Abstract
Context
The mechanisms of Traditional Chinese Medicine (TCM) Guizhi-Gancao Decoction (GGD) remain unknown.
Objective
This study explores the mechanisms of GGD against cardiac hypertrophy.
Materials and methods
Network pharmacology analysis was carried out to identify the potential targets of GGD. In vivo experiments, C57BL/6J mice were divided into Con, phenylephrine (PE, 10 mg/kg/d), 2-chloroadenosine (CADO, the stable analogue of adenosine, 2 mg/kg/d), GGD (5.4 g/kg/d) and GGD (5.4 g/kg/d) + CGS15943 (a nonselective adenosine receptor antagonist, 4 mg/kg/d). In vitro experiments, primary neonatal rat cardiomyocytes (NRCM) were divided into Con, PE (100 µM), CADO (5 µM), GGD (10−5 g/mL) and GGD (10−5 g/mL) + CGS15943 (5 µM). Ultrasound, H&E and Masson staining, hypertrophic genes expression and cell surface area were conducted to verify the GGD efficacy. Adenosine receptors (ADORs) expression were tested via real-time polymerase chain reaction (PCR), western blotting and immunofluorescence analysis.
Results
Network pharmacology identified ADORs among those of the core targets of GGD. In vitro experiments demonstrated that GGD attenuated PE-induced increased surface area (with an EC50 of 5.484 × 10−6 g/mL). In vivo data shown that GGD attenuated PE-induced ventricular wall thickening. In vitro and in vivo data indicated that GGD alleviated PE-induced hypertrophic gene expression (e.g., ANP, BNP and MYH7/MYH6), A1AR over-expression and A2aAR down-expression. Moreover, CADO exerts effects similar to GGD, whereas CGS15943 eliminated most effects of GGD.
Discussion and conclusions
Our findings suggest the mechanism by which GGD inhibits cardiac hypertrophy, highlighting regulation of ADORs as a potential therapeutic strategy for HF.
Introduction
Cardiac hypertrophy refers to an increase in the mass of individual cardiac muscle cells in response to elevation in the hemodynamic load (Nakamura and Sadoshima Citation2018). Pathological hypertrophy can progress to heart failure (HF), which severely impairs cardiac function and is associated with high rates of morbidity and mortality (Li et al. Citation2018; Monda et al. Citation2021). The prevalence of HF is estimated to be 1–2% globally, affecting 26 million people (Mosterd and Hoes Citation2007; Savarese and Lund Citation2017; Groenewegen et al. Citation2020). The incidence and prevalence of HF increase with age, especially above 50 years of age (Kannel Citation2000). Patients with HF have a 5-year survival rate of <50%, making it one of the leading causes of mortality worldwide (Riehle and Bauersachs Citation2019). Due to ageing of the population, the prevalence of HF is on the rise. Thus, cardiac hypertrophy and HF are severe cardiovascular disorders, necessitating further mechanistic studies and the development of treatment strategies.
Traditional Chinese Medicine (TCM) has long been used as an alternative therapy to treat cardiovascular diseases, including HF. However, there is a dearth of systematic research on the specific mechanisms underlying the clinical therapeutic effects of TCM agents. Recently, network pharmacology has rapidly evolved into a popular and powerful tool in TCM research, aiding in elucidating the mechanisms of action of TCM. It is a novel strategy that can be used to guide and assist the discovery of drug targets, identify the pathways involved in pathological processes (Ye et al. Citation2016), and explore the material basis and molecular mechanisms, providing new approaches and ideas for clinical medication or drug development.
Guizhi-Gancao Decoction (GGD) is composed of two TCM compounds, viz. Cinnamomi ramulus (CR) (Cinnamomum cassia Presl. [Lauraceae], 12 g) and licorice (Glycyrrhiza uralensis Fisch. [Fabaceae], 6 g), in a 2:1 ratio. Previously, we reported that GGD protects against pressure overload-induced HF in mice (Chen et al. Citation2017). Another study confirmed that GGD reduces myocardial ischaemia–reperfusion injury by inhibiting inflammation and myocardial apoptosis (Gao et al. Citation2019). CR has been shown to exhibit antihypoglycemic, antihypercholesterolemic, antibacterial and anti-inflammatory properties (Dorri et al. Citation2018). Studies have also shown that CR extracts can protect against myocardial ischaemia–reperfusion injury (Sedighi et al. Citation2018; Peng et al. Citation2021). Several lines of evidence have demonstrated that cinnamaldehyde, one of the major constituents of CR, has multiple therapeutic effects against cardiovascular diseases, including anti-inflammatory and antioxidant effects, which encompass prevention of oxidative injury to cardiomyocytes, antiviral effects that prevent myocarditis and anti-hypertrophic effects (Ding et al. Citation2010; Ismail et al. Citation2022; Tian et al. Citation2022). CR also contains cinnamic acid, which exerts cardioprotective effects, including improving cardiac diastolic function, limiting the size of the myocardial infarct, preventing myocardial apoptosis and retarding structural abnormalities of the heart (Luan et al. Citation2022). Peroxyergosterol, another active ingredient of CR, has antioxidant and anti-inflammatory effects (Nowak et al. Citation2016). Licorice has been confirmed to have several cardioprotective effects, such as the reduction of oxidative stress, restoration of heart metabolism and maintenance of mitochondrial function (Upadhyay et al. Citation2020). Glycyrrhizic acid, one of the main active ingredients of licorice, protects the myocardium from ischaemic injury caused by isoproterenol (Li et al. Citation2020). Glycyrrhizic acid alleviates autophagy, cardiomyocyte death and cardiotoxicity (Xu et al. Citation2022). These data indicate that the GGD may treat HF in a multicomponent, multitarget and multipathway manner.
Adenosine, an intrinsic purine nucleoside, plays a crucial role in controlling the cardiovascular system by engaging adenosine receptors (ADORs), which belong to the G protein-coupled receptor superfamily (Gaudry et al. Citation2020; Zhang et al. Citation2021). The four ADOR subtypes A1, A2a, A2b and A3 regulate coronary artery circulation, cardiac rhythm and pump function. The events modulated by ADORs also include suppression of inflammation, promotion of cell proliferation and tissue repair, regulation of cellular energy metabolism and defense against stress or damage (Olah and Stiles Citation1995; Headrick et al. Citation2011, Citation2013). Therefore, ADOR signaling pathways may be considered potential targets for the treatment of cardiovascular diseases.
In this study, we investigated the potential mechanisms of GGD involved in the treatment of HF using network pharmacology analysis combined with molecular docking and experimental verification. A mouse model of phenylephrine (PE)-induced cardiac hypertrophy was established to confirm the therapeutic effects of different GGD doses. Thereafter, the potential compounds and targets of GGD were predicted using network pharmacology analysis. Finally, the core targets of GGD were verified via molecular docking as well as in vitro and in vivo experiments.
Materials and methods
Screening of active GGD components and prediction of potential targets
The ingredients of GGD (CR and licorice) were identified from the traditional Chinese medicine systems pharmacology database and analysis platform (TCMSP) database (http://tcmspw.com/tcmsp.php) with oral bioavailability (OB) ≥ 30% and drug-likeness (DL) ≥ 0.18 set as the screening conditions. The Standard Delay Format structure of the above-mentioned components was obtained from the PubChem database (https://pubchem.ncbi.nlm.nih.gov/), and subsequently introduced to the Swiss target prediction database (http://www.SwisstargetPrediction.ch/) to predict the potential targets of these ingredients.
HF-related target screening
HF-related targets were acquired from the OMIM (https://omim.org/), GeneCards (https://www.genecards.org/) and Therapeutic Target Database (TTD) (http://db.idrblab.net/ttd/).
Common targets between the potential targets of GGD and HF-related targets
The screened ingredients’ potential targets and disease-related targets were imported into the Venny version 2.1 Online Software Module Tool Platform (https://bioinfogp.cnb.csic.es/tools/venny/index.html). The overlapping targets were represented in the form of a Wayne map for further analysis.
Network construction
A ‘drug-component-target-disease network’ map was constructed using the Cytoscape version 3.7.2 software (San Diego, California, USA), while the NetWork Analyser function was employed to analyse the main active ingredients of GGD.
