Abstract
Context
Tabersonine has been investigated for its role in modulating inflammation-associated pathways in various diseases. However, its regulatory effects on triple-negative breast cancer (TNBC) have not yet been fully elucidated.
Objective
This study uncovers the anticancer properties of tabersonine in TNBC cells, elucidating its role in enhancing chemosensitivity to cisplatin (CDDP).
Materials and methods
After tabersonine (10 μM) and/or CDDP (10 μM) treatment for 48 h in BT549 and MDA-MB-231 cells, cell proliferation was evaluated using the cell counting kit-8 and colony formation assays. Quantitative proteomics, online prediction tools and molecular docking analyses were used to identify potential downstream targets of tabersonine. Transwell and wound-healing assays and Western blot analysis were used to assess epithelial–mesenchymal transition (EMT) phenotypes.
Results
Tabersonine demonstrated inhibitory effects on TNBC cells, with IC50 values at 48 h being 18.1 μM for BT549 and 27.0 μM for MDA-MB-231. The combined treatment of CDDP and tabersonine synergistically suppressed cell proliferation in BT549 and MDA-MB-231 cells. Enrichment analysis revealed that the proteins differentially regulated by tabersonine were involved in EMT-related signalling pathways. This combination treatment also effectively restricted EMT-related phenotypes. Through the integration of online target prediction and proteomic analysis, Aurora kinase A (AURKA) was identified as a potential downstream target of tabersonine. AURKA expression was reduced in TNBC cells post-treatment with tabersonine.
Discussion and conclusions
Tabersonine significantly enhances the chemosensitivity of CDDP in TNBC cells, underscoring its potential as a promising therapeutic agent for TNBC treatment.
Introduction
Breast cancer (BC), the most common cancer among women, is considered a crucial global public health concern (Nedeljkovic and Damjanovic Citation2019). Triple-negative BC (TNBC), an aggressive subtype of BC, accounts for approximately 15–20% of all BC cases. TNBC is characterized by the absence of oestrogen receptor (ER), progesterone receptor (PR) and human epidermal growth factor receptor 2 (HER2) expression, which limits the effectiveness of targeted therapies (Yin et al. Citation2020). Although cytotoxic chemotherapy is the main treatment for TNBC, patients with this subtype experience a higher mortality rate of 40% (Bou Zerdan et al. Citation2022). The considerable heterogeneity of TNBC cells often leads to inherent resistance to chemotherapeutic agents, posing a significant obstacle to managing this cancer (Deepak et al. Citation2020). Consequently, it is imperative to identify innovative approaches to improve TNBC treatment outcomes.
Cisplatin (CDDP), one of the first metal-based chemotherapeutic drugs, is widely applied in treating multiple cancers (Ghosh Citation2019). CDDP exerts its cytotoxic effects on tumour cells by inducing the DNA damage response and subsequently triggering apoptosis (Chen and Chang Citation2019). In TNBC, CDDP has been reported to induce pyroptosis by activating the maternally expressed gene 3 (MEG3)/NOD-like receptor family pyrin domain-containing 3 (NLRP3)/caspase-1/gasdermin D (GSDMD) pathway, thus exerting antitumor effects (Yan et al. Citation2021). CDDP-based combination chemotherapy can also improve therapeutic efficacy in TNBC patients (Lin et al. Citation2022). A randomized phase 3 trial has shown that CDDP combined with nab-paclitaxel significantly prolongs progression-free survival of TNBC patients (Wang B et al. Citation2022). However, the emergence of drug resistance and undesirable side effects has been observed in TNBC patients undergoing CDDP treatment (Yu et al. Citation2020). Consequently, there is an urgent need to identify innovative strategies that improve CDDP sensitivity in TNBC.
Recently, researchers have increasingly focused on investigating the potential of natural products in treating BC. Tabersonine, a terpene indole alkaloid derived from the medicinal plant Catharanthus roseus (Linn.) G. Don (Apocynaceae) has received growing interest due to its bioactive properties in regulating inflammatory diseases. For example, tabersonine has been found to inhibit the activation of the nuclear factor-κB (NF-κB) signalling pathway, resulting in the amelioration of obesity-induced renal injury (Qian et al. Citation2023). Tabersonine has shown efficacy in mitigating inflammation-associated cardiomyopathy by binding directly to transforming growth factor beta-activated kinase 1 (TAK1) and inhibiting phosphorylation (Chen, Lin, Y et al. 2023). Furthermore, tabersonine has been reported to serve as an intermediate in the synthesis of vindoline (Liu T et al. Citation2021), indicating its potential to generate antitumor activity. However, the specific effects of tabersonine on TNBC progression need to be elucidated.
This study identified tabersonine as a promising antitumor compound that enhanced the sensitivity of TNBC cells to CDDP. Using quantitative proteomics and bioinformatic analysis, it was discovered that a combination of tabersonine and CDDP effectively inhibited cell invasion and migration by inhibiting the epithelial–mesenchymal transition (EMT) process. Furthermore, our findings indicate that tabersonine exerts its inhibitory effects by down-regulating the expression of Aurora kinase A (AURKA) in TNBC cells. These findings suggest that tabersonine, a potential antitumor agent, has the potential to overcome CDDP resistance in TNBC therapy.
Materials and methods
Bioinformatics analyses
To identify potential tabersonine targets, the open-access PharmMapper Server (http://lilab.ecust.edu.cn/pharmmapper/) was used to predict binding potentials (Liu X et al. Citation2010). Subsequently, the online UALCAN database tool (https://ualcan.path.uab.edu/) (Chandrashekar et al. Citation2022) was used to validate AURKA expression in normal and BC samples, as well as in various BC subtypes. The association between AURKA expression and platinum sensitivity was investigated using data sets from GSE21653, GSE22219, GSE97342 and TCGA_BRCA, accessed through the Biomarker Exploration of Solid Tumours (BEST) database (https://rookieutopia.com/app_direct/BEST/) (Chen, Yan, X et al. 2023). To further assess the impact of AURKA expression on prognosis post-chemotherapy, the Kaplan–Meier plotter (https://kmplot.com/analysis/) was used to analyse distant-metastasis-free survival (DMFS) and relapse-free survival (RFS) in TNBC patients (Gyorffy Citation2021). The STRING database (https://string-db.org/) (Gyorffy Citation2021) was used to construct protein association networks of AURKA with EMT-related genes.
