Abstract
Context
In China, HUANGQI is widely used for the treatment of Alzheimer’s disease (AD). However, a comprehensive understanding of its mechanism of anti-AD effects is lacking.
Objective
To explore the active ingredients of HUANGQI and its potential targets and mechanisms of action in AD.
Materials and methods
The active ingredients and targets of HUANGQI were screened from databases (TCSMP, ETCM, and BATMan), and AD-related genes were obtained from DrugBank and GeneCards. The same target genes were screened, and a drug-target disease network was constructed. The PPI network was constructed and GO and KEGG pathway enrichment analyses of the targets. The Cell Counting Kit-8 (CCK-8) assay was used to determine suitable HUANGQI treatment concentrations for HT-22 cells between 0-480 μg/mL. CCK-8, FITC-phalloidin and propidium iodide (PI) assays were used to examine the protective effect of (0, 60, 120, 240 μg/mL) of HUANGQI on 20 μM Aβ1-42-induced HT-22 cell cytotoxicity.
Results
Twelve active ingredients of HUANGQI were selected, with 679 common targets associated with AD. GO and KEGG analysis revealed that the therapeutic mechanisms of HUANGQI involve TNF, AGE, the NF-κB pathway, and nuclear receptor activity-related processes. The CCK-8 assay indicated that HUANGQI was not cytotoxic to HT-22 cells at concentrations less than 240 μg/mL and was able to attenuate Aβ1-42-induced cellular damage (EC50 = 83.46 μg/mL). FITC-phalloidin and PI assays suggested that HUANGQI could alleviate 20 μM Aβ1-42-induced neuronal cell cytotoxicity in a dose-dependent manner.
Conclusion
HUANGQI has a protective effect on Aβ1-42-induced nerve cell injury; further mechanism research was needed.
Introduction
Alzheimer’s disease (AD) is a multifaceted neurodegenerative disease (Jiang et al. Citation2017) characterized by progressive memory impairment, impaired cognitive function, behavioral changes, and aberrant behavior (Eckert Citation2010); this disease is age-dependent (Sun et al. Citation2019). As our societies age, the number of people living with dementia across the world is expected to rise from 55 million in 2019 to 139 million in 2050, according to the World Health Organization (WHO) (Long et al. Citation2023). Except for the two monoclonal antibody drugs Aducanumab and Lencanezumab approved by FDA for injection against Alzheimer’s disease in recent years (Yang, Citation2024), there are only five FDA-approved oral drugs for the treatment of AD, namely, tacri
ne, donepezil, levovastatin, galantamine, and melizine (Bhardwaj et al. Citation2017). Indeed, these current oral drug treatments only treat AD symptoms, and treatments that target the underlying mechanisms of the disease are not yet available. Therefore, there is an urgent need to develop new drugs for treating Alzheimer’s disease. Traditional Chinese medicine can treat multiple pathways and multiple targets in diseases and has unique advantages in the treatment of complex neurodegenerative diseases.
HUANGQI also known as Astragalus, is the heel of Astragalus mongolicus or Astragalus membranaceus in the Fabaceae family, which has a medicinal history of more than 2000 years in China. It functions to nourish qi, strengthen the spleen and stomach, discharge pus and relieve pain, promote blood circulation and treat danger (Liu et al. Citation2019). According to the records of the ‘Shennong Classic of Materia Medica’ of the Han Dynasty, HUANGQI can treat gale and leprosy, making it a top-grade Chinese medicine. In medical books such as the ‘Compendium of Materia Medica’ of the Ming Dynasty, HUANGQI also has the reputation of increasing the efficacy of other medicines (Xiang et al. Citation2016). Buyang Huanwu decoction, proposed by Wang Qingren in the Qing Dynasty in his book ‘Correcting Mistakes in the Medical Forest’, has been used to treat dementia caused by qi deficiency and blood stasis (Gao et al. Citation2018). HUANGQI is included in this prescription, and modern research has shown that the Buyang Huanwu decoction can maintain the normal morphological structure of neurons in Alzheimer’s disease model mice, increase the indices of the brain and spleen, and reduce β-amyloid (Aβ) levels (Fei et al. Citation2014). In recent years, with the application of modern biomedical methods, substantial progress has been made in elucidating the mechanisms by which HUANGQI treats central nervous system injury and degeneration (Zhou et al. Citation2018). Total HUANGQI glycosides alleviate dexamethasone- and Aβ-induced neuronal loss and impair learning and memory in Alzheimer’s disease model rats (Li Citation2010). In addition, calycosin, which is an active ingredient of HUANGQI, can inhibit the activity of the oxidative stress markers malondialdehyde and glutathione in the brain tissue of Alzheimer’s disease model mice, prevent oxidative stress damage, and protect neurocognitive function (Gao Citation2017). However, as a traditional Chinese medicine, HUANGQI has many active components and a wide range of action targets, and the pathogenesis of Alzheimer’s disease is complex. Therefore, it is difficult to elucidate the mechanism through which HUANGQI exerts an anti-AD effect using traditional pharmacological methods due to its complex composition and multitarget features. It is necessary to find a method that can be used to comprehensively study these various components and targets.
Network pharmacology is a newly emerging discipline in which modern bioinformatic methods are utilized to mine, analyze, and organize big data to elucidate drug actions and interactions with multiple targets. In addition to analyzing diseases, the targets and interaction networks of drugs that act on diseases can be identified. Here, the possible mechanism of action of HUANGQI in the treatment of Alzheimer’s disease was explored through network pharmacology. The active compounds and active targets of HUANGQI were identified and analyzed to provide new insights and a theoretical basis for further research on the pharmacological mechanism of HUANGQI in the treatment of Alzheimer’s disease.
Materials and methods
Materials and reagents
HT-22 cells (RRID: CVCL-0321) were purchased from Shanghai Zhongqiao Xinzhou Biotechnology Co., LTD (Shanghai, China), catalogue number: ZQ0476. Aβ1–42 [amyloid β peptide (1–42), rat, J0426A] was purchased from Dalian Meilun Biotechnology Co., Ltd. (Dalian, China). FITC-phalloidin was obtained from Sigma–Aldrich LLC. (Shanghai, China). A propidium iodide test kit (including DAPI) was purchased from Beyotime Biotechnology Co., Ltd. (Shanghai, China). A Cell Counting Kit-8 (CCK-8) was obtained from Bimake (Houston, TX, USA). HUANGQI was purchased from Chongqing Tongjunge Co., Ltd. (Chongqing, China).
