Abstract
Objective. In this study we tested the hypothesis that α-2 adrenergic antagonism could facilitate induction of previously non-inducible ventricular tachycardia (VT) during acute ischemia. Previous reports suggest that VT during ischemia may be modulated by α-2 adrenergic agonists. Design. The left anterior descending artery was occluded after instrumentation of the ischemic risk zone with 21 multipolar plunge needles, each recording 6 bipolar electrograms. Three dimensional mapping characterized the mechanism of VT induced with extrastimuli. Results. Of 16 non-inducible dogs included, eight which were given the α-2 adrenergic antagonist yohimbine all had inducible VT, while all eight in the control group remained non-inducible (p <0.05). Six of the VTs were of focal Purkinje origin. The cycle length of the VT was 119±4 ms. Mean arterial pressure (81±8 to 82±8 mmHg, p =ns), ventricular effective refractory period (146±6 to 144±5 ms, p =ns) and ischemic zone size (55±6% vs. 61±4%, p =0.45) were not altered by yohimbine indicating minimal central or pre-junctional effects of the drug. Conclusions. Yohimbine facilitates induction of VT, especially those with focal Purkinje fiber origin, suggestive of an effect mediated through antagonism of post-junctional α-2 adrenoceptors on Purkinje fibers.
Ventricular tachycardia (VT) is a well known consequence of acute ischemia of the myocardium. Different mechanisms may underlie this arrhythmia and both VT with reentrant mechanisms and VT with a focal origin have been demonstrated during the period of early ischemia Citation1–3. It has recently been described that the cardiac Purkinje system may be an important source of VTs with a focal origin occurring during acute ischemia and after reperfusion of the myocardium in a canine model Citation4–6.
Until recently the heart was thought to be devoid of post-junctional α-2 adrenoceptors. However, there is now convincing evidence that α-2 adrenergic receptors exist on canine Purkinje fibers Citation6–10. Stimulation of these receptors results in prolongation of both the Purkinje action potential duration and the Purkinje relative refractory period Citation7, Citation8. In isolated Purkinje fibers, induction of sustained triggered activity by β adrenergic stimulation and rapid pacing was prevented when concomitant α-2 and β adrenergic stimulation was administered along with rapid pacing Citation8. In an intact animal model, α-2 adrenergic agonists prevented induction of previously inducible VT of Purkinje origin during acute ischemia Citation6. This indicates that stimulation of these α-2 adrenoceptors may have antiarrhythmic effects on VT with a Purkinje fibers origin.
In this study we tested the hypothesis that administration of the α-2 adrenergic antagonist yohimbine might facilitate induction of VT of Purkinje origin in an intact canine model in those cases where no VT could be induced in the first and second hour after coronary artery occlusion.
Methods
Animal preparation
Large adult mongrel dogs (18–24 kg) of either gender were used for this study. The protocol was approved by the University of Iowa Animal Use and Care Committee and conformed to the Guide for the care and use of laboratory animals, published by the National Institutes of Health (NIH publication No. 85–23, revised 1996). The animals were anesthetized with sodium thiopental 250 mg and alpha-chloralose 100–200 mg/kg intravenously as a bolus. A continuous intravenous infusion of α-chloralose dissolved in polyethylene glycol at 8 mg/kg/hr was used for anesthesia maintenance. The animals were intubated and ventilated on a volume controlled ventilator and tidal volume was adjusted to maintain an arterial PCO2 within physiologic range (25–35 torr). Positive end expiratory pressure was applied to maintain PO2 in normal range (80–150 torr) utilizing oxygen enriched air. Sodium bicarbonate was infused as necessary to maintain the pH within normal range (7.30–7.45). Arterial pressure was continuously monitored via an arterial line. The femoral vein was accessed for administration of drugs and saline.
The heart was exposed through a median sternotomy. The left anterior descending coronary artery (LAD) was dissected proximally and a snare placed around the vessel. The site of the dissection was chosen to obtain as large an ischemic zone as possible without involving the first septal perforator. The sternal wound was covered by plastic sheet and an infrared heating lamp was directed to the wound area in order to maintain intrathoracic temperature at around 37°C, while monitored by an intrathoracic thermometer. After the experiments, the animals were sacrificed by induction of ventricular fibrillation.
