ABSTRACT
Attachment theory suggests that interindividual differences in attachment security versus insecurity (anxiety and avoidance) contribute to the ways in which people perceive social emotional signals, particularly from the human face. Among different facial features, eye gaze conveys crucial information for social interaction, with a straight gaze triggering different cognitive and emotional processes as compared to an averted gaze. It remains unknown, however, how interindividual differences in attachment associate with early face encoding in the context of a straight versus averted gaze. Using electroencephalography (EEG) and recording event-related potentials (ERPs), specifically the N170 component, the present study (N = 50 healthy adults) measured how the characteristics of attachment anxiety and avoidance relate to the encoding of faces with respect to gaze direction and head orientation. Our findings reveal a significant relationship between gaze direction (irrespective of head orientation) and attachment anxiety on the interhemispheric (i.e. right) asymmetry of the N170 and thus provide evidence for an association between attachment anxiety and eye gaze processing during early visual face encoding.
Introduction
Attachment constitutes a basic human need for survival in early life. It furthermore importantly influences socioemotional and cognitive child development and maturation of corresponding brain circuits (Callaghan & Tottenham, Citation2016). Through influencing the initiation and maintenance of social bonds throughout the life span, attachment is also linked to mental and physical health (Debbane et al., Citation2016; Fonagy & Luyten, Citation2009). Interest in better understanding the biological and particularly neural basis of individual differences in attachment has therefore steadily grown (Long et al., Citation2020).
Three main attachment orientations are usually distinguished: secure, insecure-ambivalent/-resistant (hereafter called anxious – AX), and insecure-dismissive (hereafter called avoidant – AV). Secure attachment is thought to arise through early interactions with available and sensitive caregivers that promptly and appropriately respond to children’s needs and is associated with positive mental representations of the self and others. Conversely, AX is linked with early experiences of inconsistent/unpredictable caregivers, leading to a strong need for closeness, worry about relationships and fear of being abandoned/rejected. Finally, learned through early interactions with insensitive/unresponsive caregivers, AV is associated with mental representations of others as unavailable, yielding social interactions to be generally encoded as less rewarding or even a source of additional distress (Mikulincer & Shaver, Citation2007b; Vrticka et al., Citation2012). There is increasing evidence that individual differences in secure, anxious, and avoidant attachment can be related to dissociable patterns of brain anatomy and activity, the latter manifesting particularly during social information processing, including the encoding of facial expressions (Long et al., Citation2020).
Among all facial features, eye perception is critical during social interactions. When initiating social interactions, humans rely on information from other people’s faces and particularly the eyes and gaze direction. A straight gaze, for instance, allows for synchronisation between individuals in terms of mutual attention (Patterson, Citation1982). Conversely, an averted gaze is linked to the induction of an automatic shift of the observer’s spatial attention in the observed gaze direction – that is, towards the surrounding environment (George & Conty, Citation2008). The ability to quickly encode gaze direction, even if the observer’s head is at an angle, is therefore a vital mechanism for human social interactions.
Gaze is a central modality through which parents signal their availability, establish mutual engagement (straight gaze) and initiate regulation of infant arousal (Beebe & Steele, Citation2013; Slee, Citation1984). Infants are highly sensitive to their mothers’ gaze, especially straight gaze, and begin to join in mutual gaze as early as 3 months (Stern, Citation1974). It is generally understood that eye-to-eye contact is an important determinant of the quality of infant-caregiver attachment through its relationship to infant-caregiver synchrony, which is likely mediated by oxytocin (Blehar et al., Citation1977; Guastella et al., Citation2008). Therefore, early experiences of mutual gaze are critical to yield a functional adult gaze perception (Lewis et al., Citation1992; Ostrovsky et al., Citation2006; Senju et al., Citation2015). While the above clearly implies links between gaze and socioemotional development, particularly in the early postnatal months – and thus likely also mechanisms associated with attachment–, surprisingly few studies are available on the association between attachment and gaze processing.
Early neural encoding of gaze direction may be assessed at a millisecond (ms) scale using electroencephalography (EEG), specifically, event-related potentials (ERPs). Researchers studying face processing with EEG have focused their investigations mostly on the well-known N170 component. This face-sensitive component, which arises across occipitotemporal electrodes about 170 ms after stimulus onset, is generally assumed to reflect the initial stage of structural face encoding (Bentin et al., Citation1996; Rossion et al., Citation2003). This structural encoding is usually biased, with the right hemisphere N170 taking precedence over the left hemisphere N170. The right hemisphere is critical in face perception (Hillger & Koenig, Citation1991) because it is involved in the extraction of global information from faces, the latter assumed to be more important than the representation of local feature information (Sergent, Citation1982), a phenomenon being crucial for the individuation of faces. Conversely, a left hemisphere asymmetry of the N170 is usually detected during word recognition, where local feature information is more important than global information (e.g. Emmorey et al., Citation2017; Maurer, Citation2008; Mercure et al., Citation2008, Citation2011). The N170 hemispheric asymmetry measure has been extensively investigated in face perception (Rossion et al., Citation2000; Scott, Citation2006). Prior studies proposed different interpretations of this asymmetry, specifically regarding the underlying difference in terms of face processing (Rossion et al., Citation2000), and a right-hemispheric advantage for configural/global processing of the whole face and the left hemisphere dominance for feature/local processing appears to emerge. Thus, a reduced or opposite interhemispheric (e.g. left hemispheric) asymmetry may be observed especially when local features are more important than global features, like, in word recognition (Emmorey et al., Citation2017; Maurer, Citation2008; Mercure et al., Citation2008, Citation2011). In sum, a face-selective representation is already generated at 170 ms after stimulus onset, primarily in the right hemisphere and when faces are integrated as a global percept (Yovel et al., Citation2003) rather than from local features (Tanaka & Farah, Citation1993).
Whether gaze direction impacts the N170 remains controversial. While the N170 is sensitive to eyes as such (Itier & Batty, Citation2009), studies focusing on the N170 and gaze direction reported contradictory results. Whereas some researchers failed to find any N170 differences between straight and averted gaze (Burra et al., Citation2018; Latinus et al., Citation2015; Taylor et al., Citation2001), others report a larger N170 (or M170 as its magnetoencephalographic (MEG) counterpart) for an averted versus straight gaze using heads either oriented frontally (Sato et al., Citation2008; Watanabe et al., Citation2002) or deviated to the side (Burra et al., Citation2017). Thus, EEG and MEG studies in human adults have so far failed to consistently confirm the role of gaze direction during face encoding as reflected by the N170 (for a comment about this inconsistency, see Burra et al., Citation2019).
