ABSTRACT
The morphogenetic changes of the bud meristem during floral initiation in gooseberry were examined by scanning electron microscopy. Six floral stages, similar to those reported for black currants, were identified. We also studied the environmental control of shoot growth and floral initiation of cvs. Mucurines, Pax and Xenia in two experiments in daylight phytotron compartments at 12, 18 and 24°C. Under natural daylength conditions at Ås, Norway (69°40’N), shoot growth started to decline by mid-August and ceased in early September. Cessation of growth was associated with floral initiation at 18 and 12°C, while at 24°C, only ‘Mucurines’ initiated floral primordia. Floral Stage 2 was reached by 3 September in ‘Mucurines’ and ‘Xenia’ at 18 and 12°C and nearly 2 weeks later in ‘Pax’. In a second experiment with controlled photoperiods, all cultivars ceased growing and initiated flowering in 10-h SD within 2–3 weeks, while in 20-h LD, growth continued for 8 weeks without floral initiation. Under 10-h SD conditions, all cultivars initiated flowers also at 24°C. Flowering performance in the following spring verified these results. We conclude that gooseberry is an obligatory SD plant with a critical photoperiod of 15–16 h.
Introduction
The cultivated gooseberry (Ribes uva-crispa L.) is a temperate shrub of mainly European origin. However, modern cultivars also carry genes derived from the American species, R. hirtellum Michx. and R. divaricatum Dougl., which have been introgressed into these cultivars for resistance against the American gooseberry mildew disease (Maxfield, Wallis, Harrison, & Nicholson, Citation1969). Most cultivars have shoots with nodal spines and some have prickly fruit, an unpleasant armoury that has been difficult to eliminate by breeding (see ).
Although gooseberries have been widely grown on a small scale in the temperate and cold regions of the Northern hemisphere for their juicy and tasty fruits, little is known about the control of flowering in the species. In his CRC Handbook of Flowering review, Wright (Citation1985) stated that gooseberries initiate floral primordia the year before flowering and fruiting, and that initiation takes place later in the season in gooseberries than in black currants. He further mentions that gooseberries may require ‘shorter days’ for flower initiation, but that this is not clear. Thus, while floral initiation and its environmental control are well documented and described for black and red currants (Heide & Sønsteby, Citation2011; Nasr & Wareing, Citation1958; Palonen & Voipio, Citation1994; Sønsteby & Heide, Citation2011; Tinklin, Wilkinson, & Schwabe, Citation1970; Wright, Citation1985), little is known about the control of flowering in gooseberries. Because of the increasing interest in commercialising the crop, we have used scanning electron microscopy to examine the changes taking place in gooseberry buds during floral initiation. We also studied the environmental control of floral initiation under controlled-environment conditions.
Materials and methods
Plant material and handling
The gooseberry (Ribes uva-crispa L.) cultivars ‘Mucurines’, ‘Pax’ and ‘Xenia’ were used for the investigation. The experimental plants were 2-year old and purchased from a certified commercial nursery. After overwintering as bare-root plants in a cold store at −2°C, they were trimmed to two main shoots, which were cut back to about 5 cm length. All additional shoots were cut at the base. The plants were potted into 3-L plastic pots filled with a coarse-textured peat compost and raised at the NIBIO Experimental Centre Apelsvoll in South East Norway (60°40ʹN, 10°40ʹE, 250 m a.s.l.) in an open plastic tunnel until the phytotron experiments were started. The natural photoperiod in the tunnel was extended to 20 h by 70 W incandescent lamps.
Two phytotron experiments were conducted with all three cultivars in 2019/20 and in 2020/21 (Exps. I and II, respectively). In Experiment I, the plants were transferred on 11 June to the daylight phytotron at the Norwegian University of Life Sciences at Ås, Norway (59°40ʹN, 10°45ʹE) and exposed to temperatures of 12, 18, and 24°C and natural daylength conditions for 16 weeks. A fourth group of plants was maintained at ambient conditions in the open plastic tunnel at the NIBIO Experimental Centre Apelsvoll as an outdoor control. The daily mean temperatures at Apelsvoll during the 2019 growing season and in the spring of 2020 are shown in .