Electrophysiological measurements
A bipolar electrode was used to pace the right atrium at two times diastolic threshold with pulses of 2 ms duration and cycle length 300 ms. The region of the sinus node was destroyed permanently in order to control the rate. Surface electrocardiographic leads II and V5R were continuously monitored and the limb leads (I, II, III, aVR, aVL, aVF) along with V5R were recorded. Twenty-one multipolar plunge needles were inserted into and surrounding the anticipated risk zone for ischemia after LAD occlusion. Each needle recorded 6 bipolar electrograms from circumferential electrodes 1 mm apart enabling recordings from a total of 126 sites. The electrodes were made from teflon insulated tungsten wire. The bipoles were usually recorded on consecutive electrodes so that the distance between bipoles was 1 mm, although on rare instances was the intraelectrode bipole spacing 2 or 3 mm.
Most of the needles were inserted into the myocardium perpendicular to the epicardial surface except electrodes 1 through 5 which were inserted diagonally into the septum and passing into the left ventricular cavity and electrodes 19, 20 and 21 which were inserted at an angle to involve the septum in addition to the anterior wall of the right ventricle Citation4. Spacing between needles was 10 mm although this could vary by a few mm between experiments because of differences in the dogs’ coronary anatomy. The computer template for the activation maps was adjusted accordingly for each experiment.
Ventricular pacing for VT induction was from the innermost pole of each of the 16 pole needle placed in three locations (apex, septum, lateral wall) outside of the risk zone of the coronary artery occlusion.
Electrograms were recorded simultaneously on two separate computer systems, one for the three bipoles closest to the endocardium and the other for the three bipoles closest to the epicardium. The three electrodes closest to the endocardium were recorded on a custom built system consisting of a 33 MHz 80486 computer (Gateway, Sioux Falls, ND, USA) coupled with a DAP 2400/6 (Microstar Laboratories, Bellevue, WA, USA) high speed acquisition board, an analogue signal multiplexer and 64 independent amplifier circuits. Transmural signals were amplified by a gain of 100, bandpass filtered between 3 and 1300 Hz and digitized with a 12-bit AD converter at 3.2 kHz. The epicardial electrograms were recorded using commercial system (Bard Electrophysiology, Billerica, MA, USA) on a computer (Compaq, Houston TX, USA) with a 30 megabyte hard disc, 40 K base memory and an 80287 math processor. The commercial system allowed for recordings of 64 channels at 12 bit resolution with a sampling frequency of 1 kHz/channel and bandpass filtering from 30–300 Hz. Pre-sampling of the data allowed for acquisition of electrograms for up to 4 s before an event with both systems. Three-dimensional activation maps were constructed from multiplexed signals of up to 14 s using data from both acquisition systems.
Each needle (22 mm with electrodes covering the proximal 16 mm of the needle shaft) had 16 unipolar electrodes, which were used to select the 6 optimal bipolar electrograms which were chosen to maximize the capability to record Purkinje signals on the bipole closest to the endocardium. The bipole nearest to the epicardium recorded an electrogram from the epicardium and subsequent bipoles recorded electrograms sequentially through the myocardial wall. The bipole closest to the endocardium was used to record Purkinje potentials when they could be identified. Purkinje potentials were identified at their endocardial location according to previous published criteria from this laboratory, including 0.5 mV spikes lasting 1–2 ms preceding the larger and longer muscle spike and the surface QRS on the lead recording the earliest activity Citation4–6.
If a Purkinje potential was not identified for a given electrode no activation time was marked for the electrogram closest to the endocardium and this resulted in a blank on the activation map for this area. The activation maps were constructed in the following manner: A computer algorithm searched for the most rapid electrogram voltage change (dv/dt) over an interval of 20 ms prior to the surface QRS on the lead recording the earliest activity to 20 ms prior the onset of the next surface QRS complex from the same lead. The bipolar electrograms closest to the endocardium were not assigned muscle activation times as these were designated for Purkinje potentials. The Purkinje potentials were manually assigned activation times on these electrograms only when present. Activation maps were used to determine the site of origin of the VT complexes.