One way of addressing these inconsistent N170 results may be to take interindividual differences into account. In so doing, one promising interindividual difference variable may be attachment. The possible role of individual differences in attachment in relation to gaze direction processing has recently drawn increasing interest. Two behavioural studies investigated the relation between AX and the detection of gaze direction (Burra et al., Citation2019) or the physiological response to open or closed eyes (Prinsen et al., Citation2019). Prior EEG studies, furthermore, revealed that interindividual differences in attachment were associated with the neural processing of emotional stimuli, with several studies reporting effects at the N170 face encoding component (Dan & Raz, Citation2012; Fraedrich et al., Citation2010; Zhang et al., Citation2008; Zilber et al., Citation2007). Findings usually point to an increased N170 amplitude towards faces (especially with a negative emotional expression) for insecurely (versus securely) attached participants, which is interpreted as possibly reflecting altered saliency of face stimuli. More generally, early experience of gaze was reported to be critical to yield a gaze perception dissociation at the N170 by a study comparing neural activity in infants to sighted versus blind mothers (Vernetti et al., Citation2018). Although the above literature suggests that individual differences in attachment may be associated with neural responses to faces – and particularly the N170 component –, we are unaware of any literature pertaining to the question of gaze direction perception, in combination with head orientation, measured by the N170 (measure of N170 during woman-child gaze interaction, see Cecchini et al., Citation2015).
To measure the relationship between attachment and eye gaze detection, we employed an adapted version of a previous study that assessed electrophysiological measures and gaze direction (Burra et al., Citation2017). Besides eyes gaze, head orientation is another important factor in social attention (Wollaston, Citation1824), and gaze and head orientation are known to interact within a behavioral effect, the stare-in-the crowd effect (Conty et al., Citation2006; Senju et al., Citation2005), as well as a better memorizing of straight gaze (Vuilleumier et al., Citation2005). Furthermore, the N170 is enlarged for straight gaze when the head is deviated as compared to frontal (Conty et al., Citation2007; Itier et al., Citation2007), despite EEG seeming to be less sensitive to this interaction than MEG (Burra et al., Citation2017). We therefore manipulated both eye gaze direction and hear orientation in this study.
We expected to replicate one aspect of the results that were measured by the previous study across the entire sample of participants, namely a main effect of gaze following the N170, and an interaction of gaze direction by head orientation around 170 ms. Predictions were more difficult, however, regarding individual differences in attachment. We are only aware of few extant studies that tested for the association between attachment and gaze detection, particularly in the context of the N170, and these usually did not distinguish between AX and AV (if so, rather attachment security versus insecurity). According to the two-dimensional spectrum of emotion regulation capacities reflected by the employed secondary attachment strategies for AX and AV, respectively (Mikulincer & Shaver, Citation2007a), AX is generally associated with a heightened response to affective stimuli (i.e. hyperactivation), while AV is linked to a decreased response to affective expressions (i.e. deactivation). However, it may be that highly relevant social signals, like a straight gaze, convey various kinds of social intents and might trigger a more important facial encoding, which could be assess using the N170 hemispheric asymmetry. A measure for the difference between the left and the right N170 can thus provide an important index of face processing through neural correlates (Lopes et al., Citation2011; Onitsuka et al., Citation2009). This measure might be important because as mentioned above, anxiously attached individuals preferentially use hyperactive strategies, so they tend to heighten the influence of attachment-related stimuli due to their hypersensitivity to social clues (Mikulincer & Shaver, Citation2007c). In the same vein, anxiously attached females devote more cognitive resources to the configural processing of infant faces (Ma et al., Citation2017), in line with the idea that anxiously (as compared to securely) attached individuals are more responsive to emotional facial signals (Donges et al., Citation2012; Vrtička et al., Citation2008). However, how this population processes faces with different gaze directions and head orientations is unknown.
Methods
Participants
Fifty healthy right-handed psychology students (24 males, age range 18–32, mean = 21.4, SD = 2.74) were recruited for the present investigation, which was approved by the ethics committee of our Department of Psychology in accordance with the Declaration of Helsinki. All participants provided written informed consent and participated in this experiment for class credit.
Attachment questionnaire
To assess interindividual variation in attachment following the experimental procedure, participants were asked to complete the Relationship Scales Questionnaire (RSQ) (Griffin & Bartholomew, Citation1994; French version by Guédeney et al., Citation2010). According to the coding method of Simpson et al. (Citation1992), one score for AX based on 5 items and one score for AV based on 8 items were calculated per participant. Reliability (Cronbach’s alpha) of the two scales was α = .69 for AX and α = .66 for AV. For more details, please refer to the supplement.
Materials and apparatus
The experiment was conducted on a 17-inch (1280 x 1024 pixels; 60 Hz refresh rate) LCD monitor. Stimulus presentation and behavioural recording were controlled by MATLAB using the Psychophysics Toolbox v.3 extension (Brainard, Citation1997; Kleiner et al., Citation2007). Our stimuli were identical to those used by (Burra et al., Citation2017; George et al., Citation2001), comprising the faces of 24 individuals (12 female), with some improvements. First, to prevent the task being completed through the use of external and not facial features, we cropped the face outlines. Critically, all images were equated for contrast, mean luminance and spatial frequency using the SHINE toolbox (Willenbockel et al., Citation2010), with. a pixel intensity of 0.19 (SD = 0.0008) and the root mean square contrast of 0.43 (SD = 0.0008). For each individual face identity, there were four distinct conditions of eye direction (directly at the camera/observer or averted by 30°) and head orientation (frontal or rotated/deviated by 30° from the camera/observer). Right and left deviation stimulus versions were obtained by mirror imaging. We also included phase-scrambled stimuli. For more details, please refer to the supplement.
Procedure
Participants were seated 85 cm from the monitor in a dimly lit room. They were informed that the purpose of the experiment was to measure their speed and accuracy in a gender discrimination task. The experiment was split into two blocks of 240 trials each, with each block divided into five sub-blocks of 48 trials, for a total of 96 trials per condition. All combinations were randomised within each block.
Each trial began by presenting a fixation cross for a random duration of 500–1000 ms followed by the face presentation for 200 ms. A blank screen then appeared until the participants responded. They were required to answer as quickly and accurately as possible. In the case of a wrong answer, feedback was displayed in the centre of the screen, reading (in French) mauvaise réponse (incorrect response). A black screen was subsequently displayed for 1000 ms before the next trial (see ).
Figure 1. (a) Experimental conditions. Eye gaze direction could be straight (direct) or averted, and head orientation could be frontal or deviated. (b) Trial sequence. After a jittered fixation cross, a face was presented, followed by a blank screen until the response. Afterward, another blank screen was displayed.