Figure 2. Daily mean temperatures at the NIBIO Experimental Centre Apelsvoll during the growing season in 2019 and in the spring of 2020. The average daily mean temperature 1 September to 1 October in 2019 was 9.8°C.
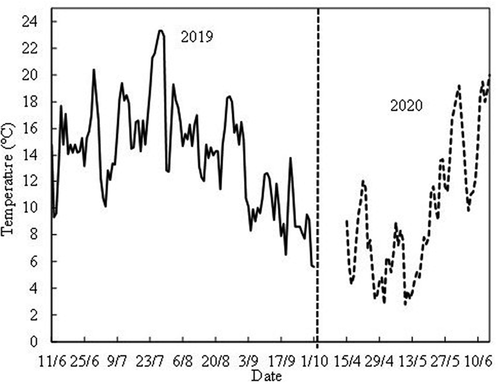
In parallel with Experiment I, a separate batch of plants of ‘Mucurines’ and ‘Pax’ were grown at 18°C in 10 h SD in the phytotron for scanning electron microscopy examination of the early changes of bud meristems during floral initiation and differentiation. Buds were sampled weekly and fixed in glutaraldehyde (1.25%) and paraformaldehyde in 0.05 M PIPES buffer at pH 7.2 and processed as described by Rivero, Sønsteby, Heide, Måge, and Remberg (Citation2017). The prepared specimens were examined in an EVO-50 scanning electron microscope (Zeiss, Jena, Germany) operated at 25 kV. The successive stages of floral initiation and differentiation were assigned Stages 1 (vegetative) to 6 (primary and secondary flower fully differentiated).
In Experiment II, the plants from Experiment I were used for a second floral induction experiment. After flowering in the spring of 2020, the plants were cut back, managed, and grown as described for Experiment I, except that the plants were maintained under ambient conditions at Apelsvoll until 6 August before they were transferred to the phytotron. They were then exposed to factorial combinations of temperature and photoperiod (12, 18, and 24°C × 10 and 20 h) for 8 weeks.
Temperatures in the phytotron were controlled to ± 1.0°C, and a water vapour pressure deficit of 530 Pa was maintained at all temperatures. When the photosynthetic photon flux (PPF) dropped below 150 µmol quanta m−2 s−1 during the day (0800–1800 h), an additional 125 µmol quanta m−2 s−1 was automatically added using 400 W Philips HPI-T lamps. For the photoperiodic treatments in Experiment II, all the plants were grown in the daylight compartments for 10 h (0800–1800 h). In order to limit the additional light energy of the long day (LD) treatment to a minimum, all plants were moved into adjacent growth rooms from 1800 to 0800 h where they were kept in darkness (10 h SD) or exposed to low-intensity light (approx. 7 µmol quanta m−2 s−1) from 70 W incandescent lamps for daylength extension to 20 h. The additional light energy of the daylength extension amounted to less than 2% of the total daily photosynthetic active radiation.
Throughout cultivation, the plants were watered daily with a slightly modified Hoagland nutrient solution with an electrical conductivity of 1.3 mS cm−1. Two broad spectrum fungicide and one insecticide spraying were applied during the first week after potting in the spring. The plants were also sprayed with a Thiovit elemental sulphur suspension for protection against mildew and subjected to biological control with A. cucumeris against spider mites and thrips at the start and 3–4 times during experimentation. After completion of the experimental treatments on 1 October, the plants in both experiments were moved directly into a cold store kept at +2°C. On 1 November, the temperature was lowered to 0°C for overwintering. In early May, the following year, the plants were then moved outdoors at Apelsvoll, and the level of flowering recorded under rain covers in an open plastic tunnel.