Cells and reagents
The human TNBC cell lines BT549 (CVCL_1092, CX0068) and MDA-MB-231 (CVCL_0062, CL-0150B) were obtained from the Center for Molecular Medicine of the Xiangya Hospital. The BT549 cell line was cultured in 1640 medium (Gibco, C11875500BT, Carlsbad, CA), while the MDA-MB-231 cell line was maintained in Dulbecco’s modified eagle medium (DMEM, Corning, 10-013-CV, NY). Culture medium was supplemented with 10% foetal bovine serum (Gibco, 10091-148) and 1% penicillin-streptomycin (Gibco, 15140-122). Tabersonine (Selleckchem, S9427, Houston, TX) and CDDP (APExBIO, A8321, Houston, TX) were respectively dissolved in dimethylsulfoxide (DMSO) to prepare 10 mM stock solutions.
Cell counting kit 8 assay
For cytotoxic analysis, the BT549 and MDA-MB-231 cell lines were seeded in 96-well plates at a density of 2 × 10³ cells per well, using complete medium. Various concentrations of tabersonine (0, 1, 5, 10, 20, 40 and 80 μM) and CDDP (0, 1, 5, 10, 20, 40 and 80 μM) were added to the wells and incubated for 48 h. To examine the effects of these agents on cell proliferation, cells were treated with 10 μM of CDDP, 10 μM of tabersonine, or a combination of both and incubated for 48 h. For cell viability assays, CCK-8 reagent (Bimake, B34304, Houston, TX) was added to each well (10 µL/well) and incubated for 2 h. The optical density (OD) at 450 nm was measured using a VICTOR X2 microplate reader (PerkinElmer, Waltham, MA). The half-maximal inhibitory concentration (IC50) values were calculated using GraphPad Prism version 8.0 software (La Jolla, CA), employing a variable slope analysis.
Colony formation assay
BT549 and MDA-MB-231 cells were seeded in 6-well plates at a density of 1 × 10³ cells per well and treated with the indicated drugs (tabersonine and/or CDDP) at concentrations of 10 μM for 48 h. Subsequently, the medium containing the drugs was replaced with a fresh complete medium, and the cells were further incubated at 37 °C for approximately 14 d. After incubation, colonies were fixed with methanol and stained with 0.3% w/v crystal violet at room temperature for 25 min. Colonies consisting of a cluster of 50 or more cells were counted.
Quantitative proteomics analysis
Label-free 4D quantitative proteomics was conducted by Majorbio (Shanghai, China) to determine alterations in protein expression in TNBC cells after tabersonine treatment. For quantitative proteomic analysis, BT549 and MDA-MB-231 cells (5 × 106 cells) were pretreated with 10 μM tabersonine for 24 h. Subsequently, cells were collected, immediately snap-frozen in liquid nitrogen, and stored at −80 °C. Total protein extraction, peptide desalination, and quantification were performed using the technology platform provided by Majorbio. The bioinformatic analysis of the proteomic data was then conducted using the Xiantao database (https://www.xiantaozi.com/).
Western-blot analysis
Proteins were extracted from cells using radioimmunoprecipitation assay (RIPA, Sidbio, SDP0001, China) lysis buffer containing a 1% fresh protease inhibitor cocktail (Selleckchem, B14001). Equal amounts of protein extracts (50 μg per well) from each sample were separated by SDS-PAGE and transferred to polyvinylidene fluoride (PVDF) membranes (Millipore, Billerica, MA). The membranes were blocked with 5% skim milk for 1 h at room temperature. Following blocking, membranes were incubated overnight at 4 °C with primary antibodies: anti-AURKA (human, 1:1,000 dilution, RRID_AB_2882103, 66757-1-Ig, Proteintech, Rosemont, IL), anti-E-cadherin (human, 1:1,000 dilution, RRID_AB_10697811, 20874-1-AP, Proteintech), anti-N-cadherin (human, 1:1,000 dilution, RRID_AB_2813891, 22018-1-AP, Proteintech), anti-Vimentin (human, 1:3,000 dilution, RRID_AB_2273020, 10366-1-AP, Proteintech) and anti-β-actin (human, 1:20,000 dilution, RRID_AB_2687938, 66009-1-Ig, Proteintech). The membranes were then washed with 1× Tris-buffered saline containing 1% Tween 20 and incubated with horseradish peroxidase-conjugated secondary antibodies (antimouse: human, 1:3,000 dilution, RRID_AB_2722565, SA00001-1, Proteintech; anti-rabbit: human, 1:3,000 dilution, RRID_AB_2722564, SA00001-2, Proteintech) for 1 h at room temperature. Finally, immunoblots were visualized using an enhanced chemiluminescence detection kit (34580, Thermo Scientific, Waltham, MA). β-actin was used as a loading control to normalize protein levels.
Transwell assay
To assess the invasive capacity of BT549 and MDA-MB-231 cells, they were first pretreated with 10 μM CDDP and/or 10 μM tabersonine for 48 h. The upper chamber of the Transwell apparatus (8.0 μm pore size; Corning, 3422) was precoated with Matrigel (Corning, 354234). Cells (1 × 105) were then seeded in the upper chamber in 200 µL of serum-free medium, while 600 μL of DMEM/1640 supplemented with 10% FBS was added to the bottom reservoir. Following a 24 h incubation at 37 °C, cells that invaded the membrane to the lower surface were fixed with 4% paraformaldehyde for 30 min and stained with 0.1% crystal violet for 5 min. Invading cells were observed and captured under a microscope in randomly selected visual fields.
