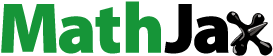
Abstract
Context
Virtually all parts of Salvadora persica L. (Salvadoraceae) are used in traditional medicine. The twigs and leaves are used for oral health, but leaves are far less investigated.
Objective
This study assesses the oral health-promoting potential of S. persica leaves with emphasis on anti-inflammatory and antiproliferative effects and provides an in depth-characterization of their metabolite profile.
Materials and methods
Hot-water and methanolic S. persica leaf extracts (1, 10, and 100 µg/mL) and their major constituents (5, 10, and 50 µM), were subjected to cellular assays on IL-8 and TNFα release in LPS-stimulated human neutrophils, NO-release in LPS/IFNγ stimulated mouse macrophages, and proliferation of HNO97 human tongue carcinoma cells. Metabolite profiling was performed by UHPLC-HRMS analysis. Major constituents were isolated and structurally elucidated.
Results and discussion
Both extracts showed pronounced anti-inflammatory activity in LPS-stimulated neutrophils. Major identified compound classes were flavonoid glycosides, the glucosinolate glucotropaeolin, phenyl- and benzylglycoside sulfates, and megastigmane glycosylsulfates, the latter ones identified for the first time in S. persica. Glucotropaeolin strongly inhibited the release of IL-8 and TNF-α (13.3 ± 2.0 and 22.7 ± 2.6% of the release of stimulated control cells at 50 µM), while some flavonoids and 3-(3′-O-sulfo-β-d-glucopyranosyloxy)-7,8-dihydro-β-ionone, a newly isolated megastigmane glycosylsulfate, were moderately active. Benzylisothiocyanate, which is likely formed from glucotropaeolin during traditional application of S. persica, showed considerable antiproliferative activity (IC50 in HNO97 cells: 10.19 ± 0.72 µM) besides strongly inhibiting IL-8 and TNFα release.
Conclusions
Glucotropaeolin and benzylisothiocyanate are likely implicated in the oral health-promoting effects of S. persica leaves. The chemistry and pharmacology of the newly identified megastigmane glycosylsulfates should be further evaluated.
Introduction
Oral diseases constitute a major global public health issue that affects almost 3.5 billion people worldwide. Three out of four affected persons live in lower- and upper-middle income countries. Next to dental caries, severe periodontal disease as well as lip and oral cavity cancer are among the five main globally occurring oral diseases (World Health Organization Citation2022). Inflammatory mediators, such as the cytokines TNF-α and IL-8 (Nussbaum and Shapira Citation2011; Hoare et al. Citation2019; Saliem et al. Citation2022), and nitric oxide (NO) (Uğar-Cankal and Ozmeric Citation2006; Hussain et al. Citation2016) are known to be implicated in the pathogenesis of periodontal disease.
Salvadora persica L. (Salvadoraceae) is an evergreen shrub or tree occurring in Northern, Southwestern, and Central Africa as well as in the dry regions of India and Western Asia. It frequently grows on sandy, dry or saline soils (Khatak et al. Citation2010; Halawany Citation2012). The plant is known under the name ‘Arak’ in Arabic and ‘Peelu’ in Urdu. Its twigs and roots are the most common traditional sources of the first historically reported oral hygiene tool, a chewing stick called miswak, meswak or siwak (Sofrata et al. Citation2008; Ahmad and Rajagopal Citation2014). Until today, miswak use remains widespread in many parts of the world, and the World Health Organization recommends its application as an effective oral hygiene measure in regions where its use is customary (WHO, Citation1987; Sofrata et al. Citation2008).
S. persica twigs, stem bark and roots have been previously subjected to thorough phytochemical analysis. This revealed the presence of flavonoids, of phenolic acids, of fatty acids, of sulfur-containing compounds such as salvadoside and the sulfur-containing alkaloid persicaline, and of nitrogen-containing compounds such as benzylurea derivatives, 3-formylindole, butanediamides and the proline-betaines stachydrine and 4-hydroxystachydrine, as major secondary compound classes (Farag et al. Citation2018; Ayoub et al. Citation2021; Farag et al. Citation2021; Binsuwaidan et al. Citation2023).
The effectiveness of miswak application for dental plaque and gingivitis reduction has been shown in clinical studies (Ramli et al. Citation2022; Scott and Marshman Citation2022; Azizan et al. Citation2023). Miswak extracts and miswak-based preparations such as toothpastes or mouthwashes have been found to exert positive effects on several aspects of oral health in vitro, in vivo, or in clinical studies. They include antimicrobial and anticariogenic effects, reduction of dental plaque due to inhibition of oral microbes, as well as elevation of plaque pH caused by the high silica content. Furthermore, oral tissue healing effects as well as positive effects on gingival health and periodontal status have been reported (Ahmad and Rajagopal Citation2014; Kumar and Sharma Citation2021; Adam et al. Citation2023; Adilah Harun et al. Citation2023; Shaalan and El-Rashidy Citation2023).
Also the leaves of S. persica are traditionally used for oral health purposes, but not as commonly as the twigs and roots: S. persica leaves are considered to strengthen the teeth (Khatak et al. Citation2010), leaf decoctions are used as a mouthwash, and leaves are masticated for treating tooth and gum problems (Sher et al. Citation2010). The phytochemical profile of the leaves is less thoroughly investigated than that of the twigs. The main compound classes known so far are flavonoids (Ali et al. Citation1997; Owis et al. Citation2021), phenylpropanoids, gallic acid (Noumi et al. Citation2017; Ibrahim et al. Citation2022), the glucosinolate glucotropaeolin (Abd El-Wahab et al. Citation1990) the indole alkaloid salvadoricine (Malik et al. Citation1987), and volatile oil (Alali and Al-Lafi Citation2003). S. persica leaf extracts reportedly have shown in vitro antimicrobial activity against different bacterial strains (Aumeeruddy et al. Citation2018) as well as anti- quorum sensing and antibiofilm activity against oral Staphylococcus strains (Noumi et al. Citation2017) and antioxidant effects (Ibrahim et al. Citation2022).
During this study, the oral health- promoting effects of S. persica leaves were assessed. We emphasized on their potential anti-inflammatory effects in stimulated neutrophils and macrophages, and on their potential antiproliferative effects on a human tongue carcinoma cell line. Furthermore, the metabolite profile of S. persica leaf extracts was studied in depth. Identified major constituents were isolated or purchased, and their oral health-promoting potential was evaluated in addition.
Materials and methods
Chemicals and reagents
Water, acetonitrile, and formic acid for UHPLC-HRMS analyses were LCMS grade, the other solvents were of analytical grade. DMSO for pharmacological assays was from Carl Roth (Karlsruhe, Germany; 4720.2). TLC plates for monitoring fractionation and purification of compounds were coated with silica gel (Kieselgel 60 F254 aluminium plates, 20 × 20 cm, coat thickness 25 mm, Merck, Darmstadt, Germany; 1.05554.0001). Silica gel for VLC and silica gel CC (particle size 0.04-0.063 mm) were from VWR International (27,623.323). Cartridges for solid phase extraction (Isolute® C18 (EC), 10 g) were from Biotage, Uppsala, Sweden (221-1000-F). Glucotropaeolin potassium salt was purchased from Phytolab, Vestenbergsgreuth, Germany (89216). Benzylisothiocyanate was from Alfa Aesar, Kandel, Germany (10752084).
Reagents for isolation and culturing of human neutrophils: Human Quantikine® Pancoll human, density 1.077 g/mL was purchased from PAN-Biotech (Aidenbach, Germany; P04-601000). Fetal bovine serum (FBS) was obtained from Gibco (Grand Island NY, USA; A5256701) and penicillin–streptomycin from PAA Laboratories (Pasching, Austria; 15140122).
Reagents for assay on inflammatory functions in LPS-stimulated human neutrophils: Phosphate buffered saline (PBS) was purchased from Biomed (Lublin, Poland; 14190-250), RPMI 1640 Medium (R7509), f-MLP (formyl-Met-Leu-Phenylalanine) (F3506), LPS (from Escherichia coli 0111:B4) (2552117), cytochalasin A (C6637), HEPES solution (H0887), luminol (A8511), L-glutamine (G7513), propidium iodide (PI) (559763) and dexamethasone (>98%; D1756) were bought from Sigma–Aldrich (Steinheim, Germany). Human TNF-α (555212) and IL-8 (555244) Quantikine ELISA Kits were purchased from BD Biosciences (Franklin Lakes, NJ, USA).
