Abstract
Context
Dayuanyin decoction is a traditional Chinese medicine formulation that is commonly used in modern clinical practice to treat viral infections such as viral pneumonia, viral fever, influenza, and hepatitis. Although the usage rate of Dayuanyin decoction is gradually increasing in clinical practice, its pharmacological constituents are still unclear.
Objective
This study comprehensively characterized the chemical constituents in Dayuanyin decoction using ultra-performance liquid chromatography quadrupole time-of-flight mass spectrometry (UPLC-QTOF-MS) and molecular networking.
Materials and methods
The overall strategy involved retrieving structural information, such as fragment ions and precursor ion masses, from self-built databases to identify the target constituents of the Dayuanyin decoction extract. The identification of non-targeted constituents was achieved by analyzing different categories, fragment pathways, mass spectrometry data, and the relationships between clusters of structures in molecular networking. Unannotated constituents were inferred from secondary mass spectrometry similarity and molecular weight differences and annotated constituents in the same constituent cluster. A few predicted constituents were selected and validated by comparing them to reference standards under identical mass spectrometry conditions.
Results
This study preliminarily identified 216 constituents, including flavonoids, amino acids, alkaloids, triterpenes, steroidal saponins, phenylpropanoids, and other constituents.
Conclusions
This integrated strategy using UPLC-QTOF-MS and molecular networking lays the foundation for clinical research on pharmacologically active substances in Dayuanyin decoction and could be popularized for the comprehensive profiling of chemical constituents of other traditional Chinese medicines.
Introduction
Dayuanyin decoction was first documented in the ‘Plague Theory’ by Wu Youke during the Ming Dynasty. According to the ‘Plague Theory’, Dayuanyin decoction was originally designed to treat the early stages of the plague and was believed to open up membranes, remove turbidity, clear heat, and detoxify the body (Hu et al. Citation2020; Ruan et al. Citation2020; Jin et al. Citation2020). A previous study found that lamivudine and Dayuanyin decoction could inhibit the secretion of hepatitis B surface antigen (HBsAg) in the culture supernatant of HepG 2.2.15 cells at 72 h and 144 h, showing significant differences compared to the control group (p < 0.05, p < 0.01, respectively). This indicates a significant anti-HBV effect in vitro (Wang et al. Citation2012). Ren (Citation2017) reported that the water extract, the supernatant of the water-extracted alcohol precipitation of Dayuanyin decoction, and other constituents could alleviate pulmonary edema in mice with acute lung injury induced by lipopolysaccharide, reducing lung tissue damage. Experiments by the author showed that Dayuanyin decoction had a significant antipyretic effect, which was related to the decreases in interleukin-6 (IL-6) and tumor necrosis factor-alpha (TNF-α) levels in serum and decreases in myeloperoxidase activity in liver tissue (Ren et al. Citation2015). Modern physicians widely use Dayuanyin decoction to treat a variety of systemic conditions, such as fever, respiratory, digestive, urinary, and endocrine diseases, as well as skin and pediatric disorders. More importantly, Dayuanyin decoction played a significant role in the treatment of Corona Virus Disease 2019 (COVID-19) pandemic (Ding et al. Citation2020). Therefore, Dayuanyin decoction is gaining increasing attention among medical professionals and researchers.
The chemical constituents contained in Dayuanyin decoction mainly include alkaloids, flavonoids, terpenes, saponins, phenylpropanoids, and essential oils (Xiao et al. Citation2023). Currently, few studies have analyzed the constituents of a water extract of Dayuanyin decoction, as most have focused on methanol and ethanol extracts. The water extraction method is generally used for the administration of Dayuanyin decoction, making it necessary to research its water-soluble constituents. Constituent research has traditionally been conducted by building databases by collecting ion information from published studies and using that information to identify constituents. Although this method can provide accurate results, it is inefficient. In contrast, the present study used a rapid detection method, combining Agilent′ s internal database with constituent similarity provided by molecular networking. This method relied on software analysis to infer unknown constituents related to identified constituents, allowing for the quick identification of constituents in the mixture. Therefore, this method was employed to analyze the constituents in a water extract of Dayuanyin decoction and summarize the fragmentation patterns of various constituent classes.
Global Natural Product Social (GNPS) molecular networking (Nothias et al. Citation2020) is a public data analysis platform established by annotating new spectra in the existing multiple mass spectrometry databases. It includes 272 public datasets and 84 million tandem mass spectroscopy (MS/MS) spectra, providing an automated molecular networking (MN) tool for analyzing MS/MS datasets (Wang et al. Citation2016). MN utilizes mass spectrometry information and fragmentation pathways to group multiple unannotated constituents in complex matrices with known constituents based on spectral similarity. MN is formed by connecting molecules with similar fragmentation spectra to study their properties (Zhao et al. Citation2022; Beniddir et al. Citation2021). MN has been successfully applied to the discovery of novel natural products, microorganisms, fungi, and marine organisms. MN combined with UPLC-QTOF-MS is also widely used for analyzing fungal metabolites and specific constituents in traditional Chinese medicinal plants (Chen et al. Citation2021; Messaili et al. Citation2020).
UPLC-QTOF-MS has advantages that include high resolution, high sensitivity, and high accuracy, making it an indispensable method for exploring chemical substances in traditional Chinese medicine (Liu et al. Citation2013). However, the processing of large amounts of data obtained from MS/MS is both time-consuming and complex. Therefore, this study employed a high-throughput and highly sensitive UPLC-QTOF-MS combined with the MN method to rapidly elucidate the structures of non-targeted and complex constituents in the traditional Chinese medicine Dayuanyin decoction. Additionally, an internal database was utilized to identify known and unknown constituents in the Dayuanyin decoction based on constituent similarity, achieving the comprehensive identification of the chemical constituents present in the Dayuanyin decoction formulation.
