph.harvard.edu/LEfSe) was performed to identify the significantly abundant taxa of bacteria between groups (LDA score > 2, p < 0.05). The Spearman coefficient shows the correlation between gut taxa and clinical parameters. A co-occurrence correlation network analysis was also performed by Networkx. Metagenome functional predictive analysis was performed based on reference genomes using the Kyoto Encyclopedia of Genes and Genomes (KEGG) with PICRUSt2 software version 2.3.0. For all analyses, p < 0.05 was considered statistically significant.
Results
Characteristics of the participant
shows the baseline characteristics of the participants. Patients were randomly assigned to the intervention or control group, and the biochemical indices of each group were compared. The use of losartan was similar in both the intervention and control groups (54/56 vs. 45/47). The intervention group had a higher level of decreased 24-h proteinuria than the control group.
Table 1. Baseline characteristics and follow-up data of the cohort.
shows the subgroup analysis based on gender. The results clarified that YSHS granule could significantly reduced 24-h proteinuria in both male and female patients.
Table 2. Baseline characteristics and follow-up data of the cohort stratified by gender.
Gut microbial diversity
The Pan curve () shows that the richness and evenness of the OTUs nearly approached saturation as the number of samples increased. The Sobs estimator was used to calculate the community richness, while the Shannon calculator reflected the community diversity for OTU definition. As shown in , no significant differences were observed between baseline and 4-month follow-up in either the control or intervention groups. PCoA analysis was performed to investigate potential differences due to Bray-Curtis dissimilarity. Beta-diversity analysis showed a non-significant divergence between enrollment and follow-up at 4 months.
Figure 2. (A) The rarefaction analysis between the number of samples and the number of OTUs. (B–C) Phylogenetic diversity of the gut microbiome between patients in the control and YSHS group at baseline (C0, T0) and at 4-month follow-up (C1, T1) according to the Sobs and Shannon index. (D) Principal Coordinate Analysis (PCoA) of the gut microbiota of patients in the control group at baseline (C0) and at 4-month follow-up (C1). (E) Principal Coordinates Analysis (PCoA) of the gut microbiota of patients in the YSHS group at baseline (T0) and 4-month follow-up (T1).
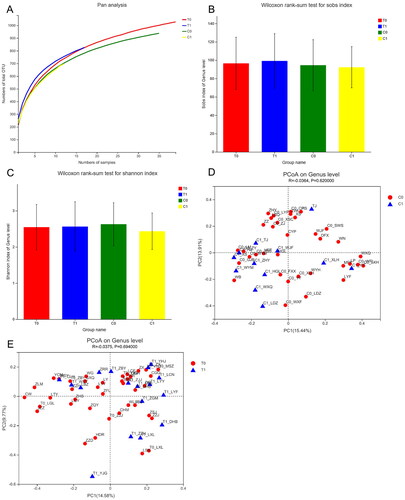
Gut microbiome profile
As shown in the Venn diagrams (), the total abundance of OTUs was 290 for the control group and 300 for the intervention group. The C0 and C1 groups shared 207 OTUs, while T0 and T1 shared 217 OTUs. The most abundant genera were Bacteroides, Faecalibacterium, Blautia, Bifidobacterium, Megamonas, and Escherichia-Shigella. There was an increase in Bacteroides, Faecalibacterium, Megamonas, and Escherichia-Shigella and a reduction in Bifidobacterium in the intervention group. Based on LEfSe, 26 genera, including Bacteroidia, Faecalibacterium, Lachnoclostridium, and Sutterella, were significantly enriched. In contrast, 15 genera, including the Clostridium_methylpentosum_group, Clostridium_innocuum_group, Saccharimonadales, Turicibacter, and Eggerthella, were significantly reduced in the intervention group at 4 months.
Figure 3. (A) Venn diagram showing the overlaps between the patients in the control group at baseline (C0) and 4-month follow-up (C1). (B) Venn diagram showing the overlaps between the patients in the YSHS group at baseline (T0) and at 4-month follow-up (T1). (C) Relative abundance of the gut microbiota in chronic kidney disease patients in the control and YSHS group at baseline (C0, T0) and 4-month follow-up (C1, T1) at the genus level. (D) Histogram of the LDA scores computed for differentially abundant bacterial taxa between baseline (T0) and 4-month follow-up (T1) in the YSHS group.
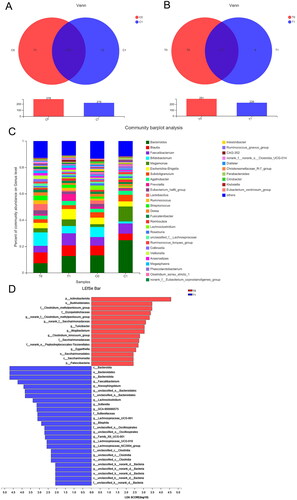
Changes in the gut microbiota in CKD patients during the intervention
At 4 months of follow-up in the intervention group, based on the genus level, the relative abundance of Faecalibacterium, Lachnoclostridium, Lachnospiraceae and Sutterella increased significantly, while the relative abundance of the Eggerthella and Clostridium innocuum group decreased significantly (). Meanwhile, there was a significant increase in Bacteroides and Lachnospira at the genus level in the control group. When comparing microbiota changes between the two groups at follow-up, the Lachnospira and Terrisporobacter genera were relatively enriched in the control group (supplementary file). To further demonstrate the microbial co-occurrence, we constructed the network correlation analysis at the genus level of the T1 and C1 groups. Compared to genera in C1 (N for edges= 219), the genera in T1 (N for edges= 226) had a stronger correlation (supplementary file). In addition, the supplemental figures also show the relative abundance of taxa between the two groups based on species level.