Wounding-healing assay
BT549 and MDA-MB-231 cells were cultured in 6-well plates and treated with CDDP and/or tabersonine for 48 h. The wounds were introduced into the cell monolayers using a 200 μL sterile pipette tip. Cells were washed twice with PBS to remove detached cells and debris. Cell migration was monitored, and the migration distance was investigated using a microscope. The area of cell migration, indicative of wound closure, was quantified as a percentage using ImageJ software.
Molecular docking analysis
SwissDock tool (http://www.swissdock.ch/) Molecular Modelling Group, Switzerland), MOE 2019 software (Chemical Computing Group, Canada) and UCSF Chimera version 1.16 software (UCSF Resource, USA) were used to predict interactions between tabersonine and AURKA or TAK1. The molecular structure of tabersonine was initially retrieved from the PubChem database (https://pubchem.ncbi.nlm.nih.gov/). The crystal structures of the AURKA protein (PDB IDs: 2X6D, 2J50, 2X6E) and the tabersonine binding protein (PDB ID: 4GS6) were obtained from the RCSB Protein Data Bank (http://www.rcsb.org/) and subsequently pre-processed using MOE software. To assess binding affinity, we individually uploaded the tabersonine ligands and protein files to SwissDock for molecular docking. The optimal interaction model was selected based on the lowest Delta G value. These models were visualized using the UCSF Chimera version 1.16 software. Two-dimensional (2D) interaction predictions were performed using MOE 2019 software (Molecular Operating Environment, version 2019).
Statistical analysis
All statistical analyses were performed with GraphPad Prism version 8 and SPSS version 23.0 software (SPSS, Chicago, IL). Group comparisons were conducted using one-way ANOVA or Student’s t-test, as appropriate. Continuous data are presented as means ± standard deviation (SD). Statistical significance was established at the following levels: *p < 0.05; **p < 0.01.
Results
Tabersonine increases the chemosensitivity of CDDP in TNBC cells
To investigate the effects of tabersonine on cell growth, TNBC cells were treated with various concentrations of tabersonine for 48 h. shows that CDDP IC50 values were 29.4 and 39.5 μM in BT549 and MDA-MB-231 cells, respectively. Furthermore, the tabersonine IC50 values in the BT549 and MDA-MB-231 cells were 18.1 and 27.0 μM, respectively (). Subsequent experiments used 10 μM of CDDP and 10 μM of tabersonine, based on these IC50 values, as these concentrations only inhibited cell growth slightly. The proliferation of TNBC cells was further assessed under combined treatment with these two agents. Combining CDDP and tabersonine significantly inhibited BT549 and MDA-MB-231 cell proliferation compared to treatment with CDDP or tabersonine alone (). Additionally, colony formation assays were conducted to corroborate the combined effects on cell growth. The results revealed that the combined treatment of CDDP and tabersonine synergistically suppressed TNBC cell growth (). These results suggest that tabersonine enhances CDDP-mediated inhibition of cell viability in TNBC cells.
Figure 1. Tabersonine increases CDDP sensitivity in TNBC cells. (A,B) CCK-8 assays were used to detect IC50 values in BT549 and MDA-MB-231 cells after incubation with CDDP for 48 h. (C,D) IC50 values of tabersonine in BT549 and MDA-MB-231 cells, after treatment for 48 h. (E,F) BT549 and MDA-MB-231 cells were treated with 10 μM CDDP, 10 μM tabersonine or (10 μM CDDP + 10 μM tabersonine), followed by the measurement of cell viability using CCK-8 assays. (G,H) The colony formation potential was determined in BT549 and MDA-MB-231 cells after treatment with 10 μM CDDP, 10 μM tabersonine or (10 μM CDDP + 10 μM tabersonine). **p < 0.01. Tab: tabersonine; CDDP: cisplatin.
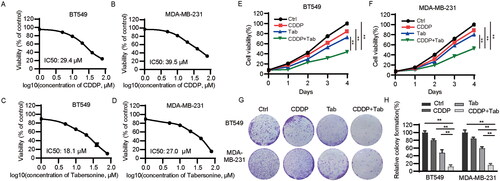
Quantitative proteomic analysis based on tabersonine-treated TNBC cells
To investigate significant protein alterations after tabersonine treatment, the TNBC cell lines BT549 and MDA-MB-231 were used for proteome profiling. Differentially expressed proteins (DEPs) were identified using volcano plots, applying a 1.2-fold change (FC) threshold and a p value < 0.05. In total, 1021 and 1046 DEPs were identified in the tabersonine-untreated and -treated groups, respectively, for BT549 and MDA-MB-231 cells (). Among these, 502 proteins were up-regulated and 519 were down-regulated in BT549 cells. In MDA-MB-231 cells, 596 proteins were up-regulated and 450 were down-regulated.
Figure 2. Quantitative proteomic analysis demonstrates differentially expressed proteins (DEPs) and enrichment analysis in tabersonine-treated TNBC cells. (A,B) The volcano plots illustrated DEPs between tabersonine-untreated and treated (10 μM) TNBC cells (BT549 and MDA-MB-231). (C,D) GO and KEGG enrichment analysis of 10 main pathways in BT549 and MDA-MB-231 cells.
