Abstract
Objectives. Circulating osteocalcin (OC), a marker which is central in bone mineralization, may be involved in the atherosclerotic process and influence the risk of developing cardiovascular disease (CVD). We conducted a systematic review and meta-analysis of published observational evidence, to assess and quantify the associations of circulating OC (total, undercarboxylated, and carboxylated OC) with cardiovascular outcomes (clinical CVD endpoints and intermediate cardiovascular phenotypes).
Design. Relevant studies were identified in a literature search of MEDLINE, EMBASE, and reference lists of relevant studies to March 2019. Mean differences and risk ratios with 95% CIs were aggregated using random-effects models.
Results. Thirty-three observational studies (prospective and retrospective cohort, case-control, and cross-sectional) with data on 21,021 unique participants were eligible. The pooled risk ratio in a comparison of extreme fourths of total OC levels was 0.98 (95% CI 0.89, 1.08) for composite CVD. Circulating total OC levels were significantly lower in patients with cardiovascular conditions compared with those without these conditions −2.58 ng/ml (95% CI −3.85, −1.32; p < .001). Prospective and cross-sectional data showed significant inverse associations between total OC and traits such as aortic or coronary calcification, coronary atherosclerosis or calcification, carotid intima-media thickness, and plaque score. There was limited data on carboxylated and undercarboxylated OC, with no evidence of associations.
Conclusion. Observational evidence generally supports inverse associations of circulating total OC with risk of atherosclerotic outcomes and CVD endpoints; however, the data were mostly based on cross-sectional evaluations. Large-scale prospective data are needed.
Introduction
Cardiovascular disease (CVD) is still the leading cause of global mortality [Citation1]. By 2030, an estimated 23.6 million people will die from CVD [Citation2]. Major risk factors for CVD include a history of diabetes, high blood pressure, raised blood lipids, as well as smoking status [Citation3]. Though these established risk factors explain a large proportion of the risk of CVD, its pathogenesis is still not fully established as it appears other additional factors may be involved. Osteocalcin (OC), a bone matrix protein mainly expressed by osteoblasts [Citation4] and used as a biochemical indicator of bone formation [Citation5], has been proposed as a potential biomarker of CVD risk. Osteocalcin is secreted by osteoblasts in a fully carboxylated form, which is then decarboxylated to a more active biological form [Citation4,Citation6,Citation7]. Circulating blood levels of total OC comprises both carboxylated and undercarboxylated OC. Serum OC has been investigated as a hormone which plays a role in regulating glucose metabolism and fat mass; it promotes insulin sensitivity and secretion by pancreatic β-cells, as well as increases proliferation of the β-cells and stimulates energy metabolism [Citation6]. Reduced circulating levels of OC have been consistently linked with high blood glucose levels, insulin resistance, and type 2 diabetes [Citation6,Citation8–10]. Indeed, it has been discussed that OC may hold potential for the prevention, delay and treatment of obesity and metabolic disorders such as diabetes [Citation8]. Emerging evidence also suggests circulating OC may be involved in CVD development, but the data are sparse and conflicting. A number of studies have shown reduced levels of circulating OC to be associated with adverse cardiovascular outcomes such as arterial calcification, carotid atherosclerosis, increased carotid intima-media thickness (CIMT), and CVD; [Citation11–13] whereas others have not demonstrated any relationships [Citation14,Citation15]. In this context, we performed a systematic review and meta-analysis of all available published observational evidence to clarify and quantify the extent of potential associations of circulating OC (total, uncarboxylated, and carboxylated OC) with (i) clinical CVD outcomes as well as all-cause mortality; and (ii) intermediate cardiovascular traits. We also sought to identify gaps in the existing evidence.
Methods
Data sources and search strategy
This review was conducted using a predefined protocol and in accordance with PRISMA and MOOSE guidelines [Citation16,Citation17]. We searched MEDLINE and EMBASE up to 22 March 2019. The computer-based searches combined terms related to the exposure (e.g. “osteocalcin”) and outcomes (e.g. “cardiovascular disease”, “coronary heart disease”, “atherosclerosis”, “carotid intima-media thickness”, “mortality”) in humans, without any language restriction. Reference lists of selected studies and relevant reviews on the topic were manually scanned for additional publications missed by the original search. Full details on the search strategy are presented in Supplementary appendix 1.
