Abstract
Context
The botanical species Bauhinia guianensis Aublet (Leguminosae-Cercidoideae) is traditionally used in the Amazon for medicinal purposes.
Objective
The acute toxicity of the hydroethanolic extracts from B. guianensis leaves and stems (HELBg and HESBg) was evaluated in zebrafish (Danio rerio), with emphasis on the embryonic developmental stage and adult alterations.
Materials and methods
Extracts were analyzed on LC-DAD-MS/MS. Zebrafish eggs were inoculated individually with concentrations of HELBg and HESBg (0.25, 0.5, 0.75, 1.0, and 1.5 µg/mL), observed for 96 h. Adult zebrafish were treated with a single oral dose (100, 200, 500, 1000, and 2000 mg/kg) of HELBg and HESBg, observed for 48 h.
Results
HELBg and HESBg analysis detected 55 compounds. Both extracts exhibited toxicity, including embryo coagulation at higher doses of HELBg and absence of heartbeats in embryos at all doses of HESBg. Behavioral variations were observed; tissue alterations in adult zebrafish were found at the highest doses, primarily in the liver, intestine, and kidneys because of HELBg and HESBg effects. The LD50 of HESBg was 1717 mg/kg, while HELBg exceeded the limit dose of 2000 mg/kg.
Conclusions
The study on acute toxicity of B. guianensis extracts exhibits significant toxic potential, emphasizing effects on embryonic and adult zebrafish. The results suggest relative safety of the species preparations, encouraging further clinical trials on potential biological activities.
Introduction
Current knowledge about the efficacy of plant use by traditional populations is of great historical importance (Souza et al. Citation2019). Thus, the secular use of plant species in traditional environments focuses on the need to prove whether these natural ingredients are effective and safe (Hosseinzadeh et al. Citation2015). In pharmacodynamic terms, ethnocognition and its peculiarities can identify therapeutic or toxic properties of many plant species (Rates Citation2001; Souza Citation2012). Therefore, knowing the toxicity of biologically active agents can initiate the formulation of valuable and promising hypotheses for the development of new drugs (Calixto Citation2003; Agustina et al. Citation2020).
Bauhinia guianenis Aublet (Leguminosae-Cercidoideae) is a plant species popularly known as ‘tortoise ladder’ (Quintans-Júnior et al. Citation2002; Pinheiro et al. Citation2013). It is a spontaneous plant native to the Amazon Biome (Wiersema Citation2019; Koga et al. Citation2021). Among its ethnopharmacological applications, it appears that the leaves and stems of B. guianensis have been used to treat parasitic infections such as amoeba (Carvalho et al. Citation1999; Viana et al. Citation1999), malaria (Muñoz et al. Citation2000), and leishmaniasis, as well as to treat asthma, inflammatory processes, and pain, in addition to helping to control menstrual flow (Quintans-Júnior et al. Citation2002; Pinheiro et al. Citation2013).
Zebrafish (Danio rerio) has emerged as an important animal model in toxicity testing and holds significant relevance in the field of pharmacology. Their high genome similarity to humans, rapid reproductive cycle, and transparent embryos makes them ideal for studying the effects of various chemicals and drugs (Teame et al. Citation2019). Zebrafish embryos can be easily manipulated and observed, allowing to monitor the development of organs, neurobehavioral responses, and cardiovascular functions in real-time. Their small size, cost-effectiveness, and ability to yield rapid results facilitate large-scale testing and screening of potential therapeutic agents (Santos et al. Citation2018; Souza et al. Citation2019).
Additionally, zebrafish models have shown remarkable predictive value for human toxicity, enabling to gain valuable insights into drug metabolism, safety, and efficacy (Cassar et al. Citation2020). The versatility and reliability of zebrafish as an animal model make it an invaluable tool in advancing our understanding of toxicology and driving pharmacological research toward safer and more effective treatments (Teixidó et al. Citation2013). Therefore, in this study, the acute toxicity of the hydroethanolic extracts from B. guianensis leaves and stems (HELBg and HESBg) was evaluated in zebrafish, with emphasis on the embryonic developmental stage and adult alterations.
Materials and methods
Collection and identification of botanical material
The botanical material, consisting of leaves and stem of B. guianensis, was collected in the city of Macapá, Amapá, Brazil, under the geographic coordinates 0° 00′37″ S and 51,06′59″ W, in February 2020.
The plant material was identified by the botanist Dr. Rosângela do Socorro Ferreira Rodrigues Sarquis by comparison with exsiccates deposited in a regional herbarium. Species samples were deposited in the IAN Herbarium of Embrapa Eastern Amazon under registration number 200724 [Leguminosae-Cercidoideae Bauhinia guianensis (embrapa.br)]. In addition, the use of the species for research was registered in the National System for the Management of Genetic Heritage and Associated Traditional Knowledge (SISGEN/MMA), under the record AA1DBBB.
Obtaining HELBg and HELBg
The collection of species occurred in February 2020. The materials (3,000 g leaves and 5,830 g stems) were stabilized and sanitized with 70% alcohol, followed by drying in a microprocessed oven with forced air circulation (Solab brand, model SL-102) at 40 °C for 24 h. After drying, the materials were ground separately in a knife mill (Brand Maqtron, model B-611). After the process, they had the following weights: leaf (1,105 g) and stem (2,658 g).
Subsequently, in individual amber glass containers (leaf and stem), the powdered materials were poured and macerated in a 70% hydroethanolic solution, at a proportion of 1:10 mass/volume at room temperature, for ten days, with occasional agitation. The macerate was filtered under vacuum pressure to separate the extract solution from the vegetable residue.
The extract solution was concentrated using a microprocessed rotary evaporator (QUIMIS brand, model Q344M2) at a temperature of 40 °C. The concentrated crude extracts, obtained after evaporation of the solvent, were stored in amber glasses and frozen at −80 °C. Then, they were lyophilized in a lyophilizer (LS 3000 brand, Terroni, Brazil) for 72 h, which resulted in yields of 7.73% (HESBg) and 18.53% (HELBg). Finally, the material was stored in an amber bottle, in a desiccator, until use.
Analysis of HELBg and HESBg by HPLC-DAD-ESI-MSn
Liquid chromatography-mass spectrometry instrumentation and conditions
A Shimadzu® (Kyoto, Japan) High-Performance Liquid Chromatography System, coupled with an Amazon X (Bruker Daltonics, Billerica, MA, USA) with an electrospray ion (ESI) source, was used to perform the ESI-MSn analysis. The LC system consisted of an LC-20AD solvent pump unit (flow rate of 600 µL/min), a DGU-20A5 online degasser, a CBM-20A system controller and an SPD-M20A (190 - 800 nm) diode array detector. The LC separation was performed on a Kromasil C-18 5 mm 100 Å, 250 × 4.6 μm (Kromasil, Bohus, Sweden) analytical column. Injections (20 μL) were performed using an autosampler (SIL-20A). The mobile phase consisted of 0.1% formic acid in water (solvent A) and methanol (solvent B). The exploratory gradient was performed to elution in 60 min. The analysis parameters are as follows: capillary 4.5 kV, ESI in negative mode, final plate offset 500 V, 40 psi nebulizer, dry gas (N2) with a flow rate of 8 mL/min and a temperature of 300 °C. CID fragmentation was achieved in auto MS/MS mode using advanced resolution mode for MS and MS/MS mode. The spectra (m/z 50–1500) were recorded every 2 s.
Study on acute toxicity in zebrafish
Experimental animals
The animals used were adult male and female zebrafish of the wild AB strain, approximately six months old, 3.7 to 4 cm long, weighing about 550 mg. They were acquired from the company Power Fish Pisciculture, located in Itaguaí-RJ, Brazil. Upon arrival, the animals were quarantined at the Zebrafish Platform of the Pharmaceutical Research Laboratory, Department of Biological and Health Sciences at the Federal University of Amapá (UNIFAP), Brazil. The animals were kept in water, under controlled temperature conditions (23 ± 2 °C), with food and a light/dark cycle (10/14) (Souza et al. Citation2016; Borges et al. Citation2018). The study received approval from the Ethics Committee for the Use of Animals (CEUA) of UNIFAP, which sets standards for animal welfare and limits on the number of animals used. The study was conducted under protocol number 007/2020. The acute toxicity study in adult zebrafish and embryos took place during the month of January 2021.