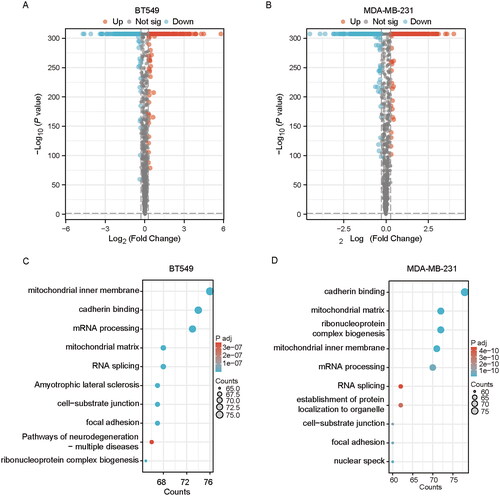
To elucidate the biological functions of these DEPs, enrichment analysis of the Gene Ontology (GO) and Kyoto Encyclopedia of Genes and Genomes (KEGG) pathways was performed using the Xiantao database. illustrates the top 10 enriched pathways, revealing that DEPs were predominantly associated with epithelial EMT-related pathways, such as cadherin binding, cell-substrate junction and focal adhesion. Previous studies have reported that EMT contributes to CDDP chemoresistance by transforming stationary epithelial cells into migratory mesenchymal cells, thus modulating adhesive functions (Ashrafizadeh et al. Citation2020). These proteomic findings suggest that tabersonine may exert its inhibitory effects by affecting EMT in TNBC cells.
Combined treatment of tabersonine and CDDP inhibits EMT in TNBC cells
To further validate whether tabersonine exerts antitumor effects and enhances the chemosensitivity of CDDP by modulating EMT, we conducted Transwell assays. These assays demonstrated that tabersonine significantly reduced cell invasion in BT549 and MDA-MB-231 cells (). The combination treatment with CDDP and tabersonine further strengthened this inhibitory effect, resulting in more than 80% reduction in cell invasiveness. We also evaluated the impact of these agents on cell migration using wound-healing assays. Although tabersonine alone effectively reduced migration in TNBC cells, combined treatment with CDDP and tabersonine resulted in a more substantial suppression of migration capacity than either agent alone in both cell lines, as demonstrated in .
Figure 3. Tabersonine increases CDDP sensitivity by inhibiting EMT in TNBC cells. (A,B) The Transwell assay demonstrates cell invasive ability in BT549 and MDA-MB-231 cells after treatment of 10 μM CDDP, 10 μM tabersonine or (10 μM CDDP + 10 μM tabersonine). (C–E) Wound healing assays of BT549 and MDA-MB-231 cells that were treated with 10 μM CDDP, 10 μM tabersonine or (10 μM CDDP + 10 μM tabersonine). (F) Western blot examined the expression of EMT-related proteins, including E-cadherin, N-cadherin and Vimentin, in BT549 and MDA-MB-231, after treatment of 10 μM CDDP, 10 μM tabersonine or (10 μM CDDP + 10 μM tabersonine). **p < 0.01. Tab: tabersonine; CDDP: cisplatin.
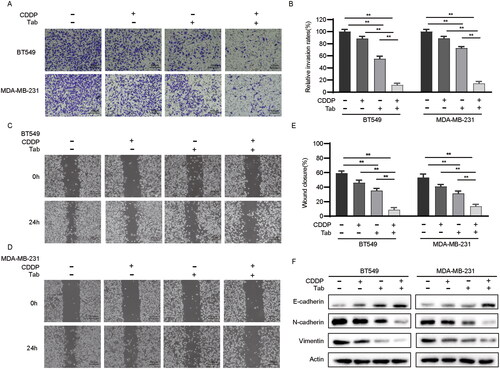
Several expressional changes in epithelial and mesenchymal markers commonly occur when tumour cells undergo EMT (Serrano-Gomez et al. Citation2016). Therefore, we examined the expression of EMT-related proteins in BT549 and MDA-MB-231 cells using Western blotting. The results revealed an up-regulation of the epithelial marker E-cadherin and a decrease in the mesenchymal markers N-cadherin and Vimentin (). These findings suggest that tabersonine may enhance the sensitivity of TNBC cells to CDDP by suppressing EMT phenotypes.
AURKA is identified as a potential target for tabersonine and is associated with chemotherapeutic sensitivity in TNBC
To identify potential targets of tabersonine, an integrated analysis was performed using the PharmMapper Server database and quantitative proteomics. This analysis identified 84 potential tabersonine targets (Norm Fit > 0.5). These predicted targets were then cross-referenced with tabersonine-regulated DEPs from quantitative proteomic analysis. As shown in , five co-DEPs were identified: 3 up-regulated (MAPK14, GSK3B and AKR1C3) and 2 down-regulated (AURKA and NQO1). MAPK14 and AURKA were selected for further investigation due to their more pronounced expression differences. However, the existing literature suggests that MAPK14 may promote BC progression (Wu X et al. Citation2014; Canovas et al. Citation2018), a finding contrary to our results. Consequently, we focused on AURKA for subsequent analyses.
Figure 4. AURKA is a potential target of tabersonine in TNBC. (A,B) Venn diagram to exhibit the intersection between predicted targets (from PharmMapper Server) and DEPs (from quantitative proteomic analysis). (C) The heatmap showing the expression of five overlapping proteins in BT549 and MDA-MB-231 cells. (D–E) Comparison of AURKA transcriptional expression between BC and control tissues (D), as well as in different subtypes of BC (E). (F–I) Scatter plots of the association between AURKA expression and platinum IC50 values in the BC (GSE21653, GSE22219 and TCGA_BRCA) and TNBC (GSE97342) datasets. (J-K) Kaplan–Meier plotter revealed the association of AURKA expression with DMFS and RFS in TNBC patients receiving chemotherapy. (L) PPI network of AURKA and EMT-related genes, performed by the STRING database. **p < 0.01.
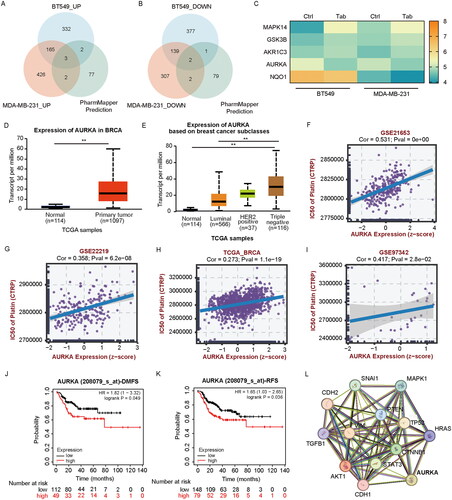
Further exploration using the UALCAN database revealed a higher transcriptional expression of AURKA in BC tissues than in normal samples, with elevated TNBC tissue levels (). The correlation between AURKA expression and platinum sensitivity was analysed using the BEST database, showing a positive association with platinum IC50 values in BC (GSE21653, GSE22219, TCGA_BRCA; p < 0.05) and TNBC data sets (GSE97342; p < 0.05) ().