Materials and methods
Chemicals and reagents
Acetonitrile (LC-MS-grade) and methanol (HPLC-grade) were purchased from Merck (Darmstadt, Germany). LC-MS-grade formic acid was purchased from Sigma-Aldrich (St. Louis, MO, USA; cat. no. 197147).
Plant material
Areca catechu L. (Arecaceae) (origin: Hainan, batch number 22011905); Anemarrhena asphodeloides Bge. (Liliaceae) (origin: Henan, batch number 22081104); Glycyrrhiza uralensis Fisch. (Fabaceae) (origin: Inner Mongolia, batch number 22062406); Scutellaria baicalensis Georgi (Lamiaceae) (origin: Shanxi, batch number 22032405); Magnoliae officinalis Rehd. et Wils. (Magnoliaceae) (origin: Sichuan, batch number 22092307); and Amommum tsao-ko Crevost et Lemarie (Zingiberaceae) (origin: Guangxi, batch number 21041507) were purchased from Hunan Zhenxing Traditional Chinese Medicine Co., Ltd (Hunan, Changsha, China). Paeonia lactiflora Pall. (Paeoniaceae) (origin: Zhejiang, batch number 20220802) was purchased from Hunan Jinxiang Pharmaceutical Co., Ltd. These herbal medicines were all purchased on October 9, 2022. All the above medicinal materials were authenticated by Professor Liu Shao from the Pharmacy Department of Xiangya Hospital, Central South University.
Sample preparation
A 180 g sample of Areca catechu (seeds), 90 g of Magnoliae officinalis (bark), 45 g of Amommum tsao-ko (fruit), 90 g of Anemarrhena asphodeloides (rhizoma), 90 g of Paeonia lactiflora (roots), 90 g of Scutellaria baicalensis (roots), and 45 g of Glycyrrhiza uralensis (roots and rhizoma) were mixed in a distillation flask. Five times the total weight of water was added for soaking for 30 min. Reflux extraction was performed for 1 h (timing was started after boiling). After filtration, 8 times its water weight was added to the residue for another 1 h of extraction, and it was filtered again.
Framework of the integrated strategy
The purpose of this study was to preliminarily determine the chemical constituents in Dayuanyin decoction. The total ion flow chart obtained by mass spectrometry and the constituent numbers indicating segmented annotations are shown in . A strategy of UPLC-QTOF-MS combined with GNPS molecular networking was used for the targeted screening of known constituents to achieve a more efficient identification method. MN was used for the structural classification of non-target tissues based on MS/MS spectral similarity. Starting from the nodes of known constituents, adjacent unreported constituents in the visualized network were determined through detailed analysis of MS/MS spectra and online database retrieval. The specific strategic process is illustrated in .
Figure 1. Total ion chromatogram of Dayuanyin decoction water extract in the positive ion mode (a). TIC of water extract of Dayuanyin decoction in the positive ion mode for 0–11 min, 11–23 min, and 23–40 min (b, c, and d).
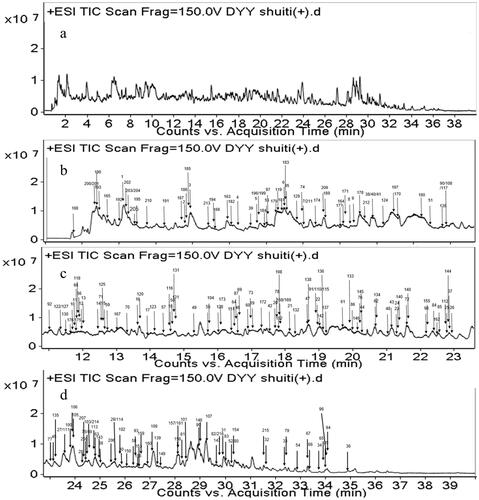
First, target constituents were identified by compiling and summarizing the mass spectrometry information of relevant constituents in Dayuanyin decoction by collating data from journal platforms, such as the Web of Science (https://webofscience.clarivate.cn), the National Library of Medicine (https://pubmed.ncbi.nlm.nih.gov), the China National Knowledge Infrastructure (https://www.cnki.net/), and the Wanfang Data Knowledge Service Platform (https://www.wanfangdata.com.cn/). The data retrieved included constituent names, relative molecular mass, ion modes, parent ion m/z values, and MS/MS spectrometry fragments to establish a constituent database. Second, the Agilent MassHunter Qualitative Analysis B08.00 software′ s library matching function was utilized to match the mass spectrometry data of the Dayuanyin extract with the traditional Chinese medicine (TCM) and Metlin databases embedded in the software. Constituents with scores of ≥80 were selected. Finally, the matched constituents were compared with the fragment ions in the self-built database to identify the corresponding constituents.
The identification of non-target constituents was achieved by GNPS molecular networking. The identification of unannotated constituent nodes in the formed constituent clusters was based on the similarity of mass spectrometry features to constituents of the same type. The following steps were followed: First the elemental composition of the unannotated points was automatically deduced using the formula predictor in Qualitative Navigator B.08.00 software. The elements carbon (C), hydrogen (H), oxygen (O), nitrogen (N), and sulfur (S) were selected to calculate the elemental composition of the constituents. Only the highest-scoring and reasonable combinations were considered. In the second step, the predicted molecular formula was input into SciFinder to search for possible constituents. If a match was found, the structure of the unknown constituents could be determined based on the structures of the annotated points in the cluster, fragmentation patterns, and the differences in fragment ions that may exist between possible constituents. In the third step, Cytoscape 3.9.2 was used to visualize and edit the entire molecular networking dataset. These steps enabled the identification of unknown constituent structures within the MN results.