Network pharmacology
Screening the active compounds and targets of HUANGQI
The active compounds of HUANGQI and their putative target proteins were obtained from multiple databases, including the Traditional Chinese Medicine Systems Pharmacology database (TCMSP, https://tcmspw.com/tcmsp.php) (Ru et al. Citation2014), the Encyclopedia of Traditional Chinese Medicine (ETCM, http://www.tcmip.cn/ETCM/index.php/Home/) (Xu et al. Citation2019) and Bioinformatics Analysis Tool for Molecular MechANism of Traditional Chinese Medicine (BATMAN-TCM, http://bionet.ncpsb.org.cn/batman-tcm/) (Liu et al. Citation2016) databases. The relevant parameters of compound kinetics provided by the database were used to screen for suitable compounds. OB indicates the oral bioavailability of compounds, and compounds with an OB greater than 30% were screened according to recommendations provided by the database; DL indicates druglikeness, that is, the similarity of the properties between the compound and existing drugs. Similarly, we used the database’s recommendation to select compounds with DLs greater than 0.18. The target compounds retrieved from the TCMSP were identified as proteins, so we standardized them by using the UniProt Knowledgebase database (UniProt KB, http://www.uniprot.org/help/uniprotkb) (Bateman et al. Citation2023).
Screening the targets of AD
The therapeutic targets of AD were also obtained from multiple databases. The sequences used were from the DrugBank database (https://go.drugbank.com/) (Wishart et al. Citation2018), the Online Mendelian Inheritance in Man (OMIM- https://omim.org/) (Amberger et al. Citation2015) and the GeneCards (https://www.genecards.org/) (Safran et al. Citation2010) databases. The common targets of HUANGQI and AD were thought to be the potential targets for HUANGQI in the treatment of AD.
Protein–protein interaction (PPI) network
The obtained intersection targets were analyzed using STRING (http://string-db.org) (Szklarczyk et al. Citation2023). The relationship between protein interactions was obtained by choosing targets with a combined score >0.4.
Compound-target network construction
Cytoscape 3.7.0 was used to visualize the HUANGQI ingredient - target - AD interaction network.
Screening of core targets
The HUANGQI-AD shared targets were imported into the STRING database. To ensure credibility, a combined score > 0.4 was selected to establish the intersection protein interaction network. Topological analysis of the protein interaction network was conducted using Cytoscape software to screen the core active ingredients and targets of HUANGQI in the treatment of AD.
GO and KEGG analysis
GO and KEGG analyses of the target genes were performed using an online platform for data analysis and visualization (https://www.bioinformatics.com.cn, last accessed on 10 July 2023), p < 0.05 was selected. The top ten enriched BP (biological process), CC (cellular component), and MF (molecular function) GO terms and KEGG pathways are displayed.
Molecular docking
The top 10 core targets and core active ingredients according to the Compound-Target-Alzheimer’s disease map and the degree value were screened for molecular docking. The species was defined as Homo sapiens in the UniProt database. After removing proteins with low resolution, the protein data were input into the RCSB PDB database (https://www.rcsb.org/) (Fermi et al. Citation1984), and the corresponding 3D gene protein structures were downloaded (pdb format). Simple processing of target proteins included removed water molecules and heteroatoms and docking small-molecule ligands. After hydrogenation and processing of the protein using AGFR-1.2, the central atom of the protein was located and set as the active pocket. The English name of the core active ingredient was subsequently input into the PubChem database (https://pubchem.ncbi.nlm.nih.gov/) to download its corresponding ligand 3D structure (sdf format), which was subsequently converted to the pdb format through PyMOL software. Molecular docking of the core targets and core active components was carried out by using the Sailvina package of AutoDock 4.2.6 software, and PyMOL software was used to visualize the docking results.
Molecular simulation
Gromacs software was used to perform a 100 ns molecular dynamics simulation of the above molecular docking results while analyzing the binding energy of the complex. The target protein first needed to be modified with a specific format of hydrogen, and AMBER99SB-ILDN was used to parameterize atoms and set the water molecular type force field parameters. The active ingredient ligand small molecule constructs were configured in Gauss View, and the electrostatic potential was calculated. Amber parameters and coordinate files of the ligand small molecule are generated through Amber Tools, and Acpype generates a topology file of the GAFF force field of the ligand small molecule. Subsequently, the following molecular dynamic simulation process was carried out: energy minimization, NVT preequilibrium, NPT preequilibrium, and MD simulation.
Preparation of the HUANGQI sample
HUANGQI samples were obtained after 1.5 h of extraction with 10-fold (w/v) 70% ethanol (v/v) reflux twice in a thermostatic water bath at 70 °C. The two parts of the extract were combined, and the solvent was removed by rotary evaporation to obtain dry Astragalus powder. The HUANGQI dry powder was stored in a refrigerator at −20 °C before use.
Cell viability assay
We seeded HT-22 cells in 96-well microtiter plates at a density of approximately 5 × 103 cells per well. The cells were incubated in fresh complete culture medium containing HUANGQI (0-480 μg/mL) once for 48 h, then we used a CCK-8 assay to determine HT-22 cell viability (Bao et al. Citation2021). HUNAGQI (0 μg/mL) was as the blank control group. The absorbance was measured at 450 nm by a SynergyH1 microplate reader (BioTek) according to the manufacturer’s instructions. Similarly, cellular damage induced by different concentrations of HUANGQI (0, 15, 30, 60, 120, 240, or 480 μg/mL) in combination with 20 μM Aβ1-42 oligomers was measured via a CCK-8 assay.
