Abstract
Objectives. Heart rate variability (HRV) and haemodynamic response to exercise (i.e. peak cardiac power output) are strong predictors of mortality in heart failure. The present study assessed the relationship between measures of HRV and peak cardiac power output. Design. In a prospective observational study of 33 patients (age 54 ± 16 years) with chronic heart failure with reduced left ventricular ejection fraction (29 ± 11%), measures of the HRV (i.e. R-R interval and standard deviation of normal R-R intervals, SDNN) were recorded in a supine position. All patients underwent maximal graded cardiopulmonary exercise testing with non-invasive (inert gas rebreathing) cardiac output assessment. Cardiac power output, expressed in watts, was calculated as the product of cardiac output and mean arterial blood pressure. Results. The mean RR and SDNN were 837 ± 166 and 96 ± 29 ms, peak exercise cardiac power output 2.28 ± 0.85 watts, cardiac output 10.34 ± 3.14 L/min, mean arterial blood pressure 98 ± 14 mmHg, stroke volume 91.43 ± 40.77 mL/beat, and oxygen consumption 19.0 ± 5.6 mL/kg/min. There was a significant but only moderate relationship between the RR interval and peak exercise cardiac power output (r = 0.43, p = .013), cardiac output (r = 0.35, p = .047), and mean arterial blood pressure (r = 0.45, p = .009). The SDNN correlated with peak cardiac power output (r = 0.42, p = .016), mean arterial blood arterial (r = 0.41, p = .019), and stroke volume (r = 0.35, p = .043). Conclusions. Moderate strength of the relationship between measures of HRV and cardiac response to exercise suggests that cardiac autonomic function is not good indicator of overall function and pumping capability of the heart in chronic heart failure.
Introduction
Heart failure is a clinical syndrome associated with reduced cardiac function and high morbidity and mortality rates [Citation1]. Chronic heart failure patients demonstrate severe derangement of the autonomic nervous system characterised by chronic sympathetic hyperactivation, vagal withdrawal, resetting of the baroreflex sensitivity, and decreased peripheral and central responsiveness to adrenergic input [Citation2,Citation3]. Chronic sympathetic activation alters heart rate variability (HRV) which is an accepted measure of cardiac autonomic regulation and is the analysis of variation in time intervals between heart beats. Overall HRV (i.e. beat-to-beat, R–R interval]) is reduced in chronic heart failure [Citation3,Citation4], and is associated with significantly higher mortality rates [Citation5–7]. Two commonly evaluated and presented time domain measures of HRV i.e. RR interval and the standard deviation of normal-to-normal (N-N) intervals (SDNN), are strong predictors of prognosis in chronic heart failure [Citation5,Citation8,Citation9]. The SDNN also appears to be associated with left ventricular ejection fraction [Citation10].
On another hand, cardiac power output (CPO) is a central hemodynamic measure that demonstrates overall function and pumping capability of the heart [Citation11]. It is calculated as the product of simultaneously measured cardiac output and mean blood arterial pressure. By incorporating pressure and flow domains of the cardiovascular system, CPO is an integrative measure of overall function and pumping capability of the heart [Citation12]. Cardiac power output, measured at peak exercise, has been shown to be a most powerful predictor of prognosis and mortality in patients with chronic heart failure [Citation13,Citation14].
Whether autonomic regulation of the heart may be used as a surrogate measure of cardiac power output and haemodynamic response to exercise in chronic heart failure has not been investigated previously. Therefore, the aim of the present study was to evaluate the relationship between measures of heart rate variability, cardiac power output and haemodynamic response to exercise in patients with chronic heart failure.
Methods
Study design and patients
The prospective observational study recruited 33 patients with chronic heart failure due to reduced left ventricular ejection fraction. Patients were on optimal medical management and their demographic and clinical features are presented in . Exclusion criteria were the inability to perform maximal graded cardiopulmonary exercise test, inability to exercise beyond anaerobic threshold, symptomatic angina limiting exercise, and unwillingness to provide a consent form. The study protocol was approved by the UK Health Research Authority – North East Tyne and Wear South Research Ethics Committee (reference: 15/NE/0190). All patients provided written informed consent and all study procedures were in accordance to Declaration of Helsinki.