Treatment groups and administration routes
Adult animals were treated in triplicate (totaling 180 animals) by gavage according to methods described by Souza et al. (Citation2019), a volumetric pipette was used (HTL Lab Solutions Co., São Paulo, Brazil), with a dose volume according to the weight of each animal. Subsequently, they were placed on a damp sponge with a hollow center to hold the animal, positioning it with its mouth facing upwards to receive the dose. The pipette tip was gently inserted 1 to 2 mm into the animal’s mouth, at an angle of 90°. Oral administration took an average of 5 s, then the animals were placed in separate tanks under the same environmental conditions as the system (25 ± 2 °C) for recovery and behavioral observation. It is important to highlight that this protocol allowed a quick assessment of the animal’s resistance, through the return of swimming activity, otherwise it could be replaced by another animal, while the embryos were subjected in triplicate to the immersion process in controls and test extracts, totaling the use of 240 embryos (Tomazi et al. Citation2021).
Study with zebrafish embryos
For embryo reproduction, two males for each female were used in breeding aquariums with artificial ornamentation (Tecniplast), separated one day before spawning. Embryo spawning occurred at 6 am, as soon as the light period began. Zebrafish embryos were carefully cleaned and selected by stereomicroscopic examination (Olympus, Japan). Fertilized eggs without cleavage alterations or damage to the chorion were selected and transferred to Petri dishes containing the controls groups (A - system water and vehicle B − 1% dimethyl sulfoxide - DMSO); concentrations of HESBg (S1 − 0.25, S2 − 0.5, S3 − 0.75, S4 − 1.0, and S5 − 1.5 µg/mL) and HELBg (L1 − 0.25, L2 − 0.5, L3 − 0.75, L4 − 1.0, and L5 − 1.5 µg/mL) ().
The embryotoxicity test in fish was performed following the OECD Guidelines for the Testing of Chemicals, 236 OECD (OECD Citation2013). Two hundred and forty eggs fertilized within 3 h post fertilization (hpf) were randomly selected and divided into six plates of 96 wells with one egg/well. Embryo groups (n = 20 animals/group) were transferred to 96 wells with a Pasteur pipette, placed individually in wells containing 250 µL of the control or studied extract, and subsequently incubated in an oven (SOLAB SL-102/630, Brazil) at 28 ± 2 °C for 96 hpf. The embryonic lethality characteristics analyzed were egg coagulation, absence of heartbeat, absence of hatching, and change in heart rate (24, 48, 72, and 96 hpf). A positive result in any of these traits meant the embryo’s death. In addition, teratogenesis parameters were evaluated, including yolk edema, growth retardation (24, 48, 72, and 96 hpf), tail malformation, cardiac edema (48, 72, 96, and 120 hpf), and scoliosis (72, and 96 hpf) being evaluated every 24 h using a stereomicroscope (Olympus, Japan) (Cerbaro et al. Citation2020; Tomazi et al. Citation2021).
Study with adult zebrafish
The acute toxicity study in adult zebrafish was based on the Organization for Economic Co-operation and Development (OECD) guidelines, with 425 OECD adaptations (OECD Citation2022). The toxicity test consisted of three steps: determination of LD50, behavioral changes, mortality, and histopathological changes ().
After the acclimatization period, the adult animals were randomly divided into 12 groups (n = 5) and deprived of food for 24 h before the beginning of the experiments. Then, they were divided into two control groups treated with saline solution and 1% DMSO (vehicle), five groups treated with doses of 100, 200, 500, 1000, and 2000 mg/kg of HESBg, and five groups treated with doses of 100, 200, 500, 1000, and 2000 mg/kg of HELBg, respectively. The animals were initially weighed and treated with adaptations of the methods described by Sampaio et al. (Citation2018) and Melo et al. (Citation2019), the animals were gently handled with a moist sponge with a hollow center, holding the animal vertically, and with the aid of a volumetric pipette, the doses were administered by gavage. After the observation period of the tests (48 h), the animals were euthanized under anesthetic cooling, water cold (< −2 °C) to loss of righting reflex, time at which the fish became unable to maintain a normal upright position, following the recommendations of the American Guidelines of the Veterinary Medical Association for Animal Euthanasia (Leary et al. Citation2013).
Determination of LD50
After the gavage procedure, the animals were individually distributed in aquaria containing system water. Each animal was evaluated for 48 h and considered dead in the absence of response to mechanical stimulation and absence of movement of the operculum (Souza et al. Citation2016).
Behavioral analysis and mortality
After the gavage procedure, the animals were observed for behavioral changes, classified as Stage I: increased swimming activity; spasms; tail tremors; Stage II: circular swimming; loss of posture; Stage III: clonus; loss of motility; animal standing at the bottom of the aquarium and death. In the absence of response to mechanical stimulation and lack of movement of the operculum, the animal was considered dead (Souza et al. Citation2016).
Histopathology
Organ preparation
For histopathology of the organs (liver, intestine, and kidney), after euthanasia in water cold (< −2 °C), the animals were fixed in Bouin’s solution for 24 h, then decalcified in EDTA (ethylenediaminetetraacetic acid, Sigma Co., São Paulo, Brazil) for 24 h. The samples were processed and stained according to the techniques described by Souza et al. (Citation2016) and Hyacienth et al. (Citation2020). The images were analyzed using an Olympus BX41-Micronal microscope and photographed with an MDCE-5C USB 2.0 (digital) camera.
Evaluation of histopathological changes
Tissue alterations in the intestine, liver, and kidneys were analyzed according to the techniques described by Souza et al. (Citation2016), Carvalho et al. (Citation2018), and Hyacienth et al. (Citation2020). The Index of Histopathological Changes (IHC) was calculated based on the tissue changes observed in the organs (levels I, II, and III). The IHC value classified the alterations as normal (0-10), moderately altered (11-20), moderate to severe (21-50), or containing severe irreversible alterations (>100) (Poleksic and Mitrovic-Tutundzic Citation1994; Rigolin-Sá Citation1998; Tomazi et al. Citation2021).
Statistical analysis
The results obtained were expressed as mean ± standard deviation (SD) of each experimental group. The mean lethal dose (LD50) of the extracts was determined by probit analysis. For the experimental parameters of the histopathological study, the one-way ANOVA test was used, followed by Dunnett’s post hoc test. Statistical analyses were performed using the GraphPad Prism program (version 5.03). Results with p < 0.05 were considered statistically significant.
Results
Mass spectrometry high-performance Liquid Chromatography analysis (LC-DAD-MS/MS)
The HPLC-DAD-ESI-MSn analysis detected the presence of 55 compounds in HELBg and HESBg ( and ), in negative ionization mode, with HELBg showing four main peaks: 36.2′ myricetin-O-pentoside (myricetin glycosides) and 39.0′ methylmyricetin-O-deoxyhexoside (methylmyricetin glycosides) in the base peak chromatogram (BPC) (A); 35.9′ myricetin-O-deoxyhexoside (myricetin glycosides) and 38.4′ myricetin-O-(O-galloyl)-deoxyhexoside (myricetin glycosides) in chromatogram UV (B) in 254 nm.
Figure 3. Base peak chromatogram (BPC) in negative ion mode (A) and UV chromatogram in 254 nm (B) of hydroethanolic extract from of B. guianensis leaves by HPLC-DAD-ESI-MSn.
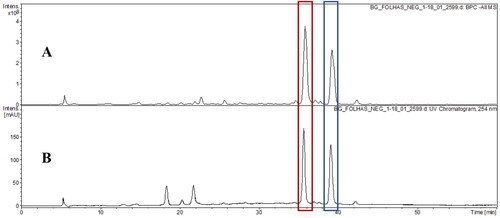
Figure 4. Base peak chromatogram (BPC) in negative ion mode (A) and UV chromatogram in 254 nm (B) of hydroethanolic extract from of B. guianensis stems by HPLC-DAD-ESI-MSn.
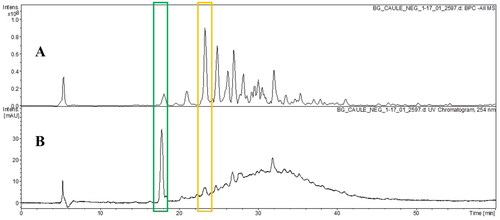
In HESBg, two main peaks were identified in 23.0′ (Epi)afzelechin-(→B→)- (epi)catechin-(→B→)-(epi)catechin (B-type procyanidin trimer) in BPC (A) and in 18.5′ (Epi)gallocatechin-(→B→)-3′-O-galloyl(epi)gallocatechin (B-type procyanidins esterified by one galloyl units) in the chromatogram UV (B) in 254 nm (B).