Experimental design, data collection, and analysis
The experiments were arranged in split-plot designs, with temperatures as the main plots and photoperiods and cultivars as subplots. Each treatment consisted of three replicated plant trollies, each with three plants of each cultivar, and the placement of the cultivars on the trollies was varied systematically among the replicates in order to eliminate positional effects. Due to the every-day movements of the trolleys between the daylight and photoperiod treatment rooms, any possible positional effects of the trollies in the rooms were also eliminated.
In both experiments, plant growth in the phytotron was monitored by weekly observations of shoot height and number of nodes (leaves) of the tallest shoot on each plant. Starting on 6 August, three lateral buds from the second shoot in each plant were also sampled weekly for the assessment of floral development by dissection and stereo microscopy. Lateral buds positioned 7–8 nodes below the apex were removed by a shallow scalpel slit and stored on 70% ethanol until dissected. Floral development was scored according to the six-stage scale shown in , where Stage 1 denotes entirely vegetative buds, and Stage 2 the first visible sign of transition to generative development, while Stage 6 denotes fully differentiated primary and secondary flower primordia. The percentage of flowering plants and nodes as well as the number of flowers per plant and per inflorescence were recorded in the following spring on the shoot from which no buds had been sampled in the previous year.
Percentage data for flowering plants were often ‘zero’ and ‘hundred’ and therefore characterised by a lack of variability (all or none of the plants showed physiological reaction). Therefore, no statistical test was performed on these data. Other percentage values were subjected to an arc sin transformation before the analysis. All the experimental data were tested for normality using the Anderson–Darling test (α = 0.05), and, when necessary, were transformed using the Yeo-Johnson family transformation (Yeo & Johnson, Citation2000; Zhou, Zhang, Huang, & Xue, Citation2022). Then, the experimental data were processed by the General Linear Model (GLM), and significant differences (P < 0.05) between the treatments were evaluated by the Tukey procedure using a MiniTab® Statistical Software programme package (Release 19, Minitab Inc., State College, PA, USA).
Results
Early stages of flower bud formation
The morphogenetic changes of the bud meristem during floral initiation and differentiation of cv. Mucurines in 10-h SD at 18°C is shown in . The Vegetative Stage 1 was characterised by a round, rather flat apical meristem with leaf primordia only (). The first visible sign of reproductive development was marked by broadening and swelling of the apical meristem, and the appearance of the secondary floral meristem as an elliptical fold of tissue between the primary floral meristem and the bract primordia (Stage 2; ). Stage 3, which was reached after 4 weeks of SD treatment, was characterised by the appearance of sepals as five small protuberances arising from the outer circumference of the broadened primary floral meristem, giving it a pentagonal shape. At this stage, the secondary floral meristem becomes more protuberant (). Stage 4 was characterised by the further development of the sepals and the differentiation of anthers (appearing in the inside of the ring, opposite to the sepals as ball-like protuberances) and petals (alternating with the sepals and likewise appearing as small ball-like protuberances). In parallel, the secondary floral meristem elongates, and its surface flattens (). At Stage 5, sepals, petals, and anthers develop further on the primary flower meristem, while sepals, petals, and anthers appear on the secondary flower meristem (). At the last stage of development (Stage 6), all floral organs including the carpel primordia and a visible ovarian cavity are differentiated on the primary and secondary flower primordia (). Two bilaterally symmetrical theca are established within the anther, and the carpel primordia appear as two small protuberances surrounding the ovarian cavity in the middle of each flower primordium. No structural differences could be detected between the cvs. Mucurines and Pax.