Reagents for assay on NO release in LPS/IFNγ-stimulated mouse macrophages: LPS (E. coli 055:B5, L5418) and Griess Reagent (G4410) were purchased from Sigma-Aldrich, interferon-γ (mouse recombinant E. coli) was from Roche Diagnostics (Rotkreuz, Switzerland; 11276905001), and L-NMMA was purchased from Enzo Life Sciences (Farmingdale, NY, USA; ALX-106-001-M025). Medium and supplements for cell culture were purchased from Gibco and Thermo Fisher Scientific (Waltham, MA, USA). Sodium nitrite (purity > 98.7%) was from Carl Roth (1L4P.1).
Reagents for the HNO97 cell proliferation assay: FBS, antibiotics and DMEM high glucose medium were from Gibco/Thermo Fisher Scientific, Cell proliferation kit II was from Roche Diagnostics (11465015001, and vinblastine was from Sigma-Aldrich (V1377).
General experimental procedures
Semipreparative HPLC separations were performed on a Shimadzu semipreparative HPLC system (Shimadzu Corporation, Kyoto, Japan), using a Luna® C18(2) (10 µm, 100 Å) column (250 × 10 mm, Phenomenex, Torrance, CA, USA, 00 G-4253-N0) as stationary phase.
NMR spectra for flavonoids were recorded in pyridine-d5 (Eurisotop, Saclay France; D013FE) on a UnityInova 400 MHz instrument (Varian, Palo Alto, CA, USA), and NMR spectra for compound 41 were recorded in CH3OH-d4 (Eurisotop) on an Avance III 700 MHz instrument (Bruker, Bremen, Germany). Spectra were recorded at 25 °C and (CH3)4Si was used as internal standard. J is in Hz.
The absolute configuration of the glucose moiety present in compound 41 was determined as described previously: 0.3 mg of compound 41 were hydrolyzed and the released glucose converted to the respective butylglucoside with (2 R)-2-butanol (Sigma Aldrich, 236691) as described by Stambolov et al. (Citation2023). The reaction mixture was evaporated to dryness and then dissolved in Sigma-Sil A (Sigma Aldrich, 1391) to prepare the respective trimethylsilyl ether. Reference sugars (d- and l- glucose, Sigma Aldrich, 158968 and G5500) were treated in the same manner, and the samples were analyzed by GC-MS as described in Alza et al. (Citation2014).
Plant material and extract preparation
The plant name is in accordance with http://www.worldfloraonline.org/ (accessed 08.02.2023).
Leaves were collected on October 15, 2016 from shrub trees of S. persica cultivated in the Botanical Garden of the Botany Department of Sohag University, Sohag, Egypt. The material was authenticated by Ahmed Mazen. The leaves were dried for two days at 50 °C. A reference sample of the studied dried leaves is kept at the Institute of Pharmaceutical Sciences, Pharmacognosy, University of Graz, Austria (Reference code SPL2016-1).
Small- and large- scale extracts were prepared in February 2017. For the preparation of the hot water extract (SPI), 2.02 g of dried, cut S. persica leaves were mixed with 20 mL boiling water and kept at room temperature for 10 min. After that, the extract was filtered through a folded filter, frozen and lyophilized (yield: 195 mg dried hot water extract). For the preparation of the methanolic extract (SPM), 1.01 g of powdered S. persica leaves were mixed with 10 mL pure methanol (CH3OH) and extracted for 5 min at room temperature in an ultrasonic bath. The mixture was centrifuged for 5 min at 4000 rpm, the supernatant was removed, and the extraction process was repeated twice with 10 mL of CH3OH. The combined supernatants were concentrated by rotary evaporation and dried under a stream of nitrogen (yield: 42 mg dried CH3OH extract). For compound isolation, 1.9 kg of powdered leaves were extracted by percolation with CH3OH at room temperature (5 subsequent 24 h-cycles with 3 L CH3OH each). The extract was concentrated on a rotary evaporator at 40 °C and dried under a stream of nitrogen (yield 300 g dried CH3OH extract, 15.8%).
UHPLC-HRMS metabolite profiling of SPI and SPM
UHPLC-HRMS analyses were performed on a Dionex Ultimate 3000 HPLC system hyphenated with a QExactive Hybrid Quadrupole Orbitrap MS (Thermo Scientific), using an Acquity™ Premier BEH C18 column (1.7 µm, 2.1 × 100 mm, Waters, 186003975) as stationary phase. The mobile phase consisted of H2O + 0.1% HCOOH (A) and CH3CN + 0.1% HCOOH (B). Column temperature was 40 °C, flow rate was 0.45 mL/min, and the gradient was as follows: 0–2 min, 2% B in A; 2–22.2 min, 2%–16% B in A; 22.2–31.2 min, 22%–70% B in A; 31.2–33.1 min, 70%–100% B in A; 33.1–34.6 min, 100% B in A; 34.6–35.3 min, 100%–2% B in A; 35.3–42 min, re-equilibration. The mass spectrometer was operated in the HESI negative ion mode, using the following parameters: probe heater temperature 350 °C; capillary temperature 330 °C; spray voltage 3.0 kV, sheath gas flow 50 arbitrary units; auxiliary gas flow 10 arbitrary units, S-lens RF level: 60 V; scan range: m/z 110–1650; resolution: 70,000 (FWHM at m/z 200) for full MS and 17,500 for data dependent MS/MS. Methanolic extract (SPM) and hot water extract (SPI) were dissolved at a concentration of 20 mg/mL with CH3OH and CH3OH:H2O 1:1, respectively, centrifuged (10 min, 13,000 rpm), and 3 µL were injected. Peaks were annotated based on their m/z values, calculated molecular formula and MS/MS fragmentation patterns, using authentic reference compounds, literature or databases or theoretical fragmentation for annotation. Some of the compounds were also isolated and structurally elucidated.
Compound isolation
The dried CH3OH extract (300 g) was suspended in 2 L water and subjected to subsequent liquid-liquid- extraction with n-hexane (5 × 600 mL), dichloromethane (CH2Cl2, 4 × 600 mL), ethyl acetate (EtOAc, 5 × 600 mL) and n-butanol (BuOH, 6 × 600 mL). The extracts were concentrated by rotary evaporation and dried under a stream of nitrogen. The EtOAc subfraction EA (2.65 g) was subjected to vacuum liquid chromatography (VLC) on silica gel (60 g, column diameter 4.5 cm, column height 12 cm), using a CH2Cl2− CH3OH gradient (9:1–0:100; 38 fractions, each 50 mL). Fractions VLC1_11-13 (CH2Cl2:CH3OH 8:2, 421 mg) and fractions VLC1_14-19 (CH2Cl2:CH3OH 8:2, 396 mg) were combined and each subjected to solid phase extraction (SPE) using CH3OH -H2O mixtures with increasing CH3OH proportion (20%–100% CH3OH) for elution. Fractions 11–13_SPE3 (40% CH3OH, 62 mg) and 14–19_SPE3 (40% CH3OH, 62 mg) were purified by semipreparative HPLC with H2O and acetonitrile (CH3CN) as mobile phase. The column temperature was 25 °C, flow rate 4 mL/min and the gradient was 18%–20% CH3CN in H2O from 0 to 27 min. Purification of 11–13_SPE3 with this method afforded compound 34 (RT 24.6 min, 3.85 mg) and 39 (RT 25.9 min, 5.89 mg), and purification of 14–19_SPE3 led to the isolation of 32 (RT 18.5 min, 4.1 mg), 33 (RT 23.2 min, 7.26 mg) and 36 (RT 24.1 min, 9.85 mg) (numbering of compounds is as in ).
Table 1. Annotation of peaks detectable in SPI and SPM in the HESI negative ion mode, numbered on basis of retention time.
The BuOH subextract (27.8 g) was fractionated by silica gel VLC (350 g, particle size 0.04–0.063 mm, column diameter 9.5 cm, column height 11 cm). The mobile phase consisted of a CH2Cl2–CH3OH–H2O-gradient, starting with CH2Cl2:CH3OH:H2O 95:5:0.5, and gradually increasing the CH3OH–H2O-proportion. In total, 36 fractions were collected.