Retention times and mass spectral data, along with peak assignments for compounds identified using negative ionization, are described in .
Table 1. Characterization of the compounds tentatively identified by HPLC-ESI-MSn in B. guianensis Aubl..
Effects of HELBg and HESBg on zebrafish embryos
Embryos exposed to HELBg and HESBg solutions were evaluated for teratogenic effects and lethality (coagulation and absence of heartbeat) by 96 hpf. No teratogenic alterations were observed in the embryos.
When comparing the HESBg treatment groups (S1 − 0.25, S2 − 0.5, S3 − 0.75, S4 − 1.0, and S5 − 1.5 µg/mL) to the control groups (A - system water and B − 1% DMSO), average lethal clotting effects on zebrafish embryos ranged from 2.0 ± 1.414 at S1 to 2.5 ± 0.707 at S2 to S5 concentrations. On the other hand, in the HELBg treatment groups (L1 − 0.25 to L5 − 1.5 µg/mL), coagulation was observed at L1 (average of 0.5 ± 0.707), L2 and L3 (1.5 ± 0.707), and was more significant at higher doses L4 and L5 (3.5 ± 0.707) ().
Table 2. Overview of lethal effects of the HESBg and HELBg on zebrafish embryos at 96 hpf.
Heartbeat absence was evident in HESBg: S1 (4.0 ± 0.0), S2 (7 ± 1.414), S3 (6.5 ± 0.707), S4 (6.5 ± 0.707), S5 (7.5 ± 0.707). In HELBg, heartbeat absence was discreetly observed: L1 (0.5 ± 0.707), L2 and L3 (1.0 ± 0.0), L4 and L5 (1.5 ± 0.707).
Regarding hatching rate, 100% of zebrafish embryos submerged in system water and 98% in 1% DMSO (vehicle) hatched. Comparing the different concentrations of HESBg to the control group, approximately 50% of embryos exposed to dose S1 hatched, while hatching rates for S2 to S4 were around 20%. The highest dose, S5, had a hatching rate of less than 10% (p < 0.05) ().
Figure 5. Zebrafish embryo hatching rate. In A, embryos treated with controls (A - system water and B – DMSO 1%) and concentrations S1 - 0.25 µg/mL, S2 - 0.5 µg/mL, S3 - 0.75 µg/mL, S4 - 1.0 µg/mL and S5 - 1.5 µg/mL of HESBg. In B, embryos treated with controls (system water and DMSO 1%) and concentrations of L1 - 0.25 µg/mL, L2 - 0.5 µg/mL, L3 - 0.75 µg/mL, L4 - 1.0 µg/mL and L5 - 1.5 µg/mL of HELBg. Data are presented as mean ± SD (n = 20/group); *p < 0.05 for group water and #p < 0.05 for group DMSO 1%. Statistical analysis was performed through one-way ANOVA followed by the post hoc Tukey test.
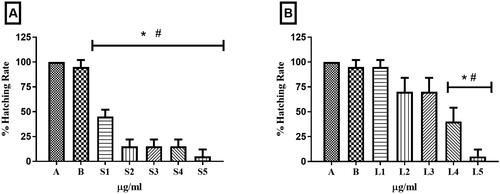
In HELBg, lower concentrations (L1: 98%, L2 and L3: 74%) exhibited higher hatching percentages, while higher concentrations (L4: 40% and L5: <10%) were significantly different from the controls (p < 0.05).
The heart rate of embryos exposed to HESBg concentrations was only higher than 150 bpm at dose S1 compared to controls. In HELBg-exposed embryos, only the L1 concentration had a heartbeat count similar to the controls. Higher concentrations (L2 to L5) resulted in a decrease in heartbeats per minute as the dose increased (). Some embryos exhibited developmental delay ().
Figure 6. Heart rate in zebrafish embryos. In A, embryos treated with controls (A - system water and B – DMSO 1%) and concentrations S1 - 0.25 µg/mL, S2 - 0.5 µg/mL, S3 - 0.75 µg/mL, S4 - 1.0 µg/mL and S5 - 1.5 µg/mL of HESBg. In B, embryos treated with controls (system water and DMSO 1%) and concentrations of L1 - 0.25 µg/mL, L2 - 0.5 µg/mL, L3 - 0.75 µg/mL, L4 - 1.0 µg/mL and L5 - 1.5 µg/mL do HELBg. Data are presented as mean ± SD (n = 20/group); *p < 0.05 for group water and #p < 0.05 for group DMSO 1%. Statistical analysis was performed through one-way ANOVA followed by the post hoc Tukey test.
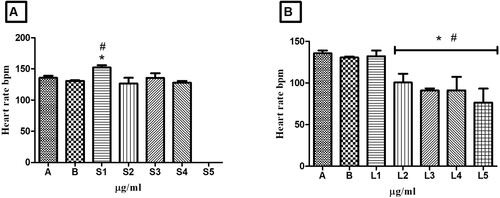
Figure 7. Zebrafish embryo micrographs at 24, 48, 72 and 96 hpf. A) normal embryo exposed to system water at 96 hpf; B) embryos treated with 100 µg/L DMSO 1% at 96 hpf, without developmental changes; C) embryo treated with 1.5 µg/mL of HESBg at 72 hpf, without developmental changes; D) embryo treated with 1.5 µg/mL of HELBg at 72 hpf, without developmental changes; E) embryo treated with 1.5 µg/mL of HELBg at 96 hpf, without developmental changes; F) embryo treated with 1.5 µg/mL of HELBg at 24 hpf, with developmental delay; G) embryo treated with 1.5 µg/mL of HELBg at 48 hpf, with developmental delay; H) embryo treated with 1.5 µg/mL of HESBg at 96 hpf, killed for not hatching.
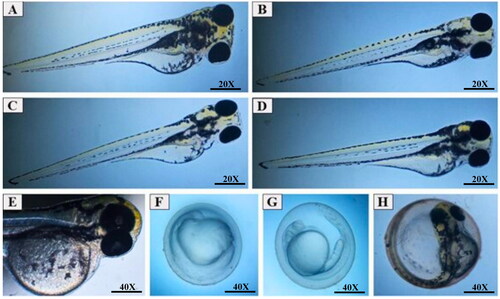
Evaluation of acute toxicity in adult zebrafish: behavioral analysis
Treatment with oral doses of HELBg and HESBg (100, 200, 500, 1000, and 2000 mg/kg) induced significant behavioral changes. The highest percentage of alterations in animals treated with HELBg was observed at the three highest doses (500, 1000, and 2000 mg/kg) (). Animals treated with HESBg also exhibited behavioral changes, with the lowest percentage observed at the lowest dose of the substance (100 mg/kg) (). Signs of stress included spasms, tail tremors, loss of posture and motility, remaining at the bottom of the aquarium, and death.
Table 3. Behavioral changes of treatments with different doses of HELBg and controls in zebrafish at different hours of observation.
Table 4. Behavioral changes of treatments with different doses of HESBg and controls in zebrafish at different hours of observation.
Assessment of acute toxicity in adult zebrafish: determination of LD50
demonstrates that animal lethality is dose-dependent. The LD50 for animals treated with HESBg was 1717 mg/kg. However, for animals treated with HELBg, the LD50 surpassed the highest dose administered (2000 mg/kg), as the animals survived ().
Histopathology
Oral treatments of HELBg and HESBg induced histopathological changes in the liver, intestine, and kidneys of zebrafish at varying doses (). Treatment with 1% DMSO resulted in minimal changes in these organs, while no changes were observed in animals treated with system water.
Figure 9. Index Of Histopathological Changes in zebrafish liver. In A: liver of animals treated with controls (A - system water and B – DMSO 1%), doses S1 – 100 mg/kg, S2 – 200 mg/kg, S3 – 500 mg/kg, S4 – 1000 mg/kg and S5 – 2000 mg/kg do HESBg. In B: liver of animals treated with controls (A - system water and B – DMSO 1%), doses L1 - 100 mg/kg, L2 - 200 mg/kg, L3 - 500 mg/kg, L4 - 1000 mg/kg and L5 - 2000 mg/kg of HELBg. Data are presented as mean ± SD (n = 5/group); *p < 0.05 for group water and #p < 0.05 for group DMSO. Statistical analysis was performed through one-way ANOVA followed by the post hoc Tukey test.