Figure 3. Stages of floral morphogenesis in ‘Mucurines’ gooseberry plants grown in 10-h photoperiod at 18°C. (A) Round, flat apex (Stage 1). (B) Protuberant apex with bract primordia and secondary floral meristem (Stage 2). (C) Primary floral meristem with pentagonal shape, bract primordia and secondary floral meristem round and protuberant (Stage 3). (D) Primary floral meristem with sepals, petals and anthers, and secondary floral meristem with flat surface (Stage 4). (E) Further development of sepals, petals and anthers on both floral meristems (Stage 5). (F) All flower organs differentiated on both primary and secondary flower primordia. (Stage 6). AM = apical meristem; LP = leaf primordium; rLP = removed LP; BP = bract primordium; rBP = removed BP; FM = floral meristem; pFM = primary FM; sFM = secondary FM; S = sepal; Pe = petal; An = anther; CP = Carpel primordium/ovarian cavity.
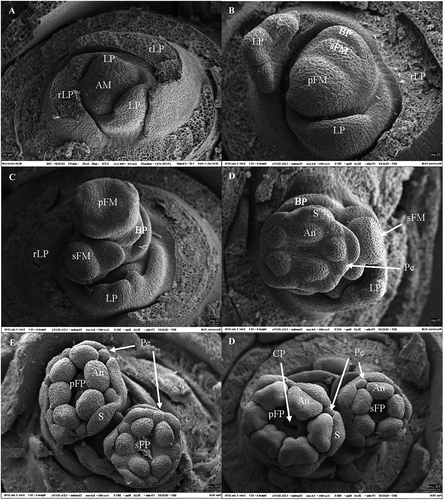
Experiment I
Under natural daylength conditions, shoot growth followed a sigmoid pattern during the season and was affected by genotype and temperature as well as their interaction (P < 0.001). Not only growth rate but also the seasonal pattern of growth varied between the cultivars. Initially, shoot elongation and leaf production were generally enhanced by high temperatures, being higher at 18 and 24°C than at 12°C and outdoor conditions which internally also had rather similar growth effects (). In general, shoot extension started to decline by mid-August (week 32), and by mid-September, growth had stopped. However, the time of growth cessation varied between cultivars and growing conditions and was particularly early in ‘Mucurines’ at 24°C where growth ceased by late July. For this reason, the final height of the ‘Mucurines’ plants were lowest at the highest temperature. The final plant heights varied between the cultivars, being largest in ‘Pax’ and lowest in ‘Mucurines’. Overall, growth rates expressed as increments of shoot height and leaf numbers were similar ().
Figure 4. Time courses of cumulative shoot growth and leaf number increments in three gooseberry cultivars grown at 12, 18 and 24°C under natural daylength conditions in the daylight phytotron at Ås, Norway for 16 weeks during the 2019 season (Exp. I). Growth of plants outdoors in an open plastic tunnel at Apelsvoll is also included. Data are means (± SE) for the main shoot of three replications with three plants each.
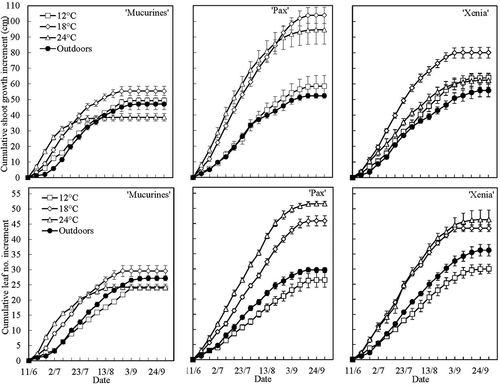
Floral initiation was earliest at 18 and 12°C, where a mean floral stage of about 2 was reached by 3 September in ‘Mucurines’ and ‘Xenia’, and two weeks later by 17 September in ‘Pax’ (). At 24°C, however, floral initiation was observed in ‘Mucurines’ only. At termination of the treatments on 1 October, ‘Xenia’ and ‘Mucurines’ had reached approximately Floral Stage 5 at 12 and 18°C, while ‘Pax’ was at Stage 3. As with shoot growth, the earliness and pattern of floral initiation was similar at 12°C in the phytotron and outdoors in ‘Mucurines’, but later outdoors in ‘Pax’ and ‘Xenia’ ().