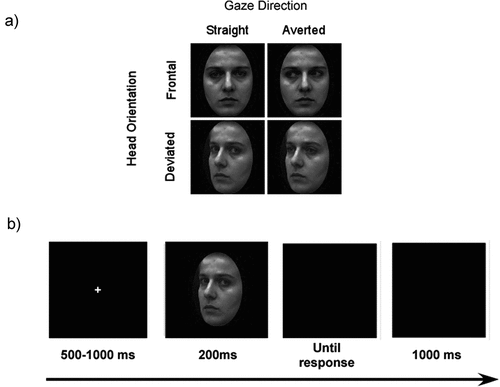
EEG recording and analysis
A Biosemi (Amsterdam, the Netherlands) ActiveTwo amplifier system AD-Box with 64 active AG/AgCL electrodes sampled at 1024 Hz was used. The international 10–20 electrode montage system was implemented to determine scalp sites, with common mode sense (CMS) and driven right leg (DRL) used as reference channels. In addition, we used the voltage difference between two horizontal electrooculograms’ (HEOG) electrodes, fixed at the outer canthi sides of both eyes, to detect horizontal eye movements and two vertical electrooculograms’ (VEOG) electrodes to detect vertical eye movements or blink. Using BrainVision Analyzer 2.1 (BrainProduct), we filtered our data to 0.1–30 Hz using a Butterworth zero phase filter. To remove eye-blink artefacts, we used independent component analysis (ICA). ICA components reflecting eye-blinks or saccade artefacts were manually identified as reflecting ocular noise on the basis of their temporal profiles and scalp topography (M = 1.2, SD = 0.57 min = 1, max = 3). To remove noise components, they were multiplied by zero and the data were then remixed into channel data. Off-line, the data were systematically re-referenced to the common average reference. Trials corresponding to incorrect behavioural performance were eliminated from the analysis, and signals were divided into epochs of 550 ms (200–800 ms). After artefact exclusion, a baseline correction (−200 ms to stimulus onset) was performed. Automatic trial exclusion occurred in the case of a minimum and maximum allowed amplitude of ±80 µV. Moreover, we removed trials with saccades larger than 40 µV (HEOG) that were associated with eye movements during stimulus presentation or with blinking from −200 to 200 ms after stimulus onset at 60 µV (VEOG). When an electrode’s signal was noisy during the entire recording, we used a spline interpolation technique (Order 4, Degree 10) to replace the electrode signal. No interpolation correction was used for the occipital-temporal electrodes, and no more than 2% of electrodes were corrected. On average, 15% of trials were removed from the data. The trials did not differ among experimental conditions (see supplement).
Statistical analyses
Behaviour
We first calculated AX and AV mean scores and their interrelations (see ). For statistical analyses, we inspected response times (RT in millisecond) and accuracy (ACC; as a score between 0 and 1) as well as all AX and AV scores for their distribution and the presence of outliers. RT, AX and AV were left skewed for some scores, so a square root transformation was performed. ACC was also particularly skewed, so an arcsin transformation was performed. This procedure markedly improved data distributions. For the sake of clarity, all statistical analyses were subsequently computed with the transformed values, but we will refer to the untransformed data throughout the manuscript. RT and ACC were first analysed with a 2 × 2 (head orientation; eye gaze) repeated measures analysis of variance (rANOVA).
Table 1. Mean scores (with standard deviation (SD) and min/max) and correlation between attachment questionnaire scores.
ERP: N170
Analyses were performed on the mean amplitude of N170, which was extracted during its maximal occurrence, i.e. at 130–200 ms (i.e. ±35 ms around the peak of the overall component), the time window typically used to investigate these ERPs in studies involving eye gaze (Burra et al., Citation2017; Carrick et al., Citation2007; Conty et al., Citation2007; Myllyneva & Hietanen, Citation2015; Naples et al., Citation2017; Senju & Hasegawa, Citation2005). An additional analysis was then also conducted at a slightly later time window between 190 and 230 ms (Burra et al., Citation2017). The anatomical sites were based on the electrodes of maximal activity during these intervals. We merged the signal of PO7, P7, and P9 (left hemisphere) and the signal of P8, PO8, and P10 (right hemisphere) to obtain two parieto-occipital clusters () consistent with previous studies. Therefore, the N170 was first analysed with a 2 × 2× 2 (hemisphere; head orientation; eye gaze) rANOVA. Despite two conditions not being normally distributed, due mainly to two outliers, we decided to keep the outliers in the overall analysis because their exclusion did not modify the main results of the analyses (see supplement).
Figure 2. N170 for straight and averted gaze (N = 50). A) The time course of the overall mean of the ERP across all participants is shown on the typical left (P7, P9, PO7) and right (P8, P10, PO8) occipitotemporal clusters for the straight (in black) and averted (in grey) gaze conditions in the frontal head direction (solid) and deviated head direction (dotted). B) From 130–200ms, there was a small but reliable interaction effect of head orientation and gaze direction. Error bars represent 1 SEM.
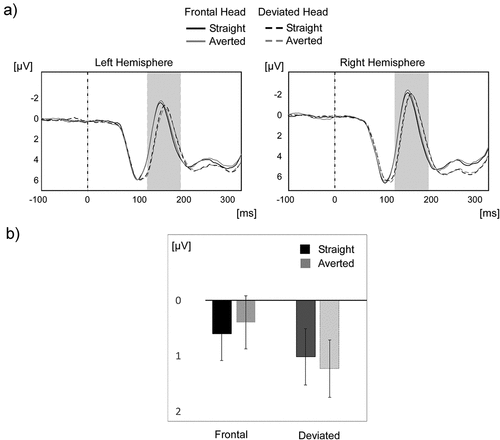
We included the results of different other analyses performed on other later ERPs (EPN, P300, and LPP) in the supplement. None of them revealed any significant effects.
Attachment analysis
Finally, interindividual variation in attachment (AX and AV scores from the RSQ) were added as covariates and allowed to simply interact with the experimental factors for the behavioural and ERP data. AX and AV scores were centered (z-scored) for statistical analyses. To illustrate interactions between experimental factors and questionnaire scores, means were estimated at ±1 standard deviation from the mean of the questionnaire score of interest.
Control analysis
We repeated all above calculations by adding participant gender and age. The observed patterns remained largely unchanged (see supplement).
Results
Questionnaire
summarises the mean scores of AX and AV (from the RSQ). There was no significant correlation between the two scores.
Behavioural data
Response times and accuracy
Two 2 × 2 factorial (head direction: frontal, deviated; gaze direction: straight, averted) rANOVAs were performed, one on the square root transformed individual median RT and one on ACC.