UPLC-QTOF-MS analysis
The chromatographic evaluation was performed using an Agilent 1290 series UPLC system (Agilent Corp., Santa Clara, CA, USA) equipped with a binary pump, micro degasser, autosampler, and temperature-controlled column compartment. The sample was separated on an Agilent Eclipse C Plus C18 column (1.8 μm, 2.1 × 100 mm). The mobile phase was composed of two solvents (A and B). The mobile phase (A) was aqueous formic acid (0.1%, v/v), and the mobile phase (B) was acetonitrile. The following mobile phase gradient was used: 0–25 min: 5-35% B; 25–35 min: 35–95% B; and 35–40 min: 95% B. A positive ionization mode was employed in this experiment to ionize the constituents of the Dayuanyin decoction extract. The flow rate was 0.3 mL/min, the injection volume was 15 μL, and the column temperature was set at 40 °C.
Then, the analytes were passed through an Agilent 6545 A Q-TOF mass spectrometer (Agilent Corp.) equipped with an electrospray ionization (ESI) interface. The operating parameters were as follows. The drying N2 gas flow rate was 8 L/min, the temperature was 320 °C, the nebulizer was set at 35 PSI, the capillary energy was set to 3500 V, the MS/MS data acquisition mode was set to auto MS/MS, the max precursor per cycle was 5, and the acquisition time was 200 ms/spectrum. The samples were analyzed in the positive ion mode, and mass spectra data were recorded across a range of m/z 100–1700. Reference masses of 121.0509 (purine) and 922.0098 (HP-0921) were utilized for the internal mass calibration during runs in the positive ion mode. Fixed collision energies of 10.00, 20.00, and 40.00 V were chosen at a scan rate of 4.0 spectra/s using auto MS/MS data acquisition with a medium MS/MS isolation width.
Molecular networking analysis
The original MS/MS spectral data were converted into an mzML format that contained all of the analysis information. Then, the mzML format file was uploaded to the client using FileZilla software and imported into the GNPS database for analysis (Chen et al. Citation2021; Rodrigues et al. Citation2022). Finally, based on the matched results, the MS/MS spectra were compared for similarity, identical ion fragments, and neutral losses to identify suitable constituents. The optimum parameters were as follows: parent mass and fragment tolerance of 0.02 Da, cosine score of ≥0.7, matched peaks ≥4, maximum connected constituent size of 100, minimum cluster size of 1, and no run MSCluster. Lastly, the results were downloaded and saved, and the GNPS results were visualized using Cytoscape 3.9.2 software [https://cytoscape.org]. The identification of the constituents was supported by GNPS spectral libraries. The MS/MS spectra of the Dayuanyin decoction constituents were compared to the MS/MS spectra of the constituents contained in the GNPS library platform using the following parameters: a minimum number of library search-matched peaks of 4, a score threshold of 0.7, and a maximum analog search mass difference of 100.
Results
Targeted identification results
The UPLC-QTOF-MS analysis of the water extract of Dayuanyin decoction was performed, with a detection time of 40 min. The error range was controlled within 5 ppm, and the matching degree was ≥90%. A total of 36 target constituents were identified in the extract through fragment ion information, TCM (https://www.tcmdatabase.com/), and Metlin databases (https://metlin.scripps.edu/landing_page.php?pgcontent=mainPage), which were mainly flavonoids and alkaloids. Specific information, including the constituent′ s names, retention times, formulas, precursor ions, and fragment ions, appears in .
Table 1. Characterisation of constituents in water extract of dayuanyin decoction by UPLC-QTOF-MS and MS/MS analysis in positive ion mode.
Untargeted identification results in MN
In this study, constituents with similar spectra were grouped into the same cluster. The relationships among the network nodes can be visualized using Cytoscape 3.9.2. Therefore, MN can be used to discover complex crude extracts of traditional Chinese medicine, effectively avoiding the drawbacks of low separation efficiency, high cost, and repetitive constituent isolation in traditional methods. In this study, a total of 24 clusters were analyzed, resulting in the identification of 180 constituents through MN analysis (). The constituent′ s names, retention times, molecular formulas, precursor ions, and fragment ions are shown in .
Table 2. The constituents in water extract of dayuanyin decoction annotated by UPLC-QTOF-MS and molecular network.