Figure 4. (A) Relative abundance of taxa between patients in the control group at baseline (C0) and at 4-month follow-up (C1). (B) Relative abundance of taxa between patients in the YSHS group at baseline (T0) and at 4-month follow-up (T1). (C) Heatmaps showing correlations between differentially abundant microbiota genera and clinical parameters in chronic kidney disease patients treated with YSHS.
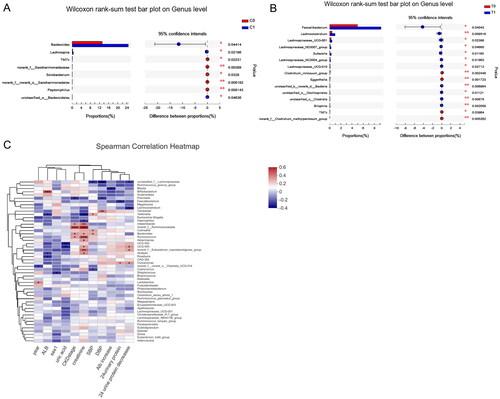
Changes in the gut microbiota in CKD patients during the intervention stratified by gender
The effect of YSHS granule on the gut flora in male and female patients was demonstrated in the supplementary material. In the intervention group, YSHS granule significantly increased the abundance of Lachnoclostridium and Lachnospiraceae in male CKD patients, and Faecalibacterium and Prevotella in female patients at genus level. After 4-month follow-up, YSHS group significantly increased the abundance of Ruminococcus genera in male patients, and Prevotella genera in female patients when compared to the control group.
Correlation between the gut microbiota and clinical parameters
The redundancy table analysis (RDA) shows several clinical factors influence on the gut microbiome compositional variation. In the intervention group, the relationship between baseline 24-h proteinuria (R2 = 0.22, p = 0.007), the decrease in 24-h proteinuria (R2 = 0.34, p = 0.001), and the gut microbiome composition was significant. According to the heatmap (), many genera were positively and negatively correlated with various clinical indices in the intervention group. Lachnoclostridium and Lachnospiraceae were negatively associated with the decrease in 24-h proteinuria. In contrast, Comamonas was positively correlated with both baseline and reduction in 24-h proteinuria. Meanwhile, baseline serum creatinine showed a significant negative correlation with Veillonella and Haemophilus and a significant positive correlation with Ruminococcus, Bacteroides, Intestinibacter, and Alistipes. However, we did not find a correlation between any decrease in gut flora and 24-h proteinuria in the control group (supplementary file).
Correlation between differential metabolites and the gut microbiota
The KEGG ID of the metabolites was used for the pathway enrichment analysis, while significant differences are shown in . The intervention group was more likely to be involved in glycan biosynthesis and metabolism and cofactor and vitamin metabolisms in the KEGG pathways based on level 2. In contrast, the control group was involved in cell growth, death and glycan biosynthesis and metabolism (supplementary file). Meanwhile, the intervention group was involved in various types of N-glycan biosynthesis, steroid hormone biosynthesis, glycosaminoglycan degradation, riboflavin metabolism, and biotin metabolism in KEGG pathways based on level 3. Interactions between the gut microbiota and metabolites were investigated using Spearman correlation analysis. Lachnoclostridium was positively related to riboflavin and biotin metabolism, while Lachnospiraceae were positively associated with N-glycan biosynthesis, steroid hormone biosynthesis, and glycosaminoglycan degradation.
Discussion
This RCT is the first to investigate the effect of the YSHS granule on intestinal microbiota diversity and proteinuria in patients with early-stage CKD (consisting of diabetic nephropathy, membranous nephropathy, and IgA nephropathy). Our results showed that YSHS granules could reduce proteinuria by altering the diversity of the gut bacterial community. We found that the YSHS intervention significantly increased the abundance of Faecalibacterium, Lachnoclostridium, Lachnospiraceae, and Sutterella, and decreased the quantity of Clostridium_innocuum_group and Eggerthella in CKD patients without dialysis. Meanwhile, YSHS might ameliorate proteinuria by various bacterial taxa, such as Lachnoclostridium, Lachnospiraceae, and Comamonas.
Proteinuria plays a critical role in the diagnosis of the severity of CKD. Persistently elevated albuminuria indicates the presence of kidney damage (Kidney Disease: Improving Global Outcomes (KDIGO) Diabetes Work Group 2020). Albuminuria is associated with the development of kidney disease (Bakris and Molitch Citation2014) and increased rate of hospitalizations encompassing circulatory, endocrine, genitourinary, respiratory, and injury-related issues (Barzilay et al. Citation2020). Effective strategies to ameliorate proteinuria are primarily experimental. Gut dysbiosis has been linked to various diseases, including colon cancer (Tilg et al. Citation2018; Song et al. Citation2020), inflammatory bowel disease (Dong et al. Citation2022), CKD (Yang et al. Citation2018), autoimmune diseases (Christovich and Luo Citation2022), type 2 diabetes (Liu et al. Citation2022; Lv et al. Citation2022) and cardiovascular diseases (Madan and Mehra Citation2020).
