Abstract
Objective.To develop a porcine model for Left Ventricular Hypertrophy (LVH) in which cardiac performance could be quantified non-invasively by Doppler ultrasound. Design.Sixteen 5 kg piglets were divided into two groups. In the first group (n=12) we performed an aortic banding and in the second group (n=4) a sham-operation. Endpoints were echo-assessed left ventricular midseptal and free-wall thickness, heart/body-weight ratio and cardiac myocyte diameter. Results.Free-wall thickness: 0.77±0.013 cm in the intervention group and 0.60±0.006 cm in the control group (p=0.015). Midseptal thickness: 0.79±0.015 cm in the intervention group and 0.58±0.010 cm in the control group (p=0.012). Heart/body-weight ratio: 7.73±0.970 in the intervention group and 6.23±0.430 in the control group (p=0.003). Cardiac myocyte diameter: 19.6±4.9 µm in the intervention group and 11.0±1.9 µm in the control group (p=0.000). Conclusion. A chronic porcine model for LVH has been established in which Doppler ultrasound can be used to quantify cardiac function non-invasively.
Left Ventricular Hypertrophy (LVH) is a common finding in patients suffering from aortic valve stenosis or systemic arterial hypertension. The increased pressure load on the left ventricle causes the cardiac myocytes to enlarge whilst the extracellular matrix and proteins accumulates Citation1–5. Many aspects of LVH are not fully understood but increased β adrenerg response Citation[6] and impaired diastolic compliance and relaxation are suggested to play a major role in the distinctive hemodynamic instability Citation7–10.
Some of these aspects could be disclosed by creating a chronic animal LVH model in which the difficulties of the hemodynamic management could be investigated systematically. Previous attempts have been made to develop such an animal model in mice, dogs and pigs using various surgical techniques Citation11–19. Quantification of cardiac performance in these models is of an invasive nature, and the use of non-invasive Doppler ultrasound imaging would be impaired due to the size of the experimental animals. Having conducted numerous studies on Danish landrace pigs, it is our experience that the quality of the Doppler ultrasound images falls short in animals with a body weight above approximately 25 kg as a consequence of the spherical thoracic shape and substantial sternal bone structure. Considering the constant development of the various Doppler ultrasound modalities as well as the extensive clinical use, it would be desirable to have an experimental animal model in which Doppler ultrasound could be utilized.
Hypothesis
We propose a model in which a slowly progressing incline in afterload is created by placing a band tightly yet not obstructively around the ascending aorta of piglets at an early stage of development. The normal growth of the animal will in due course create the narrowing of aorta at the site of the fixed size band thus mimicking aortic valve stenosis and thereby inducing LVH.
Aim
To develop and validate a chronic porcine model for left ventricular hypertrophy in which Doppler ultrasound can be used to quantify cardiac performance.
Materials and methods
The study was conducted as a prospective randomized animal experimental intervention-control study on 16 female Danish landrace pigs with a mean body weight of 5.2 kg (±0.40 kg). In the intervention group (n = 12) we performed the actual banding procedure and in the control group (n = 4) we performed a sham-operation to obtain baseline values. After the surgical intervention the animals were seen at follow-up examinations after 4, 6 and 8 weeks. At the 8 weeks follow-up the animals were euthanized. The study complied with the guidelines of The Danish Inspectorate for Animal Experimentation and was approved by this institution.
Surgical procedure
Each animal was pre-anaesthetized by an intra muscular injection of 0.5 mg/kg Midazolam and 10.0 mg/kg Ketamine (Ketolar Vet.®) to allow intravenous access via an ear vein, and the same amount of Midazolam and Ketamine was given by this route. The animal was then endotracheally intubated and coupled to a ventilator and given 0.4 mg/kg Pancuronium (Pavulon®). During the surgical intervention continuous anaesthesia and analgesia were maintained by 1.5% Isoflurane (Forene®) and 50.0 µg/kg/hour Fentanyl (i.v.).