Protein–protein interaction
The common targets were input into the String database (https://string-db.org/) for retrieval. The protein type was set as ‘HOMO SAPIENS,’ with the lowest interaction threshold set at 0.4.
Core target screening based on topological analysis
The constructed protein–protein interaction (PPI) network was imported into the Cytoscape version 3.7.2 software, and the MCODE module was used for gene cluster analysis and core-target screening. The selection criteria for MCODE were as follows: degree cutoff, 2; node score cutoff: 0.2; K-core: 2; and maximum depth, 100.
Gene ontology and Kyoto Encyclopedia of Genes and Genomes pathway enrichment analyses
The gene ontology (GO) functional analysis and Kyoto Encyclopedia of Genes and Genomes (KEGG) pathway analysis were conducted, and the results were visualized using the ‘Bioconductor’ R package. The criteria were set at p < 0.05 and Q < 0.05.
Molecular docking
The 3D structure of the compound was downloaded from PubChem and imported into the ChemDraw 3D software. The MM2 module was used to perform energy minimization to obtain the lowest energy conformer. The A1 adenosine receptor (A1AR) and adenosine A2A receptor (A2aAR) protein structures were downloaded from the UniProt database, whose PDBID was 5n2s and 5 nm4, respectively. The protein and ligand were visualized using PyMOL after water removal, hydrogen addition, charge calculation and non-polar hydrogen merging using Mgtools version 1.5.6 (Guangzhou, China). Docking was performed using Autodock Vina version 1.1.2 (California, USA), and the highest-scoring conformer was selected and visualized using PyMOL and Discovery Studio.
Preparation of GGD
GGD granules were provided by Shanghai Wanshicheng State Medicine Products Co., Ltd., Shanghai, China. GuiZhi originated from Guizhou, China (batch no. 20220921-1), and GanCao originated from Gansu, China (batch no. 20220915-1). The granules were prepared according to the Quality Control Technology Requirements and Standard Preparations of TCM Formulas issued by the Chinese State Administration of Drug Supervision. Briefly, 252 g of herbs were weighed according to the prescription ratio of 2:1 for CR and licorice. The herb mixture was first boiled in 8 times the amount of water for 1 h, followed by boiling in 6 times the amount of water for 0.5 h. The extracts were filtered through an 80-mesh sieve, and then left in 70–80 °C to achieve a concentration of 1.12–1.15 g/cm3. After purification with the alcohol precipitation method, the extracts were dried and granulated (extrusion pressure = 15 MPa, extrusion speed ≤ 35 rad/min) to obtain approximately 28 g of GGD granules (water content ≤ 6%). For in vitro experiments, GGD was serially diluted with double distilled H2O until its osmotic pressure (determined by Gonotec, OSMOMAT 030, Berlin, Germany) was equivalent to that of the complete culture medium (adjusted to pH 7.4). The sample was centrifuged at 3000 rpm for 30 min and passed through a 0.22 μm filtration system for sterilization before use.
Isolation and culture of primary mouse cardiomyocytes
The neonatal mice were purchased from SLAC Laboratory Animal Technology Co., Ltd., Shanghai, China. Ventricles from 24-h-old neonatal mice were harvested, subjected to digestion with trypsin, and cultured in Dulbecco’s Modified Eagle Medium/Nutrient Mixture F12 (DMEM/F12, Gibco BRL, Grand Island, NY) supplemented with 10% fetal bovine serum (FBS, Gibco BRL, Grand Island, NY) and 1% penicillin/streptomycin (Pen/Strep, Gibco BRL, Grand Island, NY). Cardiomyocytes were obtained through gradient centrifugation and cultured in an incubator containing CO2 for 48 h at 37 °C. The cells were divided into the control, PE (100 μM), PE (100 μM) + 2-chloroadenosine (CADO; 5 µM), a nonselective adenosine receptor agonist, PE (100 μM) + GGD (1 × 10−5 g/mL) and PE (100 μM) + GGD (1 × 10−5 g/mL) + CGS15943 (5 µM) groups. All experimental protocols were approved by the Animal Management and Use Committee of Shanghai University of TCM (PZSHUTCM2301040006). We strictly followed humane endpoint criteria throughout the animal experiments. The neonatal mice were first anesthetized by 5% isoflurane inhalation, followed by decapitation using sharp scissors. Experiments had been carried out from March to July 2023.
Animal experiments
Eight-week-old male C57BL/6J mice weighing 20–25 g were purchased from Vital River Laboratory Animal Technology Co., Ltd., Beijing, China. The animals were housed in the Experimental Animal Center of Shanghai University of TCM under specific pathogen-free conditions with free access to food and water under a 12 h light-dark cycle. All experimental protocols were approved from December 2022 to March 2023 by the Animal Management and Use Committee of Shanghai University of TCM (PZSHUTCM2301040006). We strictly followed humane endpoint criteria throughout the animal experiments. The mice were euthanized by intravenous injection of sodium pentobarbital. Specifically, each mouse received 150 mg/kg of 3% sodium pentobarbital solution.
After one week of adaptation to the raised environment, animals in the first in vivo experiment that aimed to confirm the therapeutic effects of GGD, were randomly divided into the following five groups including the control (n = 10), PE (n = 10; Sigma–Aldrich, St. Louis, MO), low-dose GGD (GGD-L, n = 10, 2.7 g/kg/d, per oral), medium-dose GGD (GGD-M, n = 10, 5.4 g/kg/d, per oral) and high-dose GGD (GGD-H, n = 10, 13.5 g/kg/d, per oral) groups. The dose was calculated using the equivalent dose conversion factor based on the surface area. In the second animal experiment aimed at verifying the core targets, the mice were randomly divided into 5 groups, viz. the control (n = 10), PE (n = 10), CADO (n = 10, 2 mg/kg/d intraperitoneally; Sigma–Aldrich) (Wakeno et al. Citation2006; Bousquenaud et al. Citation2013), GGD (n = 10, 5.4 g/kg/d, per oral), and GGD + CGS15943 [n = 10, GGD 5.4 g/kg/d with CGS15943, a nonselective adenosine receptor antagonist (Sigma–Aldrich, a, 4 mg/kg/d, per oral] (Ghai et al. Citation1987) groups. Cardiac hypertrophy was induced as described previously (Scarborough et al. Citation2021). Briefly, the mice, except the control group, were injected with PE 10 mg/kg (Sigma–Aldrich, St. Louis, MO) intraperitoneally every other day, for a total of 3 times, and sacrificed on day 8. The mice in different intervention groups received the study agents at the concentrations indicated above. C57BL/6 mice consistently develop cardiac hypertrophy upon PE stimulation, providing an ideal model to investigate mechanisms and therapies for myocardial remodeling. Compared to simplified cell experiments, animal models are more convincing for evaluating drug effects at the organism level and mimicking human disease processes.
Echocardiographic analysis
The mice were anesthetized with 3% isoflurane on day 8. After the animals were anesthetized, the isoflurane dose was lowered to 1–2% for maintenance during the procedure. Oxygen flow was kept at 1 L/min throughout. The chest of each mouse was shaved, and an ultra-high-resolution small animal imaging system (Vevo 2100, Visual Sonics Inc., Toronto, Canada) was used to detect cardiac function. A left ventricular long-axis section was obtained, and the M-mode curve was used for measurement (Tanaka et al. Citation1996).
Histological examination
After euthanasia, hearts were rapidly excised, and the left ventricle was dissected out and frozen in liquid nitrogen. The tissue samples were fixed in 4% paraformaldehyde for 48 h, followed by routine paraffin embedding. The blocks were cut into sections of 5 μm thickness, followed by hematoxylin-eosin (HE) or Masson’s trichrome staining.