In recent years, the role of the gut microbiome in CKD has been gradually explored. It is generally accepted that Bacteroidetes (abundant genera: Bacteroides, Parabacteroides, Alistipes, and Prevotella) and Firmicutes (abundant genera: Eubacterium, Clostridium, and Ruminococcus) comprise approximately 90% of the intestinal microbiome (Van Treuren and Dodd Citation2020). The microbiota bridges the gut-kidney axis, involving the intestinal barrier, inflammation, immunity, and metabolism (Huang et al. Citation2022). An increase in intestinal pH due to urea secretion in the gastrointestinal tract could change the composition of bacterial flora in CKD patients (Vaziri et al. Citation2016). Gut dysbiosis could regulate the release of toxins, the degradation of complex polysaccharides, the fermentation of amino acids, and the production of short-chain fatty acids (SCFAs). Pro-toxins originating from altered microbial metabolism, such as indoles, phenols, and trimethylamine, have been explored. Phenols and indoles metabolize to p-cresyl sulfate and indoxyl sulfate, which can negatively affect endothelial function (Huang et al. Citation2020). Phenyl sulfate could also contribute to albuminuria in patients with diabetic kidney disease (Kikuchi et al. Citation2019). Trimethylamine is a product of the metabolism of l-carnitine and choline by commensal bacteria and subsequently converts to trimethylamine-N-oxide (TMAO) by liver flavin monooxygenases (Xu et al. Citation2017). TMAO could contribute to endothelial dysfunction, atherosclerosis (Seldin et al. Citation2016), renal fibrosis, and renal tubular injury (Li et al. Citation2019) through inflammasome activation and mitogen-activated protein kinase induction. An elevated TMAO level also predicted an increased risk of major adverse cardiovascular events (Tang et al. Citation2013) and mortality (Tang et al. Citation2015).
The YSHS granule is made up of 16 herbs, as mentioned above. Seven potentially bioactive components were detected in YSHS, such as calycosin-7-O-β-d-glucoside, 6-gingerol, naringin, ginsenoside Re, liquiritigenin, isoliquiritigenin, and pocirin. It has been shown to preserve the integrity of the glomerular filtration barrier through the PI3K/AKT/mTOR signaling pathway in diabetic nephropathy (Zhao et al. Citation2022).
However, the definitive effect of YSHS on the gut microbiota or proteinuria is unclear. Our study showed that the decrease in 24-h proteinuria was significantly higher in the YSHS intervention group, which was also correlated with the alteration of the gut microbiota. Bacteroidetes were the most abundant phyla that comprised most of the gut microbiota in this study. We also identified Megamonas, Faecalibacterium, and Blautia genus enrichment in microbiota samples from our CKD patients. Bacteroides have previously been reported to be significantly more abundant in patients on hemodialysis and peritoneal dialysis (Shivani et al. Citation2022). A previous study showed that the abundance of Ruminococcus, Bacteroides, Eggerthella, and Clostridium innocuum was enriched in CKD patients (Lun et al. Citation2019).
The LEfSe analysis found that Bacteroides, Faecalibacterium, Lachnoclostridium, and Sutterella were increased after treatment with YSHS. However, the abundance of the Clostridium_methylpentosum_group, Clostridium_innocuum_group, and Eggerthella declined in CKD patients treated with YSHS. Eggerthella, which belongs to the Coriobacteriia class, was found to produce the T helper 17 (Th17) cytokine and increase intestinal permeability (Balakrishnan et al. Citation2019). It could also activate the proinflammatory cytokine interleukin (IL)-17 pathways to induce tumorigenesis (Alexander et al. Citation2022). Eggerthella species appeared to be associated with various diseases, such as frailty (Jackson et al. Citation2016) and gastrointestinal disease (Thota et al. Citation2011). Anaerobic commensals, such as Clostridium, could generate phenols that the tubules eliminated. Clostridium lipopolysaccharide-like structure was reported to be higher in atherosclerotic patients compared to healthy people (Cai et al. Citation2022). Although Sutterella could adhere to intestinal epithelial cells and induce an inflammatory reaction, the relative abundance of Sutterella was higher in the intervention group at 4 months of follow-up. Sutterella is markedly prevalent in healthy cohorts compared to end-stage renal disease patients (Vaziri et al. Citation2013). The role of Sutterella in proteinuria and outcome in CKD has yet to be determined.