Unannotated analysis points of flavonoid constituents in MN
Flavonoid aglycone
The fragmentation of flavonoid constituents mainly involves the cleavage of glycosidic bonds and rings in the MS/MS spectra. In this study, we selected quercetin 3-galactoside and licoflavone C as representative constituents to investigate the MS/MS fragmentation patterns of flavonoids. Quercetin 3-galactoside (C21H20O12) easily forms a quasi-molecular ion [M + H]+ at m/z 465.1027 in the positive ion mode. The precursor ion loses one glucose molecule to generate a fragment ion at m/z 303.0483 ([M + H–C6H10O5]+). This fragment ion can undergo various types of fragmentation. One fragmentation pathway involves breaking the [0,4] bonds within the C ring, resulting in the formation of a fragment ion at m/z 109.0729 ([0,4A]+). Another possible fragmentation pathway is the cleavage of the chemical bond at the [1,3] positions, leading to the formation of fragment ions at m/z 153.0167 ([1,3A]+) and 149.0167 ([1,3B]+). Additionally, the fragment ion at m/z 303.0483 can undergo cleavage of the [1,4] chemical bond, generating a fragment ion at m/z 127.0373 (C6H6O3, [1,4A]+). Lastly, the fragment ion at m/z 303.0483 can also undergo cleavage of the [0,2] chemical bond, resulting in the formation of fragment ions at m/z 137.0219 and 165.0165 ([M + H–C6H10O5–C7H6O3]+). For licoflavone C, in the positive ion mode, the extracted ion chromatogram (EIC) of m/z 339.1227 (C20H18O5, [M + H]+) predominantly undergoes the loss of a tert-butyl group from the A ring to generate a fragment ion at m/z 283.0596 ([M + H–C4H8]+). This fragment ion can further undergo fragmentation at either the [1,3] bond or the [0,2] bond to produce the corresponding fragments. The former fragmentation results in a fragment ion at m/z 165.0171 ([M + H–C8H6O]+), whereas the latter generates a fragment ion at m/z 121.0281 (C7H5O2, [M + H]+). Additionally, the fragment ion at m/z 165.0171 can further lose a carbon monoxide (CO) molecule to yield a fragment ion at m/z 137.0230 ([M + H-C8H6O–CO]+). In addition to glycosides and oxygenated glycosides, the flavonoid constituents in Dayuanyin water decoction also included carbonated glycosides. Carbonated glycoside fragmentation analysis was conducted using isoschaftoside as an example. In the positive ion mode, the constituents initially form a quasi-molecular ion at m/z 565.1559 ([M + H]+). Subsequently, the sugar moiety attached to the flavonoid aglycone undergoes fragmentation. Following the consecutive loss of C3H6O3 and CH2O groups, characteristic fragment ions at m/z 445.1119 ([M + H–C3H6O3–CH2O]+) and 325.0700 ([M + H–2C3H6O3–2CH2O]+) are generated. The MS/MS spectrum is displayed above. A new constituent, 5,7-dihydroxy-2-phenyl-6-(3,4,5-trihydroxy-6-(hydroxymethyl)tetrahydro-2H-pyran-2-yl)-8-(3,4,5-trihydroxy-6-methyltetrahydro-2H-pyran-2-yl)-4H-chromen-4-one, might appear in carbonated glycosides. A comparison of this constituent to the hypothesized chrysin 6,8-di-C-glucoside showed that they both had the same characteristic fragment ions at m/z 441, 363, 321, 309, indicating that their structures were essentially similar. Additionally, as the molecular ions of the two constituents differed by 16 Da, it can be inferred that an oxygen (O) atom was missing from the OH group of the glucose moiety. Therefore, it is likely that constituent is 5,7-dihydroxy-2-phenyl-6-(3,4,5-trihydroxy-6-(hydroxymethyl)tetrahydro-2H-pyran-2-yl)-8-(3,4,5-trihydroxy-6-methyltetrahydro-2H-pyran-2-yl)-4H-chromen-4-one mentioned in the previous text. Among the studied constituent clusters, the flavonoid constituents mainly originated from MN1 to MN10. The structural formulas of flavonoid constituents in Dayuanyin water decoction are shown in and , and the mass spectrometry information is provided in .
Unannotated analysis points of terpenoid constituents in MN
The terpenoid constituents in Dayuanyin water decoction mainly included monoterpenes and sesquiterpenes, primarily derived from Paeonia lactiflora. Taking albiflorin (C23H28O11) as an example, its quasi-molecular ion peak in the positive ion mode is observed at m/z 481.1709. First, the molecular ion peak loses one glucose molecule, resulting in a fragment ion at m/z 319.1181. Then, the ester bond and the adjacent C-C bond of benzoic acid break, giving rise to two fragment ions: one at m/z 123.0798 (C7H6O2, [M + H–C10H12O4]+), representing the fragment of benzoic acid, and another at m/z 197.0760 (C10H12O4, [M + H-C6H10O5–C7H6O2]+). The former further loses one water molecule, generating a fragment ion at m/z 105.0297, while the latter sequentially loses one water molecule and CO, resulting in fragment ions at m/z 179.0650 and m/z 151.0709. The relevant fragmentation pathways are shown in . The constituent oxypaeoniflorin, which lacks annotated markers, exhibits secondary fragment ions similar to those of albiflorin, including m/z 197/179/151. Additionally, the ions m/z 335 and m/z 121 are both 16 Da higher than the secondary fragment ions m/z 319 and m/z 105 present in albiflorin, suggesting the presence of a hydroxyl group on the benzene ring. The hypothesized molecular formula was matched with constituents in Scifinder, ultimately confirming that the annotated marker corresponded to oxypaeoniflorin. This class of constituents exhibits primary fragment ions, including m/z 197/179/151/105, such as benzoylpaeoniflorin, (1 R,2S,5S,5R)-5b-[(benzyloxy)-methyl]tetrahydro-5-hydroxy-2-methyl-2,5-methano-1H-3,4-dioxacyclobuta[cd]pentalen-1a(2H)-yl, oxypaeoniflorin, and paeoniflorgenin, which all contain these fragments. Therefore, it can be presumed that these constituents belong to the same class. The monoterpenoid constituents in this study primarily originated from MN1 and MN11. The relevant constituent information is shown in , and their structural formulas are shown in .
Unannotated analysis points of phenylpropane constituents in MN
The main phenylpropane constituents identified in the Dayuanyin decoction were phenylpropanol, phenylpropanoic acid, and lignan constituents derived from Magnoliae Officinalis. The lignan constituents tended to lose functional groups such as allyl, caffeoyl, and CO during the fragmentation process. The example used for fragmentation was honokiol. In positive ion mode detection, the quasi-molecular ion peak formed by honokiol is m/z 267.1390 ([M + H]+). After losing one molecule of allyl, the fragment ion m/z 225.0763 is generated ([M + H–C3H6]+), followed by the loss of one molecule of C2H2, resulting in a secondary fragment at m/z 199.0603. The quasi-molecular ion of honokiol can further lose one molecule of CH4 and C3H4, generating fragments at m/z 251.0919 and m/z 211.0609, respectively. It can also lose one molecule of C3H6, producing a fragment ion at m/z 226.0840 ([M + H–C3H6]+). Another category is simple phenylpropanes. Using chlorogenic acid (C16H18O9) as the reference constituent, its quasi-molecular ion is m/z 355.102. Initially, the benzoyloxy group of phenylpropanoic acid breaks adjacent to the C atom, generating two possible fragment ions: m/z 163.0384 ([M + H–C7H12O6]+) and m/z 193.0503 ([M + H–C9H6O3]+). The former can undergo two possible fragmentation pathways: one is the removal of one molecule of water from the ortho-benzodihydroxy group, generating a fragment at m/z 145.0274, and the other is the consecutive loss of CO and H2O, resulting in secondary fragments at m/z 135.0434 and m/z 117.0329, respectively. These simple phenylpropane constituents produce characteristic ions of [M + H–C7H12O6]+, [M + H–C7H12O6–CO]+, and [M + H–C7H12O6–CO–H2O]+. MN1 and MN12 of the Dayuanyin decoction extract contained phenylpropane constituents. Information related to the constituents is shown in , and the fragmentation pathways of the constituents are shown in .
