Abstract
Objectives. The aim of this study was to investigate how high K+ concentrations can be safely used in cardioplegic solutions without causing severe coronary artery vasocontraction.
Design. Twenty-four 50 kg pigs were used. The distal part of the left anterior descending coronary artery was cut into ring segments and transferred into organ baths with Krebs solution bubbled with 95% O2 and 5% CO2. K+ concentrations between 16 and 127 mM were used to induce vasocontractions at 37, 22, 15, and 8 °C. Mg2+ (0–20 mM) were used to attenuate K+ induced vasocontractions.
Results. K+-Krebs solution 127 mM at 37 °C induced a strong, sustained vasocontraction defined as 100%. The contractions induced by 16, 23, 30 and 127 mM K+ were: 7.7, 38, 72 and 100% at 37 °C; 1.7, 7.4, 21 and 65% at 22 °C; 1, 6.6, 15 and 33% at 15 °C; 0.6, 2.1, 6 and 14% at 8 °C, respectively. Mg2+ reduced the K+-induced contraction at 37 °C in a concentration-dependent way and Mg2+ at 8 mM practically eliminated the risk for severe vasocontraction.
Conclusions. Hypothermia (8 °C) abolishes coronary contraction induced by K+-cardioplegic solutions. In normothermic cardioplegia 8 mM Mg2+ prevents vasoconstriction.
Introduction
When cardioplegia was introduced in clinical heart surgery, it was combined with hypothermia to bring the myocardial temperature down to less than 10 °C [Citation1,Citation2]. The myocardial temperature was measured with a needle temperature-probe placed centrally in the septum. The cardioplegic solution used was taken from the refrigerator, and many surgeons used cold saline or ice-slush around the heart to ensure that the myocardial temperature was kept below 10 °C between each dose of cold cardioplegia. A typical cardioplegic solution, e.g. St. Thomas solution II (Plegisol), is an extracellular, crystalloid, Ringer-like solution with the exception that the K+ concentration is increased from 4.6 to 16 mM and the Mg2+ concentration is increased from 1.2 to 16 mM [Citation2].
This type of cold, ischemic cardioplegia is still standard for preservation of donor hearts in clinical transplantation. In routine heart surgery today, blood cardioplegia is the new standard in many clinics. Blood cardioplegia can be given cold or normothermic [Citation2–4]. In both cases, KCl is used to depolarize the cardiomyocytes and cause cardioplegia at K+ concentrations ranging from 16 to 40 mM [Citation5]. Recently, K+-cardioplegia used in aerobic hypothermic perfusion has been demonstrated to give excellent prolonged heart preservation of donor hearts [Citation6,Citation7]
K+ in preservation solutions may cause vasoconstriction [Citation8]. Mg2+ is a physiologic Ca2+ blocker and may attenuate K+-induced vasocontraction [Citation9].
The aim of the present study was to investigate how high K+ concentrations can be used in cardioplegic solutions, without causing severe coronary artery vasocontraction, and to evaluate the role of Mg2+ in avoiding K+-induced vasoconstriction.
Materials and methods
Twenty-four Swedish domestic pigs with a mean weight of 49 kg (range 40–60 kg) were used. All animals received care in compliance with the European Convention for the Protection of Vertebrate Animals Used for Experimental and Other Scientific Purposes (Directive 2010/63/EU). The Ethics Committee for Animal Research Malmö/Lund approved the study (No. M174-15).
Anaesthesia and animal preparation
Anaesthesia was induced by means of an intramuscular injection of ketamine 20 mg/kg body weight (Ketaminol Vet. Intervet, Boxmeer, Netherlands), xylazine 100 mg (Rompun Vet, Bayer, Solna, Sweden), and atropine 0.5 mg (Atropine, Mylan AB, Stockholm, Sweden). Fentanyl 4 µg/kg body weight (Fentanyl B. Braun, Melsungen, Germany) and midazolam 0.4 mg/kg body weight (Midazolam Panpharma, Panpharma S.A., Trittau, Germany) were given intravenously through an ear vein before tracheostomy. Volume-controlled and pressure-regulated ventilation with a minute volume of 100–150 mL/kg body weight and a frequency of 20 breaths/minute was used. Positive end-expiratory pressure was adjusted to 5 cm H2O and the inspired oxygen fraction was 0.5. Aortic pressure and central venous pressure were measured with catheters placed into ascending aorta and superior vena cava.