Western blotting
Extracts of proteins from cells and tissues were prepared using RIPA lysis buffer containing protease and phosphatase inhibitors. Protein concentration was determined by BCA protein assay kit (Beyotime, Shanghai, China). About 25 µg of total protein was separated using sodium dodecyl-sulfate polyacrylamide gel electrophoresis, and transferred to polyvinylidene difluoride membranes (Millipore, Billerica, MA). After blocking with 5% BSA for 1 h, the membranes were incubated with primary antibodies against A1AR (1:1000), A2aAR (1:500) and GAPDH (1:8000) overnight at 4 °C, followed by incubation with secondary antibody (1:2000) or (1:10,000) for 1 h. The blots were visualized via chemiluminescent detection (Immobilon Western HRP, Millipore, Billerica, MA), and analysed using Alpha View SA software (Alfaview Gmbh, Karlsruhe, Germany). The following primary antibodies were used in this study: A1AR (ab82477; Abcam, Cambridge, UK), A2aAR (ab79714; Abcam) and GAPDH (60004-1-Ig; Proteintech, Chicago, IL).
Immunofluorescence analyses
For immunofluorescence examination, the cardiomyocytes or myocardial tissues were fixed with paraformaldehyde, followed by treatment with 0.5% Triton X-100. After blocking with 5% BSA at room temperature for 1 h, the samples were incubated overnight at 4 °C with primary antibodies against α-actinin (1:250), A1AR (1:250), A2aAR (1:250) and CTNT (1:250), followed by incubation with Alexa Fluor 488 (1:500), Alexa Fluor 555 (1:500), Alexa Fluor 594 (1:500) or Alexa Fluor 647 (1:500) secondary antibodies at room temperature for 90 min in the dark. Nuclei were counterstained with DAPI (1:1000). After labeling and washing, fluorescent photographs were acquired through a Zeiss LSM 880 confocal microscope (Carl Zeiss, D07740 Jena, Germany) under 488 nm, 555 nm, 594 nm and 647 nm excitation. Fluorescence intensity and cell surface area were quantified using the ZEN imaging software (Carl Zeiss, D07740 Jena, Germany). The following primary antibodies were used in this study: A1AR (ab82477; Abcam, Cambridge, UK), A2aAR (ab79714; Abcam), and α-actinin (ab9465; Abcam); CTNT(15513-1-AP, Proteintech, Chicago, IL); DAPI (C1006, Beyotime, Shanghai, China); Alexa Fluor 488 (AS053, ABclonal, Wuhan, China); Alexa Fluor 555 (AS057, ABclonal, Wuhan, China); Alexa Fluor 594 (AS054, ABclonal, Wuhan, China); Alexa Fluor 647 (AS059, ABclonal, Wuhan, China).
Quantitative PCR analysis
RNA was extracted from a 6-well plate or heart tissue in the presence of Trizol (Beyotime, Shanghai, China), and the concentration was determined. The RNA was reverse transcribed into cDNA, followed by real-time polymerase chain reaction (PCR) testing (Takara Bio Inc., Shiga, Japan). The gene was obtained using the photo circulation 480 II instrument (Roche Diagnostics, Mannheim, Germany). The target-specific primers used in this study are listed in . The level of expression of the target gene was calculated using 2−ΔΔCt after quantitative PCR detection.
Table 1. Primer sequences for quantitative PCR.
Statistical analysis
The results were presented as the mean ± standard error of the mean (SEM). The data were analysed using the one-way analysis of variance (ANOVA), and inter-group differences were detected using the Newman–Keuls test if the ANOVA revealed significant differences. Statistical significance was set at p < 0.05.
Results
GGD attenuates PE-induced cardiac hypertrophy in mice
Previously, we reported that GGD attenuated pressure overload-induced cardiac hypertrophy (Chen et al. Citation2017). To determine whether GGD protected against cardiac hypertrophy caused by other stimuli, we investigated the therapeutic effects of GGD in the mice subjected to PE administration. It was consistently observed that three different dosages of GGD, viz. GGD-L, GGD-M and GGD-H, can abrogate PE-induced cardiac hypertrophy, as indicated by morphological examination and gravimetric parameters (). Morphological examination also revealed that PE administration caused myocardial necrosis with interstitial cell proliferation and mononuclear cell infiltration. Intervention with different doses of GGD, especially the medium dosage, alleviated these changes (). These findings are consistent with results of M-mode echocardiography analysis. The PE group showed increased interventricular septal thickness at end-diastole and end-systole compared to the control group (p < 0.05; ), which was blocked by medium-dose GGD treatment (p < 0.05; ). Based on the above-mentioned results, the medium dose of GGD was chosen for the subsequent in vivo experiment.
Figure 1. Different doses of GGD attenuate PE-induced cardiac hypertrophy in mice. (A) Representative myocardium pictures. (a) Gross images show the size of whole organs; (b) H&E staining heart sections from paraffin embedding samples demonstrate the thickening of ventricular walls; (c) Zooming-in H&E staining heart sections demonstrate details of tissue samples, mononuclear cell infiltration at the site indicated by the black arrow, and fibroplasia was indicated by blue arrows. (B) Quantification of HW/BW, HW/TL, LW/BW and LW/TL in mice. (C) Representative m-mode echocardiography images. (D) Quantification of echocardiography parameters. *p < 0.05 vs. Con; #p < 0.05 vs. PE; GGD: Guizhi-Gancao Decoction; PE: phenylephrine; HW/BW: heart weight body to weight ratio; HW/TL: heart weight to tibia length ratio; LW/BW: lung weight to body weight ratio; LW/TL: lung weight to tibia length ratio; EF: ejection fraction; FS: fractional shortening; IVSd: interventricular septum thickness at end-diastole; IVSs: interventricular septum thickness at end-systole.
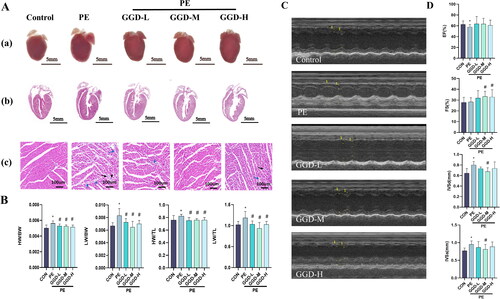
Active ingredients of GGD
We conducted network pharmacology and molecular docking studies to further explore the molecular mechanisms of GGD against cardiac hypertrophy. Ninety-eight potential active ingredients were screened from GGD using the TCMSP database with OB ≥ 30% and DL ≥ 0.18 as criteria ().
Table 2. Essential information about GGD active components.
Common target network and compound-target network construction
We sought to identify the potential targets responsible for the therapeutic effects of GGD. Accordingly, the potential targets of GGD were predicted using the Swiss Target Prediction database, which identified a total of 777 targets. Meanwhile, a total of 1271 disease targets were obtained by searching OMIM, GeneCards and TTD using ‘Heart failure’ as the keyword. As a result, 141 common drug-disease targets between HF and GGD were identified via the Venny version 2.1 online software mapping tool platform (). Finally, the 90 potential active ingredients (8 active ingredient targets that did not overlap with the disease targets were deleted) and 141 common drug-disease targets in GGD were input into the Cytoscape version 3.7.2 software to create a network diagram of the ‘drug-ingredients-target-disease’ interaction (). The blue triangles in this figure represent the 90 active ingredients of GGD, including inermine, mairin, DFV, glycyrol, peroxyergosterol, β-sitosterol, sitosterol, etc. The green circles represent the 141 drug-disease common targets, while the red diamond represents HF. The top 20 targets with the highest degree, including ESR1, EGFR, ADORA1, ADORA2A, matrix metalloproteinase (MMP)1, etc., are shown in .
Figure 2. Overlapping target genes between GGD and HF and the drug-compound-target-disease network. (A) The Venn diagram shows 141 drug-disease common targets between GGD and HF. (B) The network consists of 90 active ingredients in GGD (blue triangles), 141 drug-disease common targets (green circles) and HF (red diamond). The thickness of lines represents the binding intensities between compounds and targets. GGD: Guizhi-Gancao Decoction; HF: heart failure.
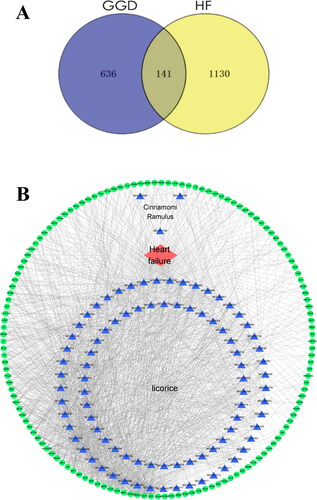
Table 3. Topological analysis of the top 20 common targets with the highest degree values.