Table 1. Patients’ demographic and clinical characteristics.
Heart rate variability
Upon arrival in the clinical physiology laboratory, patients’ weight and height were measured. Heart rate variability was measured and evaluated using the TF5 heart rate monitor and associated software (Advanced Medical Diagnostics Ltd., Leeds, UK). After fitting the patients with the TF5 chest strap in accordance with manufacturer’s instructions, patients had 5 min of recordings whilst in the supine position with controlled breathing (12 breaths per minute) using a previously validated protocol [Citation15]. All R–R interval data were obtained with the automated algorithms available in the software. SDNN was calculated by calculating the standard deviation of N-N interval as recommended by the Taskforce on HRV [Citation4].
The 12 lead ECG electrodes were attached according to the standard lead configuration for exercise testing. Following a 5-min resting period while the patient sat on a chair, arterial blood pressure was assessed from the brachial artery by cuff sphygmomanometry.
Haemodynamic assessment
Measurement of cardiac output was taken in a seated position using the inert gas rebreathing method (Innocor A/S, Innovision, Odense, Denmark) as previously described [Citation16,Citation17]. The Innocor device employs a rebreathing system that briefly uses an oxygen-enriched mixture of an inert soluble gas (0.5% nitrous oxide) and an inert insoluble gas (0.1% sulphur hexafluoride) from a 4 L pre-filled anaesthesia bag. Photoacoustic analyzers measure gas concentrations during the rebreathing manoeuvre. Nitrous oxide concentration decreases during the rebreathing at a rate proportional to pulmonary blood flow, allowing estimation of cardiac output. Three to four respiratory cycles are needed to obtain a value for nitrous oxide washout. Acceptable reproducibility (i.e. coefficient of variation of <6%) of inert gas rebreathing method for estimating cardiac output at rest and during stress testing was previously confirmed in healthy individuals and patients with chronic heart failure [Citation18–20].
The Innocor device also incorporates a breath-by-breath module to evaluate gas exchange variables (oxygen consumption and carbon dioxide production measured using an oxygen sensor and a photoacoustic gas analyser) and minute ventilation [Citation21]. The gas sample line and airflow umbilical are connected to the respiratory valve unit. Measurement of pulmonary ventilation is performed by a pressure difference pneumotach. Carbon dioxide analysis is performed by using a photoacoustic gas analyser. Gas exchange data and ventilation analysis is performed and results presented by the Innocor software.
After 3 min of measurements at rest, patients performed a maximal graded cardiopulmonary exercise test using semi-recumbent cycle ergometer, starting unloaded (0 watts) cycling, with workload increased at the rate of 10 watts per minute and cadence of 60–70 revolutions per minute. Continuous breath-by-breath sampling of respiratory gases and heart rate measurements were undertaken. Patients were instructed to give a warning approximately 30 s before they felt they would end the exercise test to obtain a final cardiac output rebreathing measurement with arterial blood pressure taken simultaneously at the peak of exercise. Respiratory exchange ratio ≥1.05 was used as an objective indicator that a patient is approaching near maximal exertion and when rebreathing manoeuvre was initiated. The patient continued to exercise through the measurement and recovery phase was initiated after the rebreathing manoeuvre was complete.
Calculations and statistical analyses
The cardiac power output was calculated from the product of cardiac output and mean arterial pressure using the following equation [16] CPO = (QT × MAP) × K, where CPO is cardiac power output measured in watts, QT is cardiac output in litres per min, MAP is mean arterial pressure in millimetres of mercury, and K is the conversion factor (2.22 × 10−3). Mean arterial pressure was calculated as: DBP + 0.412 × (SBP − DBP), where SBP is systolic blood pressure and DBP is diastolic blood pressure [Citation22].