Definitions
VT was defined as three or more premature ventricular complexes in a row. VT was considered to be sustained if it lasted longer than 30 s, or either pace termination or defibrillation was required prior to that because of hemodynamic collapse. The cycle length of VTs was averaged over the first three complexes.
VT was designated to have a focal origin when no electrical activity could be recorded on all adjacent sites in three-dimensions between the latest activation of one QRS complex and the earliest of the next QRS. Moreover, conduction from the site of earliest activity to adjacent electrodes could not manifest conduction delay, which might account for a majority of the cycle length of the VT.
VT of Purkinje fiber origin was defined as a focal endocardial mechanism with recording of a Purkinje potential prior to the QRS on the electrogram recording the earliest activity. Purkinje potentials had to be identified on electrograms during atrial pacing before and after coronary occlusion and during VT in order to be considered mechanistically involved.
Mechanisms were defined as reentrant when the earliest activation site was located immediately adjacent to the site of the latest activation from the previous complex and continuous diastolic activation was recorded between complexes. Reentrant mechanisms also demonstrated unidirectional and functional block to the subsequent earliest site of activation.
Ventricular effective refractory period was determined by delivering extrastimuli, via a programmable stimulator (Bloom, Reading, PA, USA), after eight paced complexes with effective refractory period defined as the longest interval between the drive pacing (S1) and the first extrastimulus (S2) that did not capture the ventricle. The drive CL was 300 ms. VT induction was attempted with up to four premature stimuli as follows: The first premature stimulus (S2) was fixed at 4 ms longer than the ventricular effective refractory period and a second stimulus (S3) was employed at the same coupling interval. The S3 was shortened in 10 ms decrements until either VT induction or failure to capture occurred. If no VT was induced the same procedure was followed for the third (S4) and fourth extrastimuli (S5), as required. There was a pause of 1 s before the next drive started.
Ischemia was defined as a reduction in voltage of electrograms after LAD occlusion of more than 45% from baseline (pre-LAD occlusion) for the endocardium and more than 55% reduction from baseline for the mid-myocardium and epicardium Citation11. Size of the ischemic zone in this study is expressed as the percentage of electrodes demonstrating the required drop in voltages at one hour after coronary artery occlusion compared to baseline (pre-intervention) and 30 minutes after intervention (yohimbine or saline) compared to baseline (post-intervention).
Study protocol
Only those animals which had no inducible VT after coronary artery occlusion were included. After instrumentation of the risk zone with 21 multipolar plunge needles and prior to coronary artery occlusion, induction of VT was attempted with extrastimuli to exclude artifactual VT due to the instrumentation alone. None of the animals had VT under these conditions. The LAD was then occluded and VT induction attempted using serial induction protocols using up to four extrastimuli. Pacing was from one of the three pacing electrodes placed outside the risk zone. If no VT was inducible after at least three attempts from each site, dogs were randomly chosen to receive either yohimbine (0.075 mg/kg) or saline. The dose of yohimbine was chosen based on assumptions of per body weight dosing extrapolated from humans. VT induction was then re-attempted. No spontaneous sustained VT or VF was observed during the period of 1–3 hours after coronary artery occlusion.
Statistics
All data are expressed as mean±SE. Fisher's exact test was used to test for differences in inducibility between the groups receiving the α-2 antagonist or saline. A Student's t test was used for comparison of refractory periods and mean arterial pressures before and after administration of yohimbine or saline. Student's t test was also used for comparison of the sizes of the ischemic zones before and after intervention. A p-value of <0.05 was considered significant.
Results
A total of 16 dogs which had no inducible VT with programmed extrastimuli in the 1–2 hour period after coronary artery occlusion were included in the study. They were randomly divided into two groups of eight, which received either yohimbine 0.075 mg/kg intravenously or saline. The mean number of Purkinje spike observations was 8.9±0.5 per experiment.