The rANOVA on RT revealed a main effect of head orientation, F(1,49) = 27.11, p < 0.001, η2p = 0.36, and an interaction of head orientation and gaze direction, F(1,49) = 6.66, p = 0.012, η2p = 0.12. Participants were significantly faster in categorising the gender of frontally oriented faces with a straight (M = 567, SD=77 ms) versus an averted gaze (M=575, SD=82 ms), t(49) = 2.586, p < 0.006, Cohen’s d = 0.36. This difference failed to reach significance in the deviated head orientation condition (p > 0.7).
The same rANOVA was performed on transformed ACC. Here, we observed a significant main effect of gaze direction on gender identification ACC, F(1,49) = 4.32, p < .042, η2p = 0.08. Participants were more accurate at categorising the gender of faces with a straight (M = .94, SD = 0.03) versus an averted gaze (M = .93, SD = 0.04) irrespective of head orientation.
Response times, percentage error rates, and personality
We failed to observe any covariance of attachment (AX and/or AV) and gender categorisation behavioural measures (RT and ACC).
N170: 130–200 ms
Our previous study revealed an interaction effect of head orientation and gaze direction (but using MEG instead of EEG). As depicted in , using the mean amplitude of the two parieto-occipital clusters around the peak of the N170, we found a main effect of head orientation on the N170, F(1,49) = 24.99, p < 0.001, η2p = 0.33, with a more negative N170 for frontal (M = 0.49 μv, SD = 0.56) versus deviated head orientation (M = 1.12, SD = 0.57 μv). Critically, we observed an interaction between head orientation and gaze direction, F(1,49) = 7.99, p < 0.007, η2p = 0.14. Also, the N170 amplitude was more negative in the averted (M = 0.39, SD = 3.45) versus the straight gaze condition (M = 0.6, SD = 3.45) for a frontal head orientation, t(49) = 2.11, p = 0.04 , d = 0.299, and significantly more negative in the straight (M = 1.01, SD = 3.66) versus the averted gaze condition (M = 1.23, SD = 3.69) for a deviated head orientation, t(49) = 2.08, p = 0.043, d = 0.295. For these two multiple-paired t-tests, a false discovery rate (FDR) correction was implemented using the method of Benjamin and Hochberg (Citation1995). No other main or interaction effects reached significance.
N170 and attachment
We subsequently recomputed the 2 × 2 × 2 rANOVAs for the N170 by adding the two measures of attachment (AX and AV) as covariates.
A significant relationship emerged for the interaction between hemisphere, gaze direction and AX, F(1,47) = 7.26, p < 0.010, η2p = 0.134. No such effect was present for AV, F(1,47)=1.45, p = 0.23, η²p = 0.030. No other interactions with the covariates and the other factors reached significance. Because the significant interaction contained the two factors gaze direction and hemisphere, it was followed up to further investigate the underlying pattern. To do so, estimates from the rANOVA were extracted at the AX scores ± 1 standard deviation from the mean and plotted as right hemisphere asymmetry (interhemispheric difference; left minus right N170 amplitude) for each gaze direction (). This procedure revealed that participants scoring low on AX exhibited a more significant hemispheric asymmetry for averted versus straight gaze direction, whereas participants scoring high on AX showed a tendency for an opposite pattern. Finally, this difference in gaze direction sensitivity reflected by the hemispheric asymmetry for a straight versus an averted gaze direction (irrespective of head orientation) was computed for each participant and correlated to their AX score. This measure revealed a strong positive correlation between the interhemispheric asymmetry of a straight versus an averted gaze direction and AX: rs(50) = .41, p < 0.003, R2 = 0.152 ().
Figure 3. Illustration of the N170 amplitudes for different eye gaze directions in the left and right hemispheres for participants with low AX (in panel a) and high AX (in panel b). The black lines represent straight gaze N170 amplitudes in the left and right hemispheres, and the grey lines represent averted gaze N170 amplitudes. Depicted are the estimated means of the respective 2×2 rANOVAs. Interhemispheric asymmetry for straight and averted N170 conditions is shown in panel c. A larger inter-hemispheric asymmetry (an increased involvement of the right hemisphere) means a larger involvement of holistic processing, while a lack of inter-hemispheric asymmetry means lower involvement of holistic processing, that is a larger involvement of analytic processing. Finally, in panel d, the correlation between the difference in inter-hemispheric asymmetry between straight versus averted N170 amplitudes and AX is depicted. While participants scoring low on AX showed a tendency to more holistically perceive an averted over a straight gaze direction, participants scoring high on AX displayed the opposite tendency. Error bars represent 1 SEM.
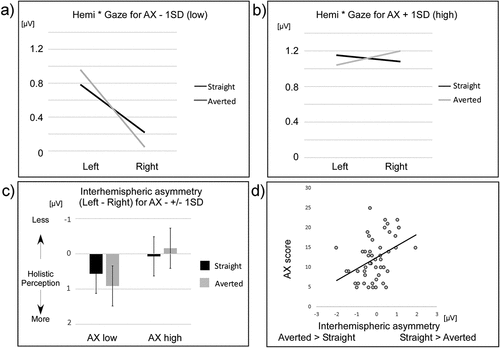
Discussion
The present study investigated how eye gaze direction associates with early face encoding at the N170 in relation to an aspect of individual personality, specifically attachment. To do so, we used pictures of faces with their head orientations and gaze directions manipulated and an attachment self-report (RSQ). Our results replicate previous behavioural and electrophysiological research by revealing a head orientation by gaze direction interaction on the N170 across all participants (irrespective of attachment orientation). Furthermore, we observed an interaction between hemisphere and gaze direction and attachment anxiety (AX) regardless of head orientation on the N170. Such interhemispheric asymmetry (i.e. the difference between the left and the right N170 amplitude) is a marker of early perceptual processing (Rossion et al., Citation2000) and might be objectivated as the ratio of left minus right N170 activity (Onitsuka et al., Citation2009; Lopes, Citation2011). Critically, the interhemispheric asymmetry for a straight versus an averted gaze varied as a function of AX. For participants scoring lower on AX it was more pronounced and showed the directionality averted > straight, whereas for participants scoring higher on AX it was less pronounced and showed the directionality straight > averted. These findings suggest a role of AX in the early neural encoding of faces and particularly eye gaze direction (but not head orientation). However, opposite to our prediction, we measured a low configural/global processing in participants high in AX as compared to participants low in AX.