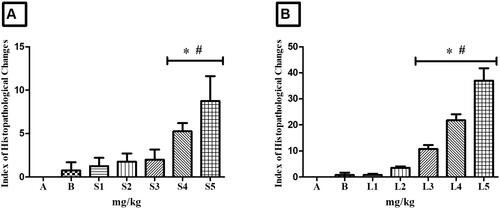
Figure 10. Histopathological changes observed in zebrafish liver, intestine and kidneys in different treatments. In A, B and C, liver tissue with normal hepatocytes (H), cytoplasmic vacuolization (VaC), nuclear atypia (an), nuclear atrophy (AtN), nuclear degeneration (DgN) and cell degeneration (DgC); in D, E and F, renal tissue with normal glomerulus (G), Bowman’s capsule space (ECB), tubular degeneration (DgT), mild tubular hyaline degeneration (DgHL) and severe hyaline degeneration (DgHS); in G, H and I, intestinal tissue with normal goblet cells (GC), lymphocyte infiltration (ILi), goblet cell hypertrophy (HtCC), dilation of vessels present in the villi (DVV), stromal leukocyte infiltration (ILe) as well as displacement of the lamina propria (DLP). Coloring (H&E).
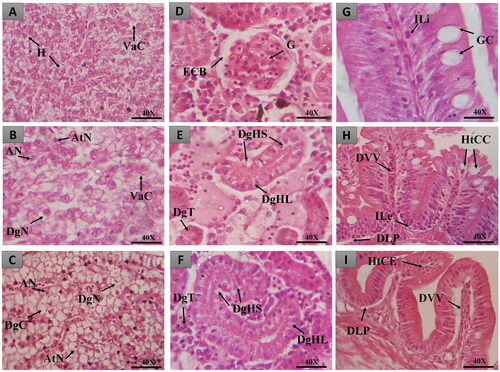
Figure 11. Index of Histopathological Changes in zebrafish intestine. In A: intestine of animals treated with controls (A - system water and B – DMSO 1%), doses S1 - 100 mg/kg, S2 - 200 mg/kg, S3 - 500 mg/kg, S4 - 1000 mg/kg and S5 - 2000 mg/kg of HESBg. In B: intestine of animals treated with controls (A - system water and B – DMSO 1%), doses L1 - 100 mg/kg, L2 - 200 mg/kg, L3 - 500 mg/kg, L4 - 1000 mg/kg and L5 - 2000 mg/kg of HELBg. Data are presented as mean ± SD (n = 5/group); *p < 0.05 for group water and #p < 0.05 for group DMSO 1%. Statistical analysis was performed through one-way ANOVA followed by the post hoc Tukey test.
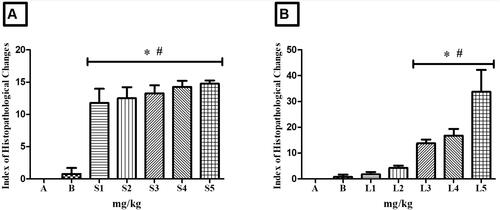
Figure 12. Index of Histopathological Changes in zebrafish kidney. In A: kidney of animals treated with controls (A - system water and B - DMSO), doses S1 - 100 mg/kg, S2 - 200 mg/kg, S3 - 500 mg/kg, S4 - 1000 mg/kg and S5 - 2000 mg/kg of HESBg. In B: kidney of animals treated with controls (A - system water and B - DMSO), doses L1 - 100 mg/kg, L2 - 200 mg/kg, L3 - 500 mg/kg, L4 - 1000 mg/kg and L5 - 2000 mg/kg of the HELBg. Data are presented as mean ± SD (n = 5/group); *p < 0.05 for group water and #p < 0.05 for group DMSO. Statistical analysis was performed through one-way ANOVA followed by the post hoc Tukey test.
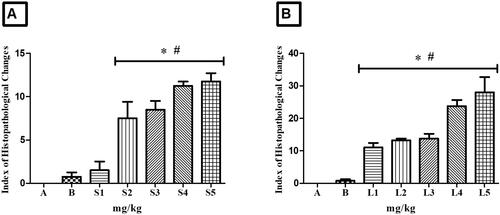
Animals treated with HESBg (doses S4 and S5) exhibited stage I histopathological changes in the liver, indicating normal organ functionality (). Common alterations included loss or atypia of cellular/nuclear contour, increased volume, cytoplasmic vacuolization, decreased glycogen, and biliary stagnation.
For HELBg, doses of 500, 1000, and 2000 mg/kg caused mild to moderate liver variations, as shown in . Stage I alterations included loss or atypia of cellular/nuclear contour, increased cell volume, cytoplasmic vacuolization, decreased glycogen, and biliary stagnation. Stage II alterations comprised nuclear atrophy, decreased frequency of nuclei, cytoplasmic/nuclear degeneration, and hyperemia. Statistical analysis showed significant differences (p > 0.05) compared to control groups.
Oral treatment with HESBg (S1-S5) resulted in mild to moderate histopathological variations in the intestine, as indicated in . Common alterations included detachment of the epithelial lining, villous atrophy, epithelial cell hypertrophy, goblet cell hyperplasia, muscle layer degeneration, stromal lymphocyte infiltration, thinning of the lamina propria, enterocyte vacuolization, leukocyte infiltration, and cell degeneration (stage I). Displacement of the lamina propria was observed in stage II.
Similarly, HELBg (L3-L5) caused mild to moderate histopathological changes in the zebrafish intestine, as shown in . Alterations included vessel dilation, detachment of the epithelial lining, villous atrophy, villous hypertrophy, epithelial cell hypertrophy, goblet cell hyperplasia, muscle layer degeneration, stromal lymphocyte infiltration, leukocyte infiltration, cell degeneration (stage I), and displacement of the lamina propria, desquamation of the mucosa, hemorrhage in the lamina propria, and villous degeneration (stage II). Statistical analysis confirmed significant differences (p < 0.05) compared to control groups.
Oral administration of HESBg (S2-S5) resulted in mild to moderate histopathological changes in the kidneys, including loss or atypical contour of cells, tubular hyaline degeneration, cell hypertrophy, tubular disorganization, decreased Bowman’s capsule space, dilated glomerular capillaries, regenerating tubules, tubular obstruction, enlarged tubular lumen (stage I), and hyperemia (stage II) ().
Animals treated with HELBg displayed mild to moderate histopathological variations in the kidneys, with tubular hyaline degeneration, cell hypertrophy, decreased Bowman’s capsule space, dilated glomerular capillaries, increased tubular lumen (stage I), and hyaline tubular degeneration, tubular degeneration, cytoplasmic degeneration of tubular cells, nuclear degeneration of tubular cells, and hyperemia (stage II) ().
Discussion
In this study, hydroethanolic extracts of leaves and stem of B. guianensis produced toxic effects on embryos and specific organs of adult zebrafish.
Using HPLC-DAD-ESI-MSn, the compounds were provisionally characterized, and their fragmentation patterns were obtained from mass spectra. In this step, the presence of 55 compounds in HELBg and HESBg was identified, the main chemical constituents found are proanthocyanidins. The identification was possible due to four main fragmentation mechanisms: retro-Diels-Alder (RDA), quinone methide (QM), heterocyclic ring fission (HFR) and benzofuranforming (BFF). The galloyl residue was assigned based on the loss of a gallic acid (170 Da or 152 Da, neutral loss). In addition, the peaks were evaluated according to UV 280 nm absorbance (Jaiswal et al. Citation2012; Saldanha et al. Citation2013; Tala et al. Citation2013; Dantas et al. Citation2021).
Procyanidins are composed exclusively of catechin and epicatechin and can be categorized into type A or B according to their configuration and the connection between the monomers. Type B procyanidins are more abundant and have a single interflavan bond between the C-4 carbon of the B ring and the C-8 or C-6 carbon of the C ring; alternatively, type-A procyanidins are characterized by having an additional ether bond between the C-2 carbon of the A ring and the hydroxyl group attached to the C-7 carbon of the ring C (Jaiswal et al. Citation2012; Saldanha et al. Citation2013; Tala et al. Citation2013; Dantas et al. Citation2021).