Our finding confirmed the potential protective effect of YSHS in reducing proteinuria associated with the alleviation of flora disorder in CKD patients. Lachnospira and Terrisporobacter were relatively enriched in patients without YSHS compared to YSHS treatment for 4 months. However, the association of flora and a 24-h decrease in proteinuria was weak in the control group. When the key differential microorganisms were searched after YSHS, Faecalibacterium, Lachnospiraceae, and Lachnoclostridium were observed to increase significantly. In contrast, the Clostridium_innocuum_group and Eggerthella decreased. Faecalibacterium is considered to be a marker of a healthy intestine. Bacteroides and Faecalibacterium could metabolize various carbohydrate substrates to create SCFAs (Yang et al. Citation2018). SCFAs could be involved in the maintenance of intestinal homeostasis by inhibiting the production of proinflammatory cytokines (McDermott and Huffnagle Citation2014), promoting cell reparation, and protecting intestinal barrier function (Donohoe et al. Citation2012; Kelly et al. Citation2015). A decrease in SCFAs by producing Faecalibacterium might contribute to CKD progression (Jiang et al. Citation2016). Lachnospiraceae were more abundant in healthy individuals than in patients with immune thrombocytopenia (Yu et al. Citation2022) and lung cancer (Chen et al. Citation2022).
Furthermore, Lachnospira decreased in CKD patients with idiopathic nephrotic syndrome (Zhang et al. Citation2020). A higher number of OTUs from Lachnospiraceae were found in subjects with recurrent kidney stones (Suryavanshi et al. Citation2016). Lachnoclostridium was also relatively enriched in diabetes patients without gastrointestinal autonomic neuropathy, which may be involved in carotenoid and flavonoid biosynthesis (Du et al. Citation2021). SCFAs produced by Lachnoclostridium and Lachnospiraceae could regulate intestinal homeostasis by promoting the production of IL-10 in Th1 cells (Sun et al. Citation2018). In another study, the abundance of Lachnoclostridium was lower in colorectal cancer patients with a higher Treg density, which was correlated with poor clinical outcomes (Oshi et al. Citation2022). Several inflammatory responses were involved, such as interferon (IFN)-γ response, IFN-α response, and IL6/JAK/STAT signaling. The pathogenesis of proteinuria involves a complex interaction between the immune system, inflammation factors, the glomerular microenvironment, and the genome. Subgroup analysis for the effect of YSHS on proteinuria and gut flora was also performed based on gender. YSHS could ameliorate proteinuria in both male and female patients. The changes of gut flora in male patients were similar to our main results. In our female patients, we found that Prevotella is significantly enriched after 4-month treatment of YSHS. The abundance of Prevotellaceae families is decreased in CKD (Vaziri et al. Citation2013). Prevotella was positively correlated with hemoglobin, albumin and eGFR, but negatively correlated with 24-h proteinuria (Zhang et al. Citation2023). The SCFAs produced by Prevotella might play a protective role in regulating CKD patient homeostasis (Liu et al. Citation2022). The differences of gut flora in male and female might be due to sex hormones (Dominianni et al. Citation2015). The abundance of the Prevotella genera is lower in pre-menopausal women than in post-menopausal women (Santos-Marcos et al. Citation2018). A relatively lower abundance of the Prevotella genera was also observed in women with metabolic syndrome than in men (Santos-Marcos et al. Citation2019).
Microbial networks may reflect disease-specific microenvironments. In our study, the correlation network of the T1 group was more complex than that of the C1 group, suggesting a potentially beneficial effect of the intestinal flora to improve proteinuria. As shown in the heatmap, Lachnoclostridium and Lachnospiraceae were significantly correlated with a decrease in 24-h proteinuria, probably involving various inflammatory or immune system mechanisms. A previous study suggested an enrichment of Megamonas among overweight and obese Asian individuals (Chen et al. Citation2020), which were regarded as potential pathogens for cardiovascular disease, along with Bacteroides (Liu et al. Citation2019). Meanwhile, Bacteroides fragilis could regulate the subsets of CD4+ and CD8+ T cells and immunosuppressive cytokines to suppress inflammation (Ramakrishna et al. Citation2019). However, the association between the composition of such taxa and proteinuria was weak in this study, and the definite mechanism of these altered bacterial taxa by YSHS to prevent proteinuria warrants further investigation.
To determine the effect of YSHS, we also analyzed gene expression between T0 and T1 (i.e., at baseline and the follow-up of the intervention group). Among the KEGG pathways significantly enriched after YSHS treatment are those involved in N-glycan biosynthesis, steroid hormone biosynthesis, glycosaminoglycan degradation, riboflavin, and biotin metabolism. Interestingly, the genus Lachnoclostridium and Lachnospiraceae, which were relatively enriched after applying YSHS for 4 months, were positively related to such KEGG pathways in the correlation analysis. YSHS granules might alter the gut flora to regulate glycan, lipid, and vitamin metabolism to reduce proteinuria. The definite mechanism needs further investigation.
Some limitations of this study should be acknowledged. The small sample size could have led to the volatility of the data. The duration of the study may be too short to see a stable effect on proteinuria. The diversity of the gut microbiome in the two groups would be very similar. Larger sample sizes and the latest database were preferred to detect significant variations in the gut microbiome in these groups. We did not consider the effects of diet intake, other inflammation factors, and various medications on the microbiome. We could not draw a definitive causal relationship between YSHS therapy that decreases proteinuria and the regulation of intestinal flora in CKD patients.
