Abstract
Although the deleterious impact of chemotherapy regimen used to treat women of reproductive age with breast cancer on ovarian reserve has been extensively studied, hardly anything has been reported on the effect of these protocols on theca cell function and ovarian androgen secretion. The aim of this prospective multicentric cohort study was to describe serum levels of total testosterone and androstenedione during chemotherapy and 24-month follow-up in 250 patients <40 years treated for breast cancer. Mean basal levels of androstenedione and total testosterone at diagnosis were 1.68 ng/mL and 0.20 ng/mL respectively. No correlation with age was found. Serum levels of androstenedione and total testosterone rapidly decreased after chemotherapy completion, before slowly increasing and almost returning to basal levels in all patients during 2-year follow-up. In conclusion our study demonstrates a chemotherapy-induced alteration of ovarian thecal function, resulting in a significant decrease in serum androgen levels. This alteration of theca cell function adds to the well-known alteration of granulosa cell function, resulting in a global, but partly transient, ovarian failure in young women treated for breast cancer. These data bring new insight into ovarian physiology and emphasize the need for pre and post-treatment ovarian follow-up. Trial registration: ClinicalTrial.gov identifier NCT01114464
Introduction
Breast cancer stands as the most prevalent form of cancer among women globally (Ghoncheh et al., Citation2016). While its incidence has seen a steady rise in recent decades, a noteworthy decline in mortality has concurrently been observed, attaining a commendable 5-year survival rate of 88% (Cronin et al., Citation2018; Early Breast Cancer Trialists’ Collaborative Group (EBCTCG), Citation2005; Eifel et al., Citation2001). Women in their reproductive years constitute approximately 25% of breast cancer cases and confront a prognosis less favourable than their older counterparts (Chung et al., Citation1996). Consequently, a systematic combination of surgical treatment and neoadjuvant chemotherapy has become the norm (Fidler et al., Citation2017; Goldhirsch et al., Citation1998). This improved prognosis, however, has given rise to heightened concerns about post-remission well-being and quality of life, notably in terms of reproductive health. A majority of women under 45 treated for breast cancer indeed express a desire for childbearing following recovery (Partridge & Ruddy, Citation2007).
Extensive documentation exists on the chemotherapy-induced impact on female fertility during breast cancer treatment (Anderson et al., Citation2018; Blumenfeld, Citation2012; Mastro et al., Citation2006; Partridge & Ruddy, Citation2007). Nevertheless, the effects on gametogenesis, intrinsic gamete quality, and overall reproductive function vary significantly. The influence of chemotherapy on ovarian function is contingent upon factors such as age, chemotherapy type and dosage, and individual susceptibility (Sklar et al., Citation2006). While the dynamics of serum Anti-Müllerian Hormone (AMH) in women undergoing chemotherapy have been well-documented (Dewailly et al., Citation2014; Jamil et al., Citation2016; Kim et al., Citation2018; Lambertini et al., Citation2017; Citation2019; Rosendahl et al., Citation2010), studies on circulating androgens in this population are surprisingly scarce.
Androgens, playing a crucial role in reproductive function (Lebbe & Woodruff, Citation2013; Walters, Citation2015), are implicated in women’s reproductive health disorders when present in excessive or insufficient levels (Lucis et al., Citation1966; Pache & Fauser, Citation1993). Comprising both precursors (DHEAS, DHEA, androstenedione) and active molecules (testosterone and DHT), androgen synthesis occurs in both the ovaries (thecal cells) and adrenal glands. Generally, it is acknowledged that both organs contribute equally to circulating androstenedione levels (Davison & Davis, Citation2003). Regarding testosterone, approximately half of the circulating levels arise from theca cells and adrenal glands, while the other half originates from peripheral conversion of precursors like androstenedione in skin, muscles, liver, or adipocytes (Burger, Citation2002).
Chemotherapy-induced alterations in ovarian function impact all follicular cell types, disrupting both granulosa and theca cells and ultimately affecting hormonal functions through direct and indirect damage (Morgan et al., Citation2012). Androgens have been observed to primarily influence early folliculogenesis, which coincides with the main period of AMH synthesis (Walters et al., Citation2019). Moreover, their levels decrease with increasing female age (Davison et al., Citation2005), suggesting a potential similar pattern for AMH and androgens throughout ovarian function (Drakopoulos et al., Citation2021), particularly during and after chemotherapy.