Eligibility criteria
We systematically searched for observational cohort, case-control, or cross-sectional population-based studies that had reported on associations of circulating levels of OC (total OC, undercarboxylated OC, and carboxylated OC) with (i) CVD-related outcomes [composite CVD, coronary heart disease (CHD), stroke, congestive heart failure (CHF), or all-cause mortality]; and (ii) intermediate cardiovascular traits [carotid atherosclerosis, aortic atherosclerosis, coronary artery calcification (CAC), carotid intima-media thickness (CIMT), abdominal aortic calcification (AAC)].
Data extraction and quality assessment
The titles and abstracts of all articles identified by the broad literature search were assessed independently by two reviewers (SKK and SS). Studies that did not meet inclusion criteria were discarded. Full text of selected articles were retrieved and assessed to determine if they met the inclusion criteria. Those studies which met the inclusion criteria were included in the review and data was extracted independently by two reviewers (SKK and SS) using a standard data extraction form. The quality of the studies was assessed independently by both reviewers
When available, data were extracted on: publication date; study design; geographical location; population source; year of baseline survey; sample population; mean/median age at baseline; duration of follow-up (for cohort studies); type of OC; mean/median levels of OC; type of outcome; and reported risk estimates. In the case of multiple publications involving the same study, data were extracted from the most up-to-date study or study with the most comprehensive information was abstracted. We also corresponded with study investigators to provide missing information where relevant. Any discrepancies regarding eligibility of an article were discussed, and consensus reached with a third reviewer (K.K.). For cohort and case-control studies, study quality was assessed based on the nine-star Newcastle–Ottawa Scale (NOS) [Citation18] using three pre-defined domains namely: selection of participants (population representativeness), comparability (adjustment for confounders), and ascertainment of outcomes of interest. The NOS assigns a maximum of four points for selection, two points for comparability, and three points for outcome. Nine points on the NOS reflects the highest study quality. For cross-sectional studies, quality was evaluated using the NOS modified for cross-sectional studies (Supplementary appendix 2(10)). A score of 8 reflected the highest study quality.
Statistical analysis
Mean differences for continuous outcomes and risk ratios for categorical outcomes were used as summary measures across studies. A narrative synthesis was performed for studies that could not be pooled. For data reported as medians, standard errors, ranges, and 95% confidence intervals (CIs), means and standard deviations were estimated using methods as described by Hozo and colleagues [Citation19]. To enable a consistent approach to the meta-analysis, enhance comparability and interpretation of the findings, units of measurements were converted where appropriate and reported study-specific risk ratios (per-unit or standard deviation change, quintiles, or other groupings) were also transformed to involve comparisons between the top quartile and bottom quartile of each study population’s baseline distribution of OC levels, using standard statistical methods. [Citation20,Citation21] described previously. [Citation22–24] To account for the effect of between-study heterogeneity anticipated, we used the inverse variance weighted method to combine summary measures using random-effects models [Citation25]. We evaluated for publication bias using funnel plots and Egger’s regression symmetry tests [Citation26]. All tests were two-tailed and p-values of .05 or less were considered significant. STATA release 14 (Stata Corp, College Station, Texas, USA) was used for all statistical analyses.
Results
Study identification and selection
165 potentially relevant citations were identified. 40 articles were selected for full text evaluation after an initial screen based on titles and abstracts. After detailed assessments, 8 articles were excluded because (i) the outcomes were not relevant to review (n = 5); (ii) the exposure was not relevant (n = 2); and (ii) one article used the same population sample as another study included in the review. The remaining 32 articles based on 33 unique observational studies met all inclusion criteria and were included in the review (; Supplementary appendix 3).