Conclusions
Our findings suggest a protective effect of YSHS on alleviating proteinuria in CKD patients, presumably caused by several microbial taxa. More studies with a larger sample size are needed to confirm these findings and establish the causal mechanism of the YSHS granule in CKD.
Authors’ contribution
A.H.Z. contributed to the study concept and design; J.L.Z, X.T.D, W.L, Y.P.L, L.P.J, Z.H.L, W.J.F contributed to data collection; J.L.Z, X.T.D contributed to the statistical analysis; J.L.Z, X.T.D, A.H.Z contributed to the original draft. J.L.Z and X.T.D contributed equally. All authors approved the final version of the manuscript.
Ethical approval
The study was conducted following the guidelines stated in the Declaration of Helsinki. All patients gave their written informed consent to the protocol, which was approved by the Medical Ethics Committee of Xuanwu Hospital, Capital Medical University guidelines. This project was approved by the Xuanwu Hospital Medical Ethic Committee [No.2020(025)].
Supplemental Material
Download PDF (1 MB)Disclosure statement
No potential conflict of interest was reported by the author(s).
Data availability statement
The data described in the manuscript will be made available upon reasonable request.
Additional information
Funding
References
- Alexander M, Ang QY, Nayak RR, Bustion AE, Sandy M, Zhang B, Upadhyay V, Pollard KS, Lynch SV, Turnbaugh PJ. 2022. Human gut bacterial metabolism drives Th17 activation and colitis. Cell Host Microbe. 30(1):17–30.e9. doi:10.1016/j.chom.2021.11.001.
- Bakris GL, Molitch M. 2014. Microalbuminuria as a risk predictor in diabetes: the continuing saga. Diabetes Care. 37(3):867–875. doi:10.2337/dc13-1870.
- Balakrishnan B, Luckey D, Taneja V. 2019. Autoimmunity-associated gut commensals modulate gut permeability and immunity in humanized mice. Mil Med. 184(Suppl 1):529–536. doi:10.1093/milmed/usy309.
- Barzilay JI, Buzkova P, Shlipak MG, Bansal N, Garimella P, Mukamal KJ. 2020. Hospitalization rates in older adults with albuminuria: the Cardiovascular Health Study. J Gerontol A Biol Sci Med Sci. 75(12):2426–2433. doi:10.1093/gerona/glaa020.
- Cai YY, Huang FQ, Lao X, Lu Y, Gao X, Alolga RN, Yin K, Zhou X, Wang Y, Liu B, et al. 2022. Integrated metagenomics identifies a crucial role for trimethylamine-producing Lachnoclostridium in promoting atherosclerosis. NPJ Biofilms Microbiomes. 8(1):11. doi:10.1038/s41522-022-00273-4.
- Chen S, Gui R, Zhou XH, Zhang JH, Jiang HY, Liu HT, Fu YF. 2022. Combined microbiome and metabolome analysis reveals a novel interplay between intestinal flora and serum metabolites in lung cancer. Front Cell Infect Microbiol. 12:885093. doi:10.3389/fcimb.2022.885093.
- Chen X, Sun H, Jiang F, Shen Y, Li X, Hu X, Shen X, Wei P. 2020. Alteration of the gut microbiota associated with childhood obesity by 16S rRNA gene sequencing. PeerJ. 8:e8317. doi:10.7717/peerj.8317.
- Christovich A, Luo XM. 2022. Gut microbiota, leaky gut, and autoimmune diseases. Front Immunol. 13:946248. doi:10.3389/fimmu.2022.946248.
- Dominianni C, Sinha R, Goedert JJ, Pei Z, Yang L, Hayes RB, Ahn J. 2015. Sex, body mass index, and dietary fiber intake influence the human gut microbiome. PLoS One. 10(4):e0124599. doi:10.1371/journal.pone.0124599.
- Dong Y, Xu T, Xiao G, Hu Z, Chen J. 2022. Opportunities and challenges for synthetic biology in the therapy of inflammatory bowel disease. Front Bioeng Biotechnol. 10:909591. doi:10.3389/fbioe.2022.909591.
- Donohoe DR, Collins LB, Wali A, Bigler R, Sun W, Bultman SJ. 2012. The Warburg effect dictates the mechanism of butyrate-mediated histone acetylation and cell proliferation. Mol Cell. 48(4):612–626. doi:10.1016/j.molcel.2012.08.033.
- Du Y, Neng Q, Li Y, Kang Y, Guo L, Huang X, Chen M, Yang F, Hong J, Zhou S, et al. 2021. Gastrointestinal autonomic neuropathy exacerbates gut microbiota dysbiosis in adult patients with type 2 diabetes mellitus. Front Cell Infect Microbiol. 11:804733. doi:10.3389/fcimb.2021.804733.
- Felizardo RJ, Castoldi A, Andrade-Oliveira V, Câmara NO. 2016. The microbiota and chronic kidney diseases: a double-edged sword. Clin Transl Immunol. 5(6):e86.
- GBD Chronic Kidney Disease Collaboration. 2020. Global, regional, and national burden of chronic kidney disease, 1990-2017: a systematic analysis for the Global Burden of Disease Study 2017. Lancet. 395(10225):709–733.
