Abstract
The alignment behaviour of a nematic‐columnar phase forming discotic liquid crystal was explored on various substrates. The mesogen employed was a new star‐shaped monodisperse oligomesogen characterized by three flat radial pentaalkynylbenzene moieties grafted to a central benzene linking unit via flexible alkyl spacers. It is shown that suitable polyimide orientation layers along with the mesomorphic properties give rise to a tilted alignment of the disc‐shaped pentayne cores. The photoluminescence spectra of oriented films were lineary polarized upon isotropic excitation and unambigiously reveal that, beside the tilted alignment of the rigid cores, the molecular directors of the discotic building blocks preferentially are oriented along the rubbing direction of the orientation layer. The three‐dimensional ordering is stable even at room temperature due to the glass forming properties of the applied discotic mesogen.
1. Introduction
In recent years discotic liquid crystals have emerged considerable attention because of their unique optic and electronic properties like charge migration phenomena along a column axis Citation1 Citation2. As a result, discotic mesogens are considered for use in electronic devices such as photoconducting layers Citation1 Citation3, organic light‐emitting diodes Citation4 Citation5 and organic field effect transistors Citation6 Citation7. For device applications the orientation control of discotic liquid crystals is a crucial factor. The mesogenic cores have to be aligned uniformly in the desired configuration within a monodomain.
Surprisingly, compared with alignment control of conventional calamitic, in particular, nematic liquid crystals Citation8 Citation9 reports on surface alignment of discotic mesogens are less frequent.
Thus, columnar discotic liquid crystals such as alkoxy substituted triphenylenes show a strong tendency towards a homeotropic alignment Citation10–12. Hexagonal columnar (Colh) phase forming fluoroalkylated triphenylenes were also reported to align homeotropically on various substrates Citation13 whereas columnar stacks of alkyl substituted hexabenzocoronenes and triphenylenes, respectively, lie parallel to a poly(tetrafluoroethylene) surface Citation14 Citation15. Molecular alignment switching of discotic triphenylenes could be achieved, i.e., by means of an external magnetic field Citation16 or by vibrational excitation Citation17 Citation18. Molecular hybrid orientation proved to be successful for nematic‐discotic (ND) mesogens based on a triphenylene core by photoalignment technique Citation19 and on polymer‐coated substrates Citation20 leading to the invention of an optical compensator for improvement of the narrow viewing angle of liquid‐crystal displays Citation21.
We have shown that benzene centred nematic‐discotic multiynes substituted with one terminal polar group form two‐dimensional monolayer assemblies at the air/water interface with the flat aromatic planes oriented perpendicular to the surface Citation22–24. The edge‐on orientation is preserved during the formation of periodical multilayers by the LB technique Citation23 Citation24. More recently we reported on photoalignment of low molar mass nematic‐discotic pentaalkynyl‐benzene derivatives on an azobenzene sub‐layer Citation25 Citation26.
A problem regarding long‐term stability of the oriented films, however, may arise from the tendency of the monomeric radial multialkynes to crystallize upon cooling and storing at room temperature. For calamitic liquid crystals it is known that grafting a defined number of rod‐shaped sub‐units to a central linker may lead to a supression of cystallization Citation27 Citation28. Following this concept we have synthezised new discotic oligomesogens with three flat radial pentaalkyne building blocks linked to a central benzene moiety via flexible alkyl spacers.
In this contribution we focus on the alignment behaviour of the nematic‐columnar (NCol) propyl substituted star‐shaped pentayne oligomer 1 (figure ) on non‐treated and on polyimide modified substrates. To our best knowledge, investigations regarding molecular orientation control of discotic liquid crystals displaying a NCol phase have not been reported yet.
2. Results and discussion
The star‐shaped monodisperse pentayne trimer 1 with three radial multiyne groups linked to a central benzene ring via ester bonds exhibits a glass transition at 24.0°C and a phase transition from the nematic columnar phase into the isotropic liquid at 141.9°C. Full details regarding the synthesis and the mesomorphic structure formation of compound 1 and of further homologues will be presented elsewhere Citation29. The nematic columnar bulk phase of the pentayne 1 is presented schematically in figure .
Figure 2 Structure model of the nematic columnar(NCol) bulk mesophase formed by the star‐shaped pentayne oligomer 1.