Study characteristics and study quality
shows a summary of the key characteristics of the included. 21,021 unique participants were included in this review. However, not all studies provided relevant data that could be included in the quantitative synthesis. The majority of studies (n = 19) were conducted in Asia (China, Japan, and South Korea); with 10 in Europe (Austria, France, Germany, Italy, The Netherlands, and Spain); 2 in the Pacific (Australia); and 2 in North America (USA). The mean/median baseline age of participants ranged from 49 to 77 years. The majority of studies (n = 20) were cross-sectional in design; 9 prospective cohorts; 2 case-controls; 1 retrospective cohort and 1 prospective case-control. The average follow-up for cohort studies ranged from 0.5 to 10.0 years. For studies that provided relevant data on their recruitment processes, majority of studies (n = 22) reported recruiting patients from healthcare settings, with 7 studies reporting recruitment from a population register. There was considerable variability in study populations which included healthy and community-dwelling participants, post-menopausal women, and patients with pre-existing conditions such as type 2 diabetes, chronic kidney disease, essential hypertension and those at high cardiovascular risk. Among cohort, case-control, and cross-sectional studies, quality score ranged from 3 to 8.
Table 1. Characteristics of studies included in review.
Association of circulating osteocalcin with cardiovascular outcomes
Cohort analysis The pooled risk ratio of 5 cohort studies (3481 participants and 686 events) for composite CVD risk comparing individuals in the top versus bottom fourths of circulating total OC levels, adjusted for several established cardiovascular risk factors was 0.98 (95% CI 0.89, 1.08; p = .669) (). The prospective corresponding risk ratios for CHD and stroke based on results of a single study were 1.06 (95% CI 0.38, 2.93) and 0.65 (95% CI 0.29, 1.46) respectively.
Figure 2. Association of circulating total osteocalcin with cardiovascular outcomes and all-cause mortality. CI: confidence interval (bars); OC: osteocalcin.
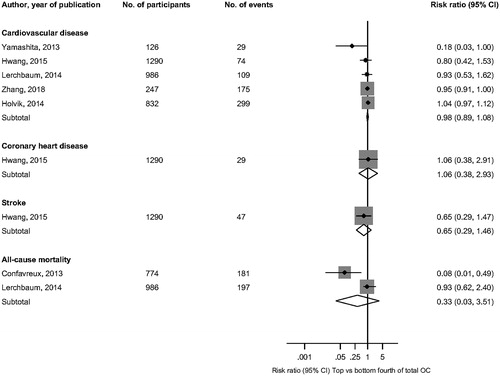
In pooled analysis of 2 prospective studies (1,760 participants and 378 events), the multivariate-adjusted risk ratio for all-cause mortality was 0.33 (95% CI 0.03, 3.51; p = .355) comparing individuals in the top versus bottom fourths of circulating total OC levels. Four studies could not be included in the meta-analysis because of differences in the exposure categories. In analysis adjusted for several conventional risk factors, Yeap and colleagues reported a U-shaped relationship of circulating total OC with CVD mortality and all-cause mortality in men – the risk being increased at both ends of the distribution of OC levels [Citation27]. In a follow-up of the same study that evaluated CHD and stroke outcomes, total OC was not associated with MI or stroke events [Citation15]. In the Ludwigshafen Risk and Cardiovascular Health (LURIC) prospective cohort study [Citation28], findings reported in men showed a U-shaped association of total OC with fatal CVD and all-cause mortality - with increased risk at both ends of the distribution of OC levels. In a prospective case-control study of 102 MI patients and 200 control subjects, total OC was demonstrated to be associated with premature MI at one-year follow-up [Citation29].
In the single prospective cohort study that evaluated the associations between undercarboxylated OC and cardiovascular endpoints, there was no evidence of an association of undercarboxylated OC with CHD or stroke [Citation15].
Cross-sectional analysis Comparing individuals in the top versus bottom fourths of circulating total OC levels, the multivariate-adjusted risk ratio for CHD in pooled analysis of 3 cross-sectional studies (766 participants and 383 events) was 1.20 (95% CI 0.32, 4.50; p = .789).