- Huang Y, Xin W, Xiong J, Yao M, Zhang B, Zhao J. 2022. The intestinal microbiota and metabolites in the gut-kidney-heart axis of chronic kidney disease. Front Pharmacol. 13:837500. doi:10.3389/fphar.2022.837500.
- Huang Y, Zhou J, Wang S, Xiong J, Chen Y, Liu Y, Xiao T, Li Y, He T, Li Y, et al. 2020. Indoxyl sulfate induces intestinal barrier injury through IRF1-DRP1 axis-mediated mitophagy impairment. Theranostics. 10(16):7384–7400. doi:10.7150/thno.45455.
- Ichii O, Otsuka-Kanazawa S, Nakamura T, Ueno M, Kon Y, Chen W, Rosenberg AZ, Kopp JB. 2014. Podocyte injury caused by indoxyl sulfate, a uremic toxin and aryl-hydrocarbon receptor ligand. PLoS One. 9(9):e108448. doi:10.1371/journal.pone.0108448.
- Jackson MA, Jeffery IB, Beaumont M, Bell JT, Clark AG, Ley RE, O’Toole PW, Spector TD, Steves CJ. 2016. Signatures of early frailty in the gut microbiota. Genome Med. 8(1):8. doi:10.1186/s13073-016-0262-7.
- Jha V, Garcia-Garcia G, Iseki K, Li Z, Naicker S, Plattner B, Saran R, Wang AY, Yang CW. 2013. Chronic kidney disease: global dimension and perspectives. Lancet. 382(9888):260–272. doi:10.1016/S0140-6736(13)60687-X.
- Jiang S, Xie S, Lv D, Zhang Y, Deng J, Zeng L, Chen Y. 2016. A reduction in the butyrate producing species Roseburia spp. and Faecalibacterium prausnitzii is associated with chronic kidney disease progression. Antonie Van Leeuwenhoek. 109(10):1389–1396. doi:10.1007/s10482-016-0737-y.
- Josefsdottir KS, Baldridge MT, Kadmon CS, King KY. 2017. Antibiotics impair murine hematopoiesis by depleting the intestinal microbiota. Blood. 129(6):729–739. doi:10.1182/blood-2016-03-708594.
- Karbach SH, Schönfelder T, Brandão I, Wilms E, Hörmann N, Jäckel S, Schüler R, Finger S, Knorr M, Lagrange J, et al. 2016. Gut microbiota promote angiotensin ii-induced arterial hypertension and vascular dysfunction. J Am Heart Assoc. 5(9):e003698. doi: 10.1161/JAHA.116.003698.
- Kelly CJ, Zheng L, Campbell EL, Saeedi B, Scholz CC, Bayless AJ, Wilson KE, Glover LE, Kominsky DJ, Magnuson A, et al. 2015. Crosstalk between microbiota-derived short-chain fatty acids and intestinal epithelial HIF augments tissue barrier function. Cell Host Microbe. 17(5):662–671. doi:10.1016/j.chom.2015.03.005.
- Kelly JR, Keane VO, Cryan JF, Clarke G, Dinan TG. 2019. Mood and microbes: gut to brain communication in depression. Gastroenterol Clin North Am. 48(3):389–405. doi:10.1016/j.gtc.2019.04.006.
- Kidney Disease: Improving Global Outcomes (KDIGO) Diabetes Work Group. 2020. KDIGO 2020 clinical practice guideline for diabetes management in chronic kidney disease. Kidney Int. 98(4s):S1–s115.
- Kikuchi K, Saigusa D, Kanemitsu Y, Matsumoto Y, Thanai P, Suzuki N, Mise K, Yamaguchi H, Nakamura T, Asaji K, et al. 2019. Gut microbiome-derived phenyl sulfate contributes to albuminuria in diabetic kidney disease. Nat Commun. 10(1):1835. doi:10.1038/s41467-019-09735-4.
- Li W, Tan L, Li X, Zhang X, Wu X, Chen H, Hu L, Wang X, Luo X, Wang F, et al. 2019. Identification of a p.Trp403* nonsense variant in PHEX causing X-linked hypophosphatemia by inhibiting p38 MAPK signaling. Hum Mutat. 40(7):879–885.
- Liang M, Zhu X, Zhang D, He W, Zhang J, Yuan S, He Q, Jin J. 2022. Yi-Shen-Hua-Shi granules inhibit diabetic nephropathy by ameliorating podocyte injury induced by macrophage-derived exosomes. Front Pharmacol. 13:962606. doi:10.3389/fphar.2022.962606.
- Liu F, Sheng J, Hu L, Zhang B, Guo W, Wang Y, Gu Y, Jiang P, Lin H, Lydia B, et al. 2022. Salivary microbiome in chronic kidney disease: what is its connection to diabetes, hypertension, and immunity? J Transl Med. 20(1):387. doi:10.1186/s12967-022-03602-5.
- Liu W, Luo Z, Zhou J, Sun B. 2022. Gut microbiota and antidiabetic drugs: perspectives of personalized treatment in type 2 diabetes mellitus. Front Cell Infect Microbiol. 12:853771. doi:10.3389/fcimb.2022.853771.