Recognizing the significant role of androgens in reproductive function and their potential parallels with AMH, we undertook, for the first time, a longitudinal study with the aim of elucidating the dynamics of serum testosterone and androstenedione in a sizable cohort of reproductive-age women diagnosed with breast cancer and undergoing chemotherapy.
Material and methods
Patients
This work is based on the RESOVA study described elsewhere (Dezellus et al., Citation2017). Briefly, this observational, multicentric, prospective study (ClinicalTrial.gov identifier NCT01114464) was conducted in 250 eligible patients, aged 18-40, diagnosed with breast cancer (T0-T4, N1-N3, M0) and about to be treated with chemotherapy in adjuvant/neoadjuvant setting. The standard polychemotherapy sequential ‘FEC→Taxane’ regimen consisted in 3 consecutive ‘FEC’ cycles (5-Fluorouracil 500 mg/m2, Epirubicin 100 mg/m2, Cyclophosphamide 500 mg/m2), followed by 3 cycles of taxanes (docetaxel 100 mg/m2), repeated every 21 days. The exclusion criteria were menopause, breast cancer not treated with chemotherapy, history of previous malignancy treated with chemotherapy, and comorbidities related to fertility. All patients provided written informed consent before starting study procedure.
Study design
Patients were included at diagnosis and followed up until 24 months after chemotherapy completion. A medical interview and blood sampling were performed for each patient at diagnosis (sample S0), before each cycle of chemotherapy (sample S1 - S6) and 6, 12 and 24 months after completion of chemotherapy treatment (samples S7, S8 and S9). For this study, only samples S0 (diagnosis), S6 (end of chemotherapy), S8 (12-month follow up) and S9 (24-month follow up) were used for androgens assays (). Patients were also asked to register all menstruations occurrences throughout the study.
Androgens assays
Peripheral blood was collected and processed within 2 h. After clotting and centrifugation, serum was aliquoted and stored at −80 C°. All assays were performed in the same centralized laboratory from January to August 2019 with LIAISON® Androstenedione and Testosterone assay according to the manufacturer instructions. Each series of 100 assays was validated by 2 internal controls at the start and the end of the series.
Serum androstenedione and testosterone concentrations were measured in the same time. Androstenedione concentration is presented in ng/mL (conversion factor to nmol/L = 3.49 ng/mL). The lower limit of detection was 0.24 ng/mL. The lower limit of quantification (LoQ) was 0.32 ng/mL. The analytical measurement range was 0.24-10 ng/mL. Repeatability (intra-assay precision) and reproducibility (inter-assay precision) coefficients were between from 1% to 2.6% and from 2.4% to 9.7%, respectively. Testosterone concentration are presented in ng/mL (conversion factor to nmol/L = 3.47 ng/mL). The lower limit of detection was 0.10 ng/mL. The lower limit of quantification (LoQ) was 0.16 ng/mL. The analytical measurement range was 0.05-15 ng/mL. Repeatability (intra-assay precision) and reproducibility (inter-assay precision) coefficients were between from 3.2% to 7.3% and from 7.1% to 14%, respectively.
Statistical analysis
The value of the lower limit of detection was arbitrarily assigned to every value below it, i.e. serum androstenedione level <0.24 ng/mL was assigned to 0.24 ng/mL, and serum testosterone level <0.10 ng/mL was assigned to 0.10 ng/mL. The variation of serum androgens level between basal level (S0) and any other time point (S6, S8 or S9) was calculated as the ratio between the 2 androgens values according to the following formula: DeltaS0Sx = ((SxS0) * 100)/(S0-0.24) for androstenedione and DeltaS0Sx = ((SxS0) * 100)/(S0-0.10) for testosterone. Any androstenedione level <0.24 ng/ml or testosterone level <0.10 ng/ml achieved during chemotherapy led to a decrease rate of 100%. The variation of serum androgens level between 2 time points was calculated according to the following formula: DeltaSxS(x + 1) = deltaS0S(x + 1) -deltaS0Sx.