Of the eight dogs given yohimbine all had inducible VT after the drug administration (), while all animals in the group which received saline remained noninducible (p < 0.05). Six VTs were of focal Purkinje fiber origin, one had epicardial focal and the remaining one had a VT with a mechanism of epicardial reentry. An example of inducible VT of Purkinje fiber origin is shown in . There are Purkinje spikes preceding the earliest muscle activity on the intramural electrocardiogram taken from the site of earliest activity during the first VT complex. shows an example of an activation map of Purkinje VT. The earliest activity is in the Purkinje layer and spreads out from this focus to adjacent Purkinje fibers and overlying myocardium without significant conduction delay, suggesting a focal origin. shows another complex of focal Purinje VT but also demonstrates subsequent conduction delay within the epicardium, suggesting that if the epicardial map was viewed alone this might be suggestive of a reentrant mechanism.
Figure 1. Electrograms during a ventricular pacing protocol (last in the drive, S1 and two prematures, S2 S3) followed by 3 VT complexes (vertical lines indicate the onset of surface ECG of VT complexes). Shown are surface ECG tracing lead II, and electrograms from Purkinje (P) or overlying endocardial (E) recording sites at focus of origin (P-F) and from west (P-W), north (E-N), south (P-S), southwest (P-SW), and east (E-E) of the focus. During pacing Purkinje potentials are indicated by downward arrows; all Purkinje potentials show delay with S2 and S3 with the greatest delay seen in P-SW, which in being greater than the cycle length between S3 and VT1, could suggest reentry within the Purkinje network. This delay argues for Purkinje origin of the potentials since the expected refractory period of Purkinje system would be greater than overlying endocardium. A similar delay is recorded in P-S and both recordings also are delayed between VT1 and VT2 (upward arrows), also suggesting possible reentry within the Purkinje system. VT3 complex originates from a Purkinje focus (P-F, with no major delay from VT2). An alternative explanation is that all three VT complexes originate from a focus at P-F.
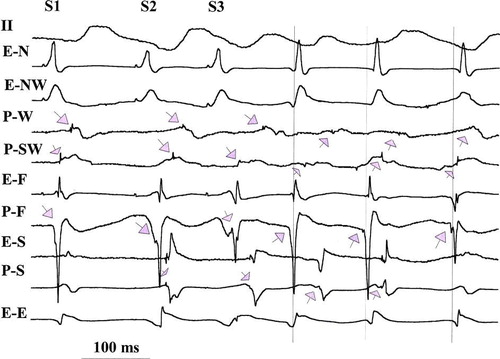
Figure 2. 3-D activation mapping of second VT complex shown in the electrograms in . Maps are shown in six layers each representing epicardial (EPI), subepicardial (S-EPI), midwall, subendocardial (S-ENDO), endocardial (ENDO) and Purkinje (PURK) planes. Activation times are in ms at each recording site. Maps are drawn in 20 ms isochrones (different colors, with pink the earliest, then yellow, red, sky blue, orange, green, lavender and dark blue representing the latest activation). Earliest activity is seen in the PURK layer (−28 ms) with activation then proceeding out to EPI. Activation of the Purkinje layer is slow with late activity (34 ms) recorded adjacent to the early, suggesting possible reentry in the Purkinje layer.
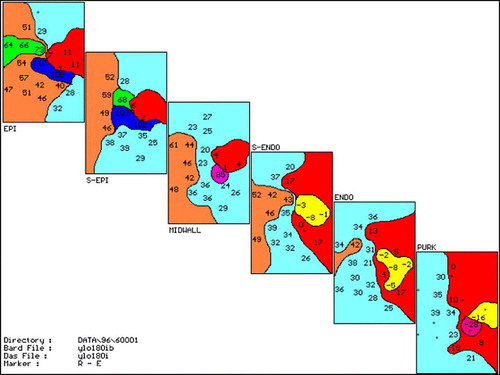
Figure 3. Same format as in . Map of third VT complex seen in the electrograms in . Note earliest Purkinje activation at −18 ms with much less delay to adjacent areas than , suggesting focal Purkinje origin of third VT complex. If viewed alone, the epicardium would suggest a reentrant excitation with early site at −11 ms next to late site 89 ms.