- Liu Z, Li J, Liu H, Tang Y, Zhan Q, Lai W, Ao L, Meng X, Ren H, Xu D, et al. 2019. The intestinal microbiota associated with cardiac valve calcification differs from that of coronary artery disease. Atherosclerosis. 284:121–128. doi:10.1016/j.atherosclerosis.2018.11.038.
- Lun H, Yang W, Zhao S, Jiang M, Xu M, Liu F, Wang Y. 2019. Altered gut microbiota and microbial biomarkers associated with chronic kidney disease. Microbiologyopen. 8(4):e00678. doi:10.1002/mbo3.678.
- Lv Q, Li Z, Sui A, Yang X, Han Y, Yao R. 2022. The role and mechanisms of gut microbiota in diabetic nephropathy, diabetic retinopathy and cardiovascular diseases. Front Microbiol. 13:977187. doi:10.3389/fmicb.2022.977187.
- Madan S, Mehra MR. 2020. Gut dysbiosis and heart failure: navigating the universe within. Eur J Heart Fail. 22(4):629–637. doi:10.1002/ejhf.1792.
- McDermott AJ, Huffnagle GB. 2014. The microbiome and regulation of mucosal immunity. Immunology. 142(1):24–31. doi:10.1111/imm.12231.
- Oshi M, Sarkar J, Wu R, Tokumaru Y, Yan L, Nakagawa K, Ishibe A, Matsuyama R, Endo I, Takabe K. 2022. Intratumoral density of regulatory T cells is a predictor of host immune response and chemotherapy response in colorectal cancer. Am J Cancer Res. 12(2):490–503.
- Ramakrishna C, Kujawski M, Chu H, Li L, Mazmanian SK, Cantin EM. 2019. Bacteroides fragilis polysaccharide A induces IL-10 secreting B and T cells that prevent viral encephalitis. Nat Commun. 10(1):2153. doi:10.1038/s41467-019-09884-6.
- Rinninella E, Raoul P, Cintoni M, Franceschi F, Miggiano GAD, Gasbarrini A, Mele MC. 2019. What is the healthy gut microbiota composition? A changing ecosystem across age, environment, diet, and diseases. Microorganisms. 7(1):14. doi:10.3390/microorganisms7010014.
- Rooks MG, Garrett WS. 2016. Gut microbiota, metabolites and host immunity. Nat Rev Immunol. 16(6):341–352. doi:10.1038/nri.2016.42.
- Santos-Marcos JA, Haro C, Vega-Rojas A, Alcala-Diaz JF, Molina-Abril H, Leon-Acuña A, Lopez-Moreno J, Landa BB, Tena-Sempere M, Perez-Martinez P, et al. 2019. Sex differences in the gut microbiota as potential determinants of gender predisposition to disease. Mol Nutr Food Res. 63(7):e1800870.
- Santos-Marcos JA, Rangel-Zuñiga OA, Jimenez-Lucena R, Quintana-Navarro GM, Garcia-Carpintero S, Malagon MM, Landa BB, Tena-Sempere M, Perez-Martinez P, Lopez-Miranda J, et al. 2018. Influence of gender and menopausal status on gut microbiota. Maturitas. 116:43–53. doi:10.1016/j.maturitas.2018.07.008.
- Seldin MM, Meng Y, Qi H, Zhu W, Wang Z, Hazen SL, Lusis AJ, Shih DM. 2016. Trimethylamine N-oxide promotes vascular inflammation through signaling of mitogen-activated protein kinase and nuclear factor-κB. J Am Heart Assoc. 5(2):e002767. doi:10.1161/JAHA.115.002767.
- Shivani S, Kao CY, Chattopadhyay A, Chen JW, Lai LC, Lin WH, Lu TP, Huang IH, Tsai MH, Teng CH, et al. 2022. Uremic toxin-producing bacteroides species prevail in the gut microbiota of Taiwanese CKD patients: an analysis using the new Taiwan microbiome baseline. Front Cell Infect Microbiol. 12:726256. doi:10.3389/fcimb.2022.726256.
- Song M, Chan AT, Sun J. 2020. Influence of the gut microbiome, diet, and environment on risk of colorectal cancer. Gastroenterology. 158(2):322–340. doi:10.1053/j.gastro.2019.06.048.
- Su WY, Wu PY, Huang JC, Chen SC, Chang JM. 2020. Increased proteinuria is associated with increased aortic arch calcification, cardio-thoracic ratio, rapid renal progression and increased overall and cardiovascular mortality in chronic kidney disease. Int J Med Sci. 17(8):1102–1111. doi:10.7150/ijms.45470.
- Sun M, Wu W, Chen L, Yang W, Huang X, Ma C, Chen F, Xiao Y, Zhao Y, Ma C, et al. 2018. Microbiota-derived short-chain fatty acids promote Th1 cell IL-10 production to maintain intestinal homeostasis. Nat Commun. 9(1):3555. doi:10.1038/s41467-018-05901-2.
- Suryavanshi MV, Bhute SS, Jadhav SD, Bhatia MS, Gune RP, Shouche YS. 2016. Hyperoxaluria leads to dysbiosis and drives selective enrichment of oxalate metabolizing bacterial species in recurrent kidney stone endures. Sci Rep. 6(1):34712. doi:10.1038/srep34712.