FITC-phalloidin assay
The cytoskeleton of HT-22 cells was stained with FITC-phalloidin (Li et al. Citation2022). We plated HT-22 cells in 24-well plates and cultured them for 3 days in culture medium supplemented with RA. After the cells were pretreated with different concentrations (0, 60, 120, 240 μg/mL) of HUANGQI for 2 h (0 μg/mL was as the blank control group.), 20 μM Aβ1-42 oligomers were added. Forty-eight hours later, the cells were fixed with 4% (wt/vol) paraformaldehyde for 15 min and sequentially incubated with a FITC-phalloidin solution and DAPI at 37 °C. Finally, images were taken under a fluorescence microscope at 200 × magnification.
Propidium iodide (PI) assay
The cytotoxicity of Aβ1-42 oligomers on HT-22 cells and the protective effect of HUANGQI were detected via a propidium iodide (PI) assay (Li et al. Citation2022). HT-22 cells were seeded in 24-well plates at a density of approximately 1 × 104 cells per well. The next day, HT-22 cells were pretreated with fresh complete medium containing different concentrations (0, 60, 120, 240 μg/mL) of HUANGQI or solvent for 2 h and incubated with Aβ1-42 oligomers at 37 °C, while the control group was treated with solvent only. Forty-eight hours later, the culture medium was removed, and the cells were washed once with 1 × PBS and stained with a propidium iodide detection kit obtained from Beyotime Biotechnology. Images were taken with a fluorescence microscope at 100× magnification.
Statistical analysis
All the data are presented as the mean ± standard deviation and were analyzed by using two-tailed Student’s t-tests or one-way ANOVA with Tukey’s multiple comparison tests with GraphPad Prism 8.0.1 software. p < 0.05 was considered to indicate statistical significance. At least three independent experiments were included in each statistical analysis.
Results
Active ingredients and targets of HUANGQI
Through OB > 30% and DL > 0.18 screening, a total of 76 active compounds in HUANGQI were obtained, including 17 from the TCMSP, 23 from the ETCM and 36 from the BATMAN database (). The list of 76 active compounds were shown in . A total of 1275 corresponding targets were obtained, and the duplicated proteins were removed to obtain the common targets of 1091 HUANGQI active ingredients. There were 8233, 15 and 7 AD-related genes in the GeneCard, OMIM and DrugBank databases, respectively. After removing duplicate genes, 8235 genes were obtained. The targets of the active components of HUANGQI and AD-related genes were compared, and 679 shared targets were obtained, corresponding to 12 active compounds of HUANGQI ().
Figure 2. Venn diagram. (a) Compounds from 3 databases. (b) Shared targets of HUANGQI and Alzheimer’s disease.
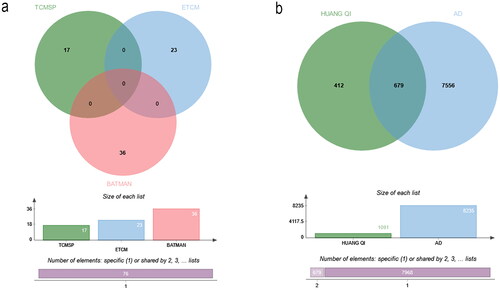
Table 1. The List of 76 active compounds.
The PPI network
The interaction network between compounds and targets was obtained by STRING. The independent targets were removed, and those with a relationship with a degree greater than 0.4 were selected to construct the PPI network. There were 676 nodes and 13760 lines in . The thicker the lines were, the greater the degree value was and the closer the connections between the targets were. The PPI network contained 3 clusters, namely, Cluster 1, Cluster 2 and Cluster 3 (). Due to the large amount of data, to facilitate subsequent analysis, we screened a total of 14 targets with degree values greater than or equal to 40 for inclusion ().
HUANGQI – ingredients – targets - AD interaction network diagram
Using the visualization function of Cytoscape 3.7.0, the interaction network of HUANGQI, active ingredients, targets, and AD was constructed. The target points were the first 100 intersection targets (). Each node represents a target or ingredient, the edges between different nodes represent an interaction relationship with each other, and the degree value represents the number of connected nodes in the network. A total of 146 nodes were identified, with a total of 466 edges, reflecting the characteristics of HUANGQI in treating AD through its multiple targets, multiple components, and collaborative use. Network topology analysis was visualized and performed through Cytoscape 3.7.0, including betweenness centrality, closeness centrality, and degree parameters. Among the 76 active ingredients, the highest ranked by degree was quercetin (DC = 41), followed by dioctyl phthalate (DC = 18) (). The specific data are shown in . The top 10 targets included AR, RXRA, ESR1, HSP90AA1, IL1B, PPARG, CALM1, NOS2, IL6, and HDAC2, suggesting that these factors may be the key core active components and targets of HUANGQI in the treatment of AD (); the detailed data can be found in and .
Figure 4. (a) Network diagram of HUANGQI-component-target-AD. (b) Degree values of the main active ingredients in HUANGQI. (c) Degree values of the core intersection targets.
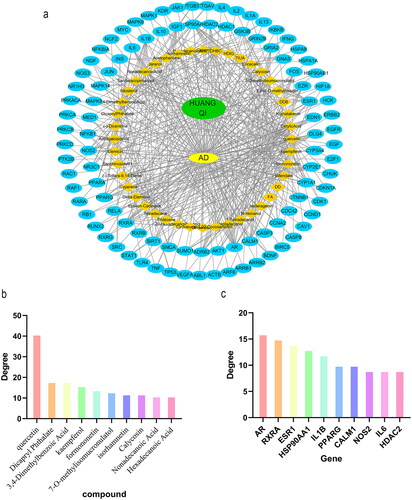
Table 2. Information on the main active ingredients of HUANGQI.
Table 3. Topological parameters of the core targets of HUANGQI for AD treatment.
GO and KEGG enrichment analysis of common targets
Cellular component analysis
There were 251 enriched GO cellular component terms. The top 10 enriched cellular component terms included the succinate dehydrogenase complex, respiratory chain complex II, succinate dehydrogenase complex, fumarate reductase complex, fumarate reductase complex, mitochondrial respiratory chain complex II, oxoglutarate dehydrogenase complex, α-amino-3-hydroxy-5-methyl-4-isoxazolepropionic acid-selective glutamate receptor complex, lateral loop, flotillin complex and interphase microtubule organizing center ().