The EEG results focusing on the N170 also replicated and extended previously reported results. In addition to a significant sensitivity of the N170 to head orientation, we were able to establish that the N170 was sensitive to both head orientation and gaze direction as reported in a previous study, as well as to gaze direction only at the offset of the N170 (Burra et al., Citation2017). Despite the gaze direction effect at the offset of the N170 being highly significant, we should acknowledge that the interaction of head orientation and gaze direction during the N170 failed to highlight a reliable difference between straight and averted gaze in frontal or deviated head direction conditions. However, the question of whether the N170 is modulated by gaze direction still remains controversial, mainly due to inconsistent results in past studies (Burra et al., Citation2017, Citation2018; Latinus et al., Citation2015; Sato et al., Citation2008; Taylor et al., Citation2001; Watanabe et al., Citation2002). Among other parameters, like task-demand or low-level visual features, one possible reason for the observed inconsistency regarding the influence of gaze direction on the N170 may be interindividual differences (as suggested by Burra et al., Citation2019).
In this study, we therefore also assessed the N170 regarding both gaze direction and head orientation during a gender categorisation task as a function of individual differences in attachment. Eye gaze is thought to be crucial for initiating social interactions and allows for synchronisation between individuals in terms of mutual attention (Patterson, Citation1982). Being able to quickly encode gaze direction is therefore a critical mechanism for human social interactions. Importantly, this preferential gaze direction encoding might facilitate gender categorisation (Macrae et al., Citation2002) by influencing face processing. In this study, we found that the influence of gaze direction on the N170 may differ in the case of AX but not AV. AX is associated with the secondary attachment strategy of hyperactivation of the attachment system, which is generally linked to an increased sensitivity to particularly negative social clues that could signal social rejection or abandonment (Mikulincer & Shaver, Citation2007b). Here, gaze direction influenced the right interhemispheric asymmetry of the N170, a marker of a precedence of global over local feature percept (Tanaka & Farah, Citation1993; Yovel et al., Citation2003). In our study, the pattern differed as a function of AX. We may thus speculate that highly anxiously attached participants’ attention was more strongly drawn to an averted gaze direction because it possibly contained a stronger social clue of rejection or abandonment (Burra et al., Citation2019). When eye gaze is averted and attention is increasingly directed to capture such local perception of gaze direction (as was the case for highly anxiously attached participants), a more holistic processing of the entire face required to perform the task at hand (gender categorisation) may be impaired. Thus, because gaze direction is manipulated but not relevant for the task, it is likely that gaze might impair the featural/global face processing dedicated to the gender categorisation. In sum, AX characterised by hyperactivating strategies may make individuals more prone to local rather than global face processing on a neural level. Further research is necessary to replicate and extend these findings to better understand how AX may associate with local versus global early face processing and what consequences this may have on the neural encoding of other properties of faces, e.g. emotion, trustworthiness, familiarity, etc.
Our new data highlight the early sensitivity of the visual system to eye gaze. A prominent face processing model suggests that the structural encoding of faces related to the N170 provides information for the analysis of gaze direction and not the other way around (Bentin et al., Citation1996). Our present data, especially in association with interindividual differences in attachment, imply that the opposite may also be true. We found that gaze direction may impact early face encoding as early as 170 ms after stimulus onset, and that this effect may vary as a function of AX. Accordingly, an early neural encoding of gaze direction during or already before structural encoding appears likely, which questions extant face models implying that eye gaze perception follows structural encoding of faces (Bruce & Young, Citation1986; Haxby et al., Citation2000). Our data rather point to a precedence of eye gaze processing, specifically a straight gaze, at an early stage of visual processing (Senju & Johnson, Citation2009), and a modulation of such mechanism by interindividual differences in attachment. The findings presented here thus replicate and importantly extend our understanding of the relationship between gaze direction perception and personality on a neural level. Our data furthermore imply that interindividual differences might modulate gaze processing at very early stages of face perception, likely via top-down influences shaped by social experience (Burra et al., Citation2019). The underlying mechanisms leading to this modulation, however, remain to be more deeply investigated. For instance, an investigation of interindividual differences in behavioural and electrophysiological correlates of gaze perception depending of the context would be of major interest (see, for instance, McCrackin & Itier, Citation2018). Although we may interpret the observed patterns as preliminary evidence that gaze perception may impact face encoding in relation to secondary attachment strategies aimed at capturing clues for social rejection or abandonment that characterise AX, more work is still needed.
This work has some limitations. First, we interpret the interhemispheric difference as a marker of holistic versus featural processing based on previous literature. However, it could be argued that greater amplitude in the right versus left hemisphere does not necessarily imply that the two hemispheres are functionally different in terms of processes. An alternative explanation would be that the neural generators in the right hemisphere are tuned in such a way that this part of the scalp EEG would produce greater amplitudes. We therefore acknowledge that differences between hemispheres may also reflect differences in terms of structural factors like the orientation of neural generators of the N170, which might vary among participants. However, because we demonstrate an interaction between hemisphere, attachment and gaze in this study, we argue that the modulation of the interhemispheric asymmetry more likely is functional (modulated by the gaze direction) rather than structural. While the neural source of the interhemispheric difference is unknown, further studies should systematically seek for the interhemispheric neural generators as well as its sensitivity to holistic or featural processing using dedicated measures such as source analysis by high-density EEG or MEG.
Furthermore, the reliability of our AX and AV dimensions obtained from the RSQ was somewhat low, although the method to derive the latter has been validated and successfully applied in the past. Ideally, the same associations reported here should be replicated by using the RSQ and/or other self-report measures of attachment, such as the Experiences in Close Relationships (ECR) questionnaire. It would also be interesting to see whether such findings can be replicated by employing a narrative attachment measure (e.g. the Adult Attachment Interview).
In addition, although our study focused primarily on the N170 during time windows and electrodes based on previous findings, a more exploratory analysis of the EEG signal could be performed through larger time windows and including all electrodes. In the absence of a priori assumptions and in order address the multiple comparison issues, analyses such as univariate mass approaches (Groppe et al., Citation2011), have been suggested to have equivalent or greater power (under equivalent spatial and temporal assumptions) with greater flexibility than the widely used average amplitude approach. However, with respect to our interhemispheric measurements, we note that, at this time, differences between hemispheres are not suggested using this technique or alternative data modelling. Further progress in analysis should be made to fill this gap in the literature.
Overall, our results highlight the critical role of interindividual differences on N170 sensitivity to gaze direction and may unify previously inconsistent results. Further experiments on the visual processing of social information in association with gaze direction and head orientation are necessary, especially regarding interindividual differences.
Supplemental Material
Download MS Word (50.6 KB)Acknowledgment
This research did not receive any specific grant from funding agencies in the public, commercial, or not-for-profit sectors. NB is supported by the Swiss National Foundation Grant 10001C_204387 and the Ernst and Lucy Schmidheiny Foundation.
Disclosure statement
No potential conflict of interest was reported by the author(s).