PPI analysis
We constructed a PPI network based on the STRING database to predict the protein interactions. As shown in , 141 nodes and 1466 edges were embodied, the average node degree was 20.8, and the PPI enrichment p-value was <1.0e-16. A protein interaction network diagram was drawn using the Cytoscape software (). Each circle represents the interaction between the proteins. The change in color depth represents the magnitude of the degree value, where the darker the color, the greater the strength of the association. The data showed that VEGFA, AKT1, TNF, EGFR, STAT3 and NOS3 were the most relevant proteins.
Figure 3. Protein–protein interaction network of overlapping targets between GGD and HF. (A) Each edge represents the interaction between the proteins, and the more lines the greater the degree of association. (B) Cross-target gene network map between 90 active ingredients and HF-related targets. Each circle represents the interaction between the proteins. The change in color depth represents the size of the degree value, the darker the color, the stronger the degree between the associations.
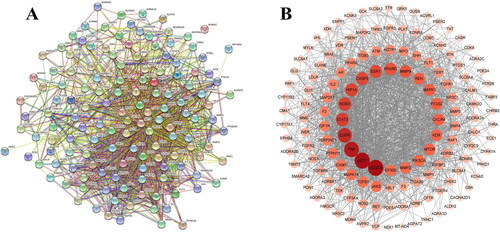
Clusters of common targets of GGD against HF
Clustering analysis of the PPI network using the Cytoscape plugin (MCODE) (K-core = 2) yielded a total of 7 gene clusters ( and ) core genes. The core genes were MMP2, PTPN11, A2aAR, endothelin receptor B (EDNRB) and Mitogen-Activated Protein Kinase 2 (MAP2K2). Clustering analysis suggested that these clusters may be most relevant for HF treatment, as shown in detail in . MMP2 was the core target of cluster 1, which contained 32 nodes and 385 edges, with a score of 24.8 (). MMP-2, a member of the MMP gene family, is a zinc-dependent enzyme that cleaves components of the extracellular matrix and molecules involved in signal transduction. PTPN11 was the core target of cluster 2, which contained 21 nodes and 59 edges, with a score of 5.9 (). PTPN11 is a protein tyrosine phosphatase and acts as a signaling molecule that regulates various cellular processes such as cell growth, differentiation, etc. Cluster 3 contained 4 nodes and 5 edges, with a score of 3.3 (). Cluster 4 contained 3 nodes and 3 edges, with a score of 3 (). Cluster 5 contained 3 nodes and 3 edges, with a score of 3. The core target of this cluster was A2aAR (). A2aAR is a G protein-coupled receptor that acts as an autoreactive substance and is also involved in the regulation of intercellular conductance mediators. EDNRB was the core target of cluster 6, which contained 3 nodes and 3 edges, with a score of 3 (). EDNRB is a G protein-coupled receptor that is closely associated with vasoconstriction, myocardial ischaemia, and vascular injury. Cluster 7 contained 7 nodes and 8 edges, with a score of 2.67, and the core target of this cluster was MAP2K2 (). MAP2K2/MEK2 can regulate a variety of cellular physiological/pathological processes such as cell growth, differentiation, and the inflammatory response.
GO and KEGG pathway enrichment analyses
As shown in , biological process annotation suggested that the potential therapeutic mechanism of GGD in HF was mainly associated with epithelial cell proliferation, regulation of blood circulation, response to oxygen levels and response to hypoxia and vascular processes in the circulatory system. Cellular compartment annotation indicated that the action of GGD in HF was mainly related to the membrane raft, membrane microdomain, membrane region and early endosome and focal adhesion. Meanwhile, molecular function annotation indicated that protein serine/threonine kinase activity, protein tyrosine kinase activity, transmembrane receptor protein kinase activity and growth factor binding may be involved in the therapeutic effect of GGD in HF. Moreover, KEGG pathway enrichment analysis showed the underlying therapeutic mechanism of GGD against HF mainly involved proteoglycans in cancer, and the calcium, Rap1, PI3K-Akt and mitogen-activated protein kinase (MAPK) signaling pathways ( and ).
Table 4. The top 20 KEGG pathway terms for GGD anti-HF.
Molecular docking
Based on degree centrality, target clustering analysis and KEGG analysis, we postulated that ADORs may play an important role in the therapeutic action of GGD against HF. We performed molecular docking analysis to validate the binding of the main ingredient compounds of GGD to ADORs. A molecular docking score of ˂−7.0 kcal/mol indicates strong binding affinity between the target and compound (Hsin et al. Citation2013). Our results showed that the binding energies of inermine, jaranol and peroxyergosterol with the A2aAR were −8.3, −9.7 and −10.3 kcal/mol, respectively. The binding energies of DFV, jaranol and peroxyergosterol with the A1AR were −8.9, −8.2 and −9.9 kcal/mol, respectively. These results indicate that these six compounds have strong affinity for the A2aAR and A1AR. The A2aAR-inermine and A2aAR-jaranol complexes were stabilized by hydrogen bonding interactions between Val97 and Asn358, and Ala68 and Asn358 (). The A2aAR-peroxyergosterol complex was mainly stabilized by hydrophobic interactions between Leu354, Ile379, Met375, Leu176 and Phe177 (). The A1AR-DFV and A1AR-jaranol complexes were stabilized by hydrogen bonding interactions between Val1188 and Asn1359, Leu1170 and Asn1359 (). The A1AR-peroxyergosterol complex was mainly stabilized by hydrophobic interactions between Trp1352, Ile1379, Leu1355, Glu1275, Phe1276, Ile1174, Val1192 and Ala1196 (). These interactions effectively lock the various ligands into the active pockets of A2aAR and A1AR to form stable complexes.
Quantitative analysis of the key ingredients of GGD
The chemical components of GGD were analysed using high performance liquid chromatography (), which demonstrated that GGD contained cinnamaldehyde (0.082 mg/g), cinnamic acid (0.564 mg/g), schisandrin (0.013 mg/g), β-sitosterol (0.137 mg/g), isorhamnetin (0.043 mg/g), glycyrrhizic acid (7.476 mg/g) and DFV (0.214 mg/g).
Figure 7. HPLC chromatograms of reference standards and GGD sample. (A) Reference chromatogram: (a) cinnamic acid; (b) cinnamaldehyde. (B) Sample chromatogram: (a) cinnamic acid; (b) cinnamaldehyde. (C) Reference chromatogram: (a) isorhamnetin; (b) schisandrin (is); (c) β-sitosterol. (D) Sample chromatogram: (a) isorhamnetin; (b) schisandrin (is); c. β-sitosterol. (E) Reference chromatogram: (a) DFV; (b) glycyrrhizic acid. (F) Sample chromatogram: (a) DFV; (b) glycyrrhizic acid.
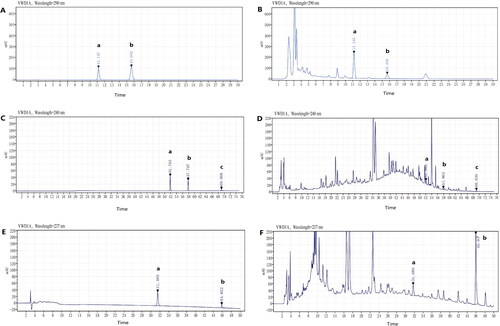
GGD attenuates PE-induced cardiomyocyte hypertrophy in vitro
The results of network pharmacology analysis were consistent with a previous study that reported that the activation of multiple adenosine receptor subtypes inhibited PE-induced cardiomyocyte hypertrophy (Gan et al. Citation2005). Therefore, we sought to investigate the mechanisms underlying the therapeutic effects of GGD. To that end, we constructed a PE-stimulated in vitro cardiomyocyte hypertrophy model. First, a dose-effect relationship was analysed. As shown in , GGD (ranging from 3 × 10−6 to 3 × 10−4 g/mL) attenuated the pe-induced increase in surface area, with a half maximal effective concentration (EC50) = 5.484 × 10−6 g/mL. As shown in , PE significantly increased the surface area of cardiac myocytes and the expression of atrial natriuretic peptide (ANP), brain natriuretic peptide (BNP) and myosin heavy chain (MYH)7/MYH6 (p < 0.05), whereas GGD or CADO (a nonselective adenosine receptor agonist) blocked these changes (p < 0.05). Moreover, the anti-hypertrophic effects of GGD were reversed by CGS15943, a nonselective adenosine receptor antagonist. We then observed the expression of the A1AR and A2aAR in neonatal rat cardiomyocytes (NRCM). As shown in , PE increased A1AR expression and decreased A2aAR expression (p < 0.05), both of which were blocked by GGD and CADO administration (p < 0.05). Similarly, the effects of GGD were reversed by CGS15943.