The combined fraction VLC2_13–14 (1.24 g) as well as fractions VLC2_15 (0.89 g) and VLC2_16 (0.81 g) were individually further fractionated by SPE with CH3OH and H2O, both containing 0.1% trifluoroacetic acid (TFA), as eluent (5%–100% CH3OH/0.1% TFA in H2O/0.1% TFA). From each of these three SPE separations, fractions 3 and 4 (15 and 20% CH3OH/0.1% TFA in H2O/0.1% TFA), and in case of VLC2_15 also fraction 5 (30% CH3OH/0.1% TFA in H2O/0.1% TFA) were combined due to similarities in their TLC and LC-MS profiles, yielding subfraction SPE_345 (237 mg). This subfraction was further separated by column chromatography on silica gel (30 g, particle size 0.04–0.063 mm; column diameter 2 cm, column height 30 cm), using a gradient of CH2Cl2–CH3OH with increasing proportions of CH3OH (5%–50% CH3OH), and 59 fractions (500 drops/fraction) were collected with an automatic fraction collector. A combined subfraction of this separation, CC_32_33 (CH2Cl2:CH3OH 88:12, 12 mg) was purified on silica gel TLC plates, using n-BuOH:n-propanol:glacial acetic acid:H2O (38:18:2:2) as mobile phase. This afforded compound 41 (Rf 0.67, 4.66 mg). Fractions VLC2_20–21 (3.27 g) were combined, and a part of this fraction (1.17 g) was further separated by SPE, eluting with CH3OH–H2O mixtures with increasing CH3OH proportion (20%–50% CH3OH). Fraction 20–21_3 (49 mg) was purified with semipreparative HPLC, using CH3CN:H2O 14:86 (isocratic) as mobile phase. This separation afforded four more compounds: 28 (RT 31.4 min, 3.89 mg), 29 (RT 34.0 min, 5.39 mg), 30 (RT 36.2 min, 3.42 mg) and 31 (RT 39.0 min, 3.11 mg).
41, experimental data: white amorphous solid; ESIMS [M-H]− 451.1646 (calcd. or C19H31O10S−: 451.1643; 1H NMR (700 MHz, CD3OD) and 13C NMR (700 MHz, CD3OD), see ; experimental NMR and HRMS spectra, see Supplemental Material, Figures S1–S6. 28–34, 36, 39 (known compounds): 1H and 13C data, see Supplemental Material, Tables S1–S9.
Figure 1. Effects of SPM and SPI on proinflammatory functions in human neutrophils stimulated with LPS. (A) Effect on IL-8 production (% of stimulated control sample) (B) Effect on TNF-α production (% of stimulated control cells). NST: non-stimulated control cells; ST: stimulated control cells. Statistical comparison between treatments was performed by ANOVA with Dunnett’s multiple comparison post hoc test. #, p < 0.001 versus non stimulated control; ***, p < 0.001 versus stimulated control.
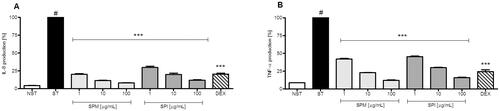
Table 2. 13C (175 MHz) and 1H (700 MHz) NMR resonance values of compound 41 in methanol-d4 and comparison with carbon data of icariside B6 recorded in pyridine-d5 (Miyase et al. Citation1988).
Cellular in-vitro assays
Assay on IL-8 and TNF-α production in LPS- stimulated human neutrophils
All tested samples were dissolved in DMSO (stock solutions with 10 mg/mL for extracts or 10 mM for pure compounds) and further diluted with RPMI 1640 medium. Prepared dilutions were directly added to the cell cultures. Dexamethasone at 50 μM was used as a positive control. The final concentration of DMSO (<0.1%) did not influence the assays.
Peripheral venous blood taken from healthy human donors (18–35 years old) was purchased from Warsaw Blood Donation Centre. Donors did not smoke or take any medication. They were clinically recognized to be healthy and routine laboratory tests showed all values to be within the normal ranges. Neutrophils were isolated by dextran sedimentation and centrifugation in a Ficoll Hypaque gradient and then re-suspended in an RPMI 1640 medium supplemented with 10% Fetal Bovine Serum (FBS), 10 mM HEPES, 2 mM l-glutamine and Penicillin/Streptomycin (10,000 U/mL). The cell suspension was transferred to 24-well plates and incubated for 24 h at 37 °C under humidified 5% CO2 air for cytokines analysis. Neutrophils were treated with extracts at final concentration of 100, 10 and 1 µg/mL or compounds at final concentrations 50 µM, in the case of active compounds also at 10 and 5 µM.
Cytotoxicity of the test samples was determined by a standard flow cytometric probe using propidium iodide (PI) staining: Neutrophils (2 × 106/mL) were cultured in 4-well plates in RPMI 1640 medium containing 10% FBS, 10 mM HEPES, 2 mM l-glutamine and Penicillin/Streptomycin (10,000 U/mL) for 24 h at 37 °C with 5% CO2 in presence of extracts or compounds. After 24 h the neutrophils were harvested and centrifuged (1500 rpm; 10 min; 4 °C), washed once with cold PBS and re-suspended in 400 µL of PBS. Five µL of PI (50 µg/mL) solution were added to the cell suspensions. After 15 min of incubation at room temperature cells were analyzed by BD FACSCalibur flow cytometer (BD Biosciences), 10,000 events were recorded per sample. Cells that displayed high permeability to PI were expressed as a percentage PI(+) (i.e., dead) cells.
For assay on IL-8 and TNF-α production, neutrophils (2 × 106) were cultured under the same conditions as for cytotoxicity assay, in the absence or presence of LPS (100 ng/mL) and test samples (added 30 min prior to LPS addition). After 24 h the neutrophils were harvested and centrifuged (774 × g; 10 min; 4 °C). The amount of released cytokines in the supernatant was measured by enzyme-linked immunosorbent assay (ELISA) following the manufacturer’s instructions (BD Biosciences). The effects on IL-8 and TNF-α production were calculated relative to the respective cytokine levels in the stimulated control samples which lacked the test compounds.
The results were expressed as mean ± SD from at least three independent experiments, each performed using cells from a different donor. The Shapiro-Wilk test was applied to determine the normality of the results. The statistical significance of differences between means was assessed by ANOVA with Dunnett’s multiple comparison post hoc test. P values below 0.05 were considered statistically significant. All analyses were performed using Statistica 13.1. GraphPad Prism (version 5.01), which was also used to plot the data.
Assay on nitric oxide (NO) production in from LPS/IFNγ stimulated mouse macrophages
Extracts and compounds were dissolved in DMSO and further diluted with PBS buffer. Diluted samples were added to the cell suspensions at final concentrations of 50 µg/mL (extracts) and 50 µM (pure compounds). The final DMSO concentration of 0.1% did not affect cell viability. RAW 264.7 mouse macrophages (Mus musculus, CVCL_0493, Elabscience, EP-CL-0190) were routinely cultivated in house (Fischer Citation2006; Blunder Citation2010) in high glucose DMEM containing 10% FBS, 100 U/mL penicillin, 100 µg/mL streptomycin, 1.25 µg/mL amphotericin B, 4 mM glutamine, 4.5 g/L glucose and 25 mM HEPES. For the assay, cells were seeded in 24-well plates (1 mL/well) at a density of 1 × 106 cells/mL and incubated for 24 h. Inflammation was induced by exchanging medium to DMEM without phenol red (supplements remain the same as in routine culture) and stimulating the cells with LPS (0.5 µg/mL) and interferon-γ (IFNγ, 5 ng/mL) as previously described (Nikzad-Langerodi et al. Citation2017), using the non-specific NOS-inhibitor L-NMMA at 100 µM as positive control. Afterwards, 100 µL of the supernatants were transferred to 96-well plates to evaluate NO production. 100 µL of Griess reagent (40 mg/mL) were added and optical density was photometrically determined at 535 nm using a Hidex Sense plate reader (HIDEX, Turku, Finland). NO-production was calculated by comparison with a NaN3 standard curve and set in relation to a control sample stimulated with LPS and containing 0.1% DMSO instead of test samples. Tests were performed in two replicates on two consecutive days (n = 4). Statistical significance between solvent treated LPS/IFNγ-stimulated control samples and test samples was determined by Kruskal-Wallis One Way Analysis on Ranks followed by Dunn’s test using SigmaPlot 13.0 (Systat Software Inc., Chicago, IL, USA).
Assay on inhibition of HNO97 carcinoma cell growth
The ability to inhibit the growth of HNO97 human tongue carcinoma cells was examined by means of an XTT assay. Samples were dissolved in DMSO and diluted with PBS to reach final concentrations of 50 µg/mL (extracts) and 50 µM (pure compounds). The final DMSO concentration of 0.5% did not affect cell viability. The cell line (Homo sapiens, CVCL_D227) was purchased from Cytion (Eppelheim, Germany; 300129) and kindly provided to us by Core Facility Alternative Biomodels and Preclinical Imaging, Medical University of Graz (Graz, Austria). Cells were cultured in DMEM high glucose medium (4.5 g/L d-glucose, Gibco, Thermo Fisher) containing 10% FBS, 100 U/mL penicillin and 100 µg/mL streptomycin and 4 mM l-glutamine. The XTT viability assay was performed in accordance with the manufacturer’s protocol. In brief, 5,000 cells/well of HNO97 carcinoma cells were seeded into 96-well plates (100 μL, flat bottom) and grown overnight before the test compounds were added. After 72 h of treatment, a freshly prepared XTT solution (5 mL of XTT solution mixed with 100 μL of electron coupling reagent) was added (50 µL/well) and analyzed after 90 min of incubation at 37 °C using a HIDEX sense plate reader. Vinblastine served as positive control (0.01 μM). Results were expressed relative to solvent control incubated with 0.5% DMSO instead of test samples. Statistical significance between solvent-treated control samples and test samples was determined with One Way Analysis on Ranks followed by Dunn’s test using SigmaPlot 13.0, and IC50 values were determined with the same software using the four-parameter logistic curve. Eight different concentrations and two different cell passages each tested in three independent wells were used.