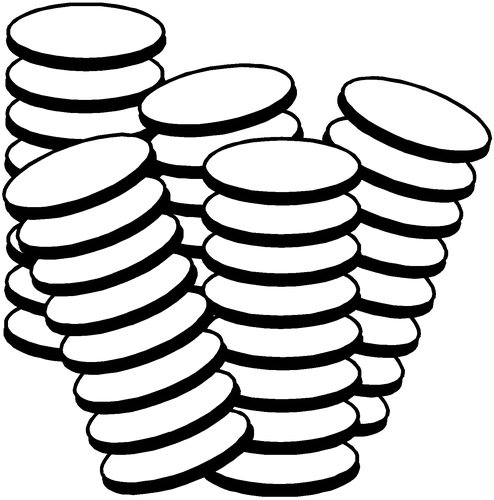
Thin films of the star pentayne 1 with a thickness of 0.25–1.5 µm first were prepared by spin‐coating on glass or quartz substrates without alignment layers. As evident from optical microscopy the films are transparent. The rapid film formation clearly suppresses the formation of liquid crystalline textures which would lead to scattering. Heating the samples into the temperature range of the mesophase and subsequently cooling down slowly (1 K min−1) led to the development of optical Schlieren and marbled textures (figure ). No crystallization was detectable by polarizing microscopy which is in agreement with the calorimetric measurements. The optical textures are similar to those observed for bulk samples between glass slides and indicate the formation of the NCol phase but without a uniform director orientation of the discotic groups in a monodomain.
Figure 3 Schlieren texture of a spin‐coated pentayne 1 film on glass substrate between crossed polarizers after thermal treatment within the mesophase and cooling down to room temperature.
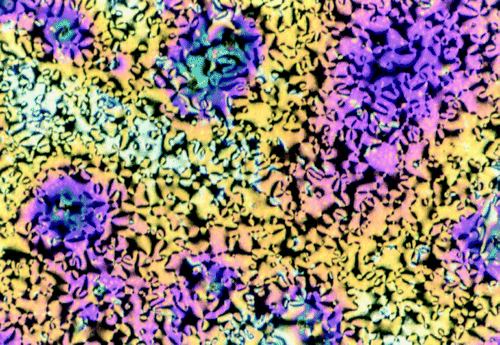
It has been demonstrated that ND phase forming triphenylenes can be aligned on top of polyimide orientation layers Citation20 and we assumed that rubbed polymer films might allow orientation control of the nematic columnar star multiyne 1 investigated here. Two commercial polyimides, rubbed in one direction, SE‐3140 (Nissan) and Liquicoat PI Kit ZLI‐2650 (Merck), respectively, were employed which are widely used as coating reagent to obtain alignment of rod‐like liquid crystals. Spin coated films of the pentayne trimer 1 with a thickness range from approximately 1 µm to 5 µm were prepared on the polyimide sub‐layers. Thermal treatment was performed as described above for films without alignment layer.
On cooling thin films of compound 1 on top of SE‐3140, optical textures develop which do not resemble a typical nematic Schlieren texture anymore. An example is given in figure .
The elongated disclination lines are predominantly aligned along the rubbing direction of the polyimide sub‐layer. This finding suggests that the polyimide layer along with the thermal treatment induces a preferred orientation of the aromatic discs of compound 1. More homogeneous grayish‐blue textures without remarkable disclination defects were observed for thermally treated films of the pentayne 1 on ZLI‐2650 (figure ) which may be indicative for a more pronounced uniform alignment of the flat disc‐shaped cores of the star oligomer 1 in a (mono)domain. Considering the birefringence, a homeotropic orientation of the anisometric pentayne moieties can be excluded. Rotation of the samples through crossed polarizers gave rise to an alternately appearance of darkness with a periodicity of 90° (figure ). However, solely based on polarizing microscopy we cannot distinguish satisfactory wether the director uniformly aligns parallel to the surface or if a tilted alignment is present.
Figure 4 Optical textures of spin‐coated films of the star‐shaped oligomesogen 1 on polyimide alignment layers at room temperature after annealing within the mesophase; a) Polyimide SE‐3140. The disclination lines run predominantly parallel to the rubbing direction of the orientation layer; b) Polyimide ZLI‐2650; c) after rotation of the sample in b) by 45° arround the optical axis.