Figure 5. Time courses of floral initiation and differentiation in three gooseberry cultivars grown at four temperatures under natural daylength conditions at Ås and Apelsvoll. Data are means (± SE) of three lateral buds from the main shoot of three replications with three plants each (Exp. I).
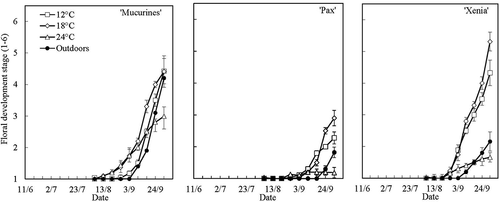
Flowering outdoors at Apelsvoll in the following spring confirmed that no flower initiation had taken place at 24°C in ‘Pax’ and ‘Xenia’, whereas ‘Mucurines’ flowered well, even in plants from 24°C (). However, none of the cultivars flowered abundantly. Many of the plants had ‘weak’ flowers and had indications of partial flower abortion. In all cultivars, the number of flowers per plant was highest in plants at 18°C in the phytotron, and across all temperatures, flowering was most abundant in ‘Mucurines’. The main effects of temperature and cultivar as well as their interaction, were significant for both percentage of flowering nodes and the number of flowers per plant and per inflorescence (). However, while shoot growth in the previous year was similar in plants outdoors and at 12°C in the phytotron (), flowering was consistently poorer outdoors in all cultivars. Time of bud break and anthesis were advanced by low temperatures during growth cessation in the previous year ().
Table 1. Level of flowering and dates of budbreak and anthesis under spring outdoor conditions in three gooseberry cultivars grown during the previous season in a daylight phytotron under natural daylength conditions at Ås, Norway, and at temperatures of 12, 18, and 24°C as indicated, and under outdoor conditions at Apelsvoll, Norway. Data are means for the main annual shoot of three replicates with three plants each (Exp. I)
Experiment II
Under controlled photoperiodic conditions, shoot growth started to decline almost immediately in 10-h SD and stopped within 3 weeks in all cultivars at all temperatures (). In contrast, in 20-h LD shoot growth continued at a nearly constant rate until the treatments were terminated after 8 weeks. At 24°C only, growth rate tended to decline over time in ‘Mucurines’ and ‘Pax’ due to shorter internodes. Otherwise, growth rate in LD did not vary greatly between temperatures, but was usually highest at 18°C, whereas leaf initiation rate usually was highest at 24°C and declined with decreasing temperature. Across the temperatures, shoot extension was higher in ‘Pax’ than in the other cultivars, whereas leaf production varied less.
Figure 6. Time courses of cumulative shoot growth and leaf number increment in three gooseberry cultivars grown at 12, 18 and 24°C under 10-h SD and 20-h LD conditions for eight weeks. Data are means (± SE) for the main shoot of three replications with three plants each (Exp. II).