Kaplan–Meier plotter analysis indicated that high AURKA expression was correlated with poorer DMFS and RFS in TNBC patients undergoing chemotherapy (). Previous research has highlighted the critical role of AURKA in regulating migration and invasion by promoting EMT in various cancers (Liu X et al. Citation2016; Chen C et al. Citation2017). We further analysed AURKA and EMT-related genes using the STRING database, building a protein–protein interaction (PPI) network as shown in . These findings suggest that AURKA may be a potential target for tabersonine and could be positively correlated with platinum resistance in TNBC.
The modulation of tabersonine on AURKA expression in TNBC cells
To investigate how tabersonine regulates AURKA, we assessed the expression of AURKA protein in TNBC cells treated with varying concentrations of tabersonine. Tabersonine treatment led to a significant decrease in AURKA expression in BT549 and MDA-MB-231 cells (). Additionally, a molecular docking study was conducted to evaluate the potential interactions between AURKA and tabersonine, using the SwissDock tool. Given previous reports of tabersonine interaction with TAK1, identified through a biotinylated compound–protein interaction pull-down assay (Chen, Lin, Y et al. 2023), TAK1 was used as a positive control in this docking prediction.
Figure 5. Combined experimental analysis and molecular docking assays identify the relationship between tabersonine and AURKA. (A) Expression of AURKA in BT549 and MDA-MB-231 cells incubated with a specified concentration of tabersonine (0, 5, 10 and 20 μM). (B–E) the 3D structure of AURKA (PDB: 2X6D; PDB: 2J50; PDB: 2X6E) and TAK1 (positive control, PDB: 4GS6), downloaded from the PDB database. (F–I) Predictions of tabersonine binding to AURKA or TAK1. (J–L) The interaction map (2D) between tabersonine and AURKA (PDB: 2X6D; PDB: 2J50; PDB: 2X6E).
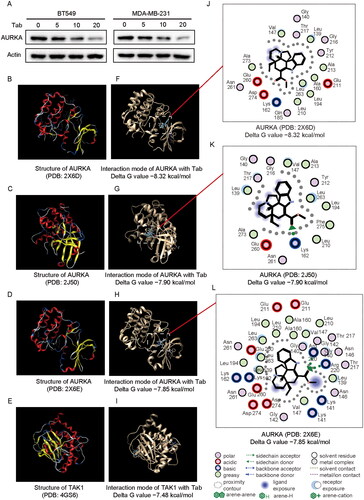
The 3D structures of TAK1 (PDB: 4GS6) and AURKA (PDB: 2X6D, 2J50, 2X6E) were retrieved from the PDB database (Fancelli et al. Citation2006; Bavetsias et al. Citation2010; Wu J et al. Citation2013) and are shown in . The molecular docking results, including the interaction modes of tabersonine with TAK1 and AURKA, are presented in . The 2D interaction map indicated potential interactions of tabersonine with AURKA at amino acid residues such as Val 147, Leu 139 and Arg 220 (). Furthermore, the binding free energies were evaluated by negative Delta G values. The binding prediction results revealed that the lowest Delta G values for the interaction between tabersonine and AURKA were −8.32 kcal/mol (PDB: 2X6D), −7.90 kcal/mol (PDB: 2J50) and −7.85 kcal/mol (PDB: 2X6E). In comparison, the lowest Delta G value for the interaction between tabersonine and TAK1 was −7.48 kcal/mol (PDB: 4GS6). These findings suggest that tabersonine suppresses AURKA expression in TNBC cells and implies a potential binding affinity between tabersonine and AURKA.
Discussion
Tabersonine, mainly extracted from Catharanthus, is widely used as a biosynthetic precursor in the synthesis of several alkaloids, including vindoline, a notable chemotherapeutic agent for treating various tumours (Zhang et al. Citation2018; Lemos Cruz et al. Citation2021). In addition to its role in chemotherapy, multiple studies have highlighted the anti-inflammatory properties of tabersonine in various diseases such as liver injury (Zhang et al. Citation2018), osteoporosis (Sun et al. Citation2020) and atherosclerosis (Shi et al. Citation2020). However, the specific mechanisms of the tumour-inhibitory effects of tabersonine require further elucidation. In this study, we have demonstrated significant antitumor properties of tabersonine in two TNBC cell lines. When combined with CDDP, tabersonine effectively reduced cell proliferation, invasion and migration, primarily by inhibiting EMT phenotypes in these TNBC cells. These findings suggest that tabersonine can enhance CDDP sensitivity, underscoring its potential for further exploration in cancer treatment.
EMT promotes metastasis and drug resistance in various cancer types (Xu et al. Citation2022). Research has shown that EMT contributes to CDDP resistance in BC, a process regulated by the focal adhesion kinase (FAK)/phosphatidylinositol 3-kinase (PI3K)/AKT signalling pathway activated by 14,15-epoxyeicosatrienoic acid (Luo et al. Citation2018). Similarly, Rab31 is known to mediate CDDP resistance and metastasis in stomach adenocarcinoma through Twist1-induced EMT (Chen, Xu, K et al. 2023). Various studies have identified antitumor compounds that can reverse EMT phenotypes, potentially leading to reduced tumour invasion and increased chemosensitivity (Hashemi et al. Citation2022). Our study used quantitative proteomic analysis to identify DEPs regulated by tabersonine, followed by functional enrichment analysis.