Association of serum total osteocalcin with intermediate cardiovascular traits
Cohort analysis The pooled risk ratio for aortic calcification in pooled analysis of 2 prospective cohort studies was 0.87 (95% CI 0.76, 0.99; p = .030) comparing individuals in the top versus bottom fourths of circulating total OC levels. In a prospective cohort study that monitored changes in total OC and plaque score in Japanese patients with type 2 diabetes, changes in circulating total OC was inversely associated with changes in plaque score from baseline in analysis adjusted for atherosclerotic risk factors (β = −0.30; p = .047) [Citation30].
Cross-sectional analysis In pooled analysis of 8 studies (3356 participants), the multivariate-adjusted risk ratio for vascular atherosclerosis or calcification when comparing individuals in the top versus bottom fourths of circulating total OC levels was 0.67 (95% CI 0.37, 1.22; p = .187) (). The risk ratio for vascular atherosclerosis or calcification when comparing individuals in the top versus bottom fourths of circulating undercarboxylated OC levels in pooled analysis of 4 studies (762 participants) was 0.76 (95% CI 0.13, 4.63; p = .767) ().
Figure 3. Associations of circulating total and undercarboxylated osteocalcin with intermediate cardiovascular traits. AAC: abdominal aortic calcification; CAC: coronary artery calcification; CI: confidence interval (bars); OC: osteocalcin.
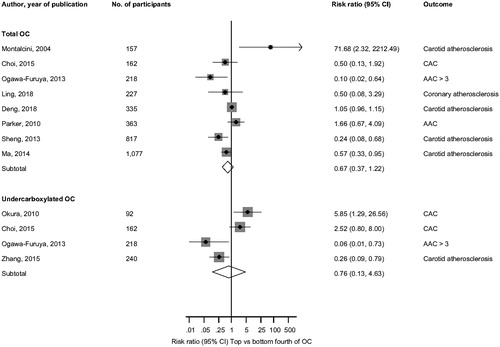
Five studies could not be included in the meta-analysis. Kanazawa and colleagues in their assessment of the associations of serum total OC with atherosclerosis parameters in patients with type 2 diabetes, total OC was significantly and inversely correlated with CIMT in men (r = −0.181; p = .023), but not in women (r = 0.022; p = .803) [Citation31]. In a sample of 817 men and postmenopausal women with type 2 diabetes, serum total OC was independently and inversely associated with CIMT (β = −0.181; r = −0.187; p < .001) [Citation12]. Yang et al. also demonstrated a significant and inverse association between serum total OC and CIMT (β = −0.117; r = −0.107; p < .01) [Citation13]. Kim and colleagues also reported total OC to be significantly and inversely correlated with aortic calcification as measured by the aortic calcium score (r = −0.238; p < .001) [Citation11]. An independent and inverse correlation (β = −0.497; p = .003) was also demonstrated between serum total OC and coronary atherosclerosis index in the study by Bao et al. [Citation32].
Circulating osteocalcin levels in patients with cardiovascular outcomes and traits compared with controls
The pooled random-effects mean difference across 9 studies showed significantly lower circulating levels of total OC −2.58 ng/ml (95% CI −3.85, −1.32; p < .001) in patients with cardiovascular conditions compared to subjects without cardiovascular conditions (). In pooled analysis of 4 studies, there was no significant difference in circulating undercarboxylated OC levels comparing subjects with and without cardiovascular conditions. In a case-control study, circulating carboxylated OC level was significantly lower comparing subjects with and without CAD −0.50 ng/ml (95% CI −0.71, −0.29; p < .001).