Supplementary material
Supplemental data for this article can be accessed online at https://doi.org/10.1080/14616734.2022.2091337.
Additional information
Funding
References
- Beebe, B., & Steele, M. (2013). How does microanalysis of mother–infant communication inform maternal sensitivity and infant attachment? Attachment & Human Development, 15(5–6), 583–602. https://doi.org/10.1080/14616734.2013.841050
- Benjamini, Y., & Hochberg, Y. (1995). Controlling the False Discovery Rate: A Practical and Powerful Approach to Multiple Testing. Journal of the Royal Statistical Society. Series B (Methodological), 57(1), 289–300. https://doi.org/10.1111/j.2517-6161.1995.tb02031.x
- Bentin, S., Allison, T., Puce, A., Perez, E., & McCarthy, G. (1996). Electrophysiological studies of face perception in humans. Journal of Cognitive Neuroscience, 8(6), 551–565. https://doi.org/10.1162/jocn.1996.8.6.551
- Blehar, M. C., Lieberman, A. F., & Ainsworth, M. D. S. (1977). Early face-to-face interaction and its relation to later infant–mother attachment. Child Development, 48(1), 182–194. https://doi.org/10.2307/1128897
- Brainard, D. H. (1997). The psychophysics toolbox. Spatial Vision, 10(4), 433–436. https://doi.org/10.1163/156856897X00357
- Bruce, V., & Young, A. (1986). Understanding face recognition. British Journal of Psychology, 77(3), 305–327. https://doi.org/10.1111/j.2044-8295.1986.tb02199.x
- Burra, N., Baker, S., & George, N. (2017). Processing of gaze direction within the N170/M170 time window: A combined EEG/MEG study. Neuropsychologia, 100, 207–219. https://doi.org/10.1016/j.neuropsychologia.2017.04.028
- Burra, N., Framorando, D., & Pegna, A. J. (2018). Early and late cortical responses to directly gazing faces are task dependent. Cognitive, Affective, & Behavioral Neuroscience, 18(4), 796–809. https://doi.org/10.3758/s13415-018-0605-5
- Burra, N., Massait, S., & Vrticka, P. (2019). Differential impact of trait, social, and attachment anxiety on the stare-in-the-crowd effect. Scientific Reports, 9(1), 1797. https://doi.org/10.1038/s41598-019-39342-8
- Burra, N., Mares, I., & Senju, A. (2019). The influence of top-down modulation on the processing of direct gaze. WIREs Cognitive Science, 10(5), e1500. https://doi.org/10.1002/wcs.1500
- Callaghan, B. L., & Tottenham, N. (2016). The neuro-environmental loop of plasticity: A cross-species analysis of parental effects on emotion circuitry development following typical and adverse caregiving. Neuropsychopharmacology, 41(1), 163–176. https://doi.org/10.1038/npp.2015.204
- Carrick, O. K., Thompson, J. C., Epling, J. A., & Puce, A. (2007). It’s all in the eyes: Neural responses to socially significant gaze shifts. Neuroreport, 18(8), 763–766. https://doi.org/10.1097/WNR.0b013e3280ebb44b
- Cecchini, M., Iannoni, M. E., Pandolfo, A. L., Aceto, P., & Lai, C. (2015). Attachment style dimensions are associated with brain activity in response to gaze interaction. Social Neuroscience, 10(3), 282–293. https://doi.org/10.1080/17470919.2014.998344
- Conty, L., Tijus, C., Hugueville, E., Coelho, L., & George, N. (2006). Searching for asymmetries in the detection of gaze contact versus averted gaze under different head views: A behavioural study. Spatial Vision, 19(6), 529–545. https://doi.org/10.1163/156856806779194026
- Conty, L., N’Diaye, K., Tijus, C., & George, N. (2007). When eye creates the contact! ERP evidence for early dissociation between direct and averted gaze motion processing. Neuropsychologia, 45(13), 3024–3037. https://doi.org/10.1016/j.neuropsychologia.2007.05.017
- Dan, O., & Raz, S. (2012). Adult attachment and emotional processing biases: An event-related potentials (ERPs) study. Biological Psychology, 91(2), 212–220. https://doi.org/10.1016/j.biopsycho.2012.06.003
- Debbane, M., Salaminios, G., Luyten, P., Badoud, D., Armando, M., Solida Tozzi, A., Fonagy, P., & Brent, B. K. (2016). Attachment, neurobiology, and mentalizing along the psychosis continuum. Frontiers in Human Neuroscience, 10, 406. https://doi.org/10.3389/fnhum.2016.00406
- Donges, U.-S., Kugel, H., Stuhrmann, A., Grotegerd, D., Redlich, R., Lichev, V., Rosenberg, N., Ihme, K., Suslow, T., & Dannlowski, U. (2012). Adult attachment anxiety is associated with enhanced automatic neural response to positive facial expression. Neuroscience, 220, 149–157. https://doi.org/10.1016/j.neuroscience.2012.06.036
- Emmorey, K., Midgley, K. J., Kohen, C. B., Sehyr, Z. S., & Holcomb, P. J. (2017). The N170 ERP component differs in laterality, distribution, and association with continuous reading measures for deaf and hearing readers. Neuropsychologia, 106, 298–309. https://doi.org/10.1016/j.neuropsychologia.2017.10.001
- Fonagy, P., & Luyten, P. (2009). A developmental, mentalization-based approach to the understanding and treatment of borderline personality disorder. Development and Psychopathology, 21(4), 1355–1381. https://doi.org/10.1017/S0954579409990198
- Fraedrich, E. M., Lakatos, K., & Spangler, G. (2010). Brain activity during emotion perception: The role of attachment representation. Attachment & Human Development, 12(3), 231–248. https://doi.org/10.1080/14616731003759724