A median sternotomy was performed. Heparin 500 IU/kg bodyweight (Heparin, LEO Pharma, Malmö, Sweden) was given intravenously. Ventricular fibrillation was induced with a 9V alkaline battery. The distal part of the left anterior descending coronary artery with surrounding tissue was immediately excised and gently flushed with normal Krebs solution. The composition of the Krebs solution was (in mM) NaCl, 119; NaHCO3, 18; KCl, 4.6; NaH2PO4, 1.2; MgCl2, 1.2; CaCl2, 1.5; and glucose, 5.5. The LAD coronary artery was dissected from surrounding tissue under a microscope (Leica WILD M691, Wild Leitz Ltd, Heerbrugg, Switzerland) and cut into 2.57 ± 0.02 mm long segments. The segments were immediately transferred to organ baths.
Pre-stretching the coronary artery segments
Isometric tension was measured in a water-mantled chamber with a volume of 5 mL Krebs solution bubbled with 95% oxygen and 5% carbon dioxide to give a pH of approximately 7.4, at a temperature of 37 °C. Each ring segment was suspended between two metal needle holders (0.2 mm in diameter). One holder was attached to a Grass FT03 transducer connected to a Grass polygraph for continuous recording of isometric tension (Grass Instrument Co, Quincy, MA). The other metal holder was fixed to an adjustable unit used to repeatedly stretch the vessel segments. Maximal contractile response could be obtained using a basal tension between 8 and 12 mN. (). Therefore, a basal tension of 10 mN was used in all experiments. The length of the vessel segments and the distance between the two metal holders were measured at the basal tension (10 mN), using a microscope equipped with an ocular scale. The diameter of the vessel segments was calculated by multiplying the distance between the metal holders by two and dividing by 3.14. Contractions were induced by replacing the normal Krebs solution in the bath with 127 mM K+-Krebs solution. After each contraction, the K+-Krebs solution was repeatedly washed out with normal Krebs solution until the basal tension was reached. The composition of 127 mM K+-Krebs solution was (in mM) NaHCO3, 18; KCl, 127; NaH2PO4, 1.2; MgCl2, 1.2; CaCl2, 1.5; and glucose, 5.5. The difference between the second and a third K+-Krebs-induced contraction varied by less than 10% and the second contraction was, therefore, used and defined as 100% contraction.
Figure 1. K+ (127 mM) induced coronary artery contraction at increasing basal tensions. A tracing from a typical experiment. The calculated diameter of the vessel is also given. Basal tension (BT) in mN and diameter (∅) in mm.
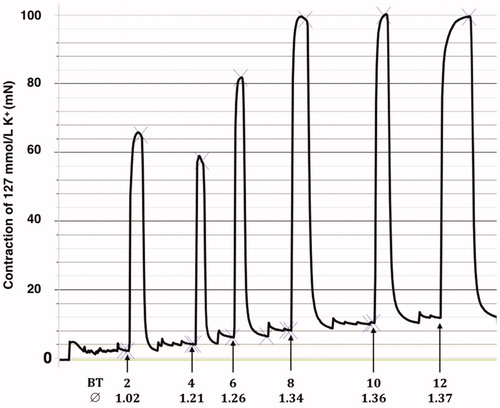
Thereafter, two series of experiments were performed. In the first series, the contractions elicited by different K+ concentrations were tested in the following order: 127, 16, 23 and 30 mM at 37 °C and at either 22, 15 or 8 °C (n = 18). In the other series of experiments, the effect on K+-induced contractions elicited by different Mg2+ concentrations was tested at 37 °C using K+ concentrations of 16, 23, 30 and 40 mM (n = 6). To obtain Krebs solution with K+ concentrations of 16, 23, 30 and 40 mM, the amount of NaCl in normal Krebs solution was exchanged for corresponding amounts of KCl.
Recording of contractility induced by K+-Krebs at different temperatures
At normothermia, 16, 23 and 30 mM K+-Krebs solutions were cumulatively added into the baths to induce contractions until plateaus of the contractile response were reached. Then, normal Krebs solution was used to repeatedly “wash out” the K+ effect until the basal tension was reached. The system temperatures were then set at 8, 15 or 22 °C, respectively, in three groups (n = 6 pigs in each group). When the basal tension had stabilized at the set temperature, two contractions were induced with 127 mM K+-Krebs solution. Then, 16, 23 and 30 mM K+-Krebs solutions were added cumulatively to induce contraction.