Discussion
Summary of findings
Using a systematic and meta-analytical approach, we have summarized all available observational studies that have assessed the associations of circulating levels of OC (total, undercarboxylated, and carboxylated OC) with clinical CVD endpoints and intermediate CVD traits, in an attempt to address the uncertainties in the evidence. In the analysis of hard CVD outcomes, pooled analysis of prospective data showed no evidence of statistically significant associations of total OC with composite CVD, CHD, or stroke endpoints. However, two prospective studies conducted in men demonstrated a U-shaped association of total OC with fatal CVD and all-cause mortality [Citation27,Citation28]. Both low and high levels of total OC were associated with an increased risk of these outcomes. In the evaluation of intermediate cardiovascular traits, prospective data showed significant inverse associations between total OC and traits such as aortic calcification and plaque score. Though the evidence from cross-sectional studies was inconsistent, majority of studies reported inverse, independent, and significant correlations between total OC and outcomes such as CIMT, aortic calcification, and coronary calcification or atherosclerosis. Circulating total OC was significantly lower in patients with cardiovascular conditions compared with those without these conditions. The data was limited for undercarboxylated and carboxylated OC and generally no significant evidence of associations were demonstrated with the outcomes assessed.
Interpretation of findings
The current evidence does not conclusively support a role of circulating OC (particularly total OC) in the pathophysiology of adverse cardiovascular lesions as well as CVD risk. Though some of the findings show that reduced levels of circulating total OC are associated with greater pathological cardiovascular changes, there was evidence to suggest that increased levels of total OC might also be associated with increased risk of these adverse outcomes. Beyond its well-established pro-osteoblastic functions [Citation33], OC has been demonstrated to have endocrine functions [Citation6]. Consistent evidence shows that reduced circulating levels of OC is associated with increased risk of adverse metabolic outcomes such as type 2 diabetes and metabolic syndrome (MetS), which is via its role in influencing insulin secretion, glucose metabolism, insulin sensitivity, fat mass, beta cell proliferation, and energy expenditure [Citation6,Citation34,Citation35]. Though the mechanistic evidence has mostly involved the use of animal models, recent epidemiological and genetic studies suggest the multiple aspects of the biology of OC are similar for both humans and rodents [Citation36,Citation37]. However, the mechanistic evidence linking OC with atherosclerotic CVD is unclear compared with metabolic conditions such as type 2 diabetes; a number of pathways have been proposed. Conditions such as insulin resistance, metabolic syndrome, non-alcoholic fatty liver disease, and type 2 diabetes, which are closed linked to circulating OC, accelerate the progression of atherosclerotic lesions and are strongly linked to the development of atherosclerosis [Citation12]. Osteocalcin may be involved in the calcification process at arterial and valvular sites, leading to reduced elasticity and compliance of the vasculature [Citation38,Citation39]. Osteocalcin may also play a direct role in the atherosclerotic process, as osteoclast-like cells have been identified in atherosclerotic lesions [Citation39]. Whether the different forms of OC may play different roles in the pathophysiology of adverse cardiovascular outcomes is not clear, as the evidence has mostly been limited to circulating total OC. However, there is evidence from animal models suggesting thatundercarboxylated OC, the more active form of OC, may be of more importance in regulating glucose and energy metabolism [Citation6,Citation34,Citation40] and therefore could be implicated in the development of adverse metabolic outcomes. This is not well established as it is not even certain if undercarboxylated OC might be the active form in humans [Citation36].
Implications of findings
The current findings provide further insight on the role of OC beyond its pro-osteoblastic properties as well as its role in glucose and energy metabolism. The clinical use of circulating OC assays has mainly been for monitoring and assessing the effectiveness of antiresorptive therapy in patients with bone conditions characterised by elevated OC levels such as osteoporosis and Paget’s disease [Citation41].