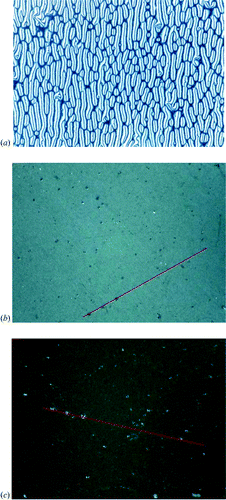
To clarify the alignment, anisotropy measurements were performed. An annealed film of the star pentayne 1 with a thickness of 3.9 μm on SE‐3140 was investigated by waveguide spectroscopy to determine the range of possible refractive indices. The measurement using s‐polarised light did not show any waveguide modes for angles beyond the angle of total reflection between the prism and the glass substrate (refractive index around 1.5). Therefore it can be deduced, that the lower principal refractive index was lower than 1.5. The measurement using p‐polarised light showed waveguide modes above the external angle of 90° leading to the conclusion that the higher principal refractive index was higher than 1.71.
To elucidate the out‐of‐plane orientational director of the pentayne groups of the aligned glassy films, transmission ellipsometry in dependence on the incident angle was performed by rotating the sample along the rubbing direction of the polyimide orientation layer. Figure displays the resulting retardation values for a 1.1 µm film on ZLI‐2650. The experimental results were fitted to a negatively uniaxial model. Supposing the limiting value of 1.5 for the extraordinary (lower) refractive index, the fitting procedure yielded a birefringence of 0.46 and an average tilt angle of 12.6° between the film normal and the molecular director of the pentayne discs. Accordingly, by assuming that the ordinary (higher) refractive index amounts to 1.71, the fit resulted in a birefringence of 0.37 and an average tilt angle of 14.2°. The real values of birefringence and tilt angles can therefore be expected to be in between these limiting values.
Figure 5 (x) Retardation of a 1.1 µm thick film of compound 1 depending on the incidence angle after thermal treatment on ZLI‐2650 polyimide layer. (+) is a fit of a negatively uniaxial tilted model to the data. The values for incidence angles from 35°–50° were determined at a azimuth differing by 6° from the linear eigenpolarisation and were excluded from the fitting procedure.
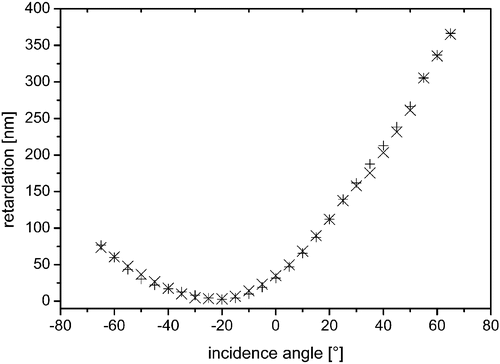
A tilted alignment of the pentayne cores of compound 1 was also proven after annealing on SE‐3140 polyimide orientation layer. The average pre‐tilt angle of the planes of the extended discs was estimated by a crystal rotation measurement Citation30 to be 14.6°±3.4° with respect to the substrate surface. Thus, the tilt angles of the aromatic pentayne cores do not differ significantly for both polyimide orientation layers.
A schematic presentation of the molecular orientation of the star pentayne 1 with a uniform tilt angle of the extended flat cores relative to the film plane is given in figure . However, we cannot exclude that the directors tilt angles continuously alter within the film in order to minimize the free energy of the outermost film/air surface Citation19 Citation20 Citation25 Citation26 as shown schematically in figure .
Figure 6 Schematic presentation of possible molecular orientations of the discotic pentayne cores of the star‐shaped oligomesogen 1 after annealing on top of a rubbed polyimide layer; a) Alignment with a uniform tilt angle; b) Splayed orientation with continuous alteration of the tilt angle. The macroscopic orientation is frozen in a glassy state at room temperature.