Among the compounds shown are (Epi)gallocatechin-(→B→)-3′-O-galloyl(epi)gallocatechin (HELBg and HESBg) (Jaiswal et al. Citation2012), (Epi)afzelechin-(→B→)- (epi)catechin-(→B→)-(epi)catechin (HESBg) (Gu et al. Citation2003), myricetin-O-deoxyhexoside (L), myricetin-O-pentoside (HELBg), myricetin-O-(O-galloyl)-deoxyhexoside (HELBg) (Saldanha et al. Citation2013), methylmyricetin-O-deoxyhexoside (HELBg) (Wang et al. Citation2018, Citation2019).
The analysis suggests that the pharmacological activity of B. guianensis is linked to procyanidins, potent compounds known for their antioxidant properties and ability to stabilize cell membranes (David et al. Citation2017), anti-inflammatory and antialgic (Carvalho et al. Citation1999; Carvalho Citation2017).
The administration of HELBg and HESBg through oral and immersion routes resulted in toxicity effects in zebrafish, contributing novel insights in both embryos and adults. Previous studies have focused on the crude methanolic extract of B. guianensis, determining its lethal dose in wistar rats (Marconato et al. Citation1998; Carvalho et al. Citation1999). Likewise, the extracts from this plant species exhibited properties consistent with traditional use in treating inflammatory conditions, as demonstrated in carrageenan-induced edema tests conducted on swiss mice. The findings emphasize the importance of further investigations to evaluate the safety, efficacy, and isolation of bioactive components from B. guianensis (Carvalho et al. Citation1999).
HELBg and HESBg exhibited lethal effects in zebrafish embryos (immersion) and adults (gavage), leading to behavioral changes, organ-specific alterations, and mortality. The metabolic and systemic interactions in zebrafish make them an effective animal model for defining toxicity parameters and mimicking human physiological processes. This offers several advantages for discovering new pharmacological agents by studying tissue changes in adult zebrafish and assessing substance toxicity effects in embryos (Ducharme et al. Citation2015; Hyacienth et al. Citation2020).
Embryo coagulation, a key acute toxicity endpoint, was more pronounced at higher doses of HELBg, aligning with OECD Chemicals Assay Guidelines no. 236 (OECD Citation2013). Coagulation is characterized by chorion turbidity and absence of heartbeats, occurring as premature death at 24 hpf or later stages such as 96 hpf (von Hellfeld et al. Citation2020). Another study confirmed higher coagulation rates at elevated substance doses (Ferreira et al. Citation2019).
The observed embryo coagulation following HELBg administration (L4 and L5) indicated that procyanidin compounds crossed the chorionic membrane and were absorbed by the embryos, causing significant toxicity (Vallverdú-Queralt et al. Citation2015).
The absence of heartbeats in zebrafish embryos, observed with all HESBg doses, suggested the involvement of procyanidins in this adverse effect. These phenolic compounds interact with membrane receptors, penetrate the chorion, and negatively affect the heart (Makarova et al. Citation2016; Sarmah et al. Citation2020).
As the doses of HELBg and HESBg increased, the hatching rate of embryos decreased, aligning with the observed clotting. Extracts acting as chemical stimuli inhibited chorionase enzyme production associated with hatching, similar to a study involving grape seed proanthocyanidin extracts and zebrafish embryos survival rates (Cerbaro et al. Citation2020).
In zebrafish treated with HESBg, the embryonic heart rate (S1) was higher than control, remaining within acceptable limits (120-180 bpm). In HELBg-exposed embryos, only the L1 concentration showed heartbeats similar to controls, while higher concentrations exhibited decreased heart rates. Heart rate monitoring is informative for assessing cardiac toxicity in zebrafish (Rubinstein Citation2006) as demonstrated in studies with extracts from Cinnamon zeylanicum Blaume (Lauraceae) bark and Eugenia polyantha Wight (Myrtaceae) leaves, which showed increased toxicity effects on survival, organ malformation, abnormal heartbeats, and delayed incubation rates (Ismail et al. Citation2017).
The study findings reveal that chemical-induced changes in zebrafish embryos can lead to developmental defects, as observed in previous studies (Rubinstein Citation2006; Kitipaspallop et al. Citation2021). Zebrafish embryos are particularly sensitive to external factors like chemicals, mechanical stress, and temperature during early development, as emphasized by Mandrell et al. (Citation2012).
The acute toxicity effects of HELBg and HESBg on adult zebrafish were analyzed. HELBg-treated animals displayed alterations in swimming activity, spasms, loss of posture, and standing at the bottom of the aquarium. Similarly, HESBg-treated animals showed increased swimming activity, spasms, tail tremors, loss of posture, and standing at the bottom of the aquarium. These behavioral changes indicate the response of fish to stressors (Kane et al. Citation2005; Souza et al. Citation2016; Carvalho et al. Citation2018).
The increase in swimming activity is an example, demonstrating the plausible indicator of the animal’s escape from the stressful situation to which it was exposed when receiving, orally, doses of the extracts under study (Little et al. Citation1993; Borges et al. Citation2018).
The LD50 of the extracts, determined through oral administration, indicated low toxicity (OECD Citation2002; Citation2022). Although the ethnopharmacological applications of extracts from B. guianensis are common in the Amazon, the metabolites responsible for the pharmacological effects, the action mechanism and the toxicity of the extracts remain practically unknown (Koga et al. Citation2021). So, the results of this work may motivate other experimental preclinical studies with B. guianensis to identify, besides the low toxicity, possible applications of the species in clinical research - which can be studied from the combination of the compounds found in HELBg and HESBg.
Histopathological evaluation of zebrafish internal organs, such as liver, intestine and kidneys, is useful to correlate the possible toxicological results of orally administered plant extracts in zebrafish (Madureira et al. Citation2012; Ferreira et al. Citation2019). Furthermore, in toxicity studies, the ‘gold standard’ for evaluating the effect of a compound on an animal system (and identifying target organs) is histopathological observation, that is, the observation of a possible toxic effect of the studied compound (Fuchs and Hewitt Citation2011).
Animals exposed to S4 and S5 oral doses of HESBg showed level I alterations in hepatocytes – which, according to classifications and parameters from previous studies, indicate normal organ functioning (Poleksic and Mitrovic-Tutundzic Citation1994; Takashima and Hibiya Citation1995; Rigolin-Sá Citation1998). Animals exposed to HELBg (L3-L5), despite having only level I alterations in hepatocytes, were the most significant in this regard, considering that the liver IHC after the administration of oral doses of the extract was higher compared to the one in the control groups. Hence, the high number of alterations observed classifies them as mild to moderate. It is known that the zebrafish liver, as well as the mammalian liver, plays an important role in the metabolic homeostasis of the organism, comprising the processing of carbohydrates, proteins, lipids, and vitamins (Roberts and Ellis Citation2001; Evensen Citation2006). In addition, the organ is responsible for the detoxification and synthesis of serum proteins, including albumin, fibrinogen, complement factors, and acute phase proteins.
As observed in the present study, other authors also demonstrated small histopathological changes in the liver of zebrafish when submitted to oral treatment with aqueous extract of Hancornia speciosa Gomes (Apocynaceae), which contained proanthocyanidins extracted in ethanolic solution from the species’ bark (Moraes et al. Citation2008; Tomazi et al. Citation2021). Furthermore, evaluating the histopathological changes in the zebrafish liver in toxicity studies such as this one allows us to observe in an animal model, in terms of drug development and clinical therapy, potential compounds obtained from natural products (Cheng et al. Citation2016). Other studies with extracts of B. guianensis can, in other circumstances, evaluate the xenobiotic metabolism of procyanidins present in the extract in an animal model of zebrafish.
The intestine, another organ evaluated after oral administration of HESBg and HELBg, also showed statistical significance in the groups tested (S1-S5 and L3-L5) compared to controls, with alterations classified, according to the IHC, as mild to moderate. Zebrafish has become a good model organism for evaluating chemicals that induce intestinal toxicity. It is a critical assessment, especially considering the high likelihood of oral ingestion of natural products, which can be potentially toxic. In zebrafish studies, the digestive tract serves as the first physiological barrier to orally ingested foreign materials. For this reason, the observation of intestinal toxicity is necessary, since the mucosal lining of the digestive tract is the first barrier to oral xenobiotics (Ryu et al. Citation2018). Furthermore, the zebrafish intestinal toxicity model has anatomically conserved enterohepatic and cholangiocyte structures that resemble the ones in humans, which is why it is proposed as a useful animal model in intestinal toxicity (Williams and Hong Citation2011).