Results
SPM and SPI display pronounced inhibitory effect on proinflammatory functions in LPS- stimulated neutrophils
Addition of LPS to human neutrophils led to highly increased production of the inflammatory mediators IL-8 and TNF-α. Both, SPM and SPI strongly and dose-dependently inhibited the production of both, IL-8 and TNF-α (), without exhibiting a negative impact on cell viability (only 12.7 ± 0.6% and 14.9 ± 1.1 of cells treated with 100 µg/mL SPI and SPM, respectively, displayed high permeability for propidium iodide). At contrast, the extracts had no effect on NO production in RAW 264.7 macrophages stimulated with LPS/IFN-γ (). In HNO97 human tongue carcinoma cells, SPI had no impact on cell viability, whilst SPM slightly, but insignificantly enhanced their proliferation ().
Figure 2. (A) Effect of SPM and SPI on NO production in RAW 254.7 macrophages stimulated with LPS/IFN-γ. ST: stimulated cells, NST: non-stimulated control cells; L-NMMA: positive control; ** p < 0.01. (B) effect of SPM and SPI on the viability of HNO97 human tongue carcinoma cells; 0.1% DMSO: control cells treated with solvent; VBN: positive control; * p < 0.05. Statistical comparison to ST (A) and to 0.1% DMSO (B) was performed by Kruskal–Wallis One way analysis of variance on ranks with Dunn’s multiple comparisons versus control group post hoc test.
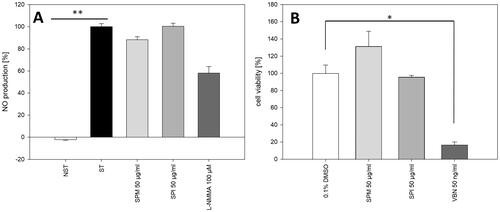
Megastigmane glycosylsulfates, flavonoids and glucosinolate are major SPM and SPI constituents
UHPLC-HRMS analysis revealed a very similar metabolite profile for SPI and SPM in the HESI negative mode (). A high number of constituents were detected in this ionization mode, while positive mode ionization was only observable for a limited number of analytes (data not shown). Therefore, negative mode data were used for compound annotation. Overall, 50 peaks could be annotated in SPI and SPM ().
Figure 3. UHPLC-HRMS analysis of SPI and SPM. HESI negative mode base peak chromatograms (m/z 110-1650) of SPI (A) and SPM (B). Peak numbers are as indicated in .
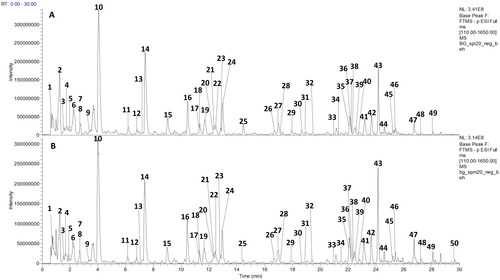
Sulfur-containing constituents
Several types of sulfur-containing constituents could be annotated in SPM and SPI: First, the major peak eluting at 4.05 min (10) was identified as the glucosinolate glucotropaeolin, which has been previously described as a constituent of S. persica (Ezmirly and Seif El-Nasr Citation1981). It was the only glucosinolate identified in SPM and SPI. Second, 32 compounds, that displayed a fragment with m/z 96.959 [HSO4]- in their MS/MS spectrum, were detected (). This fragment indicated the presence of a sulfate group in these compounds. Except for peak 4 which was tentatively annotated as methylpyrogallol sulfate, the other 31 peaks shared a further common fragment with m/z 241.002 in their MS/MS spectra. This fragment was annotated as [hexosylsulfate-H2O]−, Therefore, it was concluded that 31 of the 32 detected sulfates possessed a hexosylsulfate part.
The calculated molecular formulas of 17 sulfates indicated that they all share a molecular formula with 19 carbons. One of them, compound 41, could be isolated from the BuOH subextract of S. persica methanolic leaf extract by subsequent purification using silica gel VLC, RP-18 SPE, silica gel CC and eventually silica gel TLC. The isolation was complicated by the fact that the compound displayed no UV absorption, therefore the use of preparative HPLC with UV detection was not possible.
HRMS analysis indicated a molecular formula of C19H32O10S. From the NMR data () it could be deduced that compound 41 consisted of three parts, an exocyclic butanone moiety, a substituted cyclohexene unit, and a hexose. According to the observed HMBC correlations, the methyl groups C-11 and C-13 were attached to the same quaternary carbon and exhibited HMBC correlations to the olefinic carbon C-6 (137.5 ppm). The protons H-7 and H-8 of the exocyclic butanone showed HMBC correlation to the same carbon (137.5 ppm) defining its point of attachment. Methyl group C-13 was found to be attached to the olefinic carbon C-5, while the anomeric proton of the hexose showed an HMBC correlation to C-3 (73.2 ppm). Within the hexose, the observed coupling pattern of H-1′, H-3′ and H-4′ indicated the axial orientation of protons H-1′ to H-5′, hence the sugar was identified as glucopyranose in the β-form. The high carbon shift value (85.8 ppm) of C-3′ clearly pointed to sulfation at this position (complete proton and carbon assignments, see ).
The absolute configuration of the glucose moiety was determined to be d. Therefore, the isolated compound 41 was identified as the megastigmane glycosylsulfate 4-[2,6,6-trimethyl-4-(3-O-sulfo-β-d-glucopyranosyloxy)cyclohex-1-en-1-yl]butan-2-one, or 3-(3′-O-sulfo-β-d-glucopyranosyl-oxy)-7,8-dihydro-β-ionone, respectively (). For determination of the absolute configuration in position 3 of the megastigmane part, it would be necessary to produce the deglycosylated compound in order prepare and analyze its Mosher esters (Hoye et al. Citation2007). This was not possible with the low available amount of compound 41 and needs to be done in future studies.
Figure 4. Structures of compounds isolated and structurally identified from S. persica methanolic leaf extract (compound numbers are as in ).
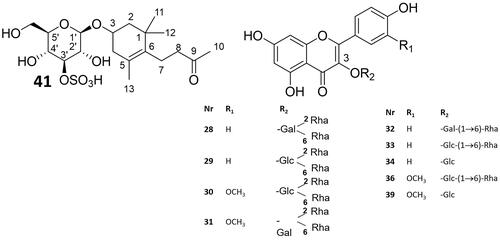
Based on comparing their MS/MS fragmentation patterns with that of isolated compound 41, further 16 peaks were tentatively annotated as megastigmane glycosylsulfates (for more details on their annotation, please refer to the text in the Supplementary Material): Peaks 35, 38, 42, 43 and 44 contained 19 carbon atoms and 10 oxygen atoms. Peaks 43 and 44 displayed the same molecular formula as compound 41 (C19H32O10S), while in peaks 38 and 42 (C19H30O10S) two hydrogen atoms less, and in peak 35 (C19H34O10S) two hydrogens more were present compared to compound 41. In peaks 17, 20, 22-27, 37 and 40, 11 instead of 10 oxygen atoms, and in peak 16, 12 oxygens were present. Three isomers were detected with a calculated molecular formula C19H30O11S (peaks 22, 23 and 27), three with C19H32O11S (peaks 20, 25, 26), four with C19H34O11S (peaks 17, 24, 37, 40), and the molecular formula of peak 16 was C19H34O11S. This is the first report on megastigmane glycosylsulfates and generally on the compound class of megastigmanes in S. persica. Due to a lack of indicative MS/MS fragments, mass spectrometry data only allowed tentative annotation, but did not enable us to conclude on the exact molecular constitution of the detected megastigmane glycosylsulfates, except for isolated compound 41.
Further hexosylsulfate peaks could be tentatively annotated as phenylglycoside sulfates (peaks 9, 11, 13) and benzylglycoside sulfates (peaks 14 and 15). The benzylglycoside sulfate salvadoside that has the same molecular formula as peaks 14 and 15, has been previously isolated from S. persica stems (Kamel et al. Citation1992), whereas phenylglycoside sulfates have hitherto not been detected in the species.