Figure 8. GGD attenuates PE-induced cardiomyocyte hypertrophy in vitro. (A) Dose-response curve and EC50 of GGD on cardiomyocyte surface area. (B) Representative immunofluorescence images of α-actinin (red) and DAPI (blue) in cardiomyocytes (scale bar = 50 μm). (C) The mRNA expression of ANP, BNP and MYH7/MYH6. (D) Representative immunofluorescence images of A1AR (green), CTNT (red) and DAPI (blue) in cardiomyocytes (scale bar = 50 μm). (E) Representative immunofluorescence images of A2aAR (green), CTNT (red) and DAPI (blue) in cardiomyocytes (scale bar = 50 μm). (F) Quantification of fluorescence intensity of A1AR and A2aAR. (G) Quantification of A1AR and A2aAR mRNA expression. (H, I) Representative blots and quantification of A1AR and A2aAR. *p < 0.05 vs. Con; #p < 0.05 vs. PE.
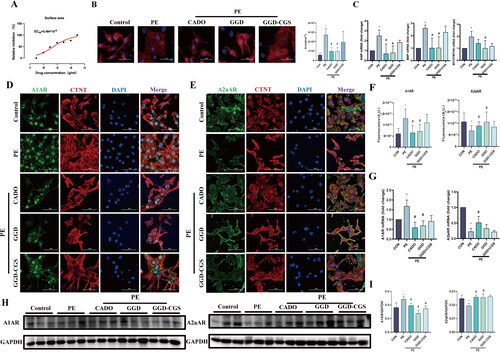
CGS15943 reverses the action of GGD on PE-induced cardiac hypertrophy
We sought to investigate whether the findings derived from cultured cardiac myocytes could be confirmed in vivo. Therefore, we analysed the effect of GGD in mice subjected to PE administration. As shown in , GGD and CADO blocked PE-induced cardiac hypertrophy as determined by morphological examination, gravimetric analysis, echocardiography analysis and ANP, BNP and MYH7/MYH6 gene expression (p < 0.05). HE staining revealed that, compared to the control group, PE-induced marked cardiomyocyte hypertrophy along with mononuclear cells infiltration and interstitial proliferation. Masson’s trichrome staining showed extensive interstitial collagen deposition with mild to moderate perivascular collagen deposition in the PE group. Those morphological examinations revealed that GGD and CADO alleviated PE-induced inflammatory cells infiltration and fibrous tissue accumulation. Moreover, our data also showed that CGS15943 reversed most of the effects exerted by GGD.
Figure 9. CGS15943 Antagonized the protective effects of GGD on PE-induced cardiac hypertrophy. (A) Representative myocardium pictures. (a) Gross images show the size of whole organs; (b) H&E staining heart sections demonstrate the thickening of ventricular walls; (c) Zooming-in H&E staining heart sections demonstrate details of tissue samples, mononuclear cell infiltration at the site indicated by the arrow; (d) Zooming-in Masson staining heart sections demonstrate collagen fibers, the arrow indicates the site of collagen deposition; (e) Zooming-in Masson’s trichrome stained section of heart surrounding blood vessels shows perivascular fibrosis, the arrow indicates the site of collagen deposition. (B) Quantification of HW/BW, HW/TL, LW/BW and LW/TL in mice. (C) Representative m-mode echocardiography images. (D) Quantification of echocardiography parameters. (E) Quantification of ANP, BNP and MYH7/MYH6 mRNA expression. *p < 0.05 vs. Con; #p < 0.05 vs. PE; GGD: Guizhi-Gancao Decoction; PE: phenylephrine; HW/BW: heart weight body to weight ratio; HW/TL: heart weight to Tibia length ratio; LW/BW: lung weight to body weight ratio; LW/TL: lung weight to Tibia length ratio; EF: ejection fraction; FS: fractional shortening; IVSd: interventricular septum thickness at end-diastole; IVSs: interventricular septum thickness at end-systole.
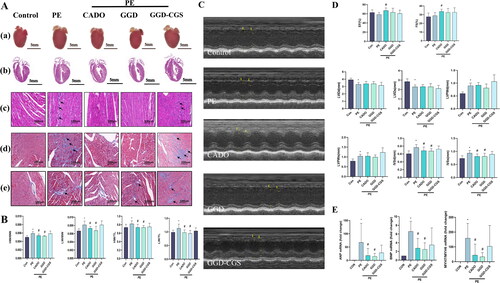
GGD blocks PE-induced expression changes in adenosine receptors
We also observed the expression of A1AR and A2aAR in the mouse myocardium. As shown in , PE caused A1AR over-expression as well as A2aAR down-expression (p < 0.05), which were blocked by GGD and CADO administration (p < 0.05). Meanwhile, the effects of GGD were reversed by CGS15943.
Figure 10. GGD blocked PE-induced expression changes of adenosine receptors. (A) Representative immunofluorescence images show A1AR (red) and DAPI (blue) (scale bar = 50 μm). (B) Representative immunofluorescence images show A2aAR (red) and DAPI (blue) (scale bar = 50 μm). (C) Quantification of A1AR and A2aAR mRNA expression. (D,E) Representative blots and quantification of A1AR and A2aAR protein expression. *p < 0.05 vs. Con; #p < 0.05 vs. PE.
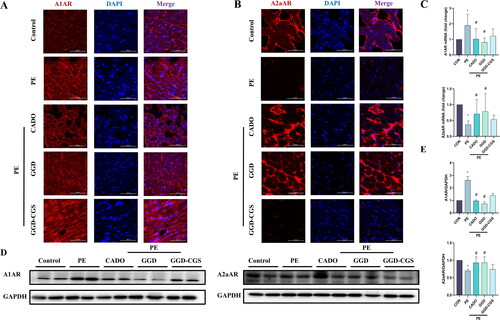
Discussion
Pathological cardiac hypertrophy caused by stress overload often precedes the occurrence of HF, a major cardiovascular disease whose incidence and mortality have risen worldwide. The current clinical treatment for HF is based on diuretics, renin-angiotensin-aldosterone system inhibitors, β-blockers, positive inotropic drugs, vasodilators, etc. The number of deaths due to HF continues to increase, despite great strides in the treatment of HF. TCM can serve as an adjunctive and alternative method for preventing HF and can effectively improve patients’ clinical symptoms and quality of life. Consistent with our previous study, which demonstrated that GGD can attenuate pressure overload-induced cardiac hypertrophy, in this study, we found that GGD could mitigate PE-induced cardiac hypertrophy in mice. GGD is a famous TCM herbal formula, which has been widely used for thousands of years. It is also used as a basic herbal combination in many other formulae to treat a variety of diseases including various cardiovascular diseases. However, to date, the mechanisms underlying the effect of GGD remain unclear.
Since GGD is an herbal formula, it contains numerous ingredients, which encumbers clarification of the mechanisms of action. Network pharmacology, based on the theory of systems biology, has shifted the drug discovery paradigm from the conventional ‘one gene, one drug, one disease’ to ‘network target, multi-components’ concept (Hopkins Citation2007, Citation2008). It analyses interactions between drugs, targets and diseases, providing insights into the relationships between TCM and disease. By bridging network science and ancient TCM, network pharmacology provides a novel methodology and opportunity to discover bioactive ingredients and better explore the underlying mechanisms of herbal formulae (Li and Zhang Citation2013; Zhao et al. Citation2023). Therefore, using the network pharmacology approach, we constructed a drug ingredient-target disease network to clarify the relevant disease targets of GGD in the treatment of cardiac hypertrophy.