Studies on the absorption and metabolism of procyanidins have revealed that, in both humans and animals, (+) - catechin and (-) - epicatechin are rapidly absorbed by the upper part of the small intestine (Ou and Gu Citation2014). Procyanidin B2 extracted from grape seeds, when injected into the small intestine of rats, is absorbed in the small intestine of these animals. It has been observed that the absorption occurs in the small intestine due to the type B dimer of procyanidin (Appeldoorn et al. Citation2009). Such research corroborates the results of the present study, as it can be inferred that the extracts spent a significant contact period with the intestine. This also explains the local toxicity of enterocytes, which, as seen, damaged the entire structure of the intestinal wall and caused an inflammatory process (Hyacienth et al. Citation2020).
In the results of renal histopathology, after administration of HELBg and HESBg, changes were mild to moderate in zebrafish kidneys. The kidney is frequently evaluated in preclinical safety studies. Its vital function is drug excretion and detoxification, making it an important organ that presents toxic responses to certain compounds (Fuchs and Hewitt Citation2011).
The area and intensity of kidney damage sustained can be directly observed and characterized. This renal exposure to high levels of metabolites can be seen through cellular damage due to increased blood flow, clearance, and metabolism of xenobiotics (Werner et al. Citation1995).
The most common alterations in the present study were of tubular origin. Other works suggest that in studies of acute toxicity in zebrafish kidneys, the main focus in kidney injury research is proximal tubule toxicity. Compound toxicity screening in zebrafish using renal histology data is the primary endpoint in preclinical toxicology (Fuchs and Hewitt Citation2011; Cassar et al. Citation2020).
Therefore, this is the first study that evaluates the acute toxicity of HESBg and HELBg in vivo with emphasis on the zebrafish embryonic stage, considering the teratogenic changes and the lethal effect (coagulation and absence of heartbeat), and, in adult zebrafish, behavioral aspects, mortality and histopathological changes. Despite the relevant potential of procyanidins present in B. guianensis, it is necessary to expand the studies so that their use by traditional populations can be proven. Hence, it must be used safely.
Conclusions
Assessment of acute toxicity in zebrafish exposed to Bauhinia guianensis extracts revealed significant effects on embryonic development and adult physiology. These results support the relative safety of the species’ ethnopharmacological applications, underlining the need for additional clinical trials to further explore its therapeutic potential, based on its promising pharmacological and toxicological characteristics, arising from its use in traditional Amazonian medicine.
Author contributions
The R.C.R.K. and G.C.S. conducted the experiments and euthanized the zebrafish. The A.V.T.L.T., A.M.F. and B.L.S.O. performed the data analysis. The L.S.A., and J.F.T. realized the phytochemistry studies, and J.C.T.C. conceptualization; Funding acquisition; Final data curation and project administration.
Disclosure statement
The authors report that there are no competing interests to declare.
Additional information
Funding
References
- Agustina R-M, Dealmy D-G, Verónica B-R, Jolanta EM, Hady K, Ansoumane K, Sergio ARG, Jesús RRA, Carvalho JCT. 2020. Jatropha dioica, an Aztec plant with promising pharmacological properties: a systematic review. Afr J Pharm Pharmacol. 14(6):169–178. doi:10.5897/AJPP2020.5147.
- Appeldoorn MM, Vincken J-P, Gruppen H, Hollman PCH. 2009. Procyanidin dimers A1, A2, and B2 are absorbed without conjugation or methylation from the small intestine of rats. J Nutr. 139(8):1469–1473. doi:10.3945/jn.109.106765.
- Barbosa FDF, Barbosa LCA, Melo EDC, Botelho FM, Santos RHS. 2006. Effect of drying air temperature upon the content and chemical composition of the essential oil from Lippia alba (Mill) N. E. Brown. Química Nova. 29.1221–1225. doi:10.1590/S0100-40422006000600014.
- Borges RS, Keita H, Ortiz BLS, dos Santos Sampaio TI, Ferreira IM, Lima ES, de Jesus Amazonas da Silva M, Fernandes CP, de Faria Mota Oliveira AEM, da Conceição EC, et al. 2018. Anti-inflammatory activity of nanoemulsions of essential oil from Rosmarinus officinalis L.: in vitro and in zebrafish studies. Inflammopharmacology. 26(4):1057–1080. doi:10.1007/s10787-017-0438-9.
- Borges RS, Lima ES, Keita H, Ferreira IM, Fernandes CP, Cruz RAS, Duarte JL, Velázquez-Moyado J, Ortiz BLS, Castro AN, et al. 2018. Anti-inflammatory and antalgic actions of a nanoemulsion of Rosmarinus officinalis L. essential oil and a molecular docking study of its major chemical constituents. Inflammopharmacology. 26(1):183–195. doi:10.1007/s10787-017-0374-8.
- Calixto JB. 2003. Biodiversidade como fonte de medicamentos. Cienc Cult [Internet]. [55(3):37–39. accessed 2021 Dec 15]. http://cienciaecultura.bvs.br/scielo.php?script=sci_arttext&pid=S0009-67252003000300022&lng=en&nrm=iso&tlng=pt.
- Carvalho J. 2017. Fitoterápicos anti-inflamatórios. 2nd ed. São Paulo: pharmabooks.
- Carvalho JCT, Keita H, Santana GR, de Souza GC, dos Santos IVF, Amado JRR, Kourouma A, Prada AL, de Oliveira Carvalho H, Silva ML. 2018. Effects of Bothrops alternatus venom in zebrafish: a histopathological study. Inflammopharmacology. 26(1):273–284. doi:10.1007/s10787-017-0362-z.
- Carvalho ACB, Lana TN, Perfeito JPS, Silveira D. 2018. The Brazilian market of herbal medicinal products and the impacts of the new legislation on traditional medicines. J Ethnopharmacol. 212:29–35. doi:10.1016/j.jep.2017.09.040.
- Carvalho JCT, Santos LS, Viana EP, de Almeida SSMS, Marconato E, Rodrigues M, Ferreira LR, Van de Kamp A. 1999. Anti-inflammatory and analgesic activities of the crude extracts from stem bark of Bauhinia guianensis. Pharm Biol. 37(4):281–284. doi:10.1076/phbi.37.4.281.5803.
- Cassar S, Adatto I, Freeman JL, Gamse JT, Iturria I, Lawrence C, Muriana A, Peterson RT, Van Cruchten S, Zon LI. 2020. Use of Zebrafish in drug discovery toxicology. Chem Res Toxicol. 33(1):95–118. doi:10.1021/acs.chemrestox.9b00335.
- Cerbaro AF, Rodrigues VSB, Rigotti M, Branco CS, Rech G, de Oliveira DL, Salvador M. 2020. Grape seed proanthocyanidins improves mitochondrial function and reduces oxidative stress through an increase in sirtuin 3 expression in EA.hy926 cells in high glucose condition. Mol Biol Rep. 47(5):3319–3330. doi:10.1007/s11033-020-05401-x.
- Cheng D, Shami GJ, Morsch M, Chung RS, Braet F. 2016. Ultrastructural mapping of the zebrafish gastrointestinal system as a basis for experimental drug studies. Biomed Res Int. 2016:8758460. doi:10.1155/2016/8758460.
- Dantas CAG, Abreu LS, Cunha HN, Veloso CAG, Souto AL, Fátima Agra M, Oliveira Costa VC, Silva MS, Tavares JF. 2021. Dereplication of phenolic derivatives of three Erythroxylum species using liquid chromatography coupled with ESI-MS n and HRESIMS. Phytochem Anal. 32(6):1011–1026. doi:10.1002/pca.3043.
- David J, Barreiros A, David J. 2017. Antioxidantes de Fontes Naturais. In Fitoterápicos anti-inflamatórios. 2nd ed. São Paulo: Pharmabooks; p. 105–137.
- Ducharme NA, Reif DM, Gustafsson J-A, Bondesson M. 2015. Comparison of toxicity values across zebrafish early life stages and mammalian studies: implications for chemical testing. Reprod Toxicol. 55:3–10. doi:10.1016/j.reprotox.2014.09.005.
- Evensen O. 2006. In Systemic Pathology of Fish. 2nd ed. London: scotian Press.
- Ferreira DQ, Ferraz TO, Araújo RS, Cruz RAS, Fernandes CP, Souza GC, Ortiz BLS, Sarquis RSFR, Miranda JCMM, Garrett R, et al. 2019. Libidibia ferrea (jucá), a traditional anti-inflammatory: a study of acute toxicity in adult and embryos zebrafish (Danio rerio). Pharmaceuticals (Basel). 12(4):175. doi:10.3390/ph12040175.