- Tang WH, Wang Z, Kennedy DJ, Wu Y, Buffa JA, Agatisa-Boyle B, Li XS, Levison BS, Hazen SL. 2015. Gut microbiota-dependent trimethylamine N-oxide (TMAO) pathway contributes to both development of renal insufficiency and mortality risk in chronic kidney disease. Circ Res. 116(3):448–455. doi:10.1161/CIRCRESAHA.116.305360.
- Tang WH, Wang Z, Levison BS, Koeth RA, Britt EB, Fu X, Wu Y, Hazen SL. 2013. Intestinal microbial metabolism of phosphatidylcholine and cardiovascular risk. N Engl J Med. 368(17):1575–1584. doi:10.1056/NEJMoa1109400.
- Thota VR, Dacha S, Natarajan A, Nerad J. 2011. Eggerthella lenta bacteremia in a Crohn’s disease patient after ileocecal resection. Future Microbiol. 6(5):595–597. doi:10.2217/fmb.11.31.
- Tilg H, Adolph TE, Gerner RR, Moschen AR. 2018. The intestinal microbiota in colorectal cancer. Cancer Cell. 33(6):954–964. doi:10.1016/j.ccell.2018.03.004.
- Van Treuren W, Dodd D. 2020. Microbial contribution to the human metabolome: implications for health and disease. Annu Rev Pathol. 15(1):345–369. doi:10.1146/annurev-pathol-020117-043559.
- Vaziri ND, Wong J, Pahl M, Piceno YM, Yuan J, DeSantis TZ, Ni Z, Nguyen TH, Andersen GL. 2013. Chronic kidney disease alters intestinal microbial flora. Kidney Int. 83(2):308–315. doi:10.1038/ki.2012.345.
- Vaziri ND, Zhao YY, Pahl MV. 2016. Altered intestinal microbial flora and impaired epithelial barrier structure and function in CKD: the nature, mechanisms, consequences and potential treatment. Nephrol Dial Transplant. 31(5):737–746. doi:10.1093/ndt/gfv095.
- Wong J, Piceno YM, DeSantis TZ, Pahl M, Andersen GL, Vaziri ND. 2014. Expansion of urease- and uricase-containing, indole- and p-cresol-forming and contraction of short-chain fatty acid-producing intestinal microbiota in ESRD. Am J Nephrol. 39(3):230–237. doi:10.1159/000360010.
- Xu KY, Xia GH, Lu JQ, Chen MX, Zhen X, Wang S, You C, Nie J, Zhou HW, Yin J. 2017. Impaired renal function and dysbiosis of gut microbiota contribute to increased trimethylamine-N-oxide in chronic kidney disease patients. Sci Rep. 7(1):1445. doi:10.1038/s41598-017-01387-y.
- Yang T, Richards EM, Pepine CJ, Raizada MK. 2018. The gut microbiota and the brain-gut-kidney axis in hypertension and chronic kidney disease. Nat Rev Nephrol. 14(7):442–456. doi:10.1038/s41581-018-0018-2.
- Yu X, Zheng Q, He Y, Yu D, Chang G, Chen C, Bi L, Lv J, Zhao M, Lin X, et al. 2022. Associations of gut microbiota and fatty metabolism with immune thrombocytopenia. Front Med (Lausanne). 9:810612. doi:10.3389/fmed.2022.810612.
- Zaky A, Glastras SJ, Wong MYW, Pollock CA, Saad S. 2021. The role of the gut microbiome in diabetes and obesity-related kidney disease. Int J Mol Sci. 22(17):9641. doi:10.3390/ijms22179641.
- Zhang J, Luo D, Lin Z, Zhou W, Rao J, Li Y, Wu J, Peng H, Lou T. 2020. Dysbiosis of gut microbiota in adult idiopathic membranous nephropathy with nephrotic syndrome. Microb Pathog. 147:104359. doi:10.1016/j.micpath.2020.104359.
- Zhang L, Lu QY, Wu H, Cheng YL, Kang J, Xu ZG. 2023. The intestinal microbiota composition in early and late stages of diabetic kidney disease. Microbiol Spectr. 11(4):e0038223. doi:10.1128/spectrum.00382-23.
- Zhang L, Wang F, Wang L, Wang W, Liu B, Liu J, Chen M, He Q, Liao Y, Yu X, et al. 2012. Prevalence of chronic kidney disease in China: a cross-sectional survey. Lancet. 379(9818):815–822. doi:10.1016/S0140-6736(12)60033-6.
- Zhao J, Chan YC, He B, Duan TT, Yu ZL. 2019. A patent herbal drug Yi-Shen-Hua-Shi granule ameliorates C-BSA-induced chronic glomerulonephritis and inhabits TGFβ signaling in rats. J Ethnopharmacol. 236:258–262. doi:10.1016/j.jep.2019.02.044.
- Zhao T, Li M, Xiang Q, Lie B, Chen D, Wang W, Li X, Xu T, Zhang X, Li Y, et al. 2022. Yishen Huashi granules ameliorated the development of diabetic nephropathy by reducing the damage of glomerular filtration barrier. Front Pharmacol. 13:872940. doi:10.3389/fphar.2022.872940.