Though some of the findings were inconsistent which impeded meaningful interpretation, the overall results of the quantitative synthesis of the available data does not underscore a potentially protective role of increased circulating total OC levels on the risk of atherosclerotic lesions and CVD outcomes. The inconsistencies in the findings could be related to the small sample sizes employed by majority of studies, different populations used, different OC assay methods employed, and the fact that circulating OC levels are influenced by ethnicity, gender and menopausal status [Citation42]. There is some suggestion from our results that there may be a more evident association in males. In addition to highlighting the lack of consistent evidence on the cardiovascular effects of OC, this review has also identified several gaps in the literature. Compared with the number of cross-sectional study designs (n = 20), only 10 observational cohort studies were identified to have evaluated the associations and these were limited by small sample sizes and populations with pre-existing disease. Furthermore, the majority of studies used assays for total OC, and therefore the role of other forms of OC is far from clear. This observation could be attributed to the fact that circulating total OC can be conveniently measured in large studies using automated immunoassays [Citation43], while assays for undercarboxylated OC are more cumbersome, labour intensive, or less precise [Citation44]. Large-scale prospective studies conducted in general population settings are needed to address the existing research gaps. Thus far, the potential of strategies that raise concentrations of circulating total OC and their value in the prevention or treatment of vascular diseases is not convincing enough. This is a topic which deserves further investigation.
Strengths and limitations
The strengths and limitations of this systematic meta-analysis merit careful consideration. The notable strengths included the comprehensive search strategy which yielded several published studies on the topic. Overall, this review involved over 21,000 participants and evaluated a wide-range of atherosclerotic and CVD outcomes and their relationships with the different forms of circulating OC. We were able to transform reported risk estimates from majority of contributing studies to a consistent comparison (mean differences and top versus bottom fourths) to allow a consistent combination of estimates across studies, therefore obtaining a reliable estimate of the magnitude of the association and enhancing interpretation of the overall findings. We also conducted a detailed quality assessment of eligible studies. Limitations included the inability to fully examine the impact of adjustment for potential confounding factors, because the review was based on variably adjusted data reported in the published literature; heterogeneity could not be explored because of the limited number of studies available for pooling; and some outcomes and risk estimates were inconsistent between studies and therefore could not be pooled.
In conclusion, aggregate observational data do not generally support inverse and independent associations of circulating total OC with risk of atherosclerotic outcomes and CVD endpoints; however, the data were mostly based on cross-sectional evaluations. There are gaps in the existing literature and large-scale prospective cohort studies are needed to explore the nature and potential magnitude of any association of circulating OC with cardiovascular outcomes.
Conflicts of interest/disclosures
KK has acted as a consultant and speaker for Novartis, Novo Nordisk, Sanofi-Aventis, Lilly and Merck Sharp & Dohme. He has received grants in support of investigator and investigator-initiated trials from Novartis, Novo Nordisk, Sanofi-Aventis, Lilly, Pfizer, Boehringer Ingelheim and Merck Sharp & Dohme.
SS has acted as consultant, advisory board member and speaker for Novo Nordisk, Amgen, Sanofi-Aventis, Lilly, Merck Sharp & Dohme, Boehringer Ingelheim, AstraZeneca and Janssen, NAPP and Novartis. He has received research grants Jansen.
SKK has nothing to declare.
Supplemental Material
Download MS Word (111 KB)Acknowledgements
We thank Rebeca Reyes-Garcia, MD, PhD, Bone Metabolic Unit (RETICEF), Endocrinology Division, Hospital Universitario San Cecilio, Av. Dr. Oloriz 16, 18012 Granada, Spain for readily providing data on request.
Additional information
Funding
References
- Roth GA, Johnson C, Abajobir A, et al. Global, Regional, and National Burden of Cardiovascular Diseases for 10 Causes, 1990 to 2015. J Am Coll Cardiol. 2017;70:1–25.
- Global Status Report on Noncommunicable Diseases 2010. Geneva, Switzerland: World Health Organization; 2011; [cited 23 Jan 2018]. Available from: http://www.who.int/nmh/publications/ncd_report_full_en.pdf.
- Wood D. Established and emerging cardiovascular risk factors. Am Heart J. 2001;141:S49–S57.
- Price PA. Gla-containing proteins of bone. Connect Tissue Res. 1989;21:51–57.
- Gerdhem P, Isaksson A, Akesson K, et al. Increased bone density and decreased bone turnover, but no evident alteration of fracture susceptibility in elderly women with diabetes mellitus. Osteoporos Int. 2005;16:1506–1512.