- Fuchs TC, Hewitt P. 2011. Biomarkers for drug-induced renal damage and nephrotoxicity—an overview for applied toxicology. Aaps J. 13(4):615–631. doi:10.1208/s12248-011-9301-x.
- Gu L, Kelm MA, Hammerstone JF, Zhang Z, Beecher G, Holden J, Haytowitz D, Prior RL. 2003. Liquid chromatographic/electrospray ionization mass spectrometric studies of proanthocyanidins in foods. J Mass Spectrom. 38(12):1272–1280. doi:10.1002/jms.541.
- Hosseinzadeh S, Jafarikukhdan A, Hosseini A, Armand R. 2015. The application of medicinal plants in traditional and modern medicine: a review of Thymus vulgaris. IJCM. 06(09):635–642. doi:10.4236/ijcm.2015.69084.
- Hyacienth BMS, Tavares Picanço KR, Sánchez-Ortiz BL, Barros Silva L, Matias Pereira AC, Machado Góes LD, Sousa Borges R, Cardoso Ataíde R, dos Santos CBR, Carvalho HO, et al. 2020. Hydroethanolic extract from Endopleura uchi (Huber) Cuatrecasas and its marker bergenin: toxicological and pharmacokinetic studies in silico and in vivo on zebrafish. Toxicol Rep. 7:217–232. doi:10.1016/j.toxrep.2020.01.011.
- Ismail HF, Hashim Z, Soon WT, Rahman NSA, Zainudin AN, Majid FAA. 2017. Comparative study of herbal plants on the phenolic and flavonoid content, antioxidant activities and toxicity on cells and zebrafish embryo. J Tradit Complement Med. 7(4):452–465. doi:10.1016/j.jtcme.2016.12.006.
- Jaiswal R, Jayasinghe L, Kuhnert N. 2012. Identification and characterization of proanthocyanidins of 16 members of the Rhododendron genus (Ericaceae) by tandem LC-MS. J Mass Spectrom. 47(4):502–515. doi:10.1002/jms.2954.
- Kane A, Salierno J, Brewer S. 2005. Fish models in behavioral toxicology: automated techniques, updates and perspectives. In: Methods in Aquatic Toxicology. Vol. 2. Boca Raton: Lewis; p. 559–590.
- Kitipaspallop W, Sillapaprayoon S, Taepavarapruk P, Chanchao C, Pimtong W. 2021. Evaluation of developmental and transcriptional effects of α-mangostin on zebrafish embryos. Toxicol Environ Chem. 103(3):254–268. doi:10.1080/02772248.2021.1960349.
- Koga RCR, Santos A, Sarquis SFRR, Carvalho JCT. 2021. Bauhinia guianensis Aubl., a plant from Amazon biome with promising biologically active properties: a systematic review. PHREV. 15(29):76–81. doi:10.5530/phrev.2021.15.9.
- Leary S, Anthony R, Cartner S, Corey D, Grandin T, Greenacre C, Gwaltney-Brant S, Mccrackin M, Meyer R, Miller D. 2013. AVMA Guidelines for the Euthanasia of Animals [Internet]. 13th ed. [place unknown]; [accessed 2021 Dec 15]. https://www.avma.org/sites/default/files/2020-01/2020-Euthanasia-Final-1-17-20.pdf
- Little E, Fairchild J, Delonay A. 1993. Behavioral methods for assessing impacts of contaminants on early life stage fishes. Bethesda: American Fisheries Society.
- Li Chunting, Seeram Navindra. 2018. Ultra-fast liquid chromatography coupled with electrospray ionization time-of- flight mass spectrometry for the rapid phenolic profiling of red maple (Acer rubrum) leaves. J Sep Sci 41. doi: 10.1002/jssc.201800037.
- Madureira TV, Rocha MJ, Cruzeiro C, Rodrigues I, Monteiro RAF, Rocha E. 2012. The toxicity potential of pharmaceuticals found in the Douro River estuary (Portugal): evaluation of impacts on fish liver, by histopathology, stereology, vitellogenin and CYP1A immunohistochemistry, after sub-acute exposures of the zebrafish model. Environ Toxicol Pharmacol. 34(1):34–45. doi:10.1016/j.etap.2012.02.007.
- Makarova K, Siudem P, Zawada K, Kurkowiak J. 2016. Screening of toxic effects of bisphenol A and oroducts of its degradation: zebrafish (Danio rerio) embryo test and molecular docking. Zebrafish. 13(5):466–474. doi:10.1089/zeb.2016.1261.
- Mandrell D, Truong L, Jephson C, Sarker MR, Moore A, Lang C, Simonich MT, Tanguay RL. 2012. Automated zebrafish chorion removal and single embryo placement. J Lab Autom. 17(1):66–74. doi:10.1177/2211068211432197.
- Marconato E, Rodrigues M, Carvalho J, Viana E, Almeida S, Santos L, Sarti S. 1998. Atividade anti-inflamatória de extratos brutos de Bauhinia guianensis Aubl. In: XV Simpósio de Plantas Medicinais Do Brasil. Florianópolis, SC, Brazil; p. 60–61.
- Melo N, Sánchez-Ortiz BL, dos Santos Sampaio TI, Matias Pereira AC, Pinheiro da Silva Neto FL, Ribeiro da Silva H, Alves Soares Cruz R, Keita H, Soares Pereira AM, Tavares Carvalho JC. 2019. Anxiolytic and antidepressant effects of the hydroethanolic extract from the leaves of Aloysia polystachya (Griseb.) Moldenke: a study on zebrafish (Danio rerio). Pharmaceuticals (Basel). 12(3):106. doi:10.3390/ph12030106.
- Moraes T de M, Rodrigues CM, Kushima H, Bauab TM, Villegas W, Pellizzon CH, Brito ARMS, Hiruma-Lima CA. 2008. Hancornia speciosa: indications of gastroprotective, healing and anti-Helicobacter pylori actions. J Ethnopharmacol. 120(2):161–168. doi:10.1016/j.jep.2008.08.001.
- Muñoz V, Sauvain M, Bourdy G, Callapa J, Bergeron S, Rojas I, Bravo JA, Balderrama L, Ortiz B, Gimenez A, et al. 2000. A search for natural bioactive compounds in Bolivia through a multidisciplinary approach. J Ethnopharmacol. 69(2):127–137. doi:10.1016/S0378-8741(99)00148-8.
- OECD. 2002. Test No. 423: acute oral toxicity - acute toxic class method, OECD guidelines for the testing of chemicals, Section 4. Paris: OECD Publishing. doi:10.1787/9789264071001-en.
- OECD. 2013. Test No. 236: fish embryo acute toxicity (FET) test, OECD guidelines for the testing of chemicals, Section 2. Paris: OECD Publishing. doi:10.1787/a13f80ee-en.
- OECD. 2022. Test No. 425: acute oral toxicity: up-and-down procedure, OECD guidelines for the testing of chemicals, Section 4. Paris: OECD Publishing. doi:10.1787/9789264071049-en.
- Ou K, Gu L. 2014. Absorption and metabolism of proanthocyanidins. J Funct Foods. 7:43–53. doi:10.1016/j.jff.2013.08.004.
- Pinheiro EAA, Carvalho JM, dos Santos DCP, Feitosa AdO, Marinho PSB, Guilhon GMSP, de Souza ADL, da Silva FMA, Marinho AMdR 2013. Antibacterial activity of alkaloids produced by endophytic fungus Aspergillus sp. EJC08 isolated from medical plant Bauhinia guianensis. Nat Prod Res. 27(18):1633–1638. doi:10.1080/14786419.2012.750316.
- Poleksic V, Mitrovic-Tutundzic V. 1994. Fish gills as a monitor of sublethal and chronic effects of pollution. In Sublethal and chronic effects of pollutants on freshwater fish. Cambridge: fishing New Books; p. 339–352.
- Quintans-Júnior L, Almeida R, Falcão A, Agra MF, Sousa MFV, Barbosa-Filho JM. 2002. Avaliação da Atividade anticonvulsivante de plantas do Nordeste Brasileiro. Acta Farmacêutica Bonaerense. 21(3):179–184. [accessed 2022 Nov 29]. http://www.latamjpharm.org/trabajos/21/3/LAJOP_21_3_1_4_QAON0AZ8CR.pdf.