All statistical analysis was carried out using SPSS version 23.0 (SPSS, Chicago, Illinois). Before statistical analysis, data were checked for univariate and multivariate outliers using standard Z-distribution cut-offs and Mahalanobis distance tests, respectively. Normality of distribution was assessed using a Kolmogorov-Smirnov test. Student’s t-test was used to assess differences in measured variables in response to cardiopulmonary exercise testing. Nominal variables were evaluated using the Chi-square test. Pearson coefficient of correlation or Spearman’s rank correlation coefficient were used, as appropriate, to determine the relationships between variables. Multiple regression analyses were used to identify predictors of peak cardiac power output. Statistical significance was indicated if p < .05. All data are presented as mean ± SD unless otherwise indicated.
Results
Patient demographic and clinical characteristics are presented in . Heart rate variability measures obtained at rest with controlled breathing were: RR interval, 837 ± 166 ms, and SDNN, 96 ± 29 ms.
In response to exercise, patients significantly increased mean arterial blood pressure, cardiac output, cardiac power output, heart rate, stroke volume, and oxygen consumption as shown in .
Table 2. Response to maximal graded cardiopulmonary exercise testing.
There was a significant positive relationship between the RR interval and peak exercise cardiac power output (), cardiac output (), and mean arterial blood pressure (), but no significant relationship between RR interval and stroke volume was found ().
Figure 1. Relationship between RR interval and peak cardiac power output, CPO (A); cardiac output, QT (B); mean arterial blood pressure, MAP (C), and stroke volume, SV (D).
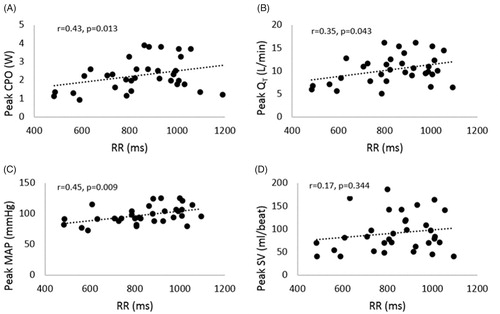
Similarly, there was a significant positive relationship between the SDNN and peak exercise cardiac power output (r = 0.42, p = .02), mean arterial blood pressure (r = 0.41, p = .02), and stroke volume (r = 0.35, p = .04), but no significant relationship was found between the SDNN and peak cardiac output (r = 0.32, p = .07). There was no a significant relationship between peak exercise O2 consumption and RR with (r = 0.05, p = .79) and SDNN (r = −0.02, p = .93).
Similarly, there was no significant relationship between anaerobic threshold, as a marker of submaximal functional capacity, and RR interval (r = 0.02, p = .0.83), and SDNN (r = −0.21, p = .16).
Measurements of cardiac function obtained at rest were not significantly correlated with RR interval and SDNN i.e. cardiac power output (r = 0.08, p = .66; and r = 0.09, p = .63), cardiac output (r = 0.12, p = .53; and r = 0.3, p = .09), heart rate (r = −0.29, p = .14; and r = −0.32, p = .12), or mean arterial blood pressure (r = 0.03, p = .88; and r = −0.17, p = .35). Stroke volume did not correlate with RR interval (r = 0.26, p = .15) but was significantly associated with SDNN (r = 0.44, p = .011).
A multiple regression analyses revealed predictors of peak cardiac power output including RR interval and NYHA status and aetiology (ischaemic vs dilated cardiomyopathy), F = 4.7, p = .01, R2 = 0.36. Furthermore analyses suggested that gender or medication regimen including therapy with ACE inhibitor and beta blocker was not significant determinants of cardiac power output (p = .26).
Discussion
To the best of our knowledge, this is the first study to investigate the relationship between measures of cardiac autonomic function, peak cardiac power output and haemodynamic response to exercise in chronic heart failure. The major findings suggest moderate strength of the relationship measures of RR and SDNN and those of haemodynamic response to exercise including peak cardiac power output. This finding indicate that cardiac autonomic function is a weak indicator of overall function and pumping capability of the heart in chronic heart failure.