Mann-Whitney and Kruskall-Wallis tests were used for group comparison and Spearman coefficient for correlation studies. GraphPad Prism (version 5) software was used. A p value < 0.05 was considered statistically significant.
Ethical approval
This study has been approved by the local ethics committee (GNEDS). All patients provided written informed consent before starting study procedure.
Results
The demographic and treatment characteristics of the 250 women included in the initial study are presented in . Most of patients were aged 35- 40 years. A total of 188 patients had at least one child before diagnosis. Average weight was 64.6 ± 0.9 kg at diagnosis and 66.2 ± 1.2 kg at the end of study, 24 months after chemotherapy completion. Over the study period, average weight gain was 3.1 ± 0.5 kg (IC95% [2.0-4.1] median 2.0 ng/mL). Patients had a predominant diagnosis of invasive ductal carcinoma (92%) of grade II-III (94%). Most women (88%) received a standard polychemotherapy sequential ‘FEC→Taxane’ regimen. Only one women received anti-aromatase treatment.
Table 1. Demographic, histological and treatment characteristics.
The serum concentration of both androgens over the study period from inclusion (S0) to the end of the study (S9) followed a biphasic evolution, with a significant decrease between diagnosis and chemotherapy completion (sample S6) followed by a recovery during the 2-year follow-up (samples S8 and S9) () (p < 0.0001). More specifically, mean basal androstenedione level at diagnosis was 1.68 ng/mL (IC95% [1.57-1.78] median 1.62 ng/mL) (). No significant correlation with age at diagnosis was found (Figure S1A). The androstenedione level fell to 0.6 ng/mL (IC95% [0.54-0.67] median 0.41 ng/mL) after chemotherapy completion (sample S6) (), before re-ascending to 1.37 ng/mL (IC95% [1.27–1.47] median 1.25 ng/mL) and 1.40 ng/mL (IC95% [1.31–1.49] median 1.34 ng/mL) after 1-year and –year follow up respectively (). The calculated cumulative decrease in androstenedione level was −72% (IC95% [−78; −68%], median −86%) (p < .05) after chemotherapy completion and the average increase in serum androstenedione level during the 2-year follow up after chemotherapy completion was +71% (IC95% [60–82%], median 57%) (p < .05) (), leading to a return to serum level almost comparable (although significantly different) to basal androstenedione concentration after 2-year follow-up (−5%) (IC95% [−15;4] median −20%) ().
Figure 2. Evolution and relative variation of serum androstenedione (A,C) and total testosterone levels (B,D) during chemotherapy and 24-month follow-up after chemotherapy completion. *:p < 0.05.
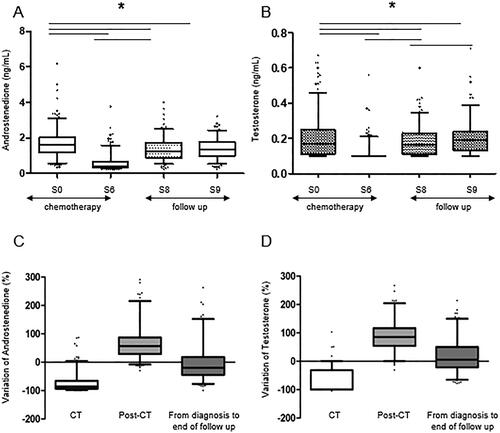
Mean basal total testosterone level at diagnosis was 0.20 ng/mL (IC95% [0.19–0.22], median 0.17 ng/mL) (). No significant correlation with age at diagnosis was found (Figure S1B). The total testosterone level fell to 0.12 ng/mL (IC95% [0,11-0,12] median 0,10 ng/mL) after chemotherapy completion (sample S6) (), before re-ascending to 0.18 ng/mL (IC95% [0,17–0,20] median 0,16 ng/mL) and 0.20 ng/mL (IC95% [0,19–0,22] median 0,19 ng/mL) after 1-year and 2-year follow up respectively (). The calculated cumulative decrease in total testosterone level was −62% (IC95% [−82; −43], median −100) after chemotherapy completion and the average increase in serum total testosterone level during the 2-year follow up after chemotherapy completion was +90.5% (IC95% [53; 117], median 85%), leading to a global variation of +14% (IC95% [−7;22.9], median 5%) between diagnosis and end of study ().