Biological process analysis
There were 2943 enriched GO biological process terms. The top enriched 10 biological processes included negative regulation of cholesterol storage, mammary gland branching involved in pregnancy, fat-soluble vitamin biosynthetic process, negative regulation of receptor biosynthetic process, 9-cis-retinoic acid metabolic process, vitamin A biosynthetic process, smooth muscle adaptation, negative regulation of mast cell activation and regulation of heterotypic cell-cell adhesion ().
Molecular function analysis
There were 658 enriched GO molecular function terms. The top 10 enriched molecular function terms were dopamine binding, monoamine transmembrane transporter activity, retinoic acid receptor activity, G-protein coupled acetylcholine receptor activity, DNA-methyltransferase activity, dopamine receptor activity, N-methyl-d-aspartate selective glutamate receptor activity, muscarinic acetylcholine receptor activity, succinate dehydrogenase activity, and epinephrine binding ().
KEGG pathway enrichment analysis revealed 313 enriched pathways. The top 10 enriched pathways are shown in . The pathways included lipid and atherosclerosis, amphetamine addiction, neuroactive ligand–receptor interaction, fluid shear stress and atherosclerosis, the cAMP signaling pathway, the AGE-RAGE signaling pathway in diabetic complications, nicotine addiction, chemical carcinogenesis-receptor activation, the calcium signaling pathway and nonalcoholic fatty liver disease.
Molecular docking
The core active ingredients and 10 core targets of the ‘HUANGQI active ingredient target AD’ interaction network was selected according to the highest degree values (AR, RXRA, ESR1, HSP90AA1, IL1B, PPARG, CALM1, NOS2, IL6, and HDAC2), after which molecular docking was conducted. The binding energy information of the docking results is shown in the heatmap (). It is generally believed that the lower the active binding energy between the active component ligand and the target receptor is, the more stable the conformation and the greater the possibility of its occurrence. According to the binding energy ≤ − 5.0 kJ/mol as the standard (Zhang et al. Citation2023), the results showed that the binding energy of each docking combination was less than −5.0 kJ/mol, indicating that the core active components of HUANGQI have suitable binding activity with the core target for treating AD. In addition, through docking binding energy and literature review, HDAC2 and IL6 were selected for molecular docking with kaempferol and quercetin (). The IDs of HDAC2 and IL6 in the RCSB PDB database are 7zzp and 4CNI, respectively. The results showed that kaempferol and quercetin mainly interacted with residues Arg60, Lys170, and Lys63 of HDAC2 through hydrogen bonds and interacted with residues His525, Gln726, and Thr685 of IL6 through hydrogen bonds. Furthermore, we identified the original ligand for the IL6 protein as 6-cyclopropyl-3-[(pyrimidin-5-yl) amino]-N-[1-(2,2,2-trifluoroethyl)-1H-pyrazol-3-yl] pyridine-2-carboxamide and the original ligand for HDAC2 as 6-methyl-4-oxidanyl-pyran-2-one using the RCSB PDB database. Subsequently, we downloaded the original ligand structures of the IL6 and HDAC2 proteins from the PubChem database and performed docking simulations with the respective ligand molecules. The docking data are shown in . IL6 interacts with kaempferol and quercetin via the His525, Gln726, and Thr685 residues through hydrogen bonding and interacts with the original ligand (6-cyclopropyl-3-[(pyrimidin-5-yl) amino]-N-[1-(2,2,2-trifluoroethyl)-1H-pyrazol-3-yl] pyridine-2-carboxamide) via the His525 and Tyr524 residues through hydrogen bonding. HDAC2 interacts with kaempferol and quercetin via hydrogen bonding to the Arg60, Lys170, and Lys63 residues and interacts with the original ligand (6-methyl-4-oxidanyl-pyran-2-one) via the His62, Tyr19, and Lys63 residues through hydrogen bonds. These results indicate that IL6 and HDAC2 interact with kaempferol, quercetin, and the original ligands through the same amino acid residues.
Figure 7. Heatmap of the binding energies of the core active ingredient and the core target docking site.
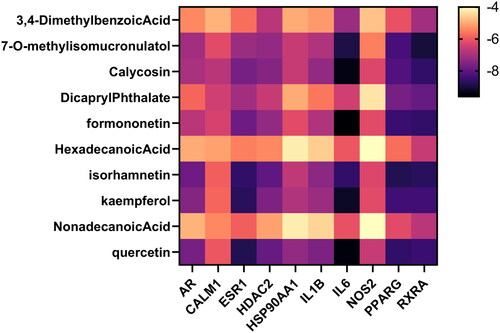
Figure 8. Molecular docking data of the core active components and targets of HUANGQI. (a) HDAC2 docking with kaempferol, quercetin and its original ligand. (b) IL6 docking with kaempferol, quercetin and its original ligand.
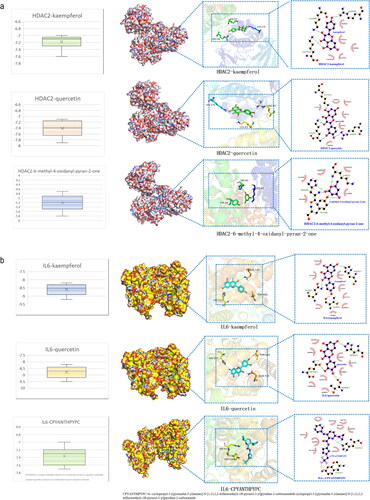
A comparison of the binding affinities revealed that the binding affinities of IL6 and HDAC2 for the original ligands were greater than those for kaempferol and quercetin. These findings confirmed that the conformations of the IL6 and HDAC2 complexes with kaempferol and quercetin were stable.