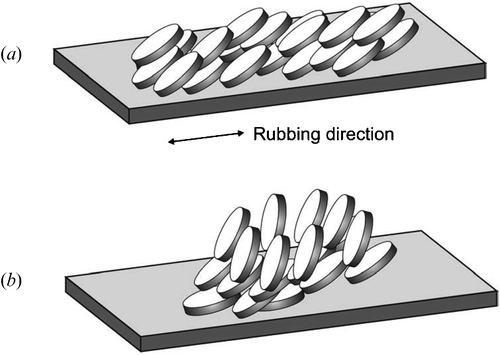
The absorption spectrum of the star oligomer 1 in chloroform solution shows a high‐intensity peak at 340 nm and two additional shoulders at 380 nm and 420 nm, respectively. The absorption bands are mainly caused by a superposition of several π‐π* transitions of the pentaalkyne moieties. The photoluminescence spectrum displays an intensive band with the emission maximum at 450 nm.
The absorption spectra of thin films of compound 1 are practically identical to the solution spectrum. Contrary, the emission peak was red‐shifted (ca. 10 nm) for films thermally treated within the mesophase and subsequently cooled down to the glassy state at room temperature. This behaviour can be attributed to an enhancement of interchromophoric interactions due to the liquid crystalline self‐organization and to excimer formation due to overlapping of the aromatic rings even in the anisotropic glassy state. A similar behaviour has been observed for discotic compounds exhibiting a hexagonal columnar mesophase Citation31 Citation32.
Polarized emission spectra of aligned samples of the pentayne trimer 1 (figure ) were recorded under isotropic excitation at 360 nm. The emitted light was detected through a linear polarizer. The optical axis of the polarizer was oriented parallel (p) and perpendicular (s), respectively, to the director of polyimide orientation layer.
Figure 7 Polarized emission spectra of an aligned film of the pentayne star oligomer1 on polyimide ZLI‐2650 by excitation with non‐polarized 360nm‐light. The polarizer was oriented perpendicular (straight line) and parallel (dotted line) to the director of the orientation layer.
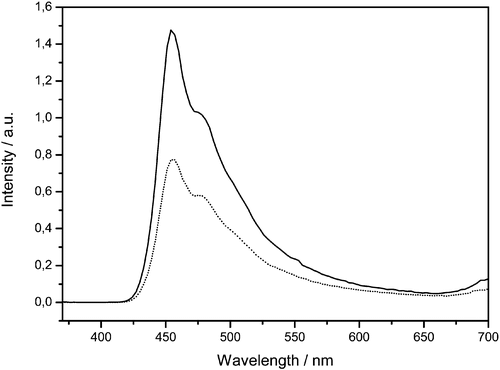
The finding is that the photoluminescence of the oriented films is lineary polarized. Thereby, s‐polarized light emits preferentially in comparison with the p‐polarized component. We have to consider that the main transition dipol moment of the pentaalkyne groups is in the plane of the disc‐shaped moieties and therefore perpendicular to the flat anisometric cores. Thus, the results confirm the molecular orientation shown schematically in figure . The disc‐shaped pentayne moieties organize on the polyimide layers such that the anisometric cores are tilted relative to the substrate plane whereby the molecular directors preferably coincide with the director of the alignment layer.
The emission dichroic ratio, defined as the ration of the intensity of the s‐polarized and p‐polarized components of the emitted light was estimated to be about 2.0 at the wavelength of the emission maximum (455 nm). This intensity ratio is of the same order of magnitude like those previously reported for polarized photoluminescence emission of splayed oriented films of monomeric nematic‐discotic multiynes Citation26 and of uniaxially oriented columnar triphenylenes Citation15.
3. Conclusions
The nematic columnar phase forming oligomesogen 1 represents a new family of monodisperse star‐shaped liquid crystals composed of three disc‐shaped penta‐alkyne building blocks attached to a central linking unit via flexible alkyl spacers. Due to the covalent linkage of the three anisometric moieties the compound does not crystallize but forms stable anisotropic glasses. A uniformly rubbed polymer orientation layer along with the mesomorphic properties gives rise to a tilted alignment of the NCol phase forming pentayne based star‐shaped oligomer which is stable even at room temperature due to the glass forming properties. Independent on the polyimide alignment layers used here the disc‐shaped moieties were tilted with their molecular plane by approximately 14° with respect to the surface plane. The surface assisted orientation differs remarkably from the original nematic columnar bulk phase of the pentayne star trimer 1. The results, furthermore, show that a tilted orientation of disc‐shaped cores induced by surface alignment is not restricted to nematic‐discotic phase forming mesogens. The effect seems to be more general provided that the discotic molecules or sub‐units do not assemble to columnar stacks with the columns aligned parallel on a two‐dimensional lattice.