Unannotated analysis points of saponin constituents in MN
Basic structure of the steroid
The saponin constituents in Dayuanyin decoction mainly included steroidal saponins and triterpenoid saponins, which are susceptible to losing sugar groups, sugar aldehyde groups, H2O, CH3, and side chain groups. These saponins are derived from Anemarrhena asphodeloides and Glycyrrhiza uralensis. Taking tomatoside A (C51H86O24) as an example for fragmentation analysis, it generates a dehydrated positive ion at m/z 1065.5534 ([M + H–H2O]+) in the positive ion mode. Subsequently, it sequentially loses four glucose molecules, resulting in m/z 903.4923 ([M + H–H2O–Glu]+), m/z 741.4403 ([M + H–H2O–2Glu]+), m/z 579.3877 ([M + H-H2O–3Glu]+), and m/z 417.3354 ([M + H–H2O–4Glu]+). Then, the oxygen-containing heterocycle is removed, resulting in a secondary fragment ion at m/z 417.3354 ([M + H-H2O–4Glu–C8H16O2]+). Further removal of one water molecule leads to a fragment at m/z 255.2109. The basic carbon scaffold of steroidal constituents is derived from spirostanes, with the F ring forming a cyclic or chain structure. The fragmentation pattern mainly involves the consecutive cleavage of glycosidic bonds on the A or F rings, as well as the loss of two oxygen-containing rings (–C8H16O2). For triterpenoid saponins, taking liquorice (C42H62O16) as an example for fragmentation analysis, the constituent exhibits a quasi-molecular ion at m/z 823.4116 in the positive ion mode. The fragmentation pattern of this constituent was similar to that of tomatoside A. It first loses two molecules of glucuronic acid consecutively, generating fragment ions at m/z 647.3780 ([M + H–GluA]+) and m/z 471.3444 ([M + H–2GluA]+). Then, the fragment ion loses one water molecule to produce a fragment at m/z 453.3373. Finally, the carboxyl group on the pentacyclic ring is cleaved, resulting in a secondary fragment ion at m/z 407.3283 ([M + H–2GluA–H2O–HCOOH]+). Additionally, m/z 353.0714 ([M + H–C30H46O4]+) is a fragment ion of tomatoside A obtained after the removal of the entire pentacyclic ring structure, leaving two glucuronic acid aldehyde groups. Subsequently, the removal of one molecule of glucuronic acid generates a fragment ion at m/z 195.1704. Finally, the carboxyl group is lost, resulting in a secondary fragment ion at m/z 149.1319 ([M + H–C30H46O4–C6H6O5–HCOOH]+). In this study, a possible new constituent of steroidal saponins was discovered, named 4,5-dihydroxy-6-((10-hydroxy-6a,8a,9-trimethyl-10-(3-methyl-4-((3,4,5-trihydroxy-6-(hydroxymethyl)tetrahydro-2H-pyran-2-yl)oxy)butyl)octadecahydro-1H-naphtho[2′,1′: 4,5]indeno[2,1-b]furan-4-yl)oxy)-3-((3,4,5-trihydroxy-6-(hydroxymethyl)tetrahydro-2H-pyran-2-yl)oxy)tetrahydro-2H-pyran-2-yl)methyl acetate. Its quasi-molecular ion was m/z 945.5043 ([M + H–H2O]+). Similar to tomatoside A, it consecutively lost two Glu molecules, resulting in a fragment ion at m/z 621.4004, which was 42 Da higher than the ion of tomatoside A (m/z 579.3877). Furthermore, combining the following secondary fragments at m/z 417, m/z 273, and m/z 255, which are the same as in tomatoside A, it can be inferred that there is likely a group of 42 Da connected to the glucose group in the structure of tomatoside A. It is speculated that the hydroxyl group on the glucose was esterified, forming a CH3COO- group. The fragmentation pathway of these constituents is illustrated in . The triterpenoid saponin constituents identified in Dayuanyin decoction were predominantly the oleanane type, characterized by a relatively stable pentacyclic ring structure that is not easily cleaved. Fragmentation mainly involves the cleavage of the entire glucuronic acid group and the chemical bonds between glucuronic acid units. In this study, steroidal and triterpenoid constituents primarily originated from the MN13–MN15 constituent clusters. The chemical structures of these two constituent classes are depicted in , and relevant information is found in .