In this study, 90 candidate ingredients, including β-sitosterol and glycyrol and others, were screened from GGD. β-Sitosterol is an effective active ingredient of CR, which can ameliorate the inflammatory response and oxidative stress, stimulate the production of mitochondrial ATP in the heart, and inhibit oxidative damage to the heart (Wong et al. Citation2014; Koc et al. Citation2021). Glycyrol is extracted from licorice and has anti-cancer, anti-inflammatory and antioxidant effects, and is a novel immunosuppressive agent (Fu et al. Citation2014; Lu et al. Citation2019; Kim et al. Citation2020).
PPI network analysis identified VEGFA, AKT1 and TNF as among the 141 major targets potentially integral to mediating the benefits of GGD in HF. VEGFA, pivotal for angiogenesis, maintains cardiac contraction and wound healing and may ameliorate myocardial infarction (Zangi et al. Citation2013; Braile et al. Citation2020). Akt1 directs cell growth and metabolism that are vital for heart development and function; its deficiency causes cardiac defects and aberrant cardiomyocyte proliferation (Chang et al. Citation2010; Hers et al. Citation2011). TNF is unexpressed in the normal heart and also in HF, in proportion with disease severity (Feldman et al. Citation2000).
Subsequently, 7 gene clusters were identified through cluster analysis-based core target screening, among which 5 core targets, including MMP2, PTPN11, A2aAR, EDNRB and MAP2K2, were delineated. Furthermore, KEGG enrichment analysis suggested that the calcium, Rap1, PI3K-Akt and MAPK signaling pathways may be involved in the action of GGD. Based on our topological analysis, cytoHubba node screening, and KEGG enrichment analysis, we chosen the A1AR and A2aAR as the basis for the compound-target interaction probe through molecular docking analysis, which found that the active compounds of GGD, such as inermine, jaranol and peroxyergosterol had strong binding affinities for the A1AR and A2aAR.
Adenosine, an endogenous purine nucleoside, governs physiological/pathological processes including vasodilation, inflammation and ischaemia-reperfusion injury, thereby reducing myocardial injury (McIntosh and Lasley Citation2012; Guerrero Citation2018). The expression of A1Ars in atrial and ventricular smooth muscle cells and cardiomyocytes can be considered as potential therapeutic targets in cardiovascular diseases (Burnstock and Pelleg Citation2015; Szentmiklosi et al. Citation2015; Effendi et al. Citation2020). Adenosine regulates adrenergic responses through A1AR-mediated mechanisms, inhibits norepinephrine-mediated indirect changes in cardiac contractility, reduces endothelin-1 production and weakens the activation of the renin-angiotensin system, attenuating cardiac hypertrophy and myocardial dysfunction (Liao et al. Citation2003; Pasquini et al. Citation2021). The selective A1AR agonist N6-cyclopentyladenosine prevents cardiac hypertrophy and heart failure in left ventricular pressure overload models (Effendi et al. Citation2020). Thus, the A1AR could be a potential therapeutic target for cardiac hypertrophy. The A2aAR, an adenosine receptor is cardioprotective and regulates heart rhythm, conduction, autonomic control, coronary flow and development (Zhan et al. Citation2011; Zhai et al. Citation2015; Lasley Citation2018). The reduction in mitochondrial ATP in HF inhibits adenosine/A2aAR signaling, increasing oxidative stress, thereby exacerbating HF. A2aAR activation may reduce reperfusion oxidative stress (Xu et al. Citation2017; Bertero and Maack Citation2018; Castro et al. Citation2020). The A2aAR protects against ischaemia/reperfusion-induced cardiac injuries and pressure overload-induced cardiac hypertrophy by regulating reactive oxygen species generation through the MAPK-NADPH oxidase signaling pathway (Ribé et al. Citation2008). The A2aAR has also been proven to increase cAMP levels and PKA activity in cardiomyocytes, which subsequently enhances calcium entry through L-type Ca2+ channels and SR Ca2+ release (Boknik et al. Citation2020).
In this study, we found that PE administration caused cardiac hypertrophy with A1AR overexpression and A2aAR down-expression. These changes were blocked by GGD and the nonselective adenosine receptor agonist CADO (Mathoôt et al. Citation1996). Moreover, the nonselective adenosine receptor antagonist CGS15943 (Yip et al. Citation2011) reversed most of the effects of GGD. Therefore, GGD may attenuate PE-induced cardiac hypertrophy, at least partially, via the adenosine receptor signaling pathway.
Conclusions
This study confirmed the anti-hypertrophic effects of GGD in a mouse model of PE-induced cardiac hypertrophy. Network pharmacology data showed that GGD exerted therapeutic effects through multiple pathways and compounds with multiple targets. The actions of ADORs were verified using molecular docking analysis as well as in vitro and in vivo experiments. Further studies are needed to explore the upstream and downstream signaling pathways of ADORs and interpret and validate other pathways and targets predicted by the network pharmacology approach.
Author contributions
KY and XS: Methodology, Investigation, Writing-original draft; SY and MF: Investigation; PZ and HC: Data curation; MX and WG: Validation; RL: Funding acquisition, Project administration; CZ: Funding acquisition, Conceptualization, Writing-review and editing; All authors reviewed the manuscript.
Availability of data and materials
The datasets used and/or analysed during this study are available from the corresponding author on reasonable request.
Disclosure statement
The authors declare that they have no competing financial interests.
Additional information
Funding
References
- Bertero E, Maack C. 2018. Metabolic remodelling in heart failure. Nat Rev Cardiol. 15(8):457–470. doi:10.1038/s41569-018-0044-6.
- Boknik P, Eskandar J, Hofmann B, Zimmermann N, Neumann J, Gergs U. 2020. Role of cardiac A2A receptors under normal and pathophysiological conditions. Front Pharmacol. 11:627838. doi:10.3389/fphar.2020.627838.
- Bousquenaud M, Maskali F, Poussier S, Zangrando J, Marie PY, Boutley H, Fay R, Karcher G, Wagner DR, Devaux Y. 2013. Cardioprotective effects of adenosine within the border and remote areas of myocardial infarction. EJNMMI Res. 3(1):65. doi:10.1186/2191-219X-3-65.
- Braile M, Marcella S, Cristinziano L, Galdiero MR, Modestino L, Ferrara AL, Varricchi G, Marone G, Loffredo S. 2020. VEGF-A in cardiomyocytes and heart diseases. Int J Mol Sci. 21(15):5294. doi:10.3390/ijms21155294.
- Burnstock G, Pelleg A. 2015. Cardiac purinergic signalling in health and disease. Purinergic Signal. 11(1):1–46. doi:10.1007/s11302-014-9436-1.
- Castro CM, Corciulo C, Solesio ME, Liang F, Pavlov EV, Cronstein BN. 2020. Adenosine A2A receptor (A2AR) stimulation enhances mitochondrial metabolism and mitigates reactive oxygen species-mediated mitochondrial injury. FASEB J. 34(4):5027–5045. doi:10.1096/fj.201902459R.
- Chang Z, Zhang Q, Feng Q, Xu J, Teng T, Luan Q, Shan C, Hu Y, Hemmings BA, Gao X, et al. 2010. Deletion of Akt1 causes heart defects and abnormal cardiomyocyte proliferation. Dev Biol. 347(2):384–391. doi:10.1016/j.ydbio.2010.08.033.
- Chen HH, Zhao P, Tian J, Guo W, Xu M, Zhang C, Lu R. 2017. The Effects of guizhi gancao decoction on pressure overload-induced heart failure and posttranslational modifications of tubulin in mice. Evid Based Complement Alternat Med. 2017:2915247–2915248. doi:10.1155/2017/2915247.
- Ding Y, Qiu L, Zhao G, Xu J, Wang S. 2010. Influence of cinnamaldehyde on viral myocarditis in mice. Am J Med Sci. 340(2):114–120. doi:10.1097/MAJ.0b013e3181dd3b43.