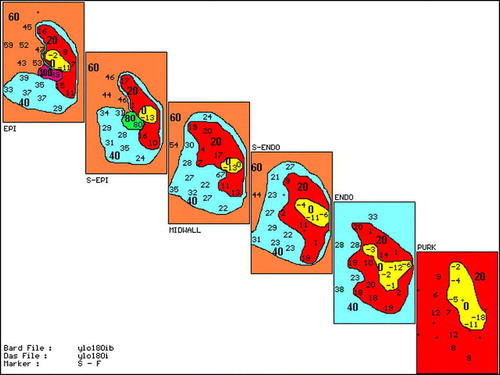
Table I. Characteristics and mechanism of VTs induced with yohimbine.
The mean cycle length of the VT was 119±4 ms. The ventricular effective refractory period did not change after yohimbine administration, 146±6 ms to 144±5 ms (p = 0.78), indicating no pre-junctional effects of the drug. Likewise, mean arterial pressure was not significantly altered by yohimbine, 81±8 mmHg to 82±8 mmHg (p = 0.48), suggesting minimal or no central effects of the drug. Yohimbine did not significantly affect the size of the ischemic zone (55±6% (pre-drug intervention) vs. 61±4% (post-drug intervention), p = 0.22).
The eight control dogs that got saline and no pharmacological intervention all remained non-inducible during repeated programmed extrastimulus testing. Neither ventricular effective refractory period, 149±6 ms to 146±6 ms (p = 0.36), mean arterial pressure, 109±9 mmHg to 107±10 mmHg (p = 0.44), nor size of ischemic zone (38±6% (pre-saline intervention) vs. 47±6% (post-saline intervention), p = 0.22) changed in this group.
Discussion
The main observation of this study was that administration of the α-2 antagonist yohimbine facilitated induction of previously non-inducible VT during acute ischemia in a canine model. The majority of VTs had a focal Pukinje fiber origin. The lack of effect of yohimbine on ventricular effective refractory period and mean arterial pressure suggests minimal or even absent central or pre-junctional effects of the drug. These effects of yohimbine might therefore be mediated via post-junctional α-2 adrenoceptors in canine Purkinje fibers.
We have previously described studies on inducible VT in a canine model in the 1 to 3 hour period following LAD occlusion Citation1, Citation6, Citation12. Although ischemia generally extends progressively to the epicardium, significant further increases in the ischemic size after the first hour of LAD occlusion in this model have not been observed. This suggests that epicardial extension was nearly complete at 1 hour Citation13.
The Purkinje or endocardial focus in vivo has been related to the incidence of delayed afterdepolarizations (DADs) and triggered activity (TA) observed in excised endocardial tissue studied from this same model Citation13. These data were strongly suggestive that DADs and TA may underlie a majority of focal VTs in ischemic endocardial tissue. Thus, ischemia and focal sites of VT seem specifically responsive to adrenergic stimuli. Although we did not study, in vitro, the foci of the VTs induced in this present study, they may be similar to those previously recorded Citation13.
The Purkinje system and VT
There has been growing interest recently in the role of the Purkinje system in the development of VT in humans Citation14, Citation15. Szumowski et al. Citation14 demonstrated that the Purkinje system has an important role in the mechanism of VT in patients after myocardial infarction. This VT was suppressed by radiofrequency ablation of the local Purkinje network Citation14. We had previously described a role for the Purkinje system during acute ischemia in a canine model Citation4. Haissaguerre et al. Citation15 have also described a potential role for the Purkinje system in the triggering of idiopathic ventricular fibrillation. Ventricular premature beats with a likely origin in the Purkinje system were frequently seen preceding runs of VT. The Purkinje potentials were subsequently used to guide ablative therapy.
In addition the Purkinje system plays an important role in patients with idiopathic left ventricular VT Citation16. A Purkinje potential is frequently demonstrated preceding the onset of the QRS complex, indicating that the Purkinje system may be a source of these VTs Citation17. This VT is treatable with radiofrequency ablation where the Purkinje potential is also used to target the ablation site.