- Rates SMK. 2001. Plants as source of drugs. Toxicon. 39(5):603–613. doi:10.1016/S0041-0101(00)00154-9.
- Rigolin-Sá O. 1998. Toxicidade do herbicida Roundup (glifosato) e do acaricida Omite (propargito) nas fases iniciais da ontogenia do bagre. Rhandiahilarii (Valenciennes, 1840) (Pimelodidade, Siluriformes) [Doutorado em Recusos Naturais]. São Carlos: Universidade Federal de São Carlos.
- Roberts R, Ellis A. 2001. The anatomy and physiology of teleosts. In Fish Pathology. 3rd ed. Philadelphia: W. B. Saunders; p. 12–54.
- Rubinstein AL. 2006. Zebrafish assays for drug toxicity screening. Expert Opin Drug Metab Toxicol. 2(2):231–240. doi:10.1517/17425255.2.2.231.
- Russo E, Scicchitano F, Whalley BJ, Mazzitello C, Ciriaco M, Esposito S, Patanè M, Upton R, Pugliese M, Chimirri S, et al. 2014. Hypericum perforatum: pharmacokinetic, mechanism of action, tolerability, and clinical drug-drug interactions. Phytother Res. 28(5):643–655. doi:10.1002/ptr.505.
- Ryu B, Kim C-Y, Oh H, Kim U, Kim J, Jung C-R, Lee B-H, Lee S, Chang S-N, Lee JM, et al. 2018. Development of an alternative zebrafish model for drug-induced intestinal toxicity. J Appl Toxicol. 38(2):259–273. doi:10.1002/jat.3520.
- Saldanha L, Vilegas W, Dokkedal A. 2013. Characterization of flavonoids and phenolic acids in Myrcia bella Cambess. Using FIA-ESI-IT-MSn and HPLC-PAD-ESI-IT-MS combined with NMR. Molecules. 18(7):8402–8416. doi:10.3390/molecules18078402.
- Sampaio TI, Melo NC, Paiva BTF, Aleluia GAS, Silva Neto FLP, Silva HR, Keita H, Cruz RAS, Sánchez-Ortiz BL, Pineda-Peña EA, et al. 2018. Leaves of Spondias mombin L. a traditional anxiolytic and antidepressant: pharmacological evaluation on zebrafish (Danio rerio). J Ethnopharmacol. 224:563–578. doi:10.1016/j.jep.2018.05.037.
- Santos I, Souza G, Santana G, Duarte J, Fernandes C, Keita H, Velázquez-Moyado J, Navarrete A, Ferreira I, Carvalho H, et al. 2018. Histopathology in zebrafish (Danio rerio) to evaluate the toxicity of medicine: an anti-inflammatory phytomedicine with janaguba milk (Himatanthus drasticus Plumel). In Histopathology-An update. Wiley Blackwell: Hoboken; p. 39–64.
- Sarmah R, Kanta Bhagabati S, Dutta R, Nath D, Pokhrel H, Mudoi LP, Sarmah N, Sarma J, Ahmed AM, Jyoti Nath R, et al. 2020. Toxicity of a synthetic phenolic antioxidant, butyl hydroxytoluene (BHT), in vertebrate model zebrafish embryo (Danio rerio). Aquac Res. 51(9):3839–3846. doi:10.1111/are.14732.
- Sarnoski PJ, Boyer RR, O’Keefe SF. 2012. Application of proanthocyanidins from peanut skins as a natural yeast inhibitory agent. J Food Sci. 77(4):M242–9. doi:10.1111/j.1750-3841.2012.02652.x.
- Souza G, Duarte J, Fernandes C, Moyado J, Navarrete A, Carvalho J. 2016. Obtainment and study of the toxicity of perillyl alcohol nanoemulsion on zebrafish (Danio rerio). J Nanomed Res 4(4):00093. doi:10.15406/jnmr.2016.04.00093.
- Souza G, Silva IDR, Viana MD, Costa de Melo N, Sánchez-Ortiz B, Oliveira MMB, Barbosa WR, Ferreira IM, Carvalho JCT. 2019. Acute toxicity of the hydroethanolic extract of the flowers of Acmella oleracea L. in aebrafish (Danio rerio): Behavioral and histopathological studies. Pharmaceuticals. 12(4):173. doi:10.3390/ph12040173.
- Souza L. 2012. Guia da biodiversidade de fabaceae do Alto Rio Negro [Internet]. Manaus: Projeto Fronteira; [accessed 2021 Dec 14]. https://repositorio.inpa.gov.br/handle/ 1/36096.
- Takashima F, Hibiya T. 1995. An atlas of fish histology – normal and pathological features. Tóquio: Kodansha Ltda.
- Tala V, Silva VC, Rodrigues C, Nkengfack A, Campaner dos Santos L, Vilegas W. 2013. Characterization of proanthocyanidins from Parkia biglobosa (Jacq.) G. Don. (Fabaceae) by flow injection analysis—electrospray ionization ion trap tandem mass spectrometry and liquid chromatography/electrospray ionization mass spectrometry. Molecules. 18(3):2803–2820. doi:10.3390/molecules18032803.
- Teame T, Zhang Z, Ran C, Zhang H, Yang Y, Ding Q, Xie M, Gao C, Ye Y, Duan M, et al. 2019. The use of zebrafish (Danio rerio) as biomedical models. Anim Front. 9(3):68–77. doi:10.1093/af/vfz020.
- Teixidó E, Piqué E, Gómez-Catalán J, Llobet JM. 2013. Assessment of developmental delay in the zebrafish embryo teratogenicity assay. Toxicol In Vitro. 27(1):469–478. doi:10.1016/j.tiv.2012.07.010.
- Tomazi R, Figueira ÂC, Ferreira AM, Ferreira DQ, de Souza GC, de Souza Pinheiro WB, Pinheiro Neto JR, da Silva GA, de Lima HB, da Silva Hage-Melim LI, et al. 2021. Hypoglycemic activity of aqueous extract of latex from Hancornia speciosa Gomes: a study in zebrafish and in silico. Pharmaceuticals (Basel). 14(9):856. doi:10.3390/ph14090856.
- Vallverdú-Queralt A, Boix N, Piqué E, Gómez-Catalan J, Medina-Remon A, Sasot G, Mercader-Martí M, Llobet JM, Lamuela-Raventos RM. 2015. Identification of phenolic compounds in red wine extract samples and zebrafish embryos by HPLC-ESI-LTQ-Orbitrap-MS. Food Chem. 181:146–151. doi:10.1016/j.foodchem.2015.02.098.
- Viana EP, Santa-Rosa RS, Almeida SSMS, Santos LS. 1999. Constituents of the stem bark of Bauhinia guianensis. Fitoterapia. 70(1):111–112. doi:10.1016/S0367-326X(98)00032-X.
- von Hellfeld R, Brotzmann K, Baumann L, Strecker R, Braunbeck T. 2020. Adverse effects in the fish embryo acute toxicity (FET) test: a catalogue of unspecific morphological changes versus more specific effects in zebrafish (Danio rerio) embryos. Environ Sci Eur. 32(1):122. doi:10.1186/s12302-020-00398-3.
- Wang Y, Fong SK, Singh AP, Vorsa N, Johnson-Cicalese J. 2019. Variation of anthocyanins, proanthocyanidins, flavonols, and organic acids in cultivated and wild diploid blueberry species. horts. 54(3):576–585. doi:10.21273/HORTSCI13491-18.
- Wang Y, Vorsa N, Harrington P de B, Chen P. 2018. Nontargeted metabolomic study on variation of phenolics in different cranberry cultivars using UPLC-IM – HRMS. J Agric Food Chem. 66(46):12206–12216. doi:10.1021/acs.jafc.8b05029.
- Werner M, Costa MJ, Mitchell LG, Nayar R. 1995. Nephrotoxicity of xenobiotics. Clin Chim Acta. 237(1-2):107–154. doi:10.1016/0009-8981(95)06068-O.
- Wiersema J. 2019. Bauhinia guianensis Aubl. GRIN Taxonomy. GRIN taxonomy. US National Plant Germplasm System. [accessed 2020 Jul 17]. https://www.gbif.org/pt/species/101356924. doi:10.15468/ao14pp.
- Williams CH, Hong CC. 2011. Multi-step usage of in vivo models during rational drug design and discovery. Int J Mol Sci. 12(4):2262–2274. doi:10.3390/ijms12042262.