Cardiac power output is a valuable measure of overall function and pumping capability of the heart [Citation11]. It has been independently associated with mortality and exercise capacity in heart failure patients [Citation14,Citation23]. Peak exercise cardiac power output <2 W is a strong predictor of mortality in chronic heart failure [Citation12–14] with heart transplant candidates demonstrating values of <1.5 W [Citation18]. Cardiac power output also appears to correlate with heart failure severity and differentiate well during cardiac restoration using mechanical circulatory support [Citation14,Citation16,Citation17]. In the present study which recruited heart failure patients NYHA class II–IV, peak cardiac power output ranged from 0.92 to 3.9 W, further confirming that it can be used as an objective marker of disease severity in clinical practice.
Previous investigation reported that commonly measured cardiopulmonary exercise testing variables are poor indicators of peak cardiac power in patients with heart failure [Citation24]. Findings from the present study for the first time demonstrate the relationship between RR interval and SDNN with peak cardiac power output. Despite the fact that HRV was suggested a strong predictor of outcomes in heart failure and its measurement can be performed easily [Citation6,Citation7], the evaluation of HRV has not been impeded in the heart failure clinical practice perhaps due to limited potential to improve diagnosis, monitoring or heart failure severity. The present study supports the notion of HRV limited utility to predict cardiac response to exercise.
Chronic heart failure manifests an overactivation of the sympathetic system and parasympathetic withdrawal. This derangement in heart rate variability is further associated with excessive levels of circulating catecholamines [Citation25]. It is expected that sympathetic drive may improve cardiac contractility and response to exercise resulting in higher values of cardiac output, stroke volume, heart rate, blood pressure and cardiac power output. Our results confirm the trend of positive relationship between HRV and exercise heamodynamics. This association may partially be explained by the role of peripheral chemoreceptors [Citation26]. In heart failure, chemoreceptor sensitivity is increased in response to sympathetic overactivity and is associated with improved exercise capacity and measures of heart rate variability as previously documented [Citation26,Citation27].
Present study further suggest that heart rate variability was not significantly correlated with a traditional cardiopulmonary exercise testing variable such as peak oxygen consumption, despite it having been shown to be a strong predictor of mortality and disease severity in heart failure [Citation1]. To some extent this should not come as a surprise considering that oxygen consumption can be influenced by multiple peripheral factors in patients with chronic heart failure [Citation28]. Indeed muscle blood flow in response to exercise stress is compromised in heart failure [Citation29]. A recent study highlights the role of peripheral factors in the context of heart failure such as anaemia, vascular stiffness and intrinsic skeletal muscle abnormalities (i.e. mitochondrial dysfunction) resulting in limited exercise capacity and reduced peak oxygen consumption [Citation30].
In our study the cardiac power increase in response to exercise stress was due to an increase in cardiac output and mean arterial blood pressure. The increase in cardiac output was due to an increase in heart rate and stroke volume. The heart rate response to exercise is blunted in chronic heart failure and is often further suppressed by beta blockers [Citation1]. As a compensatory response, one would expect an increase in stroke volume via the Frank-Starling mechanism. Although stroke volume can increase two-to-three times in response to exercise stress, patients with heart failure demonstrate a reduced cardiac reserve and inability to respond to exercise stress compared with other chronic conditions as we have recently demonstrated [Citation31].
Based on moderate positive relationship between HRV and exercise haemodynamics, it is reasonable to suggest that treatments shown to improve heart rate variability may also improve peak cardiac power output. For example, the use of beta- and aldosterone- receptor blockers and angiotensin-converting enzyme inhibitors are strategies shown to improve not only survival and exercise tolerance but also heart rate variability in chronic heart failure [Citation32–34]. However, their direct effect on haemodynamic response to exercise warrants further investigations.
Limitations
The present study is not without limitations. Although the sample size was moderate it was sufficient to identify significant relationship between measures of heart rate variability and cardiac response to exercise. In the present study we used a non-invasive (rebreathing) method for monitoring cardiac output which requires a patient familiarisation with the rebreathing manoeuvre and potential additional effort during peak exercise. However, the use of gold standard invasive methods (i.e. thermodilution) via the right heart catheterization was not indicated in our patients. Nonetheless, the inert gas rebreathing method has been shown to be valid and reliable for measuring cardiac output in chronic heart failure [Citation14].