Cardiac α-2 adrenoceptors
A number of studies in recent years have demonstrated that post-junctional α-2 adrenoceptors exist on canine Purkinje fibers Citation6–10. Stimulation of these receptors in vitro increases the Purkinje action potential duration and in an intact canine model α-2 adrenergic stimulation prolongs the Purkinje relative refractory period Citation7, Citation8. Both effects have been reversed by the α-2 adrenergic antagonist yohimbine. There were no effects seen with α2-adrenergic stimulation in ventricular muscle, which is in agreement with the observation of others that the myocardium is devoid of post-junctional α2-adrenoceptors.
α2-adrenoceptors in Purkinje tissue may have a role in counterbalancing the effects of β-adrenergic stimulation by inhibition of cAMP production and thereby counteracting arrhythmogenic effects of excess β-adrenergic activation. It has been suspected that cAMP may be an important mediator of arrhythmogenesis during ischemia and reperfusion Citation18. α2-adrenoceptor antagonism therefore might conceivably increase the possibility of ventricular arrhythmias under these same conditions. Indeed, in the present study, we found that yohimbine facilitated induction of VT, especially these with a Purkinje fiber origin under conditions of acute ischemia.
Yohimbine has occasionally been used in clinical medicine for erectile dysfunction Citation19. A single dose of the drug for that indication is similar to the dose used in this study. Yohimbine increases sympathetic outflow from the central nervous system (CNS) and can cause increased blood pressure and increased heart rate. It is a reasonable argument that enhanced sympathetic outflow from the CNS may have contributed to the development of VT seen after yohimbine. However, if such was the case, it would have been expected that VTs with equivalent Purkinje origin and an intramyocardial origin would have been seen. Mean arterial pressure did not change significantly with yohimbine, which suggests minimal enhancement in CNS sympathetic outflow with the dose of yohimbine used in this study. A lack of effect of the drug on ventricular effective refractory period additionally strongly suggests no pre-junctional effects of the drug.
Yohimbine may have partial α1-adrenergic antagonistic effects. This is unlikely to have played a role in the results of this study as α1-adrenergic effects during ischemia may be arrhythmogenic Citation20, Citation21.
While ablation therapy appears to be promising for VT of Purkinje fiber origin, modulation of this arrhythmia with treatment directed at the α-2 adrenoceptors on Purkinje fibers might provide a unique pharmacological target. Experimental data have shown this to be effective in both canine and rodent models where α-2 agonists prevented the induction of arrhythmias Citation6, Citation22. In another study in rats, a selective α-2 agonist, alpha-methyl-norepinephrine facilitated successful defibrillation from ventricular fibrillation, an effect which was abolished by yohimbine pre-treatment Citation23. Further studies will need to focus on effects of α-2 adrenenergic modulation of Purkinje fibers in humans.
In conclusion, these results strongly suggest that the α2-adrenergic antagonist yohimbine facilitates induction of VT of Purkinje fiber origin in a canine model during acute ischemia. This is additional confirmation that modulation of these receptors might play an important role in electrical stability under these conditions. α2-adrenergic receptors may be a potential pharmacologic target for therapy of ventricular arrhythmias of Purkinje fiber origin.
