Abstract
Objectives
Although a number of studies compared mortality after transcatheter aortic valve implantation (TAVI) with that after surgical aortic replacement (SAVR) in patients with chronic obstructive pulmonary disease (COPD), no meta-analysis of them has been conducted to date. To determine whether TAVI or SAVR is associated with better postprocedural survival in patients with COPD, a meta-analysis of all studies currently available was performed. Design. To identify all comparative studies of TAVI with SAVR in patients with COPD, PubMed and Web of Science were searched through January 2020. Studies meeting the following criteria were included in the present meta-analysis: the design was an observational comparative study or a randomized controlled trial; the study population was patients with COPD; patients were assigned to TAVI versus SAVR; and outcomes included all-cause mortality. Adjusted (if unavailable, unadjusted) odds or hazard ratios with their confidence intervals (CIs) of mortality for TAVI versus SAVR were extracted from each study. Study-specific estimates were combined in the random-effects model. Results. Six eligible studies with a total of 4771 patients with COPD were identified and included in the present meta-analysis. The meta-analysis indicated significantly lower early (in-hospital or 30-day) mortality after TAVI than after SAVR (odds ratio, 0.69; 95% CI, 0.53–0.90; p = .006) but no significant difference in midterm (1-year to 5-year) mortality between TAVI and SAVR (hazard ratio, 1.07; 95% CI, 0.79–1.44; p = .68). Conclusions. In patients with COPD, TAVI was associated with reduced early mortality, while midterm mortality appeared similar, as compared with SAVR.
Introduction
A large US single-center study [Citation1] indicated that baseline chronic obstructive pulmonary disease (COPD) was associated with an increase of long-term mortality, but not early mortality, after surgical aortic replacement (SAVR). A study from the Spanish national database also reported no association of baseline COPD with increased early mortality after SAVR [Citation2]. Whereas, a meta-analysis of 28 studies showed that baseline COPD was associated with an increase of both early and long-term mortality after transcatheter aortic valve implantation (TAVI) [Citation3]. Although a number of studies compared mortality after TAVI with that after SAVR in patients with COPD, no meta-analysis of them has been conducted to date. In the present article, to determine whether TAVI or SAVR is associated with better postprocedural survival in COPD patients, a meta-analysis of all studies currently available was performed.
Materials and methods
The present meta-analysis was conducted according to the PRISMA [Preferred Reporting Items for Systematic Reviews and Meta-Analyses] recommendations (http://www.prisma-statement.org). To identify all comparative studies of TAVI with SAVR in patients with COPD, PubMed (https://www.ncbi.nlm.nih.gov/pubmed/) and Web of Science (http://www.webofknowledge.com/wos) were searched through January 2020. Search terms included transcatheter; aortic valve; implantation or replacement; and lung disease, pulmonary disease, or respiratory disease. Studies meeting the following criteria were included in the present meta-analysis: the design was an observational comparative study or a randomized controlled trial (RCT); the study population was patients with COPD (defined in each study); patients were assigned to TAVI versus SAVR; and outcomes included all-cause mortality. Adjusted (if unavailable, unadjusted) odds or hazard ratios (ORs/HRs) with their confidence intervals (CIs) of mortality for TAVI versus SAVR were extracted from each study. Study-specific estimates were combined using inverse variance-weighted averages of logarithmic ORs/HRs in the random-effects model. Funnel plot asymmetry (suggesting publication bias) was mathematically evaluated using the linear regression test. All analyses were performed using Review Manager version 5.3 (http://tech.cochrane.org/revman) and Comprehensive Meta-Analysis version 3 (Biostat, Englewood, NJ, USA).
Results
Of 220 potentially relevant publications screened initially, 173 reports were excluded on the basis of the title and/or abstract, and six eligible studies [Citation4–9] with a total of 4771 patients with COPD () were identified through the detailed review of remaining 47 reports and included in the present meta-analysis. The definition of COPD was the International Classification of Diseases, 9th Revision, Clinical Modification (ICD-9-CM) (https://www.cdc.gov/nchs/icd/icd9cm.htm) codes in 2 studies [Citation4,Citation6], the Global Initiative for Chronic Lung disease (GOLD) classification [Citation10] in 2 studies [Citation5,Citation9], and the Society of Thoracic Surgeons (STS) classification (https://www.sts.org/sites/default/files/documents/ACSDTrainingManual_V2-81_July2017.pdf) in 2 studies [Citation7,Citation8]. Only one study [Citation7] was an RCT, and the others [Citation4–6,Citation8,Citation9] were observational comparative studies (including 3 propensity score matched [PSM] studies [Citation4–6]). The follow-up duration was in-hospital in 2 studies [Citation4,Citation6], 1 year in 2 studies [Citation5,Citation8], 2 years in one study [Citation7], and 5 years in one study [Citation9] (). Two different PSM studies were separately conducted by Ando et al. [Citation4] and Kwak et al. [Citation11] from the Nationwide Inpatient Sample (NIS). The former [Citation4], not the latter [Citation11], was included in the present meta-analysis because the number of patients enrolled in the former (2418 patients from the NIS 2011–2014) was more than that in the latter (1428 patients from the NIS 2010–2014).