Flavonoids
Nine of the peaks (28–34, 36, 39) were annotated as flavonoid glycosides. All nine compounds were isolated from EtOAc or BuOH sub-extracts of S. persica methanolic leaf extract by means of VLC, RP-18 SPE and semipreparative HPLC, and their structures were elucidated by HRMS and 1D- and 2D- NMR spectroscopy. For all flavonoids, although they are known compounds, de novo structural assignment was performed with pyridine-d5 as solvent. The complete proton and carbon assignments are listed in the Supplementary Material (Tables S1–S9). Compounds 28, 29 and 32–34 were identified as kaempferol glycosides, while compounds 30, 31, 36, and 39 were assigned as isorhamnetin glycosides (). Compounds 34 and 39 were elucidated as 3-O-monoglycosides of kaempferol and isorhamnetin, respectively; 32 and 33 were kaempferol-, and 36 isorhamnetin- 3-O-diglycosides. 28–31 were assigned as branched triglycosides. 28–29, 30–31 and 32–33 constituted pairs of epimers, 28, 31 and 33 having a galactopyranose, and 29, 30 and 32 having a glucopyranose moiety directly attached to the flavonoid 3-O-position. The isolated flavonoids have already been isolated previously from S. persica (Ali et al. Citation1997; Owis et al. Citation2020)
Lipids
Finally, four peaks that could be detected in SPM and partially also in SPI were annotated as lipids: Three oxidized fatty acids (peaks 47, 48 and 50), as well as one phytoprostane (49).
Glucotropaeolin and its metabolite benzylisothiocyanate display most pronounced oral health-promoting effects in vitro
The constituents isolated from S. persica methanolic leaf extract and the commercially available major SPM and SPI constituent glucotropaeolin (GT) were subjected to the same pharmacological tests as the extracts. In addition, benzylisothiocyanate (BIT), a known myrosinase product of GT, was subjected to pharmacological testing.
At a concentration of 50 µM, potent anti-inflammatory activity in LPS-stimulated neutrophils was observed for GT and its myrosinase product BIT (). They dose-dependently inhibited LPS- induced TNF-α- and IL8-release in human neutrophils without impairing cell viability ( and ). BIT also moderately inhibited NO formation in LPS/IFNγ-stimulated RAW 264.7 mouse macrophages. Moreover, it was the only compound that showed a strong dose- dependent inhibition of HNO97 cancer cell growth, with an IC50 value of 10.19 ± 0.72 µM (). The other tested SPM and SPI constituents displayed only moderate or no effects at the screening concentration of 50 µM ().
Figure 5. A, B: Effects of different concentrations of GT (10) and BIT on proinflammatory functions in human neutrophils stimulated with LPS. (A) Effect on IL-8 production. (B) Effect on TNF-α production. Statistical test was same as indicated in . #, p < 0.001 vs. unstimulated control; ***, p < 0.001 vs. stimulated control; (C) Effect of different concentrations of BIT on cell viability of HNO97 tongue carcinoma cells. Dashed lines: 95% confidence band; dotted lines: 95% prediction band.
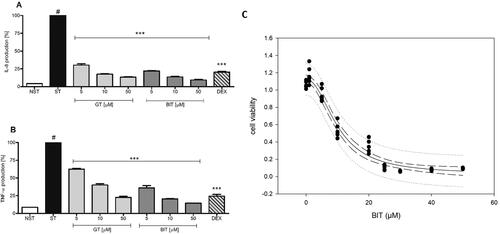
Table 3. Effects of pure compounds present in SPI and SPM on human neutrophils stimulated with LPS, on murine RAW 264.7 macrophages stimulated with LPS/IFNγ and on the viability of HNO97 cells.
Discussion
In our study, the oral health-promoting effects of S. persica leaves were assessed in different cellular models of inflammation and cancer, and their chemical composition was investigated by UHPLC-HRMS metabolite profiling. The investigated preparations were a hot water extract (SPI) and a methanolic extract (SPM) of the dried leaves. These preparations were used in order to extract both, the polar compounds which can be expected in traditionally used hot water preparations, and more non-polar compounds that can be expected to be released when leaves are masticated for the traditional treatment of tooth and gum problems (Sher et al. Citation2010).
By means of UHPLC-HRMS metabolite profiling, in addition to flavonoid glycosides, sulfur-containing constituents, including the glucosinolate GT, phenyl- and benzylglycoside sulfates, as well as megastigmane glycosylsulfates, were identified as predominantly occurring compound classes. Our study is the first report on the presence of megastigmanes in S. persica, and UHPLC-HRMS metabolite profiling indicated that the detected megastigmane glycosylsulfates occur in the studied leaf extracts at a high structural variety.
The megastigmane glycoside skeleton of the isolated and newly identified 3-(3′-O-sulfo-β-d-glucopyranosyloxy)-7,8-dihydro-β-ionone (41) is known. The 3 R-enantiomer, icariside B6, has been isolated and structurally identified from Epimedium grandiflorum MORR. var. thunbergianum (MIQ.) NAKAI. III for the first time (Miyase et al. Citation1988). From the 3S-enantiomer, only the aglycone is described in the literature. It has been isolated from Chamaecyparis formosensis Matsumara (Cupressaceae) for the first time (Lin Citation1999). No sulfated derivatives of these compounds have been described to date.
Megastigmanes are C13-norterpenoids structurally related to sesquiterpenes that are generated by oxidative cleavage of carotenoids (González-Delgado et al. Citation2017; Kornpointner et al. Citation2022). Until the early 2010s, around 150 megastigmanes have been reported (Breitmaier Citation2012), and although no more recent systematic review on this compound class is available in the literature, it can be assumed that the number has risen considerably since then (Kornpointner et al. Citation2022). Compared to that, reports on the detection of sulfated megastigmane glycosides in plants are very scarce to date. A megastigmane glycosylsulfate with the same molecular formula as in peaks 38 and 42, namely tetraenoside C, has been previously isolated from Tetraena mongolica Maxim. (Zygophyllaceae) (Wu et al. Citation2019), and a megastigmane glycosylsulfate with a molecular composition as in peak 35, anisoposide A, has been previously isolated from Mallotus anisopodus (Gagnep.) (Euphorbiaceae) (van Minh et al. Citation2009). Furthermore, corchoionoside C 6′-O-sulfate (Ozgen et al. Citation2010; Macahig et al. Citation2011) and tetraenoside A (Wu et al. Citation2019) with molecular formula C19H30O11S, like in peaks 22, 23 and 37, as well as tetraenoside B (molecular formula C19H32O11S, like in peaks 20, 25 and 26) (Wu et al. Citation2019) have been previously isolated from herbal materials. Due to a lack of indicative MS/MS fragments, we were unable to conclude whether the megastigmane glycosylsulfates detected in SPM and SPI correspond to the ones already described in the literature based on UHPLC-HRMS metabolite profiling. Their isolation and full structural elucidation are envisaged in a follow-up project.
(Kornpointner et al. Citation2022) recently isolated blumenol C sulfate, a non-glycosylated compound with sulfate directly attached to the megastigmane moiety, from the leaves of a Palicourea luxurians (Rusby) Borhidi specimen collected in a sulfate-rich environment. They suggested that the formation of this compound may be a defense mechanism against SO42− - induced salt stress in the plant tissue of this species, however they did not assess whether the compound was also formed in specimens grown under lower SO42−- exposure. As far as S. persica is concerned, (Sawidis Citation2013) has demonstrated sulfur accumulation in the parenchyma cells of its stems, however it is not known whether sulfur is also accumulated in its leaves. Therefore, broader investigations on the occurrence of the newly detected megastigmane glycosylsulfates in different S. persica accessions grown on different soils, as well of different parts of the plant will be conducted in the future.
Pharmacological testing revealed that both S. persica extracts as well as their major constituent GT showed pronounced anti-inflammatory activities in LPS-stimulated human neutrophils. The inhibitory activity of the S. persica leaf extracts and GT on IL-8 and TNFα secretion is described herein for the first time.
BIT is known to be formed by the degradation of GT catalyzed by the enzyme myrosinase. In intact plant cells, this enzyme is kept separately from GT, but as soon as the cells are disrupted, the enzymatic cleavage takes place and BIT is formed (Nastruzzi et al. Citation2000; Kleinwächter et al. Citation2008). Although BIT was not detected in SPI and SPM in this study, it can be expected to be formed in certain traditional applications, e.g., when fresh leaves are masticated to relieve tooth and gum problems (Sher et al. Citation2010).