Conclusions
The present study is the first to define the relationship between cardiac autonomic function, cardiac power output and haemodynamic response to exercise in chronic heart failure. Although there was a significant positive relationship between the measures of heart rate variability and peak cardiac power output, the strength of this relationship was only moderate. It is therefore reasonable to suggest that cardiac autonomic function is not good indicator of overall function and pumping capability of the heart in chronic heart failure.
Authors’ contribution
Study concept and design: DGJ, GAM, AK; Acquisition of data: NO, DN, AT, JS, KB; Statistical analysis and interpretation of data: AR, NO, DN, DGJ; Drafting of the manuscript: AK, NO, DN, AT. Critical revision of the manuscript for important intellectual content: DAB, PD, PMS, AR, LV, NF, DP, JS, LB, GAM; Administrative, technical, or material support: AK, NO, AT; Study Supervision: DGJ, GAM. All authors critically reviewed the manuscript and approved the final version. DGJ acts as the guarantor and takes responsibility for the content of the manuscript, including the data and analysis.
Acknowledgements
The authors thank the participants for taking part in the research programme. The authors of this manuscript have certified that they comply with the Principles of Ethical Publishing.
Disclosure statement
There are no conflicts of interest to be reported.
Additional information
Funding
References
- Ponikowski P, Voors AA, Anker SD. 2016 ESC Guidelines for the diagnosis and treatment of acute and chronic heart failure: The Task Force for the diagnosis and treatment of acute and chronic heart failure of the European Society of Cardiology (ESC)Developed with the special contribution of the Heart Failure Association (HFA) of the ESC. Eur Heart J. 2016;37:2129–2200.
- Leimbach WN, Wallin BG, Victor RG, et al. Direct evidence from intraneural recordings for increased central sympathetic outflow in patients with heart failure. Circulation 1986;73:913–919.
- Nolan J, Flapan AD, Capewell S, et al. Decreased cardiac parasympathetic activity in chronic heart failure and its relation to left ventricular function. Brit Heart J. 1992;67:482–485.
- Heart rate variability: standards of measurement, physiological interpretation and clinical use. Task Force of the European Society of Cardiology and the North American Society of Pacing and Electrophysiology. Circulation 1996;93:1043–1065.
- Bilchick KC, Fetics B, Djoukeng R, et al. Prognostic value of heart rate variability in chronic congestive heart failure (Veterans Affairs’ Survival Trial of Antiarrhythmic Therapy in Congestive Heart Failure). Am J Cardiol. 2002;90:24–28.
- La Rovere MT, Pinna GD, Maestri R, et al. Short-term heart rate variability strongly predicts sudden cardiac death in chronic heart failure patients. Circulation 2003;107:565–570.
- La Rovere MT, Pinna GD, Maestri R, et al. Autonomic markers and cardiovascular and arrhythmic events in heart failure patients: still a place in prognostication? Data from the GISSI-HF trial. Eur J Heart Fail. 2012;14:1410–1419.
- Nolan J, Batin PD, Andrews R, et al. Prospective study of heart rate variability and mortality in chronic heart failure: results of the United Kingdom heart failure evaluation and assessment of risk trial (UK-heart). Circulation 1998;98:1510–1516.
- Cygankiewicz I, Zareba W, Vazquez R, et al. Risk stratification of mortality in patients with heart failure and left ventricular ejection fraction >35%. Am J Cardiol. 2009;103:1003–1010.
- Musialik-Łydka A, Sredniawa B, Pasyk S. Heart rate variability in heart failure. Kardiol Pol. 2003;58:10–16.
- Tan LB. Cardiac pumping capability and prognosis in heart failure. Lancet. 1986;2:1360–1363.
- Cotter G, Williams SG, Vered Z, et al. Role of cardiac power in heart failure. Curr Opin Cardiol. 2003;18:215–222.
- Williams SG, Cooke GA, Wright DJ, et al. Peak exercise cardiac power output; a direct indicator of cardiac function strongly predictive of prognosis in chronic heart failure. Eur Heart J. 2001;22:1496–1503.
- Lang CC, Karlin P, Haythe J, et al. Peak cardiac power output, measured noninvasively, is a powerful predictor of outcome in chronic heart failure. Circ Heart Fail. 2009;2:33–38.