- Lee NK, Sowa H, Hinoi E, et al. Endocrine regulation of energy metabolism by the skeleton. Cell. 2007;130:456–469.
- Hauschka PV, Lian JB, Cole DE, et al. Osteocalcin and matrix Gla protein: vitamin K-dependent proteins in bone. Physiol Rev. 1989;69:990–1047.
- Villafan BJ, Sanchez ES, Munoz-Valle JF. Molecular modulation of osteocalcin and its relevance in diabetes (Review). Int J Mol Med. 2011;28:283–293.
- Starup-Linde J, Eriksen SA, Lykkeboe S, et al. Biochemical markers of bone turnover in diabetes patients–a meta-analysis, and a methodological study on the effects of glucose on bone markers. Osteoporos Int. 2014;25:1697–1708.
- Kunutsor SK, Apekey TA, Laukkanen JA. Association of serum total osteocalcin with type 2 diabetes and intermediate metabolic phenotypes: systematic review and meta-analysis of observational evidence. Eur J Epidemiol. 2015;30:599–614.
- Kim KJ, Kim KM, Park KH, et al. Aortic calcification and bone metabolism: the relationship between aortic calcification, BMD, vertebral fracture, 25-hydroxyvitamin D, and osteocalcin. Calcif Tissue Int. 2012;91:370–378.
- Sheng L, Cao W, Cha B, et al. Serum osteocalcin level and its association with carotid atherosclerosis in patients with type 2 diabetes. Cardiovasc Diabetol. 2013;12:22.
- Yang R, Ma X, Dou J, et al. Relationship between serum osteocalcin levels and carotid intima-media thickness in Chinese postmenopausal women. Menopause. 2013;20:1194–1199.
- Hwang YC, Kang M, Cho IJ, et al. Association between the circulating total osteocalcin level and the development of cardiovascular disease in middle-aged men: a mean 8.7-year longitudinal follow-up study. J Atheroscler Thromb. 2015;22:136–143.
- Yeap BB, Alfonso H, Chubb SA, et al. Proportion of undercarboxylated osteocalcin and serum P1NP predict incidence of myocardial infarction in older men. J Clin Endocrinol Metab. 2015;100:3934–3942.
- Stroup DF, Berlin JA, Morton SC, et al. Meta-analysis of observational studies in epidemiology. JAMA. 2000;283:2008–2012.
- Moher D, Liberati A, Tetzlaff J, et al. Preferred reporting items for systematic reviews and meta-analyses: the PRISMA statement. PLoS Med. 2009;6:e1000097.
- Wells GA, Shea B, O'Connell D, et al. The Newcastle-Ottawa Scale (NOS) for assessing the quality of nonrandomised studies in meta-analyses. 2011; [cited 10 Mar 2015]. Available from: www.ohri.ca/programs/clinical_epidemiology/oxford.asp. http://www.ohri.ca/programs/clinical_epidemiology/oxford.asp.
- Hozo SP, Djulbegovic B, Hozo I. Estimating the mean and variance from the median, range, and the size of a sample. BMC Med Res Methodol. 2005;5:13.
- Chêne G, Thompson SG. Methods for summarizing the risk associations of quantitative variables in epidemiologic studies in a consistent form. Am J Epidemiol. 1996;144:610–621.
- Greenland S, Longnecker MP. Methods for trend estimation from summarized dose-response data, with applications to meta-analysis. Am J Epidemiol. 1992;135:1301–1309.
- Kunutsor SK, Abbasi A, Adler AI. Gamma-glutamyl transferase and risk of type II diabetes: an updated systematic review and dose-response meta-analysis. Ann Epidemiol. 2014;24:809–816.
- Kunutsor SK, Apekey TA, Cheung BM. Gamma-glutamyltransferase and risk of hypertension: a systematic review and dose-response meta-analysis of prospective evidence. J Hypertens. 2015;33:2373–2381.