4. Experimental
Absorption spectra were measured with a UV‐vis spectrometer Lambda 19 (Perkin Elmer). Emission spectra were recorded using a fluorescence spectrometer Perkin Elmer LS 50 B. Texture observations were made with an Olympus polarizing microscope fitted with a Linkam TMH/S 600 hot stage in conjunction with a Linkam TP 92 control unit. Photo micrographs were obtained with an Olympus OM‐4 Ti system camera and with an Olympus E 20 digital camera, respectively. Thickness of the films was measured with a profilometer alpha‐step 200 (Tencor Instruments). Waveguide spectroscopy was performed using a LaSF N9 prism with a refractive index of 1.84489. The retardation values in dependence on the incidence angle were determined at a wavelength of 632.8 nm using a null ellipsometer in transmission. The azimuths of the linear eigenpolarisations were determined by generalised ellipsometry Citation33 at normal incidence before the measurement at oblique incidence and the sample was aligned accordingly. Rotation was performed around the in‐plane axis perpendicular to the in‐plane linear eigenpolarisation, which was closer to the rubbing direction. Polarized fluorescence spectra were recorded with a special set‐up Citation34 equipped with a Xe lamp (XBO 1000 W; Osram) and two monochromators (Spectra Pro 300 I; Actron Reasearch) controlled by a NCL single channel spectroscopy detection system (Actron Research). The excitation beam was positioned at 30° to the normal and on the same side of the film as the emission detector (photoncounter R 4632; Hamamatsu). A Glan‐Taylor polarizer (Coherent) was located between sample and detector and with the polarizer aligned parallel and perpendicular to the rubbing direction. The films were excited with non‐polarized light (λex = 360 nm).
Acknowledgement
The financial support of the Bundesministerium für Wirtschaft und Technologie (KF 0020803KFK0) and of the Fonds der Chemischen Industrie are kindly acknowledged. The authors are very grateful to Dr. G. Lüssem, Merck KGaA Darmstadt, Germany, for providing rubbed polyimide SE‐3140 substrates. We wish to thank N. Füchtjohann, AK Prof. J.H. Wendorff, for his kind assistance in performing the polarized fluorescence measurements at the Institute of Physical Chemistry, Philipps University Marburg, Germany.
References
- Simmerer , J. , Glüsen , B. , Paulus , W. , Kettner , A. , Schuhmacher , P. , Adam , A. , Etzbach , K. H. , Siemensmeyer , K. , Wendorff , J. H. , Ringsdorf , H. and Haarer , D. 1996 . Adv. Mater. , 8 : 815
- Adam , D. , Schuhmacher , P. , Simmerer , J. , Häussling , L. , Siemensmeyer , K. , Etzbach , K. H. , Ringsdorf , H. and Haarer , D. 1994 . Nature , 371 : 141
- Haarer , D. , Adam , D. , Simmerer , J. , Closs , F. , Funhoff , D. , Häussling , L. , Siemensmeyer , K. , Ringsdorf , H. and Schuhmacher , P. 1994 . Mol. Cryst. Liq. Cryst. Sci. Technol. , 252–253A : 155
- Benning , S. A. , Haßheider , T. , Keuker‐Baumann , S. , Bock , H. , Sala , F. , Frauenheim , T. and Kitzerow , H. ‐S. 2001 . Liq. Cryst. , 28 : 1105
- Christ , T. , Glüsen , B. , Greiner , A. , Kettner , A. , Sander , R. , Stümpflen , V. , Tsukruk , V. and Wendorff , J. H. 1997 . Adv. Mater. , 9 : 48
- Dyreklev , P. , Gustafsson , G. , Inganäs , O. and Stubb , H. 1992 . Solid State Commun. , 82 : 317
- Sirringhaus , H. , Wilson , R. J. , Friend , R. H. , Inbasekaran , M. , Wu , W. , Woo , E. P. , Grell , M. and Bradley , D. D. 2000 . Appl. Phys. Lett. , 77 : 406
- Jerome , B. 1998 . Handbook of Liquid Crystals , Edited by: Demus , D , Goodby , J , Gray , G. W , Spiess , H. ‐W and Vill , V . Weinheim : Wiley‐VCH .