- Dorri M, Hashemitabar S, Hosseinzadeh H. 2018. Cinnamon (Cinnamomum zeylanicum) as an antidote or a protective agent against natural or chemical toxicities: a review. Drug Chem Toxicol. 41(3):338–351. doi:10.1080/01480545.2017.1417995.
- Effendi WI, Nagano T, Kobayashi K, Nishimura Y. 2020. Focusing on adenosine receptors as a potential targeted therapy in human diseases. Cells. 9(3):785. doi:10.3390/cells9030785.
- Feldman AM, Combes A, Wagner D, Kadakomi T, Kubota T, Li YY, McTiernan C. 2000. The role of tumor necrosis factor in the pathophysiology of heart failure. J Am Coll Cardiol. 35(3):537–544. doi:10.1016/s0735-1097(99)00600-2.
- Fu Y, Zhou H, Wang S, Wei Q. 2014. Glycyrol suppresses collagen-induced arthritis by regulating autoimmune and inflammatory responses. PLoS One. 9(7):e98137. doi:10.1371/journal.pone.0098137.
- Gan XT, Rajapurohitam V, Haist JV, Chidiac P, Cook MA, Karmazyn M. 2005. Inhibition of phenylephrine-induced cardiomyocyte hypertrophy by activation of multiple adenosine receptor subtypes. J Pharmacol Exp Ther. 312(1):27–34. doi:10.1124/jpet.104.073122.
- Gao Y, Song G, Cao YJ, Yan KP, Li B, Zhu XF, Wang YP, Xing ZY, Cui L, Wang XX, et al. 2019. The guizhi gancao decoction attenuates myocardial ischemia-reperfusion injury by suppressing inflammation and cardiomyocyte apoptosis. Evid Based Complement Alternat Med. 2019:1947465–1947411. doi:10.1155/2019/1947465.
- Gaudry M, Vairo D, Marlinge M, Gaubert M, Guiol C, Mottola G, Gariboldi V, Deharo P, Sadrin S, Maixent JM, et al. 2020. Adenosine and its receptors: an expected tool for the diagnosis and treatment of coronary artery and ischemic heart diseases. Int J Mol Sci. 21(15):5321. doi:10.3390/ijms21155321.
- Ghai G, Francis JE, Williams M, Dotson RA, Hopkins MF, Cote DT, Goodman FR, Zimmerman MB. 1987. Pharmacological characterization of CGS 15943A: a novel nonxanthine adenosine antagonist. J Pharmacol Exp Ther. 242(3):784–790.
- Groenewegen A, Rutten FH, Mosterd A, Hoes AW. 2020. Epidemiology of heart failure. Eur J Heart Fail. 22(8):1342–1356. doi:10.1002/ejhf.1858.
- Guerrero A. 2018. A2A Adenosine receptor agonists and their potential therapeutic applications. an update. Curr Med Chem. 25(30):3597–3612. doi:10.2174/0929867325666180313110254.
- Headrick JP, Ashton KJ, Rose’meyer RB, Peart JN. 2013. Cardiovascular adenosine receptors: expression, actions and interactions. Pharmacol Ther. 140(1):92–111. doi:10.1016/j.pharmthera.2013.06.002.
- Headrick JP, Peart JN, Reichelt ME, Haseler LJ. 2011. Adenosine and its receptors in the heart: regulation, retaliation and adaptation. Biochim Biophys Acta. 1808(5):1413–1428. doi:10.1016/j.bbamem.2010.11.016.
- Hers I, Vincent EE, Tavaré JM. 2011. Akt signalling in health and disease. Cell Signal. 23(10):1515–1527. doi:10.1016/j.cellsig.2011.05.004.
- Hopkins AL. 2007. Network pharmacology. Nat Biotechnol. 25(10):1110–1111. doi:10.1038/nbt1007-1110.
- Hopkins AL. 2008. Network pharmacology: the next paradigm in drug discovery. Nat Chem Biol. 4(11):682–690. doi:10.1038/nchembio.118.
- Hsin KY, Ghosh S, Kitano H. 2013. Combining machine learning systems and multiple docking simulation packages to improve docking prediction reliability for network pharmacology. PLoS One. 8(12):e83922. doi:10.1371/journal.pone.0083922.
- Ismail BS, Mahmoud B, Abdel-Reheim ES, Soliman HA, Ali TM, Elesawy BH, Zaky MY. 2022. Cinnamaldehyde mitigates atherosclerosis induced by high-fat diet via modulation of hyperlipidemia, oxidative Stress, and inflammation. Oxid Med Cell Longev. 2022:4464180–4464115. doi:10.1155/2022/4464180.
- Kannel WB. 2000. Incidence and epidemiology of heart failure. Heart Fail Rev. 5(2):167–173. doi:10.1023/A:1009884820941.
- Kim Y, Shrestha R, Kim S, Kim JA, Lee J, Jeong TC, Kim JH, Lee S. 2020. In vitro characterization of glycyrol metabolites in human liver microsomes using HR-resolution MS spectrometer coupled with tandem mass spectrometry. Xenobiotica. 50(4):380–388. doi:10.1080/00498254.2019.1636418.
- Koc K, Geyikoglu F, Cakmak O, Koca A, Kutlu Z, Aysin F, Yilmaz A, Aşkın H. 2021. The targets of beta-sitosterol as a novel therapeutic against cardio-renal complications in acute renal ischemia/reperfusion damage. Naunyn Schmiedebergs Arch Pharmacol. 394(3):469–479. doi:10.1007/s00210-020-01984-1.
- Lasley RD. 2018. Adenosine receptor-mediated cardioprotection-current limitations and future directions. Front Pharmacol. 9:310. doi:10.3389/fphar.2018.00310.
- Li M, Wen Z, Xue Y, Han X, Ma D, Ma Z, Wu Z, Guan S, Sun S, Chu L. 2020. Cardioprotective effects of glycyrrhizic acid involve inhibition of calcium influx via L-type calcium channels and myocardial contraction in rats. Naunyn Schmiedebergs Arch Pharmacol. 393(6):979–989. doi:10.1007/s00210-019-01767-3.
- Li S, Zhang B. 2013. Traditional Chinese medicine network pharmacology: theory, methodology and application. Chin J Nat Med. 11(2):110–120. doi:10.1016/S1875-5364(13)60037-0.
- Li Y, Liang Y, Zhu Y, Zhang Y, Bei Y. 2018. Noncoding RNAs in cardiac hypertrophy. J Cardiovasc Transl Res. 11(6):439–449. doi:10.1007/s12265-018-9797-x.
- Liao Y, Takashima S, Asano Y, Asakura M, Ogai A, Shintani Y, Minamino T, Asanuma H, Sanada S, Kim J, et al. 2003. Activation of adenosine A1 receptor attenuates cardiac hypertrophy and prevents heart failure in murine left ventricular pressure-overload model. Circ Res. 93(8):759–766.,. doi:10.1161/01.RES.0000094744.88220.62.
- Lu S, Ye L, Yin S, Zhao C, Yan M, Liu X, Cui J, Hu H. 2019. Glycyrol exerts potent therapeutic effect on lung cancer via directly inactivating T-LAK cell-originated protein kinase. Pharmacol Res. 147:104366. doi:10.1016/j.phrs.2019.104366.
- Luan F, Rao Z, Peng L, Lei Z, Zeng J, Peng X, Yang R, Liu R, Zeng N. 2022. Cinnamic acid preserves against myocardial ischemia/reperfusion injury via suppression of NLRP3/Caspase-1/GSDMD signaling pathway. Phytomedicine. 100:154047. doi:10.1016/j.phymed.2022.154047.
- Mathoôt RA, Soudijn W, Breimer DD, Ijzerman AP, Danhof M. 1996. Pharmacokinetic-haemodynamic relationships of 2-chloroadenosine at adenosine A1 and A2a receptors in vivo. Br J Pharmacol. 118(2):369–377. doi:10.1111/j.1476-5381.1996.tb15412.x.
- McIntosh VJ, Lasley RD. 2012. Adenosine receptor-mediated cardioprotection: are all 4 subtypes required or redundant? J Cardiovasc Pharmacol Ther. 17(1):21–33. doi:10.1177/1074248410396877.