A left lateral mini-thoractomy was made in the third intercostal space to expose the ascending aorta. The circumference of the ascending aorta was estimated by placing a suture around the aorta. A silicone tube treaded with a guiding suture was placed around the aorta. By tying the guiding suture the silicone tube could prevent increasing aortic diameter with growth of the animal. The thoracic wall was closed in layers. Before the animal was awake prophylactic antibiotics (40.0 mg/kg Cefuroxim (i.v.)) and a NSAID (5.0 mg/kg Flunixin Vet.® (i.m.)) were administered. Finally the animal was extubated and transported back to the farm.
In order to ensure comparability between the intervention and control group, a sham operation was performed on the animals in the control group. The surgical procedure was similar, but instead of banding the ascending aorta, a silicone tube with a length of 10 mm was sutured in a non-constricting fashion at the same location as for the intervention group animals.
Follow-up examinations
At each follow-up examination the animal was anesthetized, weighed and echocardiographically scanned. At the final 8 weeks follow-up the animal was euthanized with an i.v. injection of 2,000 mg pentobarbital and the heart was excised for post mortem examinations ().
The echocardiographic scans were obtained with a 2.5 MHz Matrix transducer coupled to a VIVID7 ultrasonic echo machine (GE Healthcare, Horten, Norway). All pigs were scanned in three standardized views: a four chamber view from a subcostal acoustic window and parasternal long and short axis views achieved through a right transthoracic acoustic window (A,B). Left ventricular midseptal and free wall thickness at papillary muscle level was quantified in an offline analysis in EchoPac (GE Healthcare, Horten, Norway). The image quality of all scans were graded from 1-5 according to the method described by Jakobsen et al. Citation[20]. In order to reduce the variability of the echocardiographic examinations, these were all performed by the same person. The offline measurements were made twice by the same person, and once by a second person (both trained in echocardiographic measurements) thus enabling us to investigate the intra- and interobserver variability. Variability plots were displayed by the method described by Bland and Altman Citation[21].
Figure 2. (A) Short axis scan of a pig in the control group showing a left ventricle with normal thickness. (B) Short axis scan of a pig in the intervention group showing a left ventricle with increased thickness. (C) Doppler curve on a pig in the control group showing a transvalvular blood velocity of 1 m/s. (D) Doppler curve on a pig in the intervention group showing a transvalvular blood velocity of 4 m/s.
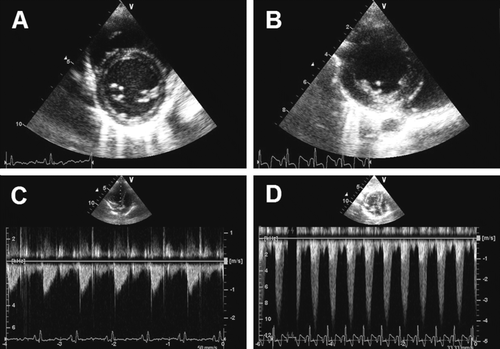
In order to monitor the progression of the stenosis in the intervention group, we furthermore made Continuous Wave (CW) echo scans across the stenosis in a four chamber view. The blood velocity across a normal aortic valve is about 1 m/s, while the velocity across a stenosis is approximately 4 m/s or higher (C,D). An estimation of the pressure drop across the stenosis can then be calculated from a simplified form of the Bernoulli's equation Citation[22]: ΔP = v2·4 (P: pressure, v: velocity) This simplified equation was used at the final follow-up examinations to ensure that a pressure drop of at least (ΔP=42·4 =) 64 mm Hg had developed in the intervention group animals-indicating a hemodynamically significant stenosis.
The endpoints compared between the control group and intervention group animals were:
Left ventricular midseptal thickness at papillary muscle level.
Left ventricular free wall thickness at papillary muscle level.
Heart/Body Weight Ratio (H/BWR).
Diameter of the cardiac myocytes.
While the first two endpoints were assessed by echo cardiographic measurements, the latter two were assessed at the post mortem examination.
At the post mortem examination the heart was excised, rinsed, weighed and the Heart/Body Weight Ratio (H/BWR) was calculated. The heart was fixated in formaldehyde and sections were cut from the left ventricle and dyed with a Haematoxyline-Eosine (HE) solution. The HE coloured sections were then microscopically examined, and the diameter of 100 randomly selected cardiac myocytes were measured in each section. The examinator was blinded in respects to which sections were from an intervention animal and which were from a control animal.