References
- Arnar DO, Xing D, Martins JB. Overdrive pacing of early ischemic ventricular tachycardia: Evidence for both reentry and triggered activity. Am J Physiol. 2005; 288: H1124–H1130
- Janse MJ, Van Capelle FJL, Morsink H, Kleber AG, Wilms-Schopman F, Cardinal R, et al. Flow of “injury” current and patterns of excitation during early ventricular arrhythmias in acute regional myocardial ischemia in isolated porcine and canine hearts. Evidence for two different arrhythmogenic mechanisms. Circ Res. 1980; 47: 151–65
- Pogwizd SM, Corr PB. Reentrant and nonreentrant mechanisms contribute to arrhythmogenesis during early myocardial ischemia. Results using three-dimensional mapping. Circ Res. 1987; 61: 352–71
- Arnar DO, Bullinga JR, Martins JB. Role of the Purkinje system in spontaneous ventricular tachycardia during acute ischemia in a canine model. Circulation. 1997; 96: 421–9
- Arnar DO, Martins JB. Purkinje involvement in arrhythmias after coronary artery reperfusion. Am J Physiol. 2002; 282: H1189–H1196
- Arnar DO, Xing D, Lee H, Martins JB. Prevention of ischemic ventricular tachycardia of Purkinje origin: Role for α-2 adrenoceptors on Purkinje?. Am J Physiol. 2001; 280: H1182–H1190
- Cable DG, Rath TE, Dreyer ER, Martins JB. Refractory period responses of cardiac Purkinje tissue to α1 and α2 adrenergic influences. Am J Physiol. 1994; 267: H376–H382
- Samson RA, Cai JJ, Shibata EF, Martins JB, Lee H. Electrophysiological effects of α2-adrenergic stimulation in canine cardiac Purkinje fibers. Am J Physiol. 1995; 268: H2024–H2035
- Lee H, Samson RA, Cai JJ. α2 adrenergic receptor binding in canine Purkinje fibers. FEBS Lett. 1996; 380: 39–43
- Stephenson RS, Cai JJ, Drews TA, Lee H. Effect of α2-adrenoceptor stimulation on isolated Purkinje fiber contraction. Eur J Pharmacol. 1998; 345: 261–7
- Ruffy R, Lovelace D, Mueller TM, Knoebel SB, Zipes DP. Relationship between changes in the left ventricular bipolar electrograms and regional myocardial blood flow during acute coronary occlusion in the dog. Circ Res. 1979; 45: 764–70
- Arnar DO, Cai JJ, Lee H, Martins JB. Electrophysiologic effects of civamide (zucapsaicin) on canine cardiac tissue in vivo and in vitro. J Cardiovasc Pharmacol. 1998; 32: 875–83
- Xing D, Martins JB. Triggered activity due to delayed afterdepolarizations recorded from sites of origin of ischemic ventricular tachycardia. Am J Physiol. 2004; 2287: H2078–H2084
- Szumowski L, Sanders P, Walczak F, Hocini M, Jais P, Kepski R, et al. Mapping and ablation of polymorphic ventricular tachycardia after myocardial infarction. J Am Coll Cardiol. 2004; 44: 1700–6
- Haissaguerre M, Shah DC, Jais P, Shoda M, Kautzner J, Arentz T, et al. Role of Purkinje conducting system in triggering of idiopathic ventricular fibrillation. Lancet. 2002; 359: 677–8
- Nakagawa H, Beckman KJ, McClelland JH, Wang X, Arruda M, Santoro I, et al. Radiofrequency catheter ablation of idiopathic left ventricular tachycardia guided by a Purkinje potential. Circulation. 1993; 88: 2607–17
- Caceres J, Jazayeri M, McKinnie J, Avitall B, Denker ST, Tchou P, et al. Sustained bundle branch reentry as a mechanism of clinical tachycardia. Circulation. 1989; 79: 256–70
- Lubbe WF, Podzuweit T, Opie LM. Potential arrhythmogenic role of cyclic adenosine monophosphate (AMP) and cytosolic calcium overload: Implications for prophylactic effects of beta-blockers on myocardial infarction and proarrhythmic effects of phosphodiasterase inhibitors. J Am Coll Cardiol. 1992; 19: 622–33
- Anonymous. Yohimbine for male sexual dysfunction. The Medical Letter. 1994;36:115–6.
- Corr PB, Heathers G, Yamada KA. Mechanisms contributing to arrhythmogenic influences of α1-adrenergic stimulation in the ischemic heart. Am J Med. 1989; 87(Suppl 2A)19S–25S
- Sheridan DJ, Penkoske PA, Sobel BE, Corr PB. Alpha-adrenergic contributions to dysrhythmia during myocardial ischemia and reperfusion in cats. J Clin Invest. 1981; 65: 161–71
- Cai JJ, Morgan DA, Haynes WG, Martins JB, Lee HC. Alpha 2-Adrenergic stimulation is protective against ischemia-reperfusion-induced ventricular arrhythmias in vivo. Am J Physiol. 2002; 283: H2606–2611
- Sun S, Weil MH, Tang W, Kamohara T, Klouche K. Alpha-methyl-norepinephrine, a selective alpha2-adrenergic agonist for cardiac resuscitation. J Am Coll Cardiol. 2001; 37: 951–6