- Kunutsor SK, Apekey TA, Hemelrijck MV, et al. Gamma glutamyltransferase, alanine aminotransferase and risk of cancer: systematic review and meta-analysis. Int J Cancer. 2015;136:1162–1170.
- DerSimonian R, Laird N. Meta-analysis in clinical trials. Control Clin Trials. 1986;7:177–188.
- Egger M, Davey Smith G, Schneider M, et al. Bias in meta-analysis detected by a simple, graphical test. BMJ. 1997;315:629–634.
- Yeap BB, Chubb SA, Flicker L, et al. Associations of total osteocalcin with all-cause and cardiovascular mortality in older men. The health in men study. Osteoporos Int. 2012;23:599–606.
- Lerchbaum E, Schwetz V, Pilz S, et al. Association of bone turnover markers with mortality in men referred to coronary angiography. Osteoporos Int. 2013;24:1321–1332.
- Goliasch G, Blessberger H, Azar D, et al. Markers of bone metabolism in premature myocardial infarction (</= 40 years of age). Bone. 2011;48:622–626.
- Kanazawa I, Yamaguchi T, Sugimoto T. Relationship between bone biochemical markers versus glucose/lipid metabolism and atherosclerosis; a longitudinal study in type 2 diabetes mellitus. Diabetes Res Clin Pract. 2011;92:393–399.
- Kanazawa I, Yamaguchi T, Yamamoto M, et al. Serum osteocalcin level is associated with glucose metabolism and atherosclerosis parameters in type 2 diabetes mellitus. J Clin Endocrinol Metab. 2009;94:45–49.
- Bao Y, Zhou M, Lu Z, et al. Serum levels of osteocalcin are inversely associated with the metabolic syndrome and the severity of coronary artery disease in Chinese men. Clin Endocrinol (Oxf). 2011;75:196–201.
- Confavreux CB, Levine RL, Karsenty G. A paradigm of integrative physiology, the crosstalk between bone and energy metabolisms. Molecul Cell Endocrinol. 2009;310:21–29.
- Ferron M, McKee MD, Levine RL, et al. Intermittent injections of osteocalcin improve glucose metabolism and prevent type 2 diabetes in mice. Bone. 2012;50:568–575.
- Hinoi E, Gao N, Jung DY, et al. The sympathetic tone mediates leptin's inhibition of insulin secretion by modulating osteocalcin bioactivity. J Cell Biol. 2008;183:1235–1242.
- Ducy P. The role of osteocalcin in the endocrine cross-talk between bone remodelling and energy metabolism. Diabetologia. 2011;54:1291–1297.
- Wei J, Karsenty G. An overview of the metabolic functions of osteocalcin. Curr Osteoporos Rep. 2015;13:180.
- Evrard S, Delanaye P, Kamel S, et al. Vascular calcification: from pathophysiology to biomarkers. Clin Chim Acta. 2015;438:401–414.
- Yamanouchi D, Takei Y, Komori K. Balanced mineralization in the arterial system: possible role of osteoclastogenesis/osteoblastogenesis in abdominal aortic aneurysm and stenotic disease. Circ J. 2012;76:2732–2737.
- Lee NK, Karsenty G. Reciprocal regulation of bone and energy metabolism. TEM. 2008;19:161–166.
- Magni P, Macchi C, Sirtori CR, et al. Osteocalcin as a potential risk biomarker for cardiovascular and metabolic diseases. Clin Chem Lab Med. 2016;54:1579–1587.
- Gundberg CM, Looker AC, Nieman SD, et al. Patterns of osteocalcin and bone specific alkaline phosphatase by age, gender, and race or ethnicity. Bone. 2002;31:703–708.
- Takahashi M, Kushida K, Nagano A, et al. Comparison of the analytical and clinical performance characteristics of an N-MID versus an intact osteocalcin immunoradiometric assay. Clin Chim Acta. 2000;294:67–76.
- Gundberg CM, Nieman SD, Abrams S, et al. Vitamin K status and bone health: an analysis of methods for determination of undercarboxylated osteocalcin. J Clin Endocrinol Metab. 1998;83:3258–3266.