Molecular simulation
According to the literature, molecular dynamics simulations can provide a basis for determining the stability of protein–ligand complexes. Using molecular docking data, this study conducted 100 ns simulation analyses of HDAC, IL6, and HDAC2-kaempferol; HDAC2-quercetin; and the IL6-kaempferol and IL6-quercetin complexes to evaluate molecular motion, trajectory, structural characteristics, binding potential, and conformational changes, respectively. The changes in the RMSD of the complex were analyzed, and the smaller the value was, the better the conformational stability of the structure was. As shown in , according to the RMSD value of the protein, HDAC2 was stable in the early and late stages, with only slight fluctuations in the middle, while IL6 was stable throughout each stage. The RMSD of HDAC2 relative to its original ligand (6-methyl-4-oxidanyl-pyran-2-one, HDAC2-kaempferol and HDAC2-quercetin) fluctuated significantly in the early stage compared to the late stage and stabilized after 50 ns, with a range of less than 0.3 nm. Comparing the simulated trajectories of IL6 protein, IL6 with its original ligan (6-cyclopropyl-3-[(pyrimidin-5-yl) amino]-N-[1-(2,2,2-trifluoroethyl)-1H-pyrazol-3-yl] pyridine-2-carboxamide, and IL6-kaempferol and IL6-quercetin complexes, it was found that the IL6 with its original ligand and IL6-kaempferol complex was more stable than the IL6-quercetin complex, and the fluctuation amplitude was less than 0.2 nm.
In conclusion, the RMSD results showed that after binding the original ligand, kaempferol or quercetin to the proteins HDAC2 and IL6, temperature or pressure had no significant impact on the structure of the complex, and the conformation was relatively stable. The RMSF data were used to analyze the changes in the main binding amino acid residues of the original ligand, kaempferol and quercetin after binding to HDAC2 and IL6. The results showed that after binding the original ligand, kaempferol or quercetin, to HDAC2 and IL6, the RMSF values of some residues changed, indicating that the original ligand, kaempferol or quercetin affected them (). Therefore, these results strongly support the effectiveness of the molecular docking results. The MMPBSA binding free energy was calculated using molecular dynamics simulation trajectories. The results indicate that the binding energies of the complexes formed by the IL6 and HDAC2 proteins with their original ligands, as well as those of kaempferol and quercetin, were less than −5 kJ/mol (1 kcal/mol = 4.184 kJ/mol). This finding further confirmed the binding stability of IL6 and HDAC2 proteins with their original ligands, kaempferol, and quercetin.
Figure 9. Molecular simulation of the core active components and targets of HUANGQI. (a) The RMSD of HDAC2 and IL6 for different core active components and their original ligands. (b) RMSF of HDAC2 and IL6 for different core active components and their original ligands. c the binding free energies of HDAC2 and IL6 to different core active components and their original ligands. Blue is the binding energy obtained from molecular docking; orange is the binding free energy calculated by molecular dynamics simulation and the MM-PBSA method.
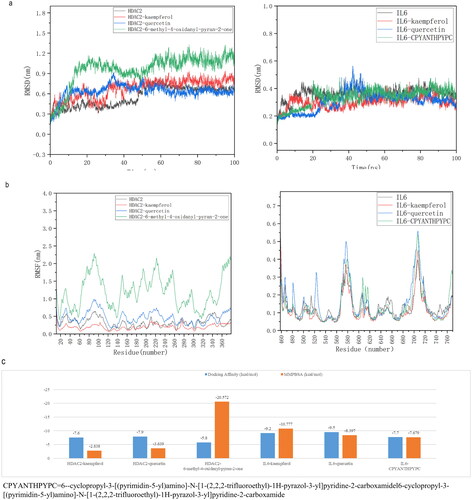
HUANGQI attenuated the cytotoxicity induced by the Aβ1–42 oligomer in HT-22 cells
We found that the dose of Aβ1-42 oligomers required to construct AD cell models was 20 μM (Jiao et al. Citation2015; Ding et al. Citation2020). As shown in , the CCK-8 assay indicated that HUANGQI was not cytotoxic to HT-22 cells at concentrations less than 240 μg/mL. Furthermore, Aβ1-42 oligomers significantly reduced the viability of HT-22 cells, which was blocked by HUANGQI in a dose-dependent manner, and the EC50 was 83.46 μg/mL ().
Figure 10. HUANGQI ameliorates Aβ1-42-induced neuronal cell cytotoxicity (a, b) A CCK-8 assay was used to test the viability of HT-22 cells. (c) FITC-phalloidin assay showing the cytoskeleton of HT-22 cells in different groups. (d) PI indicates the number of dead HT-22 cells. The results are shown as the mean ± SD. ###p < 0.001, **p < 0.01, *p < 0.05 compared with the control group (n = 3).
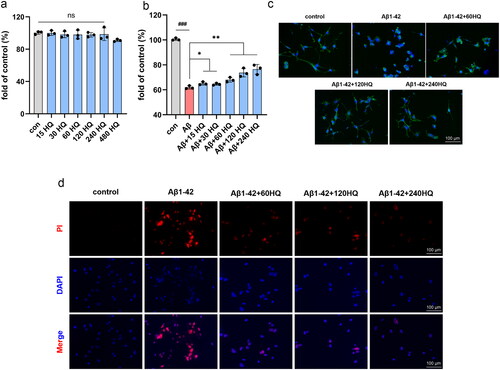
The cell cytoskeleton was subsequently stained with FITC-phalloidin. The axons of HT-22 cells treated with the Aβ1-42 oligomer were considerably shorter than those of control cells, while different doses of HUANGQI markedly reversed these effects (). As shown in , the death of HT-22 cells was induced by 20 μM Aβ1-42 oligomers, while the number of dead cells decreased significantly after treatment with different concentrations of HUANGQI. Taken together, these results suggest that HUANGQI treatment can significantly reduce the cytotoxicity of Aβ1-42 oligomers in HT-22 cells and maintain the morphology of neuronal cells.
Discussion
As a new discipline, network pharmacology uses publicly available datasets to predict the pharmacological effects of drugs, which saves the time and cost of scientific researchers, especially in terms of pathway screening. It is a powerful tool for pharmacological researchers. In this study, network pharmacology methods were used to screen and predict the active ingredients of HUANGQI and analyzed the potential mechanism of HUANGQI in treating Alzheimer’s disease. A total of 12 active ingredients of HUANGQI and 130 corresponding targets were obtained, including 41 targets related to Alzheimer’s disease. The C-T-AD map shows that even a single active ingredient can act on multiple targets, which fully demonstrates that HUANGQI can exert anti-AD effects through multiple pathways and targets. According to the prediction results for the active ingredients and targets, isorhamnetin, 7-O-methylisopropanol, calycosin, and kaempferol targeted multiple factors. Studies have shown that isorhamnetin, sorghum isoflavone, and kaempferol can all inhibit inflammatory responses (Zhao et al. Citation2018; Takashima et al. Citation2019; Li et al. Citation2020; Zaki et al. Citation2021). Sorghum isoflavone and kaempferol also have suitable antioxidant stress and neuroprotective effects (Ding et al. Citation2018; Takashima et al. Citation2019).