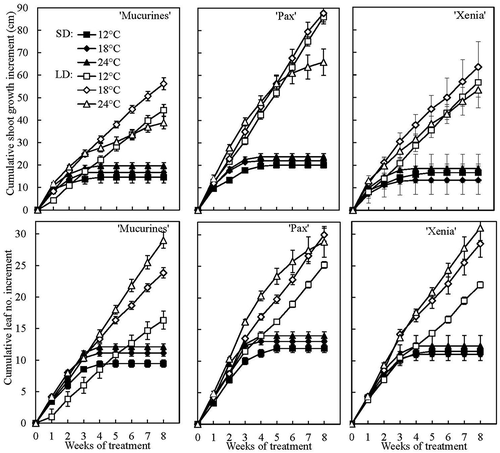
Under SD conditions, the initiation of floral primordia coincided with the cessation of shoot growth, whereas no floral initiation was observed in LD (). In all cultivars, initiation in SD started earliest at 18°C followed by 12°C and latest at 24°C. While Floral Stage 2 was reached after approximately 3 weeks at 18°C in all cultivars, the same stage was delayed by 1–2 weeks at 24°C in ‘Mucurines’ and ‘Xenia’, respectively, and by 3 weeks in ‘Pax’ in which initiation was particularly late at 24°C. After 7 weeks of SD, all cultivars had reached Floral Stage 6 at 18°C. The same stage was reached after 8 weeks in ‘Mucurines’ at 12°C, whereas none of the cultivars fully reached this stage at 24°C before the treatments were terminated after 8 weeks. Overall, floral initiation was the earliest and most uniform across the temperatures in ‘Mucurines’. Except for the slower flower development in ‘Pax’ at 24°C, and to a lesser extent at 12°C, the rate of floral differentiation was similar across cultivars and temperatures ().
Figure 7. Time courses of floral initiation and differentiation in three gooseberry cultivars grown at 12, 18 and 24°C under 10-h SD and 20-h LD conditions for eight weeks. Data are means (± SE) of three lateral buds from the main shoot of three replications with three plants each (Exp. II).
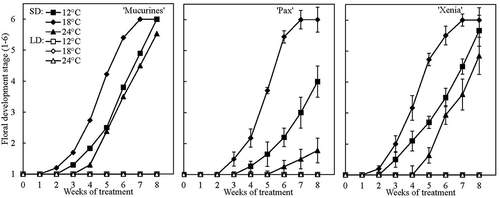
Flowering outdoors in the following spring fully confirmed the earlier results (). However, while 100% of the SD-exposed plants of ‘Mucurines’ and ‘Xenia’ flowered at all temperatures, this was true only at 18°C for the ‘Pax’ plants of which only 45% flowered at 12 and 24°C. Except for a single flower in two plants of ‘Mucurines’ in LD at 24°C, no flowering occurred in plants under LD conditions. The percentage of flowering nodes in SD-grown plants was highest in ‘Mucurines’ and lowest in ‘Pax’ at all temperatures. Under SD conditions, the proportion of flowering nodes in the former cultivar, increased with increasing temperature up to 24°C, while the other two cultivars had an optimum of 18°C. Identical responses of cultivar and environment were observed for the number of flowers per plant. As in Experiment I, the number of flowers per inflorescence increased slightly but consistently with decreasing temperature during floral initiation in all cultivars. All flowering parameters were governed by a pronounced interaction between temperature and photoperiod. Because of this interaction, the main effect of temperature was not always significant. Due to the dominant effect of photoperiod in all cultivars, there was no significant interaction between photoperiod and cultivar on any of the flowering parameters, whereas there was a highly significant three-way interaction for all the parameters.
Table 2. Level of flowering and dates of budbreak and anthesis under spring outdoor conditions in three gooseberry cultivars grown for 8 weeks (6 Aug.–1 Oct.) during the previous season under temperature and daylength conditions as indicated. Data are means for the main annual shoot of three replicates with three plants each (Exp. II)
As observed in Experiment I, bud break was delayed in the three cultivars by high temperature during dormancy and flowering induction in the previous autumn (). The effect was particularly marked in the non-flowering plants grown under LD conditions. Anthesis was also delayed by high autumn temperatures, although not to the same extent as bud break.
Discussion
The results show that the pattern of floral initiation in gooseberry is similar to that of black currant (cf. Kvam, Citation1986), except that the inflorescences of gooseberry have only 1 or 2 flowers. We never observed more than two flower primordia even at the early stages of flower formation, indicating that the small number of flowers is not a result of early abortion of primordia. Late abortion or mechanical injury of primordia often takes place so that solitary berries are common at maturity. It is interesting, however, that in both experiments, the number of flowers per inflorescence was enhanced by low temperature. Whether this effect was due to enhanced flower initiation or reduced abortion, is not known.