At contrast to the S. persica extracts and GT, BIT not only showed pronounced anti-inflammatory activities in LPS-stimulated human neutrophils, but also moderately inhibited NO release in LPS/IFNγ-stimulated RAW 264.7 macrophages, and it potently inhibited the proliferation of HNO97 tongue carcinoma cells. Similar observations have been made in a study assessing the anti-inflammatory and anticancer effects of Lepidium meyenii: in contrast to the intact glucosinolates, their myrosinase product BIT inhibited NO formation in LPS-stimulated mouse macrophages and the growth of five different tumor cell lines (Yan et al. Citation2022). Furthermore, numerous effects related to anti-inflammatory activity have been previously reported for BIT, among them inhibition of LPS-induced secretion of TNFα and several pro-inflammatory interleukins, but not of IL-8 (Ohta et al. Citation2004; Lee et al. Citation2009).
The major flavonoids present in SPM and SPI only showed moderate effects on LPS-induced TNFα secretion. Among them, isorhamnetin-3-O-β-d-glucopyranoside displayed the highest activity. The compound has previously shown moderate anti-inflammatory effects, e.g., reduction of TNF-α levels in human peripheral blood mononuclear cells stimulated with phytohemagglutinin (Abdallah and Esmat Citation2017) and in LPS/IFN-γ-stimulated mouse macrophages (Rao et al. Citation2008).
The newly isolated 3-(3′-O-sulfo-β-D-glucopyranosyloxy)-7,8-dihydro-β-ionone (41) moderately inhibited TNFα- and IL-8 secretion in LPS-stimulated neutrophils. While some unsubstituted megastigmanes and megastigmane glycosides have shown pharmacological effects, such as in vitro anti-inflammatory (Pan et al. Citation2019; Huang et al. Citation2023) and anticancer (Panza et al. Citation2011; Shu et al. Citation2013) activities, the pharmacological potential of megastigmane glucosylsulfates is hitherto more or less uninvestigated.
The onset and progression of periodontitis is attributed to the release of virulence factors by key bacteria in the dental biofilm that stimulate the inflammatory immune response in the host tissue, leading to increased interleukin production. IL-8 particularly acts as chemotactic agent, recruiting inflammatory cells such as neutrophils and macrophages to the periodontal lesion (Saliem et al. Citation2022) Arrival of these cells at an inflammation site has been shown to initiate the secretion of inflammatory chemokines, matrix metalloproteinases and cytokines, including TNF-α in circulating and wound neutrophils (Theilgaard-Mönch et al. Citation2004; Nussbaum and Shapira Citation2011). As periodontal disease proceeds, the growing populations of immune cells such as lymphocytes and T helper cells contribute to increasing levels of cytokines, such as TNF-α, IL-1, IL-4, IL-10, interferon-γ, and TGF-β (Graves Citation2008; Hoare et al. Citation2019; Saliem et al. Citation2022).
Despite being part of the nonspecific natural defense mechanisms of the oral cavity against pathogenic bacteria, NO, when produced at excessive levels, can also contribute to tissue damage in periodontal tissues and is thought to be involved in the pathogenesis of periodontal diseases (Uğar-Cankal and Ozmeric Citation2006). Endotoxins from Gram-negative bacteria occurring in periodontal disease and proinflammatory cytokines trigger resident as well as migrant cells to produce NO. The enzyme inducible NO synthase (iNOS) has been found to be upregulated during periodontitis and inflammation associated with peri-implantitis, leading to elevated NO levels (Hussain et al. Citation2016).
Conclusions
By means of UHPLC-HRMS metabolite profiling and subsequent isolation of major constituents, flavonoid glycosides, the glucosinolate GT, phenyl- and benzyl-glycoside sulfates and megastigmane glycosylsulfates were identified as major compound classes occurring in S. persica leaves. S. persica hot water and methanolic leaf extracts as well as their major constituent GT and the GT degradation product BIT were found to strongly inhibit the release of TNF-α and IL-8, two proinflammatory mediators implicated in periodontal disease, in LPS-stimulated neutrophils. BIT, which is likely formed by myrosinase-catalyzed degradation of GT during traditional application of S. persica leaves, additionally inhibited NO formation in LPS/IFNγ-stimulated RAW 264.7 macrophages as well as the proliferation of HNO97 tongue carcinoma cells, thereby further contributing to the oral health- promoting effects of S. persica leaves. Megastigmane glycosylsulfates were identified for the first time in S. persica and deserve deeper chemical and pharmacological investigation in the future.
Authors contributions
Conceptualization, EMPW, AMA, AKK,; methodology, EMPW, OK, AKK, NK; software; N/A; validation: EMPW, OK, AKK, TP; formal analysis, SK, BG, OK, NK, AMA, SK, CD, PR, TP, AKK; investigation, SK, BG, OK, AMA, PR, NK, CD, TP, EMPW; resources, AMA, RB, AKK; data curation, OK, EMPW; writing – original draft, EMPW, OK, BG; writing – review & editing, SK, BG, OK, AMA, PR, NK, CD, TP, EMPW, RB, AKK; visualization, OK, BG, AKK, NK, PR, TP, EMPW; supervision, EMPW, AMA, AKK, RB; project administration, EMPW, AMA, AKK; funding acquisition: RB, AKK, AMA.
supplemental material salvadora_R4.docx
Download MS Word (21.3 MB)Acknowledgements
We thank Ass.-Prof. Dr. Beate Rinner, Core Facility Alternative Biomodels and Preclinical Imaging, Medical University of Graz (Graz, Austria) for providing the HNO97 cell line. Robert Weis (University of Graz) is acknowledged for his support with the nomenclature of the new compound. NAWI Graz is thanked for supporting Central Lab Environmental, Plant & Microbial Metabolomics.
Disclosure statement
The authors declare no conflicts of interest.
Additional information
Funding
References
- Abd El-Wahab SM, Selim MA, El-Fiki NM, El-Falaha BMA. 1990. Investigation of glucosinolates of Salvadora persica L. Bull Fac Pharm. 28:63–66.
- Abdallah HM, Esmat A. 2017. Antioxidant and anti-inflammatory activities of the major phenolics from Zygophyllum simplex L. J Ethnopharmacol. 205:51–56. doi: 10.1016/j.jep.2017.04.022.
- Adam FA, Mohd N, Rani H, Mohd Yusof MYP, Baharin B. 2023. A systematic review and meta-analysis on the comparative effectiveness of Salvadora persica – extract mouthwash with chlorhexidine gluconate in periodontal health. J Ethnopharmacol. 302(Pt A):115863. doi: 10.1016/j.jep.2022.115863.
- Adilah Harun N, Ramli N, Rahman Kazim Azli A, Hassan H, Bariah Chi Adam K, Haikal Muhamad Halil M. 2023. The effect of Salvadora persica ethanolic extract on oral tissue healing in rats: an in vivo study. Saudi Dent J. 35(6):663–667. doi: 10.1016/j.sdentj.2023.05.019.
- Ahmad H, Rajagopal K. 2014. Salvadora persica L. (Meswak) in dental hygiene. Saudi J Dental Res. 5(2):130–134. doi: 10.1016/j.sjdr.2014.02.002.
- Alali F, Al-Lafi T. 2003. GC-MS analysis and bioactivity testing of the volatile oil from the leaves of the toothbrush tree Salvadora persica L. Nat Prod Res. 17(3):189–194. doi: 10.1080/1057563021000040790.
- Ali AA, Assaf MH, El-Shanawany MA, Kamel MS. 1997. Flavonoid glycosides from the leaves of Salvadora persica L. Bull Pharm Sci, Assiut Univ. 20(2):181–186. doi: 10.21608/bfsa.1997.68759.
- Alza NP, Pferschy-Wenzig E-M, Ortmann S, Kretschmer N, Kunert O, Rechberger GN, Bauer R, Murray AP. 2014. Inhibition of NO production by Grindelia argentina and isolation of three new cytotoxic saponins. Chem Biodivers. 11(2):311–322. doi: 10.1002/cbdv.201300193.
- Aumeeruddy MZ, Zengin G, Mahomoodally MF. 2018. A review of the traditional and modern uses of Salvadora persica L. (Miswak): toothbrush tree of Prophet Muhammad. J Ethnopharmacol. 213:409–444. doi: 10.1016/j.jep.2017.11.030.
- Ayoub N, Badr N, Al-Ghamdi SS, Alsanosi S, Alzahrani AR, Abdel-Naim AB, Nematallah KA, Swilam N. 2021. HPLC/MSn Profiling and healing activity of a muco-adhesive formula of Salvadora persica against acetic acid-induced oral ulcer in rats. Nutrients. 14(1):28. doi: 10.3390/nu14010028.