- Sandercock GR, Bromley P, Brodie DA. Reliability of three commercially available heart rate variability instruments using short-term (5-min) recordings. Clin Physiol Funct Imaging. 2004;24:359–367.
- Jakovljevic DG, George RS, Donovan G, et al. Comparison of cardiac power output and exercise performance in patients with left ventricular assist devices, explanted (recovered) patients, and those with moderate to severe heart failure. Am J Cardiol. 2010;105:1780–1785.
- Jakovljevic DG, Yacoub MH, Schueler S, et al. Left ventricular assist device as a bridge to recovery for patients with advanced heart failure. J Am Coll Cardiol. 2017;69:1924–1933.
- Jakovljevic DG, Nunan D, Donovan G, et al. Comparison of cardiac output determined by different rebreathing methods at rest and at peak exercise. Eur J Appl Physiol. 2008;102:593–599.
- Okwose NC, Zhang J, Chowdhury S, et al. Reproducibility of inert gas rebreathing method to estimate cardiac output at rest and during cardiopulmonary exercise stress testing. Int J Sports Med. 2019;40:125–132.
- Jakovljevic DG, Seferovic PM, Nunan D, et al. Reproducibility of cardiac power output and other cardiopulmonary exercise indices in patients with chronic heart failure. Clin Sci. 2012;122:175–181.
- Jakovljevic DG, Nunan D, Donovan G, et al. Lack of agreement between gas exchange variables measured by two metabolic systems. J Sports Sci Med. 2008;7:15–22.
- Meaney E, Alva F, Moguel R, et al. Formula and nomogram for the sphygmomanometric calculation of the mean arterial pressure. Heart. 2000;84:64–64.
- Mendoza DD, Cooper HA, Panza JA. Cardiac power output predicts mortality across a broad spectrum of patients with acute cardiac disease. Am Heart J. 2007;153:366–370.
- Jakovljevic DG, Birks EJ, George RS, et al. Relationship between peak cardiac pumping capability and selected exercise-derived prognostic indicators in patients treated with left ventricular assist devices. Eur J Heart Fail. 2011;13:992–999.
- Sanderson JE. Heart rate variability in heart failure. Heart Fail Rev. 1998;2:235–244.
- Chua TP, Ponikowski PP, Harrington D, et al. Contribution of peripheral chemoreceptors to ventilation and the effects of their suppression on exercise tolerance in chronic heart failure. Heart. 1996;76:483–489.
- Ponikowski P, Chua TP, Piepoli M, et al. Ventilatory response to exercise correlates with impaired heart rate variability in patients with chronic congestive heart failure. Am J Cardiol. 1998;82:338–344.
- Florea VG, Mareyev VY, Achilov AA, et al. Central and peripheral components of chronic heart failure: determinants of exercise tolerance. Int J Cardiol. 1999;70:51–56.
- Calbet JA, Lundby C. Skeletal muscle vasodilatation during maximal exercise in health and disease. J Physiol. 2012;590:6285–6296.
- Del Torto A, Corrieri N, Vignati C, et al. Contribution of central and peripheral factors at peak exercise in heart failure patients with progressive severity of exercise limitation. Int J Cardiol. 2017;248:252–256.
- McCoy J, Bates M, Eggett C, et al. Pathophysiology of exercise intolerance in chronic diseases: the role of diminished cardiac performance in mitochondrial and heart failure patients. Open Heart. 2017;4:e000632.
- Copie X, Pousset F, Lechat P, et al. Effects of beta-blockade with bisoprolol on heart rate variability in advanced heart failure: analysis of scatterplots of R-R intervals at selected heart rates. Am Heart J. 1996;132:369–375.
- Binkley PF, Haas GJ, Starling RC, et al. Sustained augmentation of parasympathetic tone with angiotensin-converting enzyme inhibition in patients with congestive heart failure. J Am Coll Cardiol. 1993;21:655–661.
- Korkmaz ME, Müderrisoğlu H, Uluçam M, et al. Effects of spironolactone on heart rate variability and left ventricular systolic function in severe ischemic heart failure. Am J Cardiol. 2000;86:649–653.