Adjuvant endocrine therapy with tamoxifen showed no effect on androstenedione and testosterone hormone recovery levels after chemotherapy. No significant difference was found in androstenedione recovery levels for patients with or without endocrine therapy (73.2% vs 66.1% respectively, (IC95% [−16.6–30.8], p = 0.5584) or in testosterone recovery levels for patients with or without endocrine therapy (90.2% vs 89.13% respectively, (IC95% [−18.8–20.8], p = 0.918).
The prevalence of chemotherapy-induced amenorrhea (CIA) was 92.4% immediately after CT completion and 64.3% after 12-month follow-up and 59,3%. after 2-year follow-up. Patients with persisting CIA after 1-year follow-up were significantly older than those resuming menses (36.04 ± 3.05 versus 33.93 ± 4.33 years, p = 0.001). Both groups had comparable basal androstenedione and testosterone levels at inclusion, i.e. 1.80 ng/mL (IC95% [1.63–1.98] median 1.75 ng/mL), and 0.21 ng/mL (IC95% [0.19–0.23] median 0.17 ng/mL) in women with resuming menses versus 1.57 ng/mL (IC95% [1.44–1.71] median 1.55 ng/mL), and 0.20 ng/mL (IC95% [0.17–0.22] median 0.15 ng/mL). However, women with persisting CIA had significantly lower androstenedione levels after 1-year follow-up than those resuming menses (1.20 ng/mL (IC95% [1.07–1.33] median 1.09 ng/mL) vs 1.48 ng/mL (IC95% [1.33–1.62] median 1.46 ng/mL) (p = 0.0092).
Five spontaneous pregnancies were recorded during the 24 months of follow-up of the study. However, these data should be interpreted with care, as no data were collected on the desire for pregnancy, marital status and/or frequency of intercourses in this population. No significant differences were found for androstenedione and testosterone levels between the status of pregnant women and other patients (data not shown).
Discussion
In this prospective study involving 250 women of reproductive age undergoing treatment for breast cancer, we present the dynamic changes in both total testosterone and androstenedione serum levels throughout the course of chemotherapy and a subsequent 2-year follow-up. Following the completion of chemotherapy, the serum levels of these two androgens experienced a rapid and significant decrease, subsequently rebounding in all patients during the follow-up period to concentrations near baseline levels.
Our findings indicate that chemotherapy-induced damage to the ovaries extends beyond the impact on granulosa cells, evident in the swift decline of serum AMH levels (Dezellus et al., Citation2017; Rosendahl et al., Citation2010), to also affect theca cells, as demonstrated by the rapid reduction in androgen levels. This underscores the concept of a comprehensive alteration of ovarian function in this context (Spears et al., Citation2019). Although this study did not delve into the potential extra-ovarian consequences of the observed decrease in androgen serum levels during chemotherapy—such as effects on psychological state, muscle tone, sexual function, and overall quality of life—this area warrants further exploration.
The specific mechanisms of theca cell alteration during chemotherapy remain unknown. However, the relatively high division rate of these cells, in conjunction with a well-developed capillary network in this vascularized compartment during folliculogenesis, suggest that these cells might be highly sensitive to the gonadotoxic effects of chemotherapy.
The existing literature on androgen production in young women of reproductive age undergoing cancer treatment is notably limited. We could only find a poster communication reporting the evolution of serum free testosterone and dehydroepiandrosterone sulfate (DHEAS) levels throughout chemotherapy (Cameron et al., Citation2016). This study reported a decrease in testosterone level but not in DHEAS was found. DHEAS is the most abundant androgen, it is exclusively produced by adrenal glands (Davison & Davis, Citation2003) and it has previously been shown to be unaffected by chemotherapy (Dnistrian et al., Citation1985), suggesting that chemotherapy affects ovarian but