- George, N., Driver, J., & Dolan, R. J. (2001). Seen gaze-direction modulates fusiform activity and its coupling with other brain areas during face processing. NeuroImage, 13(6), 1102–1112. https://doi.org/10.1006/nimg.2001.0769
- George, N., & Conty, L. (2008). Facing the gaze of others. Neurophysiologie Clinique/Clinical Neurophysiology, 38(3), 197–207. https://doi.org/10.1016/j.neucli.2008.03.001
- Griffin, D., & Bartholomew, K. (1994). Models of the self and other: Fundamental dimensions underlying measures of adult attachment. Journal of Personality and Social Psychology, 67(3), 430–445. https://doi.org/10.1037/0022-3514.67.3.430
- Groppe, D. M., Urbach, T. P., & Kutas, M. (2011). Mass univariate analysis of event-related brain potentials/fields I: A critical tutorial review. Psychophysiology, 48(12), 1711–1725. https://doi.org/10.1111/j.1469-8986.2011.01273.x
- Guastella, A. J., Mitchell, P. B., & Dadds, M. R. (2008). Oxytocin increases gaze to the eye region of human faces. Biological Psychiatry, 63(1), 3–5. https://doi.org/10.1016/j.biopsych.2007.06.026
- Guédeney, N., Fermanian, J., & Bifulco, A. (2010). La Version française du Relationship Scales Questionnaire de Bartholomew (RSQ, Questionnaire des échelles de relation) : étude de validation du construit. L’Encéphale, 36(1), 69–76. https://doi.org/10.1016/j.encep.2008.12.006
- Haxby, J. V., Hoffman, E. A., & Gobbini, M. I. (2000). The distributed human neural system for face perception. Trends in Cognitive Sciences, 4(6), 223–233. https://doi.org/10.1016/s1364-6613(00)01482-0
- Hillger, L. A., & Koenig, O. (1991). Separable mechanisms in face processing: Evidence from hemispheric specialization. Journal of Cognitive Neuroscience, 3(1), 42–58. https://doi.org/10.1162/jocn.1991.3.1.42
- Itier, R. J., Alain, C., Kovacevic, N., & McIntosh, A. R. (2007). Explicit versus implicit gaze processing assessed by ERPs. Brain Research, 1177, 79–89. https://doi.org/10.1016/j.brainres.2007.07.094
- Itier, R. J., & Batty, M. (2009). Neural bases of eye and gaze processing: The core of social cognition [Research Support, Non-U.S. Gov’t Review]. Neuroscience & Biobehavioral Reviews, 33(6), 843–863. https://doi.org/10.1016/j.neubiorev.2009.02.004
- Kleiner, M., Brainard, D., & Pelli, D. (2007). What’s new in psychtoolbox-3? Perception, 36(14), 1–16. //WOS:000250594600049
- Latinus, M., Love, S. A., Rossi, A., Parada, F. J., Huang, L., Conty, L., George, N., James, K., & Puce, A. (2015). Social decisions affect neural activity to perceived dynamic gaze. Social Cognitive and Affective Neuroscience, 10(11), 1557–1567. https://doi.org/10.1093/scan/nsv049
- Lewis, T. L., Maurer, D., Tytla, M. E., Bowering, E. R., & Brent, H. P. (1992). Vision in the “good” eye of children treated for unilateral congenital cataract. Ophthalmology, 99(7), 1013–1017. https://doi.org/10.1016/s0161-6420(92)31857-3
- Long, M., Verbeke, W., Ein-Dor, T., & Vrticka, P. (2020). A functional neuro-anatomical model of human attachment (NAMA): Insights from first- and second-person social neuroscience. Cortex, 126, 281–321. https://doi.org/10.1016/j.cortex.2020.01.010
- Lopes, R., Cabral, P., Canas, N., Breia, P., Foreid, J. P., Calado, E., Silva, R., & Leal, A. (2011). N170 asymmetry as an index of inferior occipital dysfunction in patients with symptomatic occipital lobe epilepsy. Clinical Neurophysiology, 122(1), 9–15. https://doi.org/10.1016/j.clinph.2010.05.023
- Lopes, R., Cabral, P., Canas, N., Breia, P., Foreid, J. P., Calado, E., & Leal, A. (2011). N170 asymmetry as an index of inferior occipital dysfunction in patients with symptomatic occipital lobe epilepsy. Clinical Neurophysiology, 122(1), 9–15. https://doi.org/10.1016/j.clinph.2010.05.023
- Ma, Y., Ran, G., Chen, X., Ma, H., & Hu, N. (2017). Adult attachment styles associated with brain activity in response to infant faces in nulliparous women: An event-related potentials study. Frontiers in Psychology, 8, 627. https://doi.org/10.3389/fpsyg.2017.00627
- Macrae, C. N., Hood, B. M., Milne, A. B., Rowe, A. C., & Mason, M. F. (2002). Are you looking at me? Eye gaze and person perception. Psychological Science, 13(5), 460–464. https://doi.org/10.1111/1467-9280.00481
- Maurer, U. (2008). Category specificity in early perception: Face and word n170 responses differ in both lateralization and habituation properties. Frontiers in Human Neuroscience, 2, 18. https://doi.org/10.3389/neuro.09.018.2008
- McCrackin, S. D., & Itier, R. J. (2018). Is it about me? Time-course of self-relevance and valence effects on the perception of neutral faces with direct and averted gaze. Biological Psychology, 135, 47–64. https://doi.org/10.1016/j.biopsycho.2018.03.003
- Mercure, E., Dick, F., Halit, H., Kaufman, J., & Johnson, M. H. (2008). Differential lateralization for words and faces: Category or psychophysics? Journal of Cognitive Neuroscience, 20(11), 2070–2087. https://doi.org/10.1162/jocn.2008.20137
- Mercure, E., Cohen Kadosh, K., & Johnson, M. H. (2011). The n170 shows differential repetition effects for faces, objects, and orthographic stimuli. Frontiers in Human Neuroscience, 5, 6. https://doi.org/10.3389/fnhum.2011.00006
- Mikulincer, M., & Shaver, P. R. (2007a). Attachment in adulthood: Structure, dynamics, and change. Guilford Press.