- Azizan NF, Mohd N, Nik Azis NM, Baharin B. 2023. Effectiveness of Salvadora persica toothbrush and Salvadora persica chewing stick in plaque and gingivitis control: a randomized control trial. BMC Complement Med Ther. 23(1):456. doi: 10.1186/s12906-023-04295-z.
- Binsuwaidan R, Negm WA, Elekhnawy E, Attallah NGM, Ahmed E, Magdeldin S, Moglad E, Mostafa SA, El-Sherbeni SA. 2023. In vitro antiviral effect and potential neuroprotection of Salvadora persica L. stem bark extract against lipopolysaccharides-induced neuroinflammation in mice: LC-ESI-MS/MS analysis of the methanol extract. Pharmaceuticals. 16(3):398. doi: 10.3390/ph16030398.
- Blaženović I, Kind T, Ji J, Fiehn O. 2018. Software tools and approaches for compound identification of LC-MS/MS data in metabolomics. Metabolites. 8(2):31. doi: 10.3390/metabo8020031.
- Blunder M. 2010. Phytochemische und pharmakologische Untersuchungen von Arzneipflanzen mit antiinflammatorischer Wirkung [PhD thesis]. Graz, Austria: University of Graz.
- Breitmaier E. 2012. Terpene: aromen, Düfte, Pharmaka, Pheromone. 2nd ed. Newark: John Wiley & Sons, Incorporated.
- Collado-González J, Medina S, Durand T, Guy A, Galano J-M, Torrecillas A, Ferreres F, Gil-Izquierdo A. 2015. New UHPLC-QqQ-MS/MS method for quantitative and qualitative determination of free phytoprostanes in foodstuffs of commercial olive and sunflower oils. Food Chem. 178:212–220. doi: 10.1016/j.foodchem.2015.01.097.
- Ezmirly ST, Seif El-Nasr MM. 1981. Isolation of glucotropaeolin from Salvadora persica L. J Chem Soc Pakistan. 3:9–12.
- Farag M, Abdel-Mageed WM, Basudan O, El-Gamal A. 2018. Persicaline, a new antioxidant sulphur-containing imidazoline alkaloid from Salvadora persica roots. Molecules. 23(2):483. doi: 10.3390/molecules23020483.
- Farag MA, Shakour ZT, Lübken T, Frolov A, Wessjohann LA, Mahrous E. 2021. Unraveling the metabolome composition and its implication for Salvadora persica L. use as dental brush via a multiplex approach of NMR and LC-MS metabolomics. J Pharm Biomed Anal. 193:113727. doi: 10.1016/j.jpba.2020.113727.
- Fischer MC. 2006. Phytochemische und pharmakologische Untersuchungen chinesischer Arzneipflanzen [PhD thesis]. Graz, Austria: University of Graz.
- Geng P, Harnly JM, Chen P. 2015. Differentiation of whole grain from refined wheat (T. aestivum) flour using lipid profile of wheat bran, germ, and endosperm with UHPLC-HRAM mass spectrometry. J Agric Food Chem. 63(27):6189–6211. doi: 10.1021/acs.jafc.5b01599.
- González-Delgado JA, Romero MA, Pischel U, Arteaga JF. 2017. Universal access to megastigmanes through controlled cyclisation towards highly substituted cyclohexenes. Org Biomol Chem. 15(2):408–415. doi: 10.1039/c6ob02587k.
- Graves D. 2008. Cytokines that promote periodontal tissue destruction. J Periodontol. 79(8 Suppl):1585–1591. doi: 10.1902/jop.2008.080183.
- Halawany HS. 2012. A review on miswak (Salvadora persica) and its effect on various aspects of oral health. Saudi Dent J. 24(2):63–69. doi: 10.1016/j.sdentj.2011.12.004.
- Hoare A, Soto C, Rojas-Celis V, Bravo D. 2019. Chronic inflammation as a link between periodontitis and carcinogenesis. Mediators Inflamm. 2019:1029857–1029814. doi: 10.1155/2019/1029857.
- Hoye TR, Jeffrey CS, Shao F. 2007. Mosher ester analysis for the determination of absolute configuration of stereogenic (chiral) carbinol carbons. Nat Protoc. 2(10):2451–2458. doi: 10.1038/nprot.2007.354.
- Huang Y, Pan L, Chang Y, Liang X, Hou P, Ren C, Xu W, Yang R, Li J, Liu B. 2023. Megastigmane glycosides from Streblus ilicifolius (S.Vidal) Corner and their anti-inflammatory activity. Phytochemistry. 208:113606. doi: 10.1016/j.phytochem.2023.113606.
- Hussain QA, McKay IJ, Gonzales-Marin C, Allaker RP. 2016. Detection of adrenomedullin and nitric oxide in different forms of periodontal disease. J Periodontal Res. 51(1):16–25. doi: 10.1111/jre.12273.
- Ibrahim II, Moussa AA, Chen Z, Zhang J, Cao W-G, Yu C. 2022. Bioactive phenolic components and antioxidant activities of water-based extracts and flavonoid-rich fractions from Salvadora persica L. leaves. Nat Prod Res. 36(10):2591–2594. doi: 10.1080/14786419.2021.1908281.
- Kamel MS, Ohtani K, Assaf MH, Kasai R, El-Shanawani MA, Yamasaki K, Ali AA, Tanaka O. 1992. Lignan glycosides from stems of Salvadora persica. Phytochemistry. 31(7):2469–2471. doi: 10.1016/0031-9422(92)83301-E.
- Khatak M, Khatak S, Siddqui AA, Vasudeva N, Aggarwal A, Aggarwal P. 2010. Salvadora persica. Pharmacogn Rev. 4(8):209–214. doi: 10.4103/0973-7847.70920.
- Kleinwächter M, Schnug E, Selmar D. 2008. The glucosinolate-myrosinase system in nasturtium (Tropaeolum majus L.): variability of biochemical parameters and screening for clones feasible for pharmaceutical utilization. J Agric Food Chem. 56(23):11165–11170. doi: 10.1021/jf802053n.
- Kornpointner C, Hochenegger NJ, Shi B-B, Berger A, Theiner J, Brecker L, Schinnerl J. 2022. Phytochemistry meets geochemistry – blumenol C sulfate: a new megastigmane sulfate from Palicourea luxurians (Rubiaceae: palicoureeae). Molecules. 27(21):7284. doi: 10.3390/molecules27217284.
- Kumar D, Sharma PK. 2021. Traditional use, phytochemicals and pharmacological activity of Salvadora persica: a review. CNF. 17(3):302–309. doi: 10.2174/1573401316999200807205224.
- Lee YM, Seon MR, Cho HJ, Kim J-S, Park JHY. 2009. Benzyl isothiocyanate exhibits anti-inflammatory effects in murine macrophages and in mouse skin. J Mol Med (Berl). 87(12):1251–1261. doi: 10.1007/s00109-009-0532-6.
- Lin T. 1999. Terpenes and lignans from leaves of Chamaecyparis formosensis. Phytochemistry. 51(6):793–801. doi: 10.1016/S0031-9422(99)00074-6.
- Macahig RAS, Matsunami K, Otsuka H. 2011. Chemical studies on an endemic Philippine plant: sulfated glucoside and seco-A-ring triterpenoids from Dillenia philippinensis. Chem Pharm Bull (Tokyo). 59(3):397–401. doi: 10.1248/cpb.59.397.
- Maldini M, Montoro P, Piacente S, Pizza C. 2009. Phenolic compounds from Bursera simaruba Sarg. bark: phytochemical investigation and quantitative analysis by tandem mass spectrometry. Phytochemistry. 70(5):641–649. doi: 10.1016/j.phytochem.2009.02.009.
- Malik S, Ahmad SS, Haider SI, Muzaffar A. 1987. Salvadoricine - a new indole alkaloid from the leaves of Salvadora persica. Tetrahedron Lett. 28(2):163–164. doi: 10.1016/S0040-4039(00)95675-2.
- Miyase T, Ueno A, Takizawa N, Kobayashi H, Oguchi H. 1988. Studies on the glycosides of Epimedium grandiflorum MORR. var. thunbergianum (Miq.) NAKAI. III. Chem Pharm Bull. 36(7):2475–2484. doi: 10.1248/cpb.36.2475.
- Nastruzzi C, Cortesi R, Esposito E, Menegatti E, Leoni O, Iori R, Palmieri S. 2000. In vitro antiproliferative activity of isothiocyanates and nitriles generated by myrosinase-mediated hydrolysis of glucosinolates from seeds of cruciferous vegetables. J Agric Food Chem. 48(8):3572–3575. doi: 10.1021/jf000191p.