Statistics
The endpoint data obtained from the two groups were compared by use of an unpaired t-test. Standard Deviations and p-values were calculated for all results. P-values < 0.05 were considered statistically significant. Results are displayed as mean±Standard Deviation.
Results
Complete datasets were acquired from 13 of the 16 animals. Three animals were excluded from the final study group: Two banded pigs developed acute postoperative pulmonary oedema in the hours following surgery, and one banded pig developed symptoms of dilatatory heart failure after 4 weeks and had to be euthanized prior to expected termination. The remaining nine animals in the intervention group thrived and developed no symptoms of cardiac failure. The standardized Doppler ultrasound scans could easily be performed and produced echocardiographic images of a high quality.
Discussion
This study demonstrates that it is possible to induce LVH within 8 weeks in pigs by supracoronary aortic banding via a minimal invasive procedure.
The remodelling of the LV was evident after only 4 weeks, where there was a significant difference between the intervention and the control group of animals in terms of thickness of both the septum and the free wall (). Throughout the study period the growth of the LV persisted in a practically linear fashion in the intervention group, whilst the LV growth in the control group seemed to peak after 6 weeks. This paradox growth pattern, yet puzzling, might be within the range of the normophysiologic growth of the porcine heart, seeing that this occurred within the control group. This could be explained by a two phased growth; the first phase being a myocardial growth phase (with increased myocardial thickness) followed by dilatatory phase (where the lumen expands thus creating the paradox appearance of a decreased myocardial thickness).
Table I. Results from the follow-up and post mortem examinations: Midseptal thickness, Free wall thickness, Heart/Body Weight Ratio×1000 and Diameter of the cardiac myocytes. Results are displayed as mean±Standard Deviation.
The echocardiographic results from the final follow-up examination after 8 weeks clearly showed that the septum and free wall had thickened significantly in the intervention group compared to the control group.
The post mortem examination revealed a significant variation in both the body weight of the animals as well as the weight of their hearts. This variation would bias a comparison of the isolated heart weight, seeing that a larger animal would almost certainly have a larger heart. However, by relating the weight of the heart to the animal body weight in a ratio (H/BWR), we could circumvent any problems arising from these variations. The H/BWR was significantly increased in the intervention group compared with the control group (), which supports the echocardiographic findings.
The mean diameter of the cardiac myocytes was also significantly increased in the intervention group, indicating that cellular hypertrophy had occurred (). The high number of measurements (100 cells for each of the thirteen animals) ensured a high level of precision, thus furthermore substantiating our findings of LVH in the intervention group.
Ultrasonic measurements have an inherent intra- and interobserver variability. We investigated this variability with the Bland-Altman technique and found the intraobserver data plots evenly spread around the mean difference of 0.0, with limits of agreement ranging from −0.08 to 0.08. This indicates that there was no systematic bias when the observer repeated his own offline measurements.
The interobserver data plots are also evenly spread around a mean difference, which is slightly lower (−0.01). The limits of agreement range from −0.17 to 0.17, which indicates that there was a higher degree of variability when two independent observers made the offline measurements. The interobserver variability, however, is still well within acceptable range. Based on this variability analysis we conclude that the offline measurements were made without systematic bias.
The results indicate unanimously that we were able to induce LVH in the intervention group. Other studies have succeeded in this as well, but with noteworthy differences in surgical techniques: Massie et al. Citation[12] banded the ascending aorta and tightened the band until a transvalvular pressure drop of 20 mm Hg was reached, while Ye et al. Citation[16] and Gong et al. Citation[15] tightened the band until a pressure drop of 60 – 70 mm Hg was reached. We believe that this induced acute pressure drop is too aggressive an approach and presents an unnecessary acute stress to the LV. The pathogenetics of aortic valve stenosis in humans is of a gradual nature, thus slowly adapting the LV myocytes to the slowly progressing incline in afterload. For obvious reasons it would be extremely costly if an experimental LVH model required several years for pathogenetic development in order for an accurate and unbiased comparison to the human pathogenetics of LVH. Therefore any claim of a chronic animal model being comparable to human pathogenetics has an inherent bias. As a result one should always carefully consider the possible blunders of extrapolating data from a short-term chronic animal model. However, the necessity of short-term chronic models is evident seeing that feasibility is of the essence. The banding technique applied in our model allows the pressure drop to develop with in a time frame of 8 weeks. In this way the development of LVH in our model is somewhat closer to pathophysiologic progression of aortic valve stenosis than other banding techniques used in other studies. The surgical access of choice in our study is furthermore less invasive than the traditional sternum spilt used in most open chest models. By using a minimal invasive surgical technique we assure that the animal's thoracic anatomy is as intact as possible in terms of iatrogenic alterations (scar tissue etcetera) and the model can therefore be considered as a closed chest model at follow-up examinations accordingly.