Table 1. Study design and patient characteristics.
Table 2. Predicted operative mortality, TAVI approach, and relative risk estimate of mortality.
All but one [Citation4] individual studies indicated no significant difference in early (in-hospital or 30-day) mortality between TAVI and SAVR (). The meta-analysis demonstrated significantly lower early mortality after TAVI than after SAVR (OR, 0.69; 95% CI, 0.53–0.90; p = .006; ) but no significant difference in midterm (1-year to 5-year) mortality between TAVI and SAVR (HR, 1.07; 95% CI, 0.79–1.44; p = .68; ). No funnel plot asymmetry for the meta-analysis of early mortality (p = .17) was identified (), which suggested probably no publication bias. Excluding 2 unadjusted studies [Citation8,Citation9] and combining the remaining 4 studies (one RCT [Citation7] and 3 PSM studies [Citation4–6]) indicated still significantly lower early mortality after TAVI than after SAVR (OR, 0.65; 95% CI, 0.49–0.86; p = .003).
Figure 1. Meta-analysis of early mortality after transcatheter aortic valve implantation (TAVI) versus surgical aortic valve replacement (SAVR) in patients with chronic obstructive pulmonary disease (COPD). CI: confidence interval; IV: inverse variance.
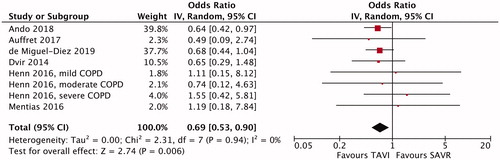
Figure 2. Meta-analysis of midterm mortality after transcatheter aortic valve implantation (TAVI) versus surgical aortic valve replacement (SAVR) in patients with chronic obstructive pulmonary disease (COPD). CI: confidence interval; IV: inverse variance.
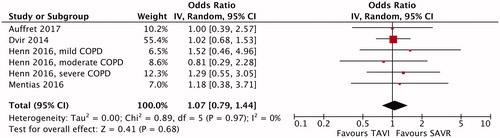
Figure 3. Funnel plot of precisions by logarithmic odds ratio of early mortality after transcatheter aortic valve implantation versus surgical aortic valve replacement in patients with chronic obstructive pulmonary disease.
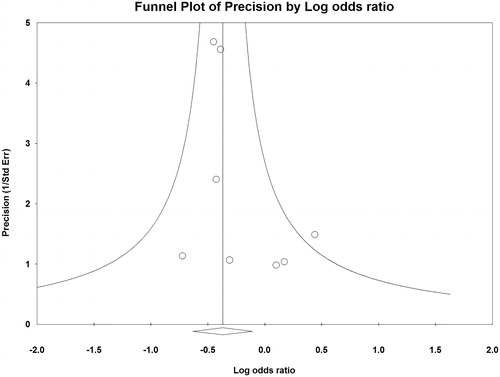
The risk of bias assessed using the Cochrane risk-of-bias tool for RCTs [Citation12] and RoBANS [Risk of Bias Assessment tool for Nonrandomized Studies] [Citation13] was summarized in the Supplementary Table S1, and covariates used for adjustment were listed in Supplementary Table S2.
Discussion
To the best of our knowledge, the present study is the first meta-analysis of 6 studies enrolling a total of 4771 patients with COPD, which suggests that in patients with COPD, TAVI was associated with reduced early mortality, while midterm mortality appeared similar, as compared with SAVR. The below-mentioned lower incidence of postprocedural adverse respiratory events after TAVI than after SAVR in COPD patients may explain the present finding with respect to early mortality. Ando et al. [Citation4] indicated, in their PSM study, that in-hospital pneumonia (OR, 0.41; p < .001), acute respiratory failure (OR, 0.63; p = .002), reintubation (OR, 0.49; p < .001), and tracheostomy (OR, 0.14; p < .001) were less frequent after TAVI than after SAVR. In the PSM study by Auffret et al. [Citation5], the 30-day incidence of prolonged (>24 h) ventilation (excluding reintubation) was lower after TAVI than after SAVR (1.4% versus 8.5%; p = .06). Henn et al. [Citation8] also reported that periprocedural prolonged (>24 h) ventilation was less frequent after TAVI than after SAVR (1.0% versus 12.1%) in patients with moderate/severe COPD. Furthermore, in the study by Mentias et al. [Citation9], the postprocedural incidence of prolonged (>24 h) ventilation was lower (14.8% versus 47.6%; p = .01) and the intubation time was shorter (median 1 day versus 3 days; p < .001) after TAVI than after SAVR.