To further explore the mechanism of HUANGQI in treating Alzheimer’s disease, we conducted protein interaction analysis and KEGG and GO analyses on the predicted targets. The results showed that the anti-AD mechanism of HUANGQI may involve regulating apoptosis, the inflammatory response, and oxidative stress. A large number of studies have confirmed that apoptosis, the inflammatory response, and oxidative stress are closely related to the pathogenesis of Alzheimer’s disease (Liang et al. Citation2017; Lapresa et al. Citation2019). The pathway enrichment and GO analysis of HUANGQI targets indicated that the mechanism by which it treats Alzheimer’s disease is related mainly to the activity of various receptors, such as nuclear receptors, steroid hormone receptors, G-protein coupled receptors, and neurotransmitter receptors. The activity of these receptors is directly or indirectly involved in the body’s inflammatory and oxidative stress responses and these receptors play a role in the TNF, AGE, and NF-κB signaling pathways. According to the PPI analysis, Caspase-3 and MAPK8 play key roles in the network, indicating that HUANGQI may exert anti-AD effects by affecting the activity or expression of Caspase-3 and MAPK8. Caspase-3 plays an irreplaceable role in cell apoptosis, while MAPK is one of the common intersection pathways of signal transduction pathways involved in processes such as cell proliferation, stress, inflammation, differentiation, functional synchronization, transformation, and apoptosis and plays an important role in the regulation of various diseases, such as cardiovascular and cerebrovascular diseases (Du et al. Citation2019). MAPK8 is a member of the MAPK family and exerts anti-inflammatory, antioxidant, and apoptotic effects (Liu et al. Citation2019). In addition, through molecular docking, we confirmed that the core active ingredients of HUANGQI have suitable binding activity with the core targets for treating AD.
Molecular dynamics simulations further confirmed that the binding of HDAC2 and IL6 to kaempferol and quercetin was stable after reaction in solution. IL-6 is an important cytokine that participates in the pathogenesis of many inflammatory diseases, including AD (Erta et al. Citation2012). The IL-6 C allele polymorphism reduces Alzheimer’s disease risk (Faltraco et al. Citation2003). Furthermore, a recent study revealed a significant association between IL-6 and elevated amyloid burden as well as subsequent deposition in individuals exhibiting diminished hippocampal volume (Oberlin et al. Citation2021). Previous studies have shown that IL-6 is associated with cognitive dysfunction and psychiatric disorders (Licastro et al. Citation2003; Brosseron et al. Citation2023). Mahady et al. reported that HDAC2 protein levels were dysregulated in the basal forebrain region, which contains cholinergic neurons of the nucleus basalis of Meynert, in AD patients. These authors suggested that HDAC2 dysregulation contributes to cholinergic neuron dysfunction in the Meynert nucleus basalis, neurofibrillary tangle pathology, and cognitive decline during the clinical progression of AD (Mahady et al. Citation2019). The histone deacetylase HDAC2 negatively regulates synaptic gene expression and neuronal plasticity and is upregulated in AD patients and mouse models. Targeted HDAC2 therapy may improve AD-related cognitive impairment (Yamakawa et al. Citation2017).
To preliminarily verify the therapeutic effect of HUANGQI on Alzheimer’s disease, we first examined the toxicity of HUANGQI on mouse hippocampal neurons (HT-22) to determine the concentration at which HUANGQI is toxic. Aβ is the most prominent risk factor for Alzheimer’s disease. Aβ1-42 is a readily aggregated peptide produced by the hydrolysis of APP proteins and is associated with the neuropathology of early-onset familial AD and early-onset familial AD (Ling and Salvaterra Citation2011). In our study, HUANGQI reversed the Aβ1-42-induced reduction in HT-22 cell viability and cell death in a dose-dependent manner.
Conclusions
This study used network pharmacology methods to systematically analyze HUANGQI, identify its potential targets of action, preliminarily elucidate its mechanism of action in treating Alzheimer’s disease, and verify that HUANGQI has a protective effect on Aβ1-42-induced nerve cell injury. This approach is highly important for comprehensively exploring the mechanism of HUANGQI in treating Alzheimer’s disease. However, the results of this study were obtained only through network prediction analysis and initial tests, and further validation of the analysis results through experimentation is needed.
Authors’ contributions
WJ Li and XR Zheng coordinated and directed the project. F Lv and M Sun performed network pharmacology and molecular docking. CM Qin and D Du collated data and performed the in vitro experiments. All authors were involved in the writing of the article. All authors read and agreed to the published version of the manuscript.
Disclosure statement
The authors declare no conflict of interest, financial or otherwise.
Additional information
Funding
References
- Amberger JS, Bocchini CA, Schiettecatte F, Scott AF, Hamosh A. 2015. OMIM.org: Oonline Mendelian Inheritance in Man (OMIM®), an online catalog of human genes and genetic disorders. Nucleic Acids Res. 43(Database issue):D789–798. doi: 10.1093/nar/gku1205.
- Bao K, Li Y, Wei J, Li R, Yang J, Shi J, Li B, Zhu J, Mao F, Jia R, et al. 2021. Fangchinoline suppresses conjunctival melanoma by directly binding FUBP2 and inhibiting the homologous recombination pathway. Cell Death Dis. 12(4):380. doi: 10.1038/s41419-021-03653-4.
- Bateman A, Martin M-J, Orchard S, Magrane M, Ahmad S, Alpi E, Bowler-Barnett EH, Britto R, Bye-A-Jee H, The UniProt Consortium., et al. 2023. UniProt: the universal protein knowledgebase in 2023. Nucleic Acids Res. 51(D1):D523–D531. doi: 10.1093/nar/gkac1052.