These DEPs were predominantly associated with EMT-related pathways. Experimental validation confirmed that tabersonine, when combined with CDDP, synergistically inhibited EMT phenotypes in TNBC cells. These findings further underscore the importance of targeting EMT to enhance CDDP sensitivity in TNBC.
We propose that tabersonine may exert its antitumour effects on TNBC by modulating the activity of AURKA. AURKA belongs to the serine/threonine kinase family and is critical to coordinate cell division by regulating mitotic processes. Increasing evidence supports the oncogenic role of AURKA, particularly its role in facilitating EMT and chemoresistance to CDDP in various malignancies (Du et al. Citation2021). For example, a study reported that AURKA overexpression promotes EMT via the PI3K/AKT pathway in hepatocellular carcinoma (Chen et al. Citation2017). AURKA has also been shown to induce TGF-β-mediated chemoresistance by enhancing SNAIL expression in TNBC cells, with both TGF-β and SNAIL being key regulators of EMT (Jalalirad et al. Citation2021). Pharmacological targeting of TGF-β and AURKA simultaneously has increased sensitivity to docetaxel in vitro and inhibited tumour relapse and metastasis in vivo (Jalalirad et al. Citation2021).
AURKA knockdown in CDDP-resistant gastric cancer cells reduced cell proliferation and was associated with down-regulation of p-eIF4E, HDM2 and c-MYC expression (Wang L et al. Citation2017). In our study, an analysis of the BEST database observed a significant positive correlation between CDDP resistance and AURKA expression. Furthermore, the high expression of AURKA was correlated with poorer survival outcomes in TNBC patients receiving chemotherapy, as shown by Kaplan–Meier plotter analysis. These findings indicate that targeting AURKA with tabersonine may be a promising strategy to enhance antitumor therapy and overcome CDDP resistance in TNBC.
The multifaceted oncogenic role of AURKA has sparked considerable interest in developing inhibitors targeting this kinase. Recent studies have highlighted a growing array of natural products capable of down-regulating AURKA (Huang et al. Citation2022). For example, tanshinone has been shown to inhibit cell proliferation and induce apoptosis in non-small cell lung cancer by down-regulating AURKA (Liu X et al. Citation2020). Similarly, curcumin has demonstrated the ability to decrease AURKA activity, enhancing chemosensitivity to CDDP in TNBC cells (Ke et al. Citation2014). Our research contributes to this field by confirming a significant decrease in AURKA expression in TNBC cells after treatment with tabersonine. Additionally, our molecular docking assays have provided insight into the binding mode of tabersonine with AURKA, indicating a potential binding affinity. These findings suggest that tabersonine treatment could be a viable strategy against TNBC through its modulation of AURKA.
Our findings indicate that tabersonine may increase sensitivity to CDDP by inhibiting EMT and down-regulating AURKA expression in TNBC cells. However, further research is essential to overcome certain limitations. First, while our in vitro models have shown the antitumor properties of tabersonine, its efficacy in in vivo models remains to be investigated. Additionally, considering the critical role of AURKA in regulating EMT and chemoresistance in various cancers (Chen C et al. Citation2017; Dawei et al. Citation2018), a more detailed investigation of how AURKA-mediated EMT is affected after tabersonine treatment is necessary. Furthermore, the possibility that tabersonine affects EMT through other significant regulators remains to be explored. Totally, these findings underscore the potential of natural small molecules in cancer therapy and offer a novel approach to enhance the therapeutic efficacy of CDDP in TNBC cells.
Conclusions
Our study demonstrates that tabersonine significantly inhibits cell proliferation, invasion and migration in TNBC cells. Tabersonine increases the sensitivity of TNBC cells to CDDP by suppressing the EMT phenotypes. Through target prediction and experimental validation, tabersonine has been identified as a regulator that decreases the expression of the oncogenic protein AURKA in TNBC. Molecular docking assays have elucidated the binding interaction between tabersonine and AURKA. These findings position tabersonine as a potential therapeutic inhibitor in TNBC treatment and suggest its use in a promising combination therapy strategy for TNBC.
Author’s contributions
Zhijie Xu conceived and designed the study. Xi Chen performed the experiments and wrote the manuscript. Yuanliang Yan, Yuanhong Liu, and Qiaoli Yi performed data analysis. All authors read and approved the final manuscript.
Disclosure statement
The authors declare no conflicts of interest.
Data availability statement
The data sets used and/or analysed during this study are available from the corresponding author on reasonable request.
Additional information
Funding
References
- Ashrafizadeh M, Zarrabi A, Hushmandi K, Kalantari M, Mohammadinejad R, Javaheri T, Sethi G. 2020. Association of the epithelial-mesenchymal transition (EMT) with cisplatin resistance. Int J Mol Sci. 21(11):4002. doi: 10.3390/ijms21114002.
- Bavetsias V, Large JM, Sun C, Bouloc N, Kosmopoulou M, Matteucci M, Wilsher NE, Martins V, Reynisson J, Atrash B, et al. 2010. Imidazo[4,5-b]pyridine derivatives as inhibitors of Aurora kinases: lead optimization studies toward the identification of an orally bioavailable preclinical development candidate. J Med Chem. 53(14):5213–5228. doi: 10.1021/jm100262j.
- Bou Zerdan M, Ghorayeb T, Saliba F, Allam S, Bou Zerdan M, Yaghi M, Bilani N, Jaafar R, Nahleh Z. 2022. Triple negative breast cancer: updates on classification and treatment in 2021. Cancers (Basel). 14(5):1253. doi: 10.3390/cancers14051253.
- Cánovas B, Igea A, Sartori AA, Gomis RR, Paull TT, Isoda M, Pérez-Montoyo H, Serra V, González-Suárez E, Stracker TH, et al. 2018. Targeting p38alpha increases DNA damage, chromosome instability, and the anti-tumoral response to taxanes in breast cancer cells. Cancer Cell. 33(6):1094–1110 e1098. doi: 10.1016/j.ccell.2018.04.010.