- Nikzad-Langerodi R, Ortmann S, Pferschy-Wenzig EM, Bochkov V, Zhao YM, Miao JH, Saukel J, Ladurner A, Heiss EH, Dirsch VM, et al. 2017. Assessment of anti-inflammatory properties of extracts from Honeysuckle (Lonicera sp. L., Caprifoliaceae) by ATR-FTIR spectroscopy. Talanta. 175:264–272. doi: 10.1016/j.talanta.2017.07.045.
- Noumi E, Snoussi M, Merghni A, Nazzaro F, Quindós G, Akdamar G, Mastouri M, Al-Sieni A, Ceylan O. 2017. Phytochemical composition, anti-biofilm and anti-quorum sensing potential of fruit, stem and leaves of Salvadora persica L. methanolic extracts. Microb Pathog. 109:169–176. doi: 10.1016/j.micpath.2017.05.036.
- Nussbaum G, Shapira L. 2011. How has neutrophil research improved our understanding of periodontal pathogenesis? J Clin Periodontol. 38(Suppl 11):49–59. doi: 10.1111/j.1600-051X.2010.01678.x.
- Ohta Y, Yamaki K, Osawa T, Kawakishi S. 2004. Effects of isothiocyanates on tumor necrosis factor-alpha production by J774A.1 (BALB/c macrophage) cells. Biosci Biotechnol Biochem. 68(6):1375–1378. doi: 10.1271/bbb.68.1375.
- Owis AI, El-Hawary MS, El Amir D, Aly OM, Abdelmohsen UR, Kamel MS. 2020. Molecular docking reveals the potential of Salvadora persica flavonoids to inhibit COVID-19 virus main protease. RSC Adv. 10(33):19570–19575. doi: 10.1039/d0ra03582c.
- Owis AI, El-Hawary MS, El Amir D, Refaat H, Alaaeldin E, Aly OM, Elrehany MA, Kamel MS. 2021. Flavonoids of Salvadora persica L. (meswak) and its liposomal formulation as a potential inhibitor of SARS-CoV-2. RSC Adv. 11(22):13537–13544. doi: 10.1039/d1ra00142f.
- Ozgen U, Sevindik H, Kazaz C, Yigit D, Kandemir A, Secen H, Calis I. 2010. A new sulfated alpha-ionone glycoside from Sonchus erzincanicus Matthews. Molecules. 15(4):2593–2599. doi: 10.3390/molecules15042593.
- Pan S-P, Pirker T, Kunert O, Kretschmer N, Hummelbrunner S, Latkolik SL, Rappai J, Dirsch VM, Bochkov V, Bauer R. 2019. C13 Megastigmane derivatives from Epipremnum pinnatum: β-damascenone inhibits the expression of pro-inflammatory cytokines and leukocyte adhesion molecules as well as NF-κB signaling. Front Pharmacol. 10:1351. doi: 10.3389/fphar.2019.01351.
- Panza E, Tersigni M, Iorizzi M, Zollo F, S de M, Festa C, Napolitano M, Castello G, Ialenti A, Ianaro A. 2011. Lauroside B, a megastigmane glycoside from Laurus nobilis (bay laurel) leaves, induces apoptosis in human melanoma cell lines by inhibiting NF-κB activation. J Nat Prod. 74(2):228–233. doi: 10.1021/np100688g.
- Pimpão RC, Dew T, Figueira ME, McDougall GJ, Stewart D, Ferreira RB, Santos CN, Williamson G. 2014. Urinary metabolite profiling identifies novel colonic metabolites and conjugates of phenolics in healthy volunteers. Mol Nutr Food Res. 58(7):1414–1425. doi: 10.1002/mnfr.201300822.
- Ramli H, Nor Aripin KN, Mohd Said S, Mohamad Hanafiah R, Mohd Dom TN. 2022. The effectiveness of miswak (Salvadora persica L. and Azadirachta indica A. Juss.) practices in reducing plaque and gingivitis among adults: a systematic review and meta-analysis. J Ethnopharmacol. 298:115598. doi: 10.1016/j.jep.2022.115598.
- Rao YK, Fang S-H, Tzeng Y-M. 2008. Antiinflammatory activities of flavonoids and a triterpene caffeate isolated from Bauhinia variegata. Phytother Res. 22(7):957–962. doi: 10.1002/ptr.2448.
- Saliem SS, Bede SY, Cooper PR, Abdulkareem AA, Milward MR, Abdullah BH. 2022. Pathogenesis of periodontitis – A potential role for epithelial-mesenchymal transition. Jpn Dent Sci Rev. 58:268–278. doi: 10.1016/j.jdsr.2022.09.001.
- Sawidis T. 2013. Anatomy and ultrastructure of Salvadora persica stem: adaptive to arid conditions and beneficial for practical use. Acta Biol Cracoviensia Series Botanica. 55(2):7–17.
- Scott J, Marshman Z. 2022. Does the use of miswak reduce plaque and gingivitis among adults? Evid Based Dent. 23(4):152–153. doi: 10.1038/s41432-022-0833-y.
- Shaalan O, El-Rashidy A. 2023. Antibacterial effect of miswak herbal toothpaste compared to fluoride toothpaste in high caries risk patients: randomized clinical Trial. J Clin Exp Dent. 15(7):e526–e534. doi: 10.4317/jced.60332.
- Sher H, Alyemeni M, Masrahi Y, Shah AH. 2010. Ethnomedicinal and ethnoecological evaluation of Salvadora persica L.: A threatened medicinal plant in Arabian Peninsula. J Med Plant Res. 4:1209–1215.
- Shu P, Wei X, Xue Y, Li W, Zhang J, Xiang M, Zhang M, Luo Z, Li Y, Yao G, et al. 2013. Wilsonols A-L, megastigmane sesquiterpenoids from the leaves of Cinnamomum wilsonii. J Nat Prod. 76(7):1303–1312. doi: 10.1021/np4002493.
- Sofrata AH, Claesson RLK, Lingström PK, Gustafsson AK. 2008. Strong antibacterial effect of miswak against oral microorganisms associated with periodontitis and caries. J Periodontol. 79(8):1474–1479. doi: 10.1902/jop.2008.070506.
- Stambolov I, Shkondrov A, Kunert O, Bucar F, Kondeva-Burdina M, Krasteva I. 2023. Cycloartane saponins from Astragalus glycyphyllos and their in vitro neuroprotective, antioxidant, and hMAO-B-inhibiting effects. Metabolites. 13(7):857. doi: 10.3390/metabo13070857.
- Sumner LW, Amberg A, Barrett D, Beale MH, Beger R, Daykin CA, Fan TW-M, Fiehn O, Goodacre R, Griffin JL, et al. 2007. Proposed minimum reporting standards for chemical analysis chemical analysis working group (CAWG) metabolomics standards initiative (MSI). Metabolomics. 3(3):211–221. doi: 10.1007/s11306-007-0082-2.
- Theilgaard-Mönch K, Knudsen S, Follin P, Borregaard N. 2004. The transcriptional activation program of human neutrophils in skin lesions supports their important role in wound healing. J Immunol. 172(12):7684–7693. doi: 10.4049/jimmunol.172.12.7684.
- Uğar-Cankal D, Ozmeric N. 2006. A multifaceted molecule, nitric oxide in oral and periodontal diseases. Clin Chim Acta. 366(1-2):90–100. doi: 10.1016/j.cca.2005.10.018.
- van Minh C, Thanh NTK, Quang TH, Cuong NX, Thin NN, Nam NH, Heyden YV, Quetin-Leclercq J, van Kiema P. 2009. Two new megastigmane sulphonoglucosides from Mallotus anisopodus. Nat Prod Commun. 4(7):889–892.
- World Health Organization. 1987. Prevention of oral diseases. Geneva: World Health Organization.
- World Health Organization. 2022. Global oral health status report: towards universal health coverage for oral health by 2030. Geneva: World Health Organization.
- Wu Z-G, Wei W, Xu H-Y, Zheng L-L, Ma C-M, Wang Y-C. 2019. Constituents from the leaves of Tetraena mongolica and their protective activity in HEK 293t cells damaged by CdCl2. J Nat Prod. 82(10):2707–2712. doi: 10.1021/acs.jnatprod.9b00212.
- Xu L, Li X, Wang X, Song A, Han F. 2020. A feasible strategy based on isotopic fine structures to enhance the reliability of metabolite identification by Fourier transform ion cyclotron resonance mass spectrometry. Rapid Commun Mass Spectrom. 34:e8560.
- Yan S, Wei J, Chen R. 2022. Evaluation of the biological activity of glucosinolates and their enzymolysis products obtained from Lepidium meyenii Walp. (Maca). Int J Mol Sci. 23(23):14756. doi: 10.3390/ijms232314756.