- Mikulincer, M., & Shaver, P. R. (2007b). Attachment, group–related processes, and psychotherapy. International Journal of Group Psychotherapy, 57(2), 233–245. https://doi.org/10.1521/ijgp.2007.57.2.233
- Mikulincer, M., & Shaver, P. R. (2007c). Boosting attachment security to promote mental health, prosocial values, and inter-group tolerance. Psychological Inquiry, 18(3), 139–156. https://doi.org/10.1080/10478400701512646
- Myllyneva, A., & Hietanen, J. K. (2015). There is more to eye contact than meets the eye. Cognition, 134, 100–109. https://doi.org/10.1016/j.cognition.2014.09.011
- Naples, A. J., Wu, J., Mayes, L. C., & McPartland, J. C. (2017). Event-Related potentials index neural response to eye contact. Biological Psychology, 127, 18–24. https://doi.org/10.1016/j.biopsycho.2017.04.006
- Onitsuka, T., Spencer, K. M., Lucia, L. C., Shenton, M. E., McCarley, R. W., & Niznikiewicz, M. A. (2009). Abnormal asymmetry of the face n170 repetition effect in male patients with chronic schizophrenia. Brain Imaging and Behavior, 3(3), 240–245. https://doi.org/10.1007/s11682-009-9066-3
- Ostrovsky, Y., Andalman, A., & Sinha, P. (2006). Vision following extended congenital blindness. Psychological Science, 17(12), 1009–1014. https://doi.org/10.1111/j.1467-9280.2006.01827.x
- Patterson, M. L. (1982). A sequential functional model of nonverbal exchange. Psychological Review, 89(3), 231–249. https://doi.org/10.1037/0033-295X.89.3.231
- Prinsen, J., Deschepper, A., Maes, E., & Alaerts, K. (2019). Attachment styles have a modulatory impact on psychophysiological arousal evoked by reciprocated and unreciprocated gaze. Biological Psychology, 148, 107773. https://doi.org/10.1016/j.biopsycho.2019.107773
- Rossion, B., Dricot, L., Devolder, A., Bodart, J. M., Crommelinck, M., De Gelder, B., & Zoontjes, R. (2000). Hemispheric asymmetries for whole-based and part-based face processing in the human fusiform gyrus. Journal of Cognitive Neuroscience, 12(5), 793–802. https://doi.org/10.1162/089892900562606
- Rossion, B., Caldara, R., Seghier, M., Schuller, A. M., Lazeyras, F., & Mayer, E. (2003). A network of occipito-temporal face-sensitive areas besides the right middle fusiform gyrus is necessary for normal face processing. Brain, 126(11), 2381–2395. https://doi.org/10.1093/brain/awg241
- Sato, W., Kochiyama, T., Uono, S., & Yoshikawa, S. (2008). Time course of superior temporal sulcus activity in response to eye gaze: A combined fMRI and MEG study. Social Cognitive and Affective Neuroscience, 3(3), 224–232. https://doi.org/10.1093/scan/nsn016
- Scott, L. S. (2006). Featural and configural face processing in adults and infants: A behavioral and electrophysiological investigation. Perception, 35(8), 1107–1128. https://doi.org/10.1068/p5493
- Senju, A., & Hasegawa, T. (2005). Direct gaze captures visuospatial attention. Visual Cognition, 12(1), 127–144. https://doi.org/10.1080/13506280444000157
- Senju, A., Hasegawa, T., & Tojo, Y. (2005). Does perceived direct gaze boost detection in adults and children with and without autism? The stare-in-the-crowd effect revisited. Visual Cognition, 12(8), 1474–1496. https://doi.org/10.1080/13506280444000797
- Senju, A., & Johnson, M. H. (2009). The eye contact effect: Mechanisms and development [Research Support, Non-U.S. Gov’t Review]. Trends in Cognitive Sciences, 13(3), 127–134. https://doi.org/10.1016/j.tics.2008.11.009
- Senju, A., Vernetti, A., Ganea, N., Hudry, K., Tucker, L., Charman, T., & Johnson, M. H. (2015). Early social experience affects the development of eye gaze processing. Current Biology, 25(23), 3086–3091. https://doi.org/10.1016/j.cub.2015.10.019
- Sergent, J. (1982). About face: Left-hemisphere involvement in processing physiognomies. Journal of Experimental Psychology: Human Perception and Performance, 8(1), 1–14. https://doi.org/10.1037/0096-1523.8.1.1
- Simpson, J. A., Rholes, W. S., & Nelligan, J. S. (1992). Support seeking and support giving within couples in an anxiety-provoking situation: The role of attachment styles. Journal of Personality and Social Psychology, 62(3), 434–446. https://doi.org/10.1037/0022-3514.62.3.434
- Slee, P. T. (1984). The nature of mother–infant gaze patterns during interaction as a function of emotional expression. Journal of the American Academy of Child and Adolescent Psychiatry, 23(4), 385–391. https://doi.org/10.1016/S0002-7138(09)60315-0
- Stern, D. N. (1974). Mother and infant at play: The dyadic interaction involving facial, vocal, and gaze behaviors.
- Tanaka, J. W., & Farah, M. J. (1993). Parts and wholes in face recognition. The Quarterly Journal of Experimental Psychology Section A, 46(2), 225–245. https://doi.org/10.1080/14640749308401045
- Taylor, M. J., Itier, R. J., Allison, T., & Edmonds, G. E. (2001). Direction of gaze effects on early face processing: Eyes-only versus full faces. Brain Research, 10(3), 333–340. https://doi.org/10.1016/s0926-6410(00)00051-3
- Vernetti, A., Ganea, N., Tucker, L., Charman, T., Johnson, M. H., & Senju, A. (2018). Infant neural sensitivity to eye gaze depends on early experience of gaze communication. Developmental Cognitive Neuroscience, 34, 1–6. https://doi.org/10.1016/j.dcn.2018.05.007
- Vrticka, P., Bondolfi, G., Sander, D., & Vuilleumier, P. (2012). The neural substrates of social emotion perception and regulation are modulated by adult attachment style. Social Neuroscience, 7(5), 473–493. https://doi.org/10.1080/17470919.2011.647410
- Vrtička, P., Andersson, F., Grandjean, D., Sander, D., & Vuilleumier, P. (2008). Individual attachment style modulates human amygdala and striatum activation during social appraisal. PLoS One, 3(8), e2868. https://doi.org/10.1371/journal.pone.0002868
- Vuilleumier, P., George, N., Lister, V., Armony, J., & Driver, J. (2005). Effects of perceived mutual gaze and gender on face processing and recognition memory. Visual Cognition, 12(1), 85–101. https://doi.org/10.1080/13506280444000120
- Watanabe, S., Miki, K., & Kakigi, R. (2002). Gaze direction affects face perception in humans. Neuroscience Letters, 325(3), 163–166. https://doi.org/10.1016/s0304-3940(02)00257-4
- Willenbockel, V., Sadr, J., Fiset, D., Horne, G. O., Gosselin, F., & Tanaka, J. W. (2010). Controlling low-level image properties: The SHINE toolbox. Behavior Research Methods, 42(3), 671–684. https://doi.org/10.3758/BRM.42.3.671
- Wollaston, W. H. (1824). Xiii. On the apparent direction of eyes in a portrait. Philosophical Transactions of the Royal Society of London, 114, 247–256.
- Yovel, G., Levy, J., Grabowecky, M., & Paller, K. A. (2003). Neural correlates of the left-visual-field superiority in face perception appear at multiple stages of face processing. Journal of Cognitive Neuroscience, 15(3), 462–474. https://doi.org/10.1162/089892903321593162
- Zhang, X., Li, T., & Zhou, X. (2008). Brain responses to facial expressions by adults with different attachment-orientations. Neuroreport, 19(4), 437–441. https://doi.org/10.1097/WNR.0b013e3282f55728
- Zilber, A., Goldstein, A., & Mikulincer, M. (2007). Adult attachment orientations and the processing of emotional pictures - ERP correlates. Personality and Individual Differences, 43(7), 1898–1907. https://doi.org/10.1016/j.paid.2007.06.015