- Ichimura , K. 2000 . Chem. Rev. , 100 : 1847
- Christ , T. , Geffarth , F. , Kettner , A. , Lüssem , G. , Schäfer , O. , Stümpflen , V. , Wendorff , J. H. and Tsukruk , V. V. 1997 . Thin Solid Films , 302 : 214
- Plesnivy , T. , Ringsdorf , H. , Schuhmacher , P. , Nütz , U. and Diele , S. 1995 . Liq. Cryst. , 18 : 185
- Destrade , C. , Foucher , P. , Gasparoux , H. , Tinh , N. H. , Levelut , A. M. and Malthete , J. 1984 . Mol. Cryst. Liq. Cryst. , 106 : 121
- Terasawa , N. , Monobe , H. , Kiyohara , K. and Shimizu , Y. 2003 . Chem. Commun. , : 1678
- van de Craats , A. M. , Stutzmann , N. , Bunk , O. , Nielsen , M. M. , Watson , M. , Müllen , K. , Chanzy , H. D. , Sirringhaus , H. and Friend , R. H. 2003 . Adv. Mater. , 15 : 495
- Zimmermann , S. , Wendorff , J. H. and Weder , C. 2002 . Chem. Mater. , 14 : 2218
- Ikeda , S. , Takanishi , Y. , Ishikawa , K. and Takezoe , H. 1999 . Mol. Cryst. Liq. Cryst. , 329 : 589
- Monobe , H. , Awazu , K. and Shimizu , Y. 2000 . Adv. Mater , 12 : 1495
- Monobe , H. , Kiyohara , K. , Terawasa , N. , Heya , M. , Awazu , K. and Shimizu , Y. 2003 . Chem. Lett. , 32 : 870
- Ichimura , K. , Furumi , S. , Morino , S. , Kidowaki , M. , Nakagawa , M. , Ogawa , M. and Nishiura , Y. 2000 . Adv. Mater. , 12 : 950
- Kawata , K. 2002 . Chem. Record , 2 : 59
- Mori , H. , Itoh , Y. , Nishiura , Y. , Nakamura , T. and Shinagawa , Y. 1997 . Jpn. J. Appl. Phys. , 36 : 143
- Janietz , D. , Ahuja , R. C. and Möbius , D. 1997 . Langmuir , 13 : 305
- Reiche , J. , Dietel , R. , Janietz , D. , Lemmetyinen , H. and Brehmer , L. 1993 . Thin Solid Films , 226 : 265
- Janietz , D. , Hofmann , D. and Reiche , J. 1994 . Thin Solid Films , 244 : 794
- Furumi , S. , Janietz , D. , Kidowaki , M. , Nakagawa , M. , Morino , S. , Stumpe , J. and Ichimura , K. 2001 . Mol. Cryst. Liq. Cryst. , 368 : 517
- Furumi , S. , Janietz , D. , Kidowaki , M. , Nakagawa , M. , Morino , S. , Stumpe , J. and Ichimura , K. 2001 . Chem. Mater. , 13 : 1434
- Chen , S. H. , Mastrangelo , J. C. , Blanton , T. N. , Bashir‐Hashemi , A. and Marshall , L. K. 1996 . Liq. Cryst. , 21 : 683
- Fan , F. Y. , Culligan , S. W. , Mastrangelo , J. C. , Katsis , D. and Chen , S. H. 2001 . Chem. Mater. , 13 : 4584
- Grafe , A. , Janietz , D. , Frese , T. and Wendorff , J. H. in press . Chem. Mater. ,
- Markovitsi , D. , Germain , A. , Millié , P. , Lecuyer , P. , Gallo , L. K. , Argyrakis , P. , Bengs , H. and Ringsdorf , H. 1995 . J. Phys. Chem. , 99 : 1005
- Uznanski , P. , Marguet , S. , Markovitsi , D. , Schuhmacher , P. and Ringsdorf , H. 1997 . Mol. Cryst. Liq. Cryst. , 293 : 123
- Scheffer , T. J. and Nehring , T. 1977 . J. Appl. Phys. , 48 : 1783
- Jung , C. 2004 . Germany : PhD Thesis, Potsdam University .
- Bayer , A. 2003 . Germany : PhD Thesis, Phillips University Marburg .