Unannotated analysis points of isoquinoline alkaloid constituents in MN
The alkaloids in Dayuanyin decoction were primarily identified in the positive ion mode, and their main sources were Areca catechu L. and Magnoliae officinalis. Tetrahydrobenzylisoquinoline alkaloids were predominantly present. Asimilobine and (–)-lotusine were used as examples for illustration purposes. Taking asimilobine as an example, its quasi-molecular ion peak in the positive ion mode is observed at m/z 268.1332. First, the N atom of the secondary amine breaks off from the adjacent two carbons, resulting in the loss of one molecule of NH3 and the generation of a fragment ion at m/z 251.1053 ([M + H–NH3]+). Then, the adjacent hydroxyl group (OH) and methoxy group (OCH3) on the phenyl ring detach one molecule of CH3OH, resulting in the formation of an oxygen-containing heterocyclic structure and a fragment ion at m/z 219.0788 ([M + H–NH3–CH3OH]+). Finally, the removal of one molecule of CO produces an MS/MS fragment ion at m/z 191.0843. (–)-Lotusine is a quaternary ammonium alkaloid, and it generates a quasi-molecular ion at m/z 314.1754 ([M]+) in the positive ion mode. It subsequently loses a dimethylamine group to form a fragment ion at m/z 269.1169. This ion can undergo multiple fragmentation pathways. First, it can sequentially lose a molecule of CH3OH and CO, resulting in fragment ions at m/z 237.0900 and m/z 209.0949 ([M–NH(CH3)2–CH3OH–CO]+), respectively. Second, ethyl group cleavage occurs between the two benzene rings, producing fragment ions at m/z 175.0749 ([M–NH(CH3)2–C6H6O]+) and m/z 107.0491 ([M–NH(CH3)2–C10H10O2]+). The former further eliminates a CH3OH molecule from the adjacent functional groups on the benzene ring, yielding a secondary fragment at m/z 143.0487. The main mass spectrometry fragmentation pathways of the alkaloid constituents in Dayuanyin decoction were the loss of N-containing groups, the condensation of adjacent O-containing groups on the benzene ring to form an O-containing ring, and the loss of CO. The possible new constituents in the alkaloid constituents had a molecular ion at m/z 266.1178, which could be either 1-hydroxy-5,6,6a,7-tetrahydro-4H-dibenzo[de,g]quinoline-2-carbaldehyde or 2-hydroxy-5,6,6a,7-tetrahydro-4H-dibenzo[de,g]quinoline-1-carbaldehyde. When compared to asimilobine, both had the same fragment ions at m/z 219 and 191. One of the fragment ions in the new constituents, at m/z 249, was 2 Da less than that of asimilobine, suggesting a reduced number of hydrogen atoms on the parent nucleus and an increased degree of unsaturation. Considering the difference between CH2OH and CHO, the position of CHO may differ in the two constituents. Although the secondary mass spectra of the two constituents were the same, the difference in the connection positions of CHO and CH2OH suggested the existence of two different constituents. The alkaloid constituents mainly belonged to the MN16 and MN17 clusters. The chemical structures of the relevant constituents are shown in . provides the related mass spectrometry information.
Unannotated analysis points of N-containing constituents in MN
This section mainly focuses on two types of N-containing constituents, including guanosine and amino acids. Taking guanosine, tetrahydroharman-3-carboxylic acid, and N-fructosyl phenylalanine as examples, guanosine exhibits a molecular ion peak at m/z 284.0971 ([M + H]+) in the positive ion mode. It first loses a pentose molecule, generating a fragment ion at m/z 152.0564. Subsequently, it undergoes various fragmentation pathways. The first pathway involves the simple loss of an NH3 molecule, resulting in a fragment ion at m/z 135.0295. The second pathway involves the cleavage of a six-membered ring containing nitrogen, leading to the elimination of an (NH2)2CH2 molecule. The remaining portion forms a cyclic lactam with four carbons, producing a fragment ion at m/z 110.0346. The third pathway involves the loss of CO from the six-membered ring, yielding a fragment ion at m/z 124.0388. This type of nucleoside has the same characteristic ions at m/z 135/110. Another adenine-based constituent, which is similar to guanosine in its basic core structure but differs by having an additional N-containing group in the six-membered ring and lacking a carbon-oxygen double-bond, exhibits common secondary ions at m/z 136/119. Tetrahydroharman-3-carboxylic acid and N-fructosyl phenylalanine were selected as reference constituents for the fragmentation patterns of amino acids in this study. Tetrahydroharman-3-carboxylic acid easily undergoes dehydration in the positive ion mode, producing a fragment ion at m/z 213.0812. Subsequently, further ring opening of the N-containing six-membered heterocyclic structure results in the formation of constituents with a fused benzopyrrole structure at m/z 158.0964. Finally, the loss of an ethylene molecule yields a fragment ion at m/z 130.0654. Another fragmentation pathway involves the direct cleavage of the N-containing six-membered heterocyclic structure on the precursor ion, resulting in a fragment ion at m/z 188.0804 ([M + H–NH = CHCH3]+). N-fructosyl phenylalanine (m/z 328.139, [M + H]+) exhibits three different fragmentation pathways. First, the constituents undergo cleavage of the pentose moiety, generating a fragment ion with a carbon-nitrogen double-bond at m/z 178.0863. Alternatively, consecutive losses of H2O and CO lead to the formation of secondary fragments at m/z 310, 282, 292, and 264. Carbon-nitrogen single-bond cleavage generates a fragment ion with an amino acid structure at m/z 166.0863 ([M + H–C6H5O10]+), followed by the loss of an HCOOH molecule, resulting in a fragment ion at m/z 120.0808. In this study, amino acid constituents were derived from MN18–MN22 constituent clusters, whereas adenine constituents originated from the MN21 cluster. The chemical structures of these constituents are shown in , and relevant information is provided in .