- Chandrashekar DS, Karthikeyan SK, Korla PK, Patel H, Shovon AR, Athar M, Netto GJ, Qin ZS, Kumar S, Manne U, et al. 2022. UALCAN: an update to the integrated cancer data analysis platform. Neoplasia. 25:18–27. doi: 10.1016/j.neo.2022.01.001.
- Chen C, Song G, Xiang J, Zhang H, Zhao S, Zhan Y. 2017. AURKA promotes cancer metastasis by regulating epithelial-mesenchymal transition and cancer stem cell properties in hepatocellular carcinoma. Biochem Biophys Res Commun. 486(2):514–520. doi: 10.1016/j.bbrc.2017.03.075.
- Chen K, Xu J, Tong YL, Yan JF, Pan Y, Wang WJ, Zheng L, Zheng XX, Hu C, Hu X, et al. 2023. Rab31 promotes metastasis and cisplatin resistance in stomach adenocarcinoma through Twist1-mediated EMT. Cell Death Dis. 14(2):115. doi: 10.1038/s41419-023-05596-4.
- Chen SH, Chang JY. 2019. New insights into mechanisms of cisplatin resistance: from tumor cell to microenvironment. Int J Mol Sci. 20(17):4136. doi: 10.3390/ijms20174136.
- Chen X, Yan Y, Liu W, Liu Y, Thakur A, Liang Q, Xu Z. 2023. Targeting RAS guanyl releasing protein 1 promotes T lymphocytes infiltrations and improves anti-programmed death receptor ligand 1 therapy response of triple-negative breast cancer. Clin Transl Med. 13(7):e1335.
- Chen Y, Lin W, Chen P, Ye B, Luo W, Wang X, Huang W, Wu G, Liang G. 2023. Tabersonine alleviates obesity-induced cardiomyopathy by binding to transforming growth factor activated kinase 1 (TAK1) and inhibiting TAK1-mediated inflammation. Phytother Res. 37(3):860–871. doi: 10.1002/ptr.7666.
- Dawei H, Honggang D, Qian W. 2018. AURKA contributes to the progression of oral squamous cell carcinoma (OSCC) through modulating epithelial-to-mesenchymal transition (EMT) and apoptosis via the regulation of ROS. Biochem Biophys Res Commun. 507(1–4):83–90. doi: 10.1016/j.bbrc.2018.10.170.
- Deepak KGK, Vempati R, Nagaraju GP, Dasari VR, S N, Rao DN, Malla RR. 2020. Tumor microenvironment: challenges and opportunities in targeting metastasis of triple negative breast cancer. Pharmacol Res. 153:104683. doi: 10.1016/j.phrs.2020.104683.
- Du R, Huang C, Liu K, Li X, Dong Z. 2021. Targeting AURKA in cancer: molecular mechanisms and opportunities for cancer therapy. Mol Cancer. 20(1):15. doi: 10.1186/s12943-020-01305-3.
- Fancelli D, Moll J, Varasi M, Bravo R, Artico R, Berta D, Bindi S, Cameron A, Candiani I, Cappella P, et al. 2006. 1,4,5,6-Tetrahydropyrrolo[3,4-c]pyrazoles: identification of a potent Aurora kinase inhibitor with a favorable antitumor kinase inhibition profile. J Med Chem. 49(24):7247–7251. doi: 10.1021/jm060897w.
- Ghosh S. 2019. Cisplatin: the first metal based anticancer drug. Bioorg Chem. 88:102925. doi: 10.1016/j.bioorg.2019.102925.
- Gyorffy B. 2021. Survival analysis across the entire transcriptome identifies biomarkers with the highest prognostic power in breast cancer. Comput Struct Biotechnol J. 19:4101–4109.
- Hashemi M, Arani HZ, Orouei S, Fallah S, Ghorbani A, Khaledabadi M, Kakavand A, Tavakolpournegari A, Saebfar H, Heidari H, et al. 2022. EMT mechanism in breast cancer metastasis and drug resistance: revisiting molecular interactions and biological functions. Biomed Pharmacother. 155:113774. doi: 10.1016/j.biopha.2022.113774.
- Huang M, Liu C, Shao Y, Zhou S, Hu G, Yin S, Pu W, Yu H. 2022. Anti-tumor pharmacology of natural products targeting mitosis. Cancer Biol Med. 19(6):774–801. doi: 10.20892/j.issn.2095-3941.2022.0006.
- Jalalirad M, Haddad TC, Salisbury JL, Radisky D, Zhang M, Schroeder M, Tuma A, Leof E, Carter JM, Degnim AC, et al. 2021. Aurora-A kinase oncogenic signaling mediates TGF-beta-induced triple-negative breast cancer plasticity and chemoresistance. Oncogene. 40(14):2509–2523. doi: 10.1038/s41388-021-01711-x.
- Ke CS, Liu HS, Yen CH, Huang GC, Cheng HC, Huang CY, Su CL. 2014. Curcumin-induced Aurora-A suppression not only causes mitotic defect and cell cycle arrest but also alters chemosensitivity to anticancer drugs. J Nutr Biochem. 25(5):526–539. doi: 10.1016/j.jnutbio.2014.01.003.
- Lemos Cruz P, Kulagina N, Guirimand G, De Craene JO, Besseau S, Lanoue A, Oudin A, Giglioli-Guivarc’h N, Papon N, Clastre M, et al. 2021. Optimization of tabersonine methoxylation to increase vindoline precursor synthesis in yeast cell factories. Molecules. 26(12):3596. doi: 10.3390/molecules26123596.
- Lin C, Cui J, Peng Z, Qian K, Wu R, Cheng Y, Yin W. 2022. Efficacy of platinum-based and non-platinum-based drugs on triple-negative breast cancer: meta-analysis. Eur J Med Res. 27(1):201. doi: 10.1186/s40001-022-00839-0.