Recording of relaxation induced by different concentrations of Mg2+ on different concentrations of K+ at 37 °C
At a steady contraction induced with 16 mM K+-Krebs solutions in the bath, increasing concentrations of Mg2+ (up to 20 mM) were cumulatively added to the bath. Then, the K+ and Mg2+ enriched solution was washed out with normal Krebs solution until the basal tension was reached. After stabilizing the vessel segments at basal tension, the same procedure with Mg2+ was repeated three times but with 23, 30 and 40 mM of K+-Krebs solution, respectively, to induce a steady contraction.
Data analysis
Results were expressed as the mean ± standard error of the mean. One-way ANOVA with Tukey´s Multiple Comparison Test was used for statistical calculation.
Results
Basal tension at different temperatures
The basal tension decreased with decreasing temperatures (). The tensions (in mN) were: 37 °C= 9.9 ± 0.1; 22 °C= 9.1 ± 0.2; 15 °C= 8.6 ± 0.4 and 8 °C= 7.9 ± 0.2.
Size of the coronary artery segments
The length of the vessel segments was 2.57 ± 0.02 mm.
In the first series of experiments, at the basal tension of 10 mN, the diameter of the coronary artery segments was 1.45 ± 0.02 mm (n = 18). There was no statistically significant difference regarding diameter between the segments used at 22, 15 and 8 °C, which were 1.43 ± 0.02, 1.46 ± 0.03, and 1.44 ± 0.04 mm, respectively.
The diameter of the vessel segments used in the Mg2+ experiments was 1.48 ± 0.04 mm.
Vasocontraction induced with K+
K+ 127 mM caused a strong vasocontraction of 60.1 ± 1.6 mN at 37 °C, defined as 100% contraction. The contractions induced with 127 mM of K+ followed by either 16, 23, or 30 mM K+ at different temperatures are shown in and .
Figure 3. K+ induced coronary artery contractions at different temperatures. Results are given as mean ± SEM, n = 6.
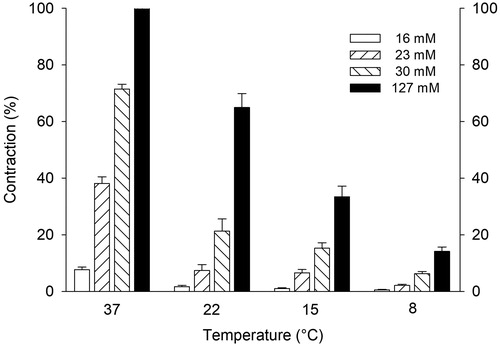
Table 1. K+ induced coronary artery contractions at different temperatures. Values are given as mean ± SEM in mN and as % of contraction induced by 127 mM K+ at 37 °C.
Reduced vasocontraction with Mg2+
The contraction induced by 127 mM of K+ was 69.4 ± 6.3 mN, defined as 100%. Increasing concentrations of Mg2+ caused concentration-depended reduction of vasocontraction of the pre-contracted vessel segments at 37 °C. ()
Discussion
To study vascular smooth muscle contractions in organ baths, the optimal basal tension needs to be defined. As seen in , we found a basal tension of 10 mN to be optimal; using less basal tension will not elicit maximal contraction by 127 mM K+.
The temperature had a significant effect on the basal tension. Cooling from 37 to 8 °C resulted in a 21% reduction in the basal tension (). In an in vivo perfusion model, Leicher et al. [Citation5] observed that after reducing the temperature from 38 to 28 °C, the coronary vascular resistance of empty beating canine hearts was halved.
Increasing the extracellular concentration of K+ in a cardioplegic solution causes a depolarization of the resting membrane potential of the cardiomyocytes (normally around 85 mV) to a value that is less negative. When the membrane potential reaches approximately -65 mV, the voltage-dependent fast Na+ channels are inactivated, thereby inducing diastolic arrest [Citation10,Citation11]. To obtain a resting membrane potential of less negative than -65 mV, extracellular K+ concentrations of up to 30 mM are used [Citation11] with the risk of causing coronary artery constriction at normothermia (, ).
As shown in the present study, both the temperature and the K+ concentration have significant effects on coronary artery vessel contraction (). K+ concentrations below 30 mM have little effect on the coronary arterial vasotension at low temperatures (). To avoid high vascular resistance in “warm” heart surgery with blood cardioplegia, K+ concentrations of 30 mM or higher should be used with caution if Mg2+ is not included (). At 37 °C, 8 mM Mg2+ effectively attenuated K+ induced vasocontractions in concentrations up to 30 mM K+. Mg2+ is a potent Ca2+ blocker and has a dilating effect on K+-contracted smooth muscles by competing with Ca2+ for nonspecific binding sites [Citation9,Citation12].