Baseline COPD may be associated with an increase of long-term mortality, but not early mortality, after SAVR. Gunter et al. [Citation1] showed, in their study with 2379 patients, that all of mild, moderate, and severe COPD was an independent predictor of long-term mortality (multivariate HR for mild COPD, 1.49; p < .001; multivariate HR for moderate COPD, 1.95; p < .001; multivariate HR for severe COPD, 1.95; p < .001), but not in-hospital mortality after SAVR. Also in the study by De Miguel-Díez et al. [Citation2] with 78,223 hospitalizations from the Spanish National Hospital Discharge Database, COPD was not associated with in-hospital mortality after SAVR (6.77% for COPD versus 6.48% for non-COPD; p = .17). Whereas, baseline COPD may be associated with an increase of both early and long-term mortality after TAVI. Liao et al. [Citation3] indicated, in their meta-analysis of 28 studies with a total of 51,530 patients, that COPD was independently associated with increased 30-day (multivariate OR, 1.43; p = .002), 1-year (multivariate HR, 1.15; p = .0002), 2-year (multivariate HR, 1.12; p < .00001), and >2-year mortality (multivariate HR, 1.34; p = .002) after TAVI. The above-mentioned findings (association of COPD with worse early survival after TAVI [Citation1,Citation2], but not after SAVR[Citation3]) of the previous studies appear incompatible with the present results (better early survival after TAVI than after SAVR in COPD patients). Because of the non-randomized design (despite the PSM design [Citation4–6]) of the studies (except for the RCT by Dvir et al. [Citation7]) included in the present meta-analysis, measurable and unmeasurable confounders may have influenced the present findings.
Despite no difference in midterm mortality between TAVI and SAVR in COPD patients indicated in the present meta-analysis, “minimalist” transfemoral (TF) TAVI (performed in the catheterization laboratory under conscious sedation, using transthoracic echocardiography) [Citation14] may improve midterm survival compared with standard TF-TAVI (performed in the hybrid operating room under general anesthesia, using transesophageal echocardiography) in COPD patients. In severe COPD patients undergoing TF-TAVI in the study by Condado et al. [Citation15], minimalist TF-TAVI was independently associated with better 1-year mortality (multivariate HR, 0.28; p = .05), which may be related to evasion of intubation and general anesthesia in minimalist TF-TAVI.
The present results should be construed with caution due to the following limitations. First, the present results were derived from non-randomized studies (except for the RCT by Dvir et al. [Citation7]), where attrition is often worse and inadequately provided, intervention and outcome evaluation are seldom practiced according to standard protocols, and outcomes are rarely blinded [Citation16]. Second, different definitions of COPD were utilized among the studies included in the present meta-analysis. The ICD-9-CM codes (490, 491, 491.0, 491.1, 491.2x, 491.8, 491.9, 492, 492.0, 492.8, 494, 496) were applied in 2 studies [Citation4,Citation6], the GOLD classification (moderate, 50% ≤ forced expiratory volume in 1 sec [FEV1] < 80% predicted; severe, 30% ≤ FEV1 < 50% predicted; very severe, FEV1 <30% predicted) in 2 studies [Citation5,Citation9], and the STS classification (moderate, 50% ≤ FEV1 < 60% of predicted; severe, FEV1 <50% of predicted) in 2 studies [Citation7,Citation8]. This heterogeneity of the COPD definition may bias the present findings. Third, publication bias favoring TAVI may influence the present results. The present statistical evaluation of the funnel plot, however, did not identify its asymmetry, which suggests minimal publication bias. Fourth, the present study was a conventional meta-analysis (extracting data from publications of included studies) but not an individual patient data meta-analysis (seeking the original investigation data for each participant directly from the investigators responsible for included studies) [Citation17].
In conclusion, in patients with COPD, TAVI was associated with reduced early mortality, while midterm mortality appeared similar, as compared with SAVR.
Supplemental Material
Download Zip (26.3 KB)Acknowledgements
No other person(s) made a substantial contribution to the current study.
Disclosure statement
No potential conflict of interest was reported by the author(s).
References
- Gunter RL, Kilgo P, Guyton RA, et al. Impact of preoperative chronic lung disease on survival after surgical aortic valve replacement. Ann Thorac Surg. 2013;96(4):1322–1328.