Unannotated analysis points of other constituents in MN
Other constituents identified in Dayuanyin decoction included phenols, phenolic glycosides, fatty acid chains, and other constituents. A few examples follow. 2,3,5-Trimethoxyphenol is a simple phenol constituent, and its quasi-molecular ion appears at m/z 185.0807 ([M + H]+) in the positive ion mode. It can lose a methyl group (CH3) to generate a fragment ion at m/z 170.0565. Alternatively, the condensation of adjacent OH and OCH3 groups on the benzene ring can occur, followed by the loss of a molecule of CH3OH, resulting in a fragment ion at m/z 153.0546. The cyclic structure containing oxygen loses a molecule of CO, generating a secondary fragment ion with a cyclopentadiene structure at m/z 125.0596 ([M + H–CH3OH–CO]+). Another fragmentation pathway is similar to the previous one, where two adjacent OCH3 groups on the benzene ring undergo condensation. The resulting cyclic structure with oxygen then loses a CO molecule, producing a fragment ion at m/z 111.0438 ([M + H–CH3OCH3–CO]+). In this study, other class constituents were mainly found in the MN1 and MN23–MN24 clusters. Specific information about the constituents appears in , and their structural formulas are shown in and .
Figure 11. Structure of the MN23–MN24 constituents and the possible fragmentation pathway of representative constituents.
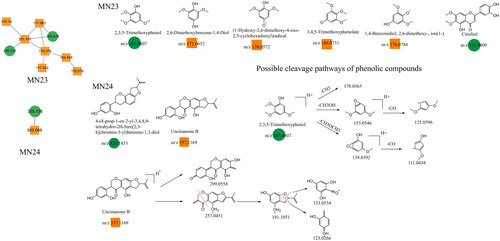
The MN of the Dayuanyin decoction extract was generated using the GNPS method. A total of 74 clusters were generated from the extract, out of which 24 were analyzable. Thirty-six constituents were found to match constituents in the database. The 24 clusters from the Dayuanyin decoction extract were visualized using Cytoscape 3.9.2, as shown in . In the figure, labeled nodes are represented by circles, while inferred unlabeled nodes are represented by squares. Each node represents a constituent, with the molecular weight of the parent ion indicated inside the node. In this study, magnoflorine, 3′-demethylnobiletin, and adenosine were inferred as unlabeled points in MN. Therefore, these three constituents were selected to verify the accuracy of MN inference using reference standards along with literature matching. The sample was then analyzed using the chromatographic conditions employed for the Dayuanyin decoction extract, and the observed secondary fragments were compared with the three inferred constituents to determine if they matched.
Validation of MN results
This study selected three unannotated nodes in MN belonging to the classes of flavonoids, adenosines, and alkaloids to ensure the correctness of the speculated constituents: 3′-demethylnobiletin, adenosine, and magnoflorine. These three constituents were chosen because they are representatives of different and abundant constituent types and are readily available for purchase. First, these three constituents were dissolved in methanol to prepare control solutions, each at a concentration of 1.0 mg/mL. Then, MS and MS/MS spectrometry data were obtained by UPLC-QTOF-MS analysis. The observed secondary fragments were compared with the three inferred constituents to determine if they matched. The results in and demonstrate that the retention time and ion fragments of the reference substances matched those of the predicted constituents, confirming the accuracy of our method. We used MN analog clustering to identify constituents with similar fragmentation pathways.
Figure 12. The extracted ion chromatogram and MS/MS spectrum of three constituents of the standard reference substance and the Dayuanyin decoction extract.
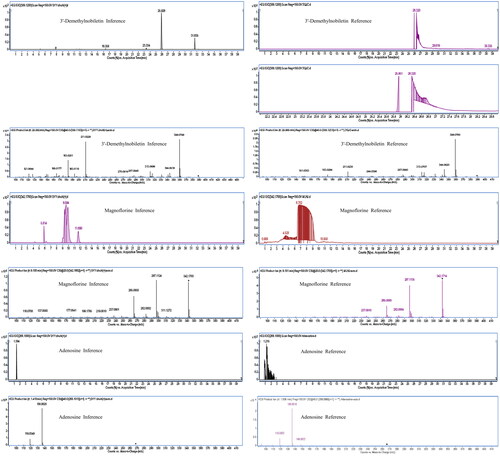
Table 3. Mass spectrum/chromatographic information of the constituents in the standard reference substance and dayuanyin decoction extract.
Discussion
The combination of UPLC-QTOF-MS and GNPS molecular networking was a fast and reliable method for analyzing the constituents of Dayuanyin decoction extract. This approach overcomes the high cost and low-efficiency issues associated with traditional methods for discovering constituents in traditional Chinese medicine and may promote the discovery of new constituents of traditional Chinese medicine. The identified unknown constituents can be validated using standard substances based on their retention time and fragment ions, thereby avoiding the complexities involved in the initial identification of novel constituents. This study identified a total of 216 constituents in Dayuanyin decoction. The main constituents were flavonoids, amino acids, alkaloids, triterpenes, steroidal saponins, phenylpropanoids, and other constituents, primarily attributed to the high polarity of constituents in aqueous extracts. Relatively fewer constituents from Amommum tsao-ko were found, which may have been due to the easy loss of its main active constituent, essential oil, during the extraction process and the limitations of UPLC-QTOF-MS. In the future, gas chromatography and other techniques can be used to study the volatile constituents of Dayuanyin decoction to improve research into its foundational components. However, his study also had certain limitations. The positive ion mode was chosen for analyzing the Dayuanyin decoction water extract. A comparison of the possible constituents under positive and negative ion modes found that the positive ion mode showed a wider variety of constituents and also covered the constituents found in the negative ion mode. However, some constituents may only be detected in negative ion mode and may not appear in positive ion mode. Thus, some constituents may have been missed in the positive ion mode analysis. Due to the limitations of the MN database, some constituents in certain clusters could not be identified because they lacked annotations. Additionally, several types of constituents may be present within the same constituent′s cluster, possibly due to partial similarities in their secondary fragments, leading them to be categorized under the same constituent′s cluster by default. Ultimately, the constituents identified in this study need to be further isolated for in vitro or in vivo activity screening.