- Bhardwaj D, Mitra C, Narasimhulu CA, Riad A, Doomra M, Parthasarathy S. 2017. Alzheimer’s disease-current status and future directions. J Med Food. 20(12):1141–1151. doi: 10.1089/jmf.2017.0093.
- Brosseron F, Maass A, Kleineidam L, Ravichandran KA, Kolbe CC, Wolfsgruber S, Santarelli F, Häsler LM, McManus R, Ising C, et al. 2023. Serum IL-6, sAXL, and YKL-40 as systemic correlates of reduced brain structure and function in Alzheimer’s disease: results from the DELCODE study. Alzheimers Res Ther. 15(1):13. doi: 10.1186/s13195-022-01118-0.
- Ding B, Lin C, Liu Q, He Y, Ruganzu JB, Jin H, Peng X, Ji S, Ma Y, Yang W. 2020. Tanshinone IIA attenuates neuroinflammation via inhibiting RAGE/NF-κB signaling pathway in vivo and in vitro. J Neuroinflammation. 17(1):302. doi: 10.1186/s12974-020-01981-4.
- Ding L, Zhang GY, Gao LN, Xuan ZB, Li YF, Lian XY. 2018. Protective effect and mechanism of callein isoflavone on hippocampal neuron injury induced by H2O2. Guangdong Chem Ind. 45:66–65. [Chinese].
- Du W, Hu H, Zhang J, Bao G, Chen R, Quan R. 2019. The mechanism of MAPK signal transduction pathway involved with electroacupuncture treatment for different diseases. Evid Based Complement Alternative Med. 2019:1–10. doi: 10.1155/2019/8138017.
- Eckert GP. 2010. Traditional used plants against cognitive decline and Alzheimer disease. Front Pharmacol. 2010 Dec 8; 1:138. doi: 10.3389/fphar.2010.00138.
- Erta M, Quintana A, Hidalgo J. 2012. Interleukin-6, a major cytokine in the central nervous system. Int J Biol Sci. 8(9):1254–1266. doi: 10.7150/ijbs.4679.
- Faltraco F, Bürger K, Zill P, Teipel SJ, Möller H, Hampel H, Bondy B, Ackenheil M. 2003. Interleukin‐6-174 G/C promoter gene polymorphism C allele reduces Alzheimer’s disease risk. J Am Geriatr Soc. 51(4):578–579. doi: 10.1046/j.1532-5415.2003.51177.x.
- Fei HX, Han YS, Du H, Zhong LL, Li BL, Pu CY, Zhang YB, Liao T, Bai Y, Jiang B, et al. 2014. Effects of bu Yang Huan Wu decoction on hippocampal morphology and amyloid beta in mice with Alzheimer’s disease. Chin J Exp Formulas Chin Med. 20:142–145. (Chinese)
- Fermi G, Perutz MF, Shaanan B, Fourme R. 1984. The crystal structure of human deoxyhaemoglobin at 1.74 A resolution. J Mol Biol. 175(2):159–174. doi: 10.1016/0022-2836(84)90472-8.
- Gao J. 2017. Mechanism of calydrin isoflavone alleviating cognitive impairment in APP/PS1 mice via PKC/Nrf2 pathway [dissertation]. Ji Lin: Ji Lin University. [ Chinese ].
- Gao LL, Li Z, Zhou HY, Ye SY, Liu RC, Zang HC. 2018. Application and research status of bu yang huan wu decoction. Food Drug. 20:400–404. [Chinese].
- Jiang Y, Gao H, Turdu G. 2017. Traditional Chinese medicinal herbs as potential AChE inhibitors for anti-Alzheimer’s disease: A a review. Bioorg Chem. 75:50–61. doi: 10.1016/j.bioorg.2017.09.004.
- Jiao S-S, Yao X-Q, Liu Y-H, Wang Q-H, Zeng F, Lu J-J, Liu J, Zhu C, Shen L-L, Liu C-H, et al. 2015. Edaravone alleviates Alzheimer’s disease-type pathologies and cognitive deficits. Proc Natl Acad Sci U S A. 112(16):5225–5230. doi: 10.1073/pnas.1422998112.
- Lapresa R, Agulla J, Sánchez-Morán I, Zamarreño R, Prieto E, Bolaños JP, Almeida A. 2019. Amyloid-ß promotes neurotoxicity by Cdk5-induced p53 stabilization. Neuropharmacology. 146:19–27. doi: 10.1016/j.neuropharm.2018.11.019.
- Li G, Wang G, Wang S, Sun M, Wen Z. 2020. Isorhamnetin attenuates Streptococcus suis virulence by inhibiting the inflammatory response. Antonie Van Leeuwenhoek. 113(2):303–310. doi: 10.1007/s10482-019-01338-9.
- Li L, Li W-J, Zheng X-R, Liu Q-L, Du Q, Lai Y-J, Liu S-Q. 2022. Eriodictyol ameliorates cognitive dysfunction in APP/PS1 mice by inhibiting ferroptosis via vitamin D receptor-mediated Nrf2 activation. Mol Med. 28(1):11. doi: 10.1186/s10020-022-00442-3.
- Li WZ. 2010. Study on the protective effect of total Astragalus glycosides on the synergistic induction of glucocorticoid and β-amyloid protein in neuronal injury and its mechanism [dissertation]. An Hui: An Hui Medical University. [Chinese].
- Liang YX, Cao GQ, Zhang WS. 2017. [ Research progress of inflammation reaction and intervention of traditional Chinese medicine in Alzheimer’s disease. ]Chin Pharmacol Bull. 33:597–602. [Chinese].
- Licastro F, Grimaldi LME, Bonafè M, Martina C, Olivieri F, Cavallone L, Giovanietti S, Masliah E, Franceschi C. 2003. Interleukin-6 gene alleles affect the risk of Alzheimer’s disease and levels of the cytokine in blood and brain. Neurobiol Aging. 24(7):921–926. doi: 10.1016/s0197-4580(03)00013-7.
- Ling D, Salvaterra PM. 2011. Brain aging and Aβ1-42 neurotoxicity converge via deterioration in autophagy-lysosomal system: a conditional Drosophila model linking Alzheimer’s neurodegeneration with aging. Acta Neuropathol. 121(2):183–191. doi: 10.1007/s00401-010-0772-0.