Cardioplegia and hypothermia are used to reduce the oxygen consumption of the heart. The oxygen consumption of the perfused cardioplegic porcine heart at 37 °C is 1.1 mL/min/100 g heart tissue, at 22 °C it is 0.33 mL/min/100 g, and at 8 °C it is 0.16 mL/min/100 g [Citation13]. The oxygen consumption of a beating porcine heart with a mean cardiac output of 5.0 ± 0.24 L/min at normal blood pressure and temperature was in the range of 6–7 mL/min/100 g [Citation13]. Thus, by using K+-induced cardioplegia at normothermia, the oxygen consumption is reduced by 85% and by cooling the heart to 8 °C, the consumption is reduced by a further 85%, to less than 3% compared with a beating, normothermic, working heart [Citation13]. This is similar to results obtained on human hearts [Citation2,Citation3].
Intracellular concentration of K+ (127 mM, as used in the present study) is widely used in organ bath studies of human and animal blood vessels to get a standardized maximal vasocontraction which pharmacological induced vasocontractions can be related to [Citation14,Citation15]. As no difference between human and animal blood vessels have been shown regarding contractile response to K+, the results of this study should be of relevance when K+ cardioplegia is used in clinical heart surgery.
To conclude, at 8 °C, K+ can safely be used in cardioplegic solutions without causing severe vasoconstriction. At normothermic cardioplegia, K+ at 30 mM may elicit strong vasoconstriction which can be abolished by 8 mM Mg2+.
Disclosure statement
The authors report no conflict of interest.
Additional information
Funding
References
- Gunnes S, Jynge P. Fundamentals of the past: cardioplegia: the first period revisited. In: Podesser BK, Chambers DJ, editors. New solutions for the heart. Vienna: Springer; 2011. p. 15–40.
- Barner HB. Historical aspects and current review of myocardial protection. In: Salerno TA, editor. Warm heart surgery. Boston (MA): Arnold; 1995. p. 1–15.
- Salerno TA, editor. Warm heart surgery. Boston (MA): Arnold; 1995.
- Calafiore AM, Mezzetti A. Intermittent antegrade normothermic blood cardioplegia. In: Salerno TA, editor. Warm heart surgery. Boston (MA): Arnold; 1995. p. 77–89.
- Leicher FG, Magrassi P, LaRaia PJ, et al. Blood cardioplegia delivery. Deleterious effects of potassium versus lidocaine. Ann Surg. 1983;198:266–272.
- Steen S, Paskevicius A, Liao Q, et al. Safe orthotopic transplantation of hearts harvested 24 hours after brain death and preserved for 24 hours. Scand Cardiovasc J. 2016;50:193–200.
- Qin G, Sjöberg T, Liao Q, et al. Intact endothelial and contractile function of coronary artery after 8 hours of heart preservation. Scand Cardiovasc J. 2016;50:362–366.
- Kimblad PO, Sjöberg T, Massa G, et al. High potassium contents in organ preservation solutions cause strong pulmonary vasocontraction. Ann Thorac Surg. 1991;52:523–528.
- Iseri LT, French JH. Magnesium: nature's physiologic calcium blocker. Am Heart J. 1984;108:188–193.
- Chambers DJ, Fallouh HB. Cardioplegia and cardiac surgery: pharmacological arrest and cardioprotection during global ischemia and reperfusion. Pharmacol Ther. 2010;127:41–52.
- Chambers DJ, Fallouh HB. New approaches to cardioplegia: alternatives to hyperkalemia. In: New solutions for the heart. Vienna: Springer; 2011. p. 199–219.
- Turlapaty PD, Altura BM. Magnesium deficiency produces spasms of coronary arteries: relationship to etiology of sudden death ischemic heart disease. Science. 1980;208:198–200.
- Qin G, Su Y, Sjoberg T, et al. Oxygen consumption of the aerobically-perfused cardioplegic donor heart at different temperatures. Ann Transpl. 2018;23:268–273.
- Steen S, Skärby TVC, Norgren L, et al. Pharmacological characterization of postjunctional α-adrenoceptors in isolated human omental arteries and veins. Acta Physiol Scand. 1984;120:109–116
- Steen S, Sjöberg T, Skärby T V C, et al. Postjunctional α1- and α2-adrenoceptors mediating contraction in isolated human groin arteries and veins. Acta Physiol Scand. 1984;122:323–329