- De Miguel-Díez J, López-De-Andrés A, Hernández-Barrera V, et al. Chronic obstructive pulmonary disease is not associated with worse in-hospital outcomes after surgical aortic valve replacement in Spain (2001–2015). J Cardiovasc Surg. 2019;60(3):413–421.
- Liao YB, He ZX, Zhao ZG, et al. The relationship between chronic obstructive pulmonary disease and transcatheter aortic valve implantation-A systematic review and meta-analysis. Catheter Cardiovasc Interv. 2016;87(Suppl 1):570–578.
- Ando T, Adegbala O, Akintoye E, et al. Is transcatheter aortic valve replacement better than surgical aortic valve replacement in patients with chronic obstructive pulmonary disease? A nationwide inpatient sample analysis. J Am Heart Assoc. 2018;7:e008408.
- Auffret V, Becerra Munoz V, Loirat A, et al. Transcatheter aortic valve implantation versus surgical aortic valve replacement in lower-surgical-risk patients with chronic obstructive pulmonary disease. Am J Cardiol. 2017;120(10):1863–1868.
- de Miguel-Diez J, López-de-Andrés A, Hernández-Barrera V, et al. Impact of COPD on outcomes in hospitalized patients treated with transcatheter aortic valve implantation or surgical aortic valve replacement in Spain. Catheter Cardiovasc Interv. 2020;95:339–347.
- Dvir D, Waksman R, Barbash IM, et al. Outcomes of patients with chronic lung disease and severe aortic stenosis treated with transcatheter versus surgical aortic valve replacement or standard therapy: insights from the PARTNER trial (placement of AoRTic TraNscathetER Valve). J Am Coll Cardiol. 2014;63(3):269–279.
- Henn MC, Zajarias A, Lindman BR, et al. Preoperative pulmonary function tests predict mortality after surgical or transcatheter aortic valve replacement. J Thorac Cardiovasc Surg. 2016;151(2):578–585, 586. e1–e2.
- Mentias A, Faza NN, Raza MQ, et al. Management of symptomatic severe aortic stenosis in patient with very severe chronic obstructive pulmonary disease. Semin Thorac Cardiovasc Surg. 2016;28(4):783–790.
- Vestbo J, Hurd SS, Agustí AG, et al. Global strategy for the diagnosis, management, and prevention of chronic obstructive pulmonary disease: GOLD executive summary. Am J Respir Crit Care Med. 2013;187(4):347–365.
- Kwak MJ, Bhise V, Warner MT, et al. National trend of utilization, clinical and economic outcomes of transcatheter aortic valve replacement among patients with chronic obstructive pulmonary disease. Curr Med Res Opin. 2019;35(8):1321–1329.
- Higgins JPT, Altman DG, Sterne JAC, et al. Chapter 8: assessing risk of bias in included studies. In: Green S, editors. Cochrane Handbook for Systematic Reviews of Interventions Version 5.1.0 updated March 2011. The Cochrane Collaboration; 2011; [cited 2020 Dec 17]. Available from www.cochrane-handbook.org.
- Kim SY, Park JE, Lee YJ, et al. Testing a tool for assessing the risk of bias for nonrandomized studies showed moderate reliability and promising validity. J Clin Epidemiol. 2013;66(4):408–414.
- Babaliaros V, Devireddy C, Lerakis S, et al. Comparison of transfemoral transcatheter aortic valve replacement performed in the catheterization laboratory (minimalist approach) versus hybrid operating room (standard approach): outcomes and cost analysis. JACC Cardiovasc Interv. 2014;7(8):898–904.
- Condado JF, Haider MN, Lerakis S, et al. Does minimalist transfemoral transcatheter aortic valve replacement produce better survival in patients with severe chronic obstructive pulmonary disease? Cathet Cardiovasc Intervent. 2017;89(4):775–780.
- Reeves BC, Deeks JJ, Higgins JPT, et al. Chapter 13: including nonrandomized studies. In: Higgins JP, Green S, editors. Cochrane Handbook for Systematic Reviews of Interventions Version 5.1.0 updated March 2011. The Cochrane Collaboration; 2011; [cited 2020 Dec 17]. Available from www.cochrane-handbook.org.
- Stewart LA, Tierney JF, Clarke M. Chapter 18: reviews of individual patient data. In: Higgins JP, Green S, editors. Cochrane Handbook for Systematic Reviews of Interventions Version 5.1.0 updated March 2011. The Cochrane Collaboration; 2011; [cited 2020 Dec 17]. Available from www.cochrane-handbook.org.