Conclusions
This study established a strategy based on UPLC-QTOF-MS combined with GNPS to analyze 216 constituents in Dayuanyin decoction extract successfully. Among them, 36 constituents were identified by matching against Agilent and Metlin databases, as well as a self-built database compiled from the literature and PubChem. One hundred and eighty constituents were identified from 24 clusters using MN provided by GNPS. These constituents included flavonoids, amino acids, alkaloids, triterpenes, steroidal saponins, phenylpropanoids, and other constituents. The fragmentation patterns of representative constituents of each type were also analyzed, providing a material basis and scientific evidence for the further development and utilization of Dayuanyin decoction.
Credit authorship contribution statement
Jing Peng: Methodology, Investigation, Data curation, Writing - Original draft preparation, Reviewing and Editing, Visualization; Chengyu Ge: Investigation, Data curation; Kaiqi Shang: Conceptualization, Data curation, Visualization; Shao Liu: Conceptualization, Resources, Funding acquisition; Yueping Jiang: Conceptualization, Resources, Funding acquisition.
Disclosure statement
The authors confirm that there are no conflicts of interest.
Additional information
Funding
References
- Beniddir MA, Kang KB, Genta-Jouve G, Huber F, Rogers S, van der Hooft JJJ. 2021. Advances in decomposing complex metabolite mixtures using substructure- and network-based computational metabolomics approach. Nat Prod Rep. 38(11):1967–1993. doi: 10.1039/d1np00023c.
- Chen S, Huang G, Liao W. 2021. Discovery of the bioactive peptides secreted by Bifidobacterium using integrated MCX coupled with LC-MS and feature-based molecular networking. Food Chem. 347:129008.
- Ding RC, Long QH, Wang P, Liu L, Huang XY, Ming SP. 2020. Experience of using Dayuanyin decoction in the treatment of novel coronavirus pneumonia. J Trad Chin Med. 61:1481–1484 + 1511.
- Hu C, Liang M, Gong F. 2020. Efficacy of Lian Hua Qing Wen compared with conventional drugs in the treatment of common pneumonia and COVID-19 pneumonia: a meta-analysis: evid based. Complement Alternat Med. Vol. 2020: 5157089.
- Jin YH, Cai L, Cheng ZS, Cheng H, Deng T, Fan YP. 2020. Rapid advice guidelines for the diagnosis and treatment of pneumonia caused by a novel coronavirus (2019-nCoV) (Standard Edition). PLA Med J. 45(01):1–20.
- Liu M-H, Tong X, Wang J-X, Zou W, Cao H, Su W-W. 2013. Rapid separation and identification of multiple constituents in traditional Chinese medicine formula Shenqi Fuzheng injection by ultra-fast liquid chromatography combined with quadrupole-time-of-flight mass spectrometry. J Pharm Biomed Anal. 74:141–155. doi: 10.1016/j.jpba.2012.10.024.
- Messaili S, Colas C, Fougère L. 2020. Combination of molecular networking and centrifugal partition chromatography fractionation for targeting and identifying Artemisia annua L. antioxidant constituents. J Chromatogr A. 1615:460785.
- Nothias LF, Petras D, Schmid R, Dührkop K, Rainer J, Sarvepalli A, Protsyuk I, Ernst M, Tsugawa H, Fleischauer M, et al. 2020. Feature-based molecular networking in the GNPS analysis environment. Nat Methods. 17(9):905–908. doi: 10.1038/s41592-020-0933-6.
- Ren HL. 2017. The therapeutic effect of Dayuanyin decoction on lipopolysaccharide-induced acute lung injury in mice [degree thesis]. Soochow University.
- Ren HL, Yan B, Liang ZT, Zhao ZZ, Dong Y, Zhang J. 2015. Study on the antipyretic effect of Dayuanyin decoction and UPLC-Q-TOF/MS analysis. Chin Patent Med. 37(01):131–137.
- Rodrigues AMS, Lami R, Escoubeyrou K, Intertaglia L, Mazurek C, Doberva M, Pérez-Ferrer P, Stien D. 2022. Straightforward N-acyl homoserine lactone discovery and annotation by LC-MS/MS-based molecular networking. J Proteome Res. 21(3):635–642. doi: 10.1021/acs.jproteome.1c00849.
- Ruan X, Du P, Zhao K, Huang J, Xia H, Dai D, Huang S, Cui X, Liu L, Zhang J. 2020. Mechanism of Dayuanyin decoction in the treatment of coronavirus disease 2019 based on network pharmacology and molecular docking. Chin Med. 15(1):62. doi: 10.1186/s13020-020-00346-6.
- Wang M, Carver JJ, Phelan VV, Sanchez LM, Garg N, Peng Y, Nguyen DD, Watrous J, Kapono CA, Luzzatto-Knaan T, et al. 2016. Sharing and community curation of mass spectrometry data with global natural products social molecular networking. Nat Biotechnol. 34(8):828–837. doi: 10.1038/nbt.3597.
- Wang LF, Li CQ, Feng HG, Zheng XR, Cao N. 2012. Inhibition of HBsAg expression in HepG2.2.15 cells. J Shaanxi Univ Trad Chin Med. 35(06):83–85.
- Xiao KN, Su M, Hou YJ, Zhang XJ, Liu YC, Li XR, Sun DD, Liang RX, Cao L, Liu SX. 2023. Rapid characterization of chemical constituents of classic Fangda original drink by UPLC-Q-Exactive Orbitrap MS. Chinese J Exper Pharm. 29(10):1–12.
- Zhao X, Heng C E, Dong H. 2022. Combination of untargeted metabolomics approach and molecular networking analysis to identify unique natural constituents in wild Morchella sp. by UPLC-Q-TOF-MS. Food Chem. 366: 130642.doi:10.1016/j.foodchem.2021.130642