The three standardized echocardiographic images acquired by Doppler ultrasound consistently proved to be of a very high quality. The high image quality and the ease in which the scans were obtained convince us that our LVH model is highly usable in closed chest experiments where a non-invasive assessment of cardiac function is of interest. A wide range of ultrasonic modalities can be applied from the three standardized views: The parasternal long axis view allowed for quantification of cardiac dimensions in M-mode, while the short axis could provide an assessment of the level of concentric hypertrophy as well as a visualization of the supply areas of each of the three branches of coronary arteries. With the recent introduction of speckle tracking it will furthermore be possible to investigate changes in LV rotation pattern form the parasternal view Citation[23].
In this study the four chamber view provided the means to measure the pressure drop across the stenosis using CW Doppler. The four chamber view could be further utilized in other studies, using this model, by applying advanced modalities for measurement of myocardial velocities like Tissue Doppler Imaging (TDI) which allows for a detailed analysis of the various stages of the cardiac cycle, for instance the isovolumetric contraction time (IVC) and isovolumetric relaxation time (IVR). From TDI quantitative measures of myocardial deformation (strain) deformation rate (strain-rate) and myocardial longitudinal displacement (e.g. tissue tracking) can be derived Citation[24]. Also speckle tracking can be applied to this view. Hence these modalities enable a comprehensive analysis of the systolic and diastolic function in a closed chest LV hypertrophy animal model.
Study limitations
The banding of the ascending aorta was made on a supracoronary level, and consequently the stenosis occurred downstream of the coronary arteries. This differs from the human pathoanatomy of aortic valve stenosis, where the stenosis occurs upstream of the coronary orifices. This might be an important difference, seeing that the pathologic changes in pressure pre- and post stenotic most likely have an effect on the blood flow to the coronary arteries. The altered blood flow through the coronary arteries might play a role in the development of LVH in aortic valve stenosis. Therefore, an infracoronary banding would be desirable, but certainly also more technically demanding due to the confined anatomic environment.
Conclusion and perspectives
A reliable chronic porcine model for LVH was developed. By supracoronary banding of the ascending aorta a hypertrophy of the left ventricle was induced within a time frame of 8 weeks. Due to the relatively small size of the animals the cardiac performance could be monitored non-invasively by Doppler ultrasound. This porcine model for LVH can consequently form the basis in a wide range of closed chest experimental settings -i.e. evaluation of surgical and pharmacologic interventions used on human LVH patients, and systematic investigations of a considerable amount of clinical problems LVH patients form concerning development of tamponade, hypovolemia, overload and congestive heart failure.
Acknowledgements
We would like to thank The Danish Government's Council for Research in Health and Disease, The Kirsten Antonius Foundation, The Jens Anker Andersen Foundation, The Institute of Clinical Medicine, The Skejby Sygehus Research Foundation, The Sophus Jacobsen & Astrid Jacobsen Foundation, The Helga and Peter Kornings Foundation and The Danish Heart Foundation.
References
- Klabunde RE. Cardiovascular physiology concepts. Lippincott Williams & Wilkins. 2004
- Zipes D, Braunwald E. Braunwald's heart disease: A textbook of cardiovascular medicine6th ed. W. B. Saunders Company. 2004
- Meerson, F. Compensatory hyperfunction of the heart and cardiac insufficiency. Circ Res. 1962;10.