- Liu T, Huang Y, Jiang L, Dong C, Gou Y, Lian J. 2021. Efficient production of vindoline from tabersonine by metabolically engineered Saccharomyces cerevisiae. Commun Biol. 4(1):1089. doi: 10.1038/s42003-021-02617-w.
- Liu X, Li Z, Song Y, Wang R, Han L, Wang Q, Jiang K, Kang C, Zhang Q. 2016. AURKA induces EMT by regulating histone modification through Wnt/beta-catenin and PI3K/Akt signaling pathway in gastric cancer. Oncotarget. 7(22):33152–33164. doi: 10.18632/oncotarget.8888.
- Liu X, Ouyang S, Yu B, Liu Y, Huang K, Gong J, Zheng S, Li Z, Li H, Jiang H. 2010. PharmMapper server: a web server for potential drug target identification using pharmacophore mapping approach. Nucleic Acids Res. 38:W609–614. doi: 10.1093/nar/gkq300.
- Liu X, Zou H, Zhao Y, Chen H, Liu T, Wu Z, Yang C, Li Q, Li Y. 2020. Tanshinone inhibits NSCLC by downregulating AURKA through Let-7a-5p. Front Genet. 11:838. doi: 10.3389/fgene.2020.00838.
- Luo J, Yao JF, Deng XF, Zheng XD, Jia M, Wang YQ, Huang Y, Zhu JH. 2018. 14, 15-EET induces breast cancer cell EMT and cisplatin resistance by up-regulating integrin alphavbeta3 and activating FAK/PI3K/AKT signaling. J Exp Clin Cancer Res. 37(1):23. doi: 10.1186/s13046-018-0694-6.
- Nedeljkovic M, Damjanovic A. 2019. Mechanisms of chemotherapy resistance in triple-negative breast cancer-how we can rise to the challenge. Cells. 8:957.
- Qian C, Wang J, Lin W, Chen Y, Yang J, Liu M, Sun X, Wu H, Zhang M, Wang Y, et al. 2023. Tabersonine attenuates obesity-induced renal injury via inhibiting NF-kappaB-mediated inflammation. Phytother Res. 37(6):2353–2363. doi: 10.1002/ptr.7756.
- Serrano-Gomez SJ, Maziveyi M, Alahari SK. 2016. Regulation of epithelial-mesenchymal transition through epigenetic and post-translational modifications. Mol Cancer. 15(1):18. doi: 10.1186/s12943-016-0502-x.
- Shi S, Song L, Liu Y, He Y. 2020. Activation of CREB protein with tabersonine attenuates STAT3 during atherosclerosis in apolipoprotein E-deficient mice. Dose Response. 18(1):1559325820912067. doi: 10.1177/1559325820912067.
- Sun X, Gan L, Li N, Sun S, Li N. 2020. Tabersonine ameliorates osteoblast apoptosis in rats with dexamethasone-induced osteoporosis by regulating the Nrf2/ROS/Bax signalling pathway. AMB Express. 10(1):165. doi: 10.1186/s13568-020-01098-0.
- Wang B, Sun T, Zhao Y, Wang S, Zhang J, Wang Z, Teng YE, Cai L, Yan M, Wang X, et al. 2022. A randomized phase 3 trial of gemcitabine or nab-paclitaxel combined with cisplatin as first-line treatment in patients with metastatic triple-negative breast cancer. Nat Commun. 13(1):4025. doi: 10.1038/s41467-022-31704-7.
- Wang L, Arras J, Katsha A, Hamdan S, Belkhiri A, Ecsedy J, El-Rifai W. 2017. Cisplatin-resistant cancer cells are sensitive to Aurora kinase A inhibition by alisertib. Mol Oncol. 11(8):981–995. doi: 10.1002/1878-0261.12066.
- Wu J, Powell F, Larsen NA, Lai Z, Byth KF, Read J, Gu RF, Roth M, Toader D, Saeh JC, et al. 2013. Mechanism and in vitro pharmacology of TAK1 inhibition by (5Z)-7-oxozeaenol. ACS Chem Biol. 8(3):643–650. doi: 10.1021/cb3005897.
- Wu X, Zhang W, Font-Burgada J, Palmer T, Hamil AS, Biswas SK, Poidinger M, Borcherding N, Xie Q, Ellies LG, et al. 2014. Ubiquitin-conjugating enzyme Ubc13 controls breast cancer metastasis through a TAK1-p38 MAP kinase cascade. Proc Natl Acad Sci USA. 111(38):13870–13875. doi: 10.1073/pnas.1414358111.
- Xu Z, Zhang Y, Dai H, Han B. 2022. Epithelial-mesenchymal transition-mediated tumor therapeutic resistance. Molecules. 27(15):4750. doi: 10.3390/molecules27154750.
- Yan H, Luo B, Wu X, Guan F, Yu X, Zhao L, Ke X, Wu J, Yuan J. 2021. Cisplatin induces pyroptosis via activation of MEG3/NLRP3/caspase-1/GSDMD Pathway in triple-negative breast cancer. Int J Biol Sci. 17(10):2606–2621. doi: 10.7150/ijbs.60292.
- Yin L, Duan JJ, Bian XW, Yu SC. 2020. Triple-negative breast cancer molecular subtyping and treatment progress. Breast Cancer Res. 22(1):61. doi: 10.1186/s13058-020-01296-5.
- Yu Z, Cao W, Ren Y, Zhang Q, Liu J. 2020. ATPase copper transporter A, negatively regulated by miR-148a-3p, contributes to cisplatin resistance in breast cancer cells. Clin Transl Med. 10(1):57–73. doi: 10.1002/ctm2.19.
- Zhang D, Li X, Hu Y, Jiang H, Wu Y, Ding Y, Yu K, He H, Xu J, Sun L, et al. 2018. Tabersonine attenuates lipopolysaccharide-induced acute lung injury via suppressing TRAF6 ubiquitination. Biochem Pharmacol. 154:183–192. doi: 10.1016/j.bcp.2018.05.004.