- Liu Y, Yang W, Sun X, Xie L, Yang Y, Sang M, Jiao R. 2019. SS31 Ameliorates sepsis-induced heart injury by inhibiting oxidative stress and inflammation. Inflammation. 42(6):2170–2180. doi: 10.1007/s10753-019-01081-3.
- Liu YD, Cai XL, Zhang WA. 2019. Study on pharmacological effect and clinical application of Astragalus. J Electrocardiography. 8:37–39. (Electronic edition) [Chinese].
- Liu Z, Guo F, Wang Y, Li C, Zhang X, Li H, Diao L, Gu J, Wang W, Li D, et al. 2016. BATMAN-TCM: a bioinformatics analysis tool for molecular mechANism of Traditional Chinese Medicine. Sci Rep. 6(1):21146. doi: 10.1038/srep21146.
- Long S, Benoist C, Weidner W. 2023. World Alzheimer Report 2023: Rreducing dementia risk: never too early, never too late. [Internet]. London, England: AaAlzheimer’s Disease International. [cited 2024 Apr 22]. Available from: https://www.alzint.org/resource/world-alzheimer-report-2023/.
- Mahady L, Nadeem M, Malek-Ahmadi M, Chen K, Perez SE, Mufson EJ. 2019. HDAC2 dysregulation in the nucleus basalis of Meynert during the progression of Alzheimer’s disease. Neuropathol Appl Neurobiol. 45(4):380–397. doi: 10.1111/nan.12518.
- Oberlin LE, Erickson KI, Mackey R, Klunk WE, Aizenstein H, Lopresti BJ, Kuller LH, Lopez OL, Snitz BE. 2021. Peripheral inflammatory biomarkers predict the deposition and progression of amyloid-β in cognitively unimpaired older adults. Brain Behav Immun. 95:178–189. doi: 10.1016/j.bbi.2021.03.015.
- Ru J, Li P, Wang J, Zhou W, Li B, Huang C, Li PD, Guo Z, Tao W, Yang Y, et al. 2014. TCMSP: a database of systems pharmacology for drug discovery from herbal medicines. J Cheminform. 6(1):13. doi: 10.1186/1758-2946-6-13.
- Safran M, Dalah I, Alexander J, Rosen N, Iny Stein T, Shmoish M, Nativ N, Bahir I, Doniger T, Krug H, et al. 2010. GeneCards Version 3: the human gene integrator. Database (Oxford). 2010(0):baq020–baq020. doi: 10.1093/database/baq020.
- Sun Y, Wang Y, Guo Z, Du K, Meng D. 2019. Systems pharmacological approach to investigate the mechanism of Ohwia caudata for application to Alzheimer’s disease. Mol. 24(8):1499. doi: 10.3390/molecules24081499.
- Szklarczyk D, Kirsch R, Koutrouli M, Nastou K, Mehryary F, Hachilif R, Gable AL, Fang T, Doncheva NT, Pyysalo S, et al. 2023. The STRING database in 2023: protein-protein association networks and functional enrichment analyses for any sequenced genome of interest. Nucleic Acids Res. 51(D1):D638–D646. doi: 10.1093/nar/gkac1000.
- Takashima M, Ichihara K, Hirata Y. 2019. Neuroprotective effects of Brazilian green propolis on oxytosis/ferroptosis in mouse hippocampal HT22 cells. Food Chem Toxicol. 132:110669. doi: 10.1016/j.fct.2019.110669.
- Wishart DS, Feunang YD, Guo AC, Lo EJ, Marcu A, Grant JR, Sajed T, Johnson D, Li C, Sayeeda Z, et al. 2018. DrugBank 5.0: a major update to the DrugBank database for 2018. Nucleic Acids Res. 46(D1):D1074–D1082. doi: 10.1093/nar/gkx1037.
- Xiang YX, Xiao LY, Zhang J, Huang Zb Hu MH, Ma Fl, Li HF. 2016. [ Mechanism of the effect of Astragalus polysaccharides treatment of diseases of the nervous system and its research progress. ]Chin J Hospital Pharm. 4:687–691. [Chinese].
- Xu HY, Zhang YQ, Liu ZM, Chen T, Lv CY, Tang SH, Zhang XB, Zhang W, Li ZY, Zhou RR, et al. 2019. ETCM: an encyclopaedia of traditional Chinese medicine. Nucleic Acids Res. 47(D1):D976–D982. doi: 10.1093/nar/gky987.
- Yamakawa H, Cheng J, Penney J, Gao F, Rueda R, Wang J, Yamakawa S, Kritskiy O, Gjoneska E, Tsai L-H. 2017. The transcription factor Sp3 cooperates with HDAC2 to regulate synaptic function and plasticity in neurons. Cell Rep. 20(6):1319–1334. doi: 10.1016/j.celrep.2017.07.044.
- Yang LC. 2024. Recent advancements in ppathogenesis, early intervention and treatment of Alzheimer’s disease. Prog Bio Eng. 45(01):14–20. [Chinese].
- Zaki AA, Xu X, Wang Y, Shie P-H, Qiu L. 2021. A new anti-inflammatory flavonoid glycoside from Tetraena aegyptia. Nat Prod Res. 35(12):1985–1990. doi: 10.1080/14786419.2019.1650356.
- Zhang YC, Gao WC, Chen WJ, Pang DX, Mo DY, Yang M. 2023. Network pharmacology and molecular docking analysis on molecular targets and mechanisms of Fei Jin Sheng formula in the treatment of lung cancer. Curr Pharm Des. 29(14):1121–1134. doi: 10.2174/1381612829666230503164755.
- Zhao HP, Li Xp Luo Jj Peng LP, Lv X, Chen D, Song L. 2018. Effects of litmus isoflavones on PM2.5 mediated inflammation and its mechanism. Chin J Veterinary Med. 38:2147–2152. [Chinese].
- Zhou LY, Song Z, Zhou LW, Qiu Y, Hu N, Hu Y, Hu X. 2018. Protective role of Astragalus injection in spinal cord ischemia-reperfusion injury in rats. Neurosciences (Riyadh). 23(2):116–121. doi: 10.17712/nsj.2018.4.20170391.