- Monda E, Lioncino M, Pacileo R, Rubino M, Cirillo A, Fusco A, Esposito A, Verrillo F, Di Fraia F, Mauriello A, et al. 2021. Advanced heart failure in special population-pediatric age. Heart Fail Clin. 17(4):673–683.,. doi:10.1016/j.hfc.2021.05.011.
- Mosterd A, Hoes AW. 2007. Clinical epidemiology of heart failure. Heart. 93(9):1137–1146. doi:10.1136/hrt.2003.025270.
- Nakamura M, Sadoshima J. 2018. Mechanisms of physiological and pathological cardiac hypertrophy. Nat Rev Cardiol. 15(7):387–407. doi:10.1038/s41569-018-0007-y.
- Nowak R, Drozd M, Mendyk E, Lemieszek M, Krakowiak O, Kisiel W, Rzeski W, Szewczyk K. 2016. A new for the isolation of ergosterol and peroxyergosterol as active compounds of hygrophoropsis aurantiaca and in vitro antiproliferative activity of isolated ergosterol peroxide. Molecules. 21(7):946. doi:10.3390/molecules21070946.
- Olah ME, Stiles GL. 1995. Adenosine receptor subtypes: characterization and therapeutic regulation. Annu Rev Pharmacol Toxicol. 35(1):581–606. doi:10.1146/annurev.pa.35.040195.003053.
- Pasquini S, Contri C, Borea PA, Vincenzi F, Varani K. 2021. Adenosine and inflammation: here, there and everywhere. Int J Mol Sci. 22(14):7685. doi:10.3390/ijms22147685.
- Peng L, Lei Z, Rao Z, Yang R, Zheng L, Fan Y, Luan F, Zeng N. 2021. Cardioprotective activity of ethyl acetate extract of Cinnamomi ramulus against myocardial ischemia/reperfusion injury in rats via inhibiting NLRP3 inflammasome activation and pyroptosis. Phytomedicine. 95:153863. doi:10.1016/j.phymed.2021.153863.
- Ribé D, Sawbridge D, Thakur S, Hussey M, Ledent C, Kitchen I, Hourani S, Li JM. 2008. Adenosine A2A receptor signaling regulation of cardiac NADPH oxidase activity. Free Radic Biol Med. 44(7):1433–1442. doi:10.1016/j.freeradbiomed.2007.12.035.
- Riehle C, Bauersachs J. 2019. Small animal models of heart failure. Cardiovasc Res. 115(13):1838–1849. doi:10.1093/cvr/cvz161.
- Savarese G, Lund LH. 2017. Global Public Health Burden of Heart Failure. Card Fail Rev. 3(1):7–11. doi:10.15420/cfr.2016:25:2.
- Scarborough EA, Uchida K, Vogel M, Erlitzki N, Iyer M, Phyo SA, Bogush A, Kehat I, Prosser BL. 2021. Microtubules orchestrate local translation to enable cardiac growth. Nat Commun. 12(1):1547. doi:10.1038/s41467-021-21685-4.
- Sedighi M, Nazari A, Faghihi M, Rafieian-Kopaei M, Karimi A, Moghimian M, Mozaffarpur SA, Rashidipour M, Namdari M, Cheraghi M, et al. 2018. Protective effects of cinnamon bark extract against ischemia-reperfusion injury and arrhythmias in rat. Phytother Res. 32(10):1983–1991. doi:10.1002/ptr.6127.
- Szentmiklosi AJ, Galajda Z, Cseppento Á, Gesztelyi R, Susán Z, Hegyi B, Nánási PP. 2015. The Janus face of adenosine: antiarrhythmic and proarrhythmic actions. Curr Pharm Des. 21(8):965–976. doi:10.2174/1381612820666141029100346.
- Tanaka N, Dalton N, Mao L, Rockman HA, Peterson KL, Gottshall KR, Hunter JJ, Chien KR, Ross J. 1996. Transthoracic echocardiography in models of cardiac disease in the mouse. Circulation. 94(5):1109–1117. doi:10.1161/01.cir.94.5.1109.
- Tian J, Shan XL, Wang SN, Chen HH, Zhao P, Qian DD, Xu M, Guo W, Zhang C, Lu R. 2022. Trans-cinnamaldehyde suppresses microtubule detyrosination and alleviates cardiac hypertrophy. Eur J Pharmacol. 914:174687. doi:10.1016/j.ejphar.2021.174687.
- Upadhyay S, Mantha AK, Dhiman M. 2020. Glycyrrhiza glabra (Licorice) root extract attenuates doxorubicin-induced cardiotoxicity via alleviating oxidative stress and stabilising the cardiac health in H9c2 cardiomyocytes. J Ethnopharmacol. 258:112690. doi:10.1016/j.jep.2020.112690.
- Wakeno M, Minamino T, Seguchi O, Okazaki H, Tsukamoto O, Okada K-i, Hirata A, Fujita M, Asanuma H, Kim J, et al. 2006. Long-term stimulation of adenosine A2b receptors begun after myocardial infarction prevents cardiac remodeling in rats. Circulation. 114(18):1923–1932. doi:10.1161/CIRCULATIONAHA.106.630087.
- Wong HS, Chen N, Leong PK, Ko KM. 2014. β-Sitosterol enhances cellular glutathione redox cycling by reactive oxygen species generated from mitochondrial respiration: protection against oxidant injury in H9c2 cells and rat hearts. Phytother Res. 28(7):999–1006. doi:10.1002/ptr.5087.
- Xu J, Bian X, Liu Y, Hong L, Teng T, Sun Y, Xu Z. 2017. Adenosine A2 receptor activation ameliorates mitochondrial oxidative stress upon reperfusion through the posttranslational modification of NDUFV2 subunit of complex I in the heart. Free Radic Biol Med. 106:208–218. doi:10.1016/j.freeradbiomed.2017.02.036.
- Xu Z, Jin Y, Gao Z, Zeng Y, Du J, Yan H, Chen X, Ping L, Lin N, Yang B, et al. 2022. Autophagic degradation of CCN2 (cellular communication network factor 2) causes cardiotoxicity of sunitinib. Autophagy. 18(5):1152–1173. doi:10.1080/15548627.2021.1965712.
- Ye H, Wei J, Tang K, Feuers R, Hong H. 2016. Drug repositioning through network pharmacology. Curr Top Med Chem. 16(30):3646–3656. doi:10.2174/1568026616666160530181328.
- Yip KH, Lau HY, Wise H. 2011. Reciprocal modulation of anti-IgE induced histamine release from human mast cells by A1 and A2B adenosine receptors. Br J Pharmacol. 164(2b):807–819. doi:10.1111/j.1476-5381.2011.01446.x.
- Zangi L, Lui KO, von Gise A, Ma Q, Ebina W, Ptaszek LM, Später D, Xu H, Tabebordbar M, Gorbatov R, et al. 2013. Modified mRNA directs the fate of heart progenitor cells and induces vascular regeneration after myocardial infarction. Nat Biotechnol. 31(10):898–907. doi:10.1038/nbt.2682.
- Zhai YJ, Liu P, He HR, Zheng XW, Wang Y, Yang QT, Dong YL, Lu J. 2015. The association of ADORA2A and ADORA2B polymorphisms with the risk and severity of chronic heart failure: a case-control study of a northern Chinese population. Int J Mol Sci. 16(2):2732–2746. doi:10.3390/ijms16022732.
- Zhan E, McIntosh VJ, Lasley RD. 2011. Adenosine A2A and A2B receptors are both required for adenosine A1 receptor-mediated cardioprotection. Am J Physiol Heart Circ Physiol. 301(3):H1183–1189. doi:10.1152/ajpheart.00264.2011.
- Zhang Y, Wernly B, Cao X, Mustafa SJ, Tang Y, Zhou Z. 2021. Adenosine and adenosine receptor-mediated action in coronary microcirculation. Basic Res Cardiol. 116(1):22. doi:10.1007/s00395-021-00859-7.
- Zhao L, Zhang H, Li N, Chen J, Xu H, Wang Y, Liang Q. 2023. Network pharmacology, a promising approach to reveal the pharmacology mechanism of Chinese medicine formula. J Ethnopharmacol. 309:116306. doi:10.1016/j.jep.2023.116306.