It was also found that ‘Mucurines’, ‘Pax’, and ‘Xenia’ are obligatory SD plants, which initiate flowers only in SD at temperatures ranging from 12 to 24°C, with optimal response at 18°C (; ). This agrees with the results of a range of black currant cultivars grown under the same conditions (Heide & Sønsteby, Citation2011; Sønsteby & Heide, Citation2011). However, floral initiation was delayed at 24°C compared with lower temperatures in ‘Pax’. The critical photoperiod for floral initiation was also similar for the two Ribes species. Under natural daylength conditions at Ås, the transition to generative development (Floral Stage 2) was observed by 3 September in ‘Mucurines’ and ‘Xenia’ at 18°C. When exposed to 10-h SD at the same temperature, it took 2–3 weeks for these cultivars to reach the same stage, indicating that the critical photoperiod was reached around mid-August when the daylength at Ås is about 16 h. This agrees with a critical photoperiod of 16–17 h that was found under the same conditions for three black currant cultivars (Heide & Sønsteby, Citation2011). These results are at variance with the statement by Wright (Citation1985) that floral initiation takes place later in gooseberries than in black currants. In ‘Pax’, however, Floral Stage 2 was reached about 2 weeks later, indicating a shorter critical photoperiod of about 15 h for this cultivar.
As with black currant (Heide & Sønsteby, Citation2011; Tinklin et al., Citation1970), floral initiation was associated with cessation of shoot growth in gooseberry. In both species, the two processes are jointly induced and controlled by the same environmental triggers. It should be noticed, however, that the ‘Pax’ and ‘Xenia’ plants which did not initiate flower primordia under natural daylength conditions at 24°C, still ceased growing at the same time as at lower temperatures (). In Experiment I, it was found that plants in which the floral development process did not progress beyond Stage 5 before the inductive treatment was terminated, flowered poorly in spring. In Experiment II on the other hand, where the plants were exposed to optimal 10-h SD conditions for 8 weeks, and (except for ‘Pax’ at 12 and 24°C) reached Floral Stage 6 well before termination of the treatments, abundant flowering took place in the spring (). Under natural field conditions, however, flower differentiation continues throughout autumn and is complete before winter.
In plants grown under ambient conditions at Apelsvoll (average daily mean temperature of 9.8°C), the rate of shoot growth and the timing of growth cessation were similar to those at 12°C in the phytotron. On the other hand, floral initiation was delayed by a couple of weeks and the level of flowering in the spring was lower in plants grown under ambient conditions (; ). In addition to the difference in temperature, the days are about 1 hour longer at Apelsvoll than at Ås in late August and early September. Together, these differences may explain the discrepancy in flowering. We conclude that the similar responses of the plants in the phytotron and outdoors, indicate that the results in the phytotron are relevant for field conditions.
The delayed spring bud burst observed in both experiments in plants grown at high temperature during the previous autumn concurs with our earlier results with black current (Heide & Sønsteby, Citation2012; Sønsteby & Heide, Citation2011) and appears to be a general phenomenon in trees and shrubs from temperate regions (Heide, Citation2003). High autumn temperatures induce a deep endogenous dormancy in the buds that is manifested in prolonged rest and delayed bud burst. The mechanism may have important implications in nature and may stabilise agricultural plant productivity under global warming by countering premature bud burst in a warming climate (Heide, Citation2003).
In summary, we conclude that cultivated gooseberries are obligate SD plants with a critical photoperiod of 15–16 h at temperatures from 12 to 24°C. As in black currents, floral initiation is associated with cessation of shoot growth, and overall, the environmental control of growth and flowering is similar in the two Ribes species. Much of the available information of flowering physiology in black currants (Sønsteby & Heide, Citation2016) may be relevant also for gooseberries. The results may also identify possible commercial growing areas for the crop in other regions.