- Opie LH, Commerford PJ, Gersh BJ, Pfeffer MA. Controversies in ventricular remodelling. Lancet. 2006; 367: 356–67
- Mancia G, Scopelliti F, Grassi G. Hypertension and the heart. Semin Cardiothorac Vasc Anesth. 2006; 10: 198–202
- Prys-Roberts C, Meloche R. Management of anesthesia in patients with hypertension or ischemic heart disease. Int Anesth Clin. 1980; 18: 181–217
- Levy D, Garrison RJ, Savage DD, Kannel WB, Castelli WP. Prognostic implications of echocardiographically determined left ventricular mass in the Framingham Heart Study. N Engl J Med. 1990; 322: 1561–6
- Levy D, Garrison RJ, Savage DD, Kannel WB, Castelli WP. Left ventricular mass and incidence of coronary heart disease in an elderly cohort. The Framingham Heart Study. Ann Intern Med. 1989; 110: 101–7
- Haider AW, Larson MG, Benjamin EJ, Levy D. Increased left ventricular mass and hypertrophy are associated with increased risk for sudden death. J Am Coll Cardiol. 1998; 32: 1454–9
- Mehta R, Bruckman D, Das S, Tsai T, Russman P, Karavite D, et al. Implications of increased left ventricular mass index on in-hospital outcomes in patients undergoing aortic valve surgery. J Thorac Cardiovasc Surg 2001; 122: 919–28
- Sharma, GP, Singal, PK, Dhalla, NS. Hemodynamic adaptation of the left ventricle during aortic stenosis in pigs. J Mol Cell Cardiol. 1980;12(Supp. 1).
- Massie BM, Schaefer S, Garcia J, McKirnan MD, Schwartz GG, Wisneski JA, et al. Myocardial high-energy phosphate and substrate metabolism in swine with moderate left ventricular hypertrophy. Circulation 1995; 91: 1814–23
- Novick RJ, Stefaniszyn HJ, Michel RP, Burdon FD, Salerno TA. Protection of the hypertrophied pig myocardium. A comparison of crystalloid, blood, and Fluosol-DA cardioplegia during prolonged aortic clamping. J Thorac Cardiovasc Surg. 1985; 89: 547–66
- Kassab GS, Gregersen H, Nielsen SL, Lu X, Tanko LB, Falk E. Remodelling of the left anterior descending artery in a porcine model of supravalvular aortic stenosis. J Hypertens. 2002; 20: 2429–37
- Gong G, Liu J, Liang P, Guo T, Hu Q, Ochiai K, et al. Oxidative capacity in failing hearts. Am J Physiol Heart Circ Physiol 2003; 285: H541–8
- Ye Y, Gong G, Ochiai K, Liu J, Zhang J. High-energy phosphate metabolism and creatine kinase in failing hearts: A new porcine model. Circulation. 2001; 103: 1570–6
- Marionneau C, Brunet S, Flagg TP, Pilgram TK, Demolombe S, Nerbonne JM. Distinct cellular and molecular mechanisms underlie functional remodeling of repolarizing K+ currents with left ventricular hypertrophy. Circ Res 2008; 102: 1406–15
- Stansfield W, Rojas M, Corn D, Willis M, Patterson C, Smyth S, Selzman C. Characterization of a model to independently study regression of ventricular hypertrophy. J Surg Res. 2007; 142: 387–93
- Hittinger L, Mirsky I, Shen YT, Patrick TA, Bishop SP, Vatner SF. Hemodynamic mechanisms responsible for reduced subendocardial coronary reserve in dogs with severe left ventricular hypertrophy. Circulation. 1995; 92: 978–86
- Jakobsen CJ, Torp P, Sloth E. Perioperative feasibility of imaging the heart and pleura in patients with aortic stenosis undergoing aortic valve replacement. Eur J Anaesthesiol. 2007; 24: 589–95
- Bland JM, Altman DG. Statistical methods for assessing agreement between two methods of clinical measurement. Lancet. 1986; 1(8476)307–10
- Landau L, Lifschitz E. Fluid mechanics. Pergamon Press. 1959
- Helle-Valle T, Crosby J, Edvardsen T, Lyseggen E, Amundsen BH, Smith HJ, et al. New noninvasive method for assessment of left ventricular rotation. Circulation 2005; 112: 3149–56
- Andersen NH, Poulsen SH. Evaluation of the longitudinal contraction of the left ventricle in normal subjects by Doppler tissue tracking and strain rate. J Am Soc Echocardiogr. 2003; 16: 716–23