Acknowledgments
The authors acknowledge the technical assistance of Kari Grønnerød and Unni M. Roos who assisted with plant cultivation and collection of the data.
Disclosure statement
No potential conflict of interest was reported by the author(s).
Additional information
Funding
References
- Heide, O.M. (2003). High autumn temperature delays spring bud burst in boreal trees, counterbalancing the effect of climate warming. Tree Physiology, 23, 931–936. doi:https://doi.org/10.1093/treephys/23.13.931.
- Heide, O.M., & Sønsteby, A. (2011). Critical photoperiod for short-day induction of flowering in black currant (Ribes nigrum L.). The Journal of Horticultural Science and Biotechnology, 86, 128–134. doi:https://doi.org/10.1080/14620316.2011.11512737.
- Heide, O.M., & Sønsteby, A. (2012). Floral initiation in black currant cultivars (Ribes nigrum L.): Effects of plant size, photoperiod, temperature, and duration of short day exposure. Scientia Horticulturae, 138, 64–72. doi:https://doi.org/10.1016/j.scienta.2012.02.008.
- Kvam, G. (1986). Vegetative and generative eigenskapar hjå solbær (Ribes nigrum L.) med vekt på ti sortar (M. Sc. Thesis, Department of Plant and Environmental Sciences, Norwegian University of Life Sciences, Ås, Norway). 84. (in Norwegian).
- Maxfield, G.B., Wallis, M., Harrison, S.G., & Nicholson, B.E. (1969). The oxford book of food plants. Oxford, UK: Oxford University Press.
- Nasr, T.A.A., & Wareing, P.F. (1958). Photoperiodic induction of flowering in black currant. Nature, 182, 269. doi:https://doi.org/10.1038/182269a0.
- Palonen, P., & Voipio, I. (1994). Floral buds, number of flower initials and fruit set in redcurrant (Ribes rubrum L.). Scientia Horticulturae, 58, 187–196. doi:https://doi.org/10.1016/0304-4238(94)90150-3.
- Rivero, R., Sønsteby, A., Heide, O.M., Måge, F., & Remberg, S.F. (2017). Flowering phenology and the interrelations between phenological stages in apple trees (Malus domestica Borkh.) as influenced by the Nordic climate. Acta Agriculturae Scandinavica, Section B – Soil and Plant Sciences, 67, 278–283.
- Sønsteby, A., & Heide, O.M. (2011). Elevated Autumn temperature promotes growth cessation and flower formation in black currant cultivars (Ribes nigrum L.). The Journal of Horticultural Science and Biotechnology, 86, 120–127. doi:https://doi.org/10.1080/14620316.2011.11512736.
- Sønsteby, A., & Heide, O.M. (2016). Black currant physiology in a changing climate. Acta Horticulturae, 1133, 159–170. doi:https://doi.org/10.17660/ActaHortic.2016.1133.24.
- Tinklin, I.G., Wilkinson, E.H., & Schwabe, W.W. (1970). Factors affecting flower initiation in the black currant ribes nigrum (L.). The Journal Horticultural Science, 45, 275–282. doi:https://doi.org/10.1080/00221589.1970.11514355.
- Wright, J.W. (1985). Ribes. In: A.H. Halevy (Ed.), CRC handbook of flowering (Vol. IV, pp. 198–201). Boca Raton, FA: CRC Press.
- Yeo, I., & Johnson, R.A. (2000). A new family of power transformations to improve normality or symmetry. Biometrika, 87, 954–959. doi:https://doi.org/10.1093/biomet/87.4.954.
- Zhou, Y., Zhang, D., Huang, H., & Xue, Y. (2022). Effect of normal transformation methods on performance of multivariate normal distribution. ASCE-ASME Journal of Risk and Uncertainty in Engineering Systems, Part A: Civil Engineering, 8, 04021074. doi:https://doi.org/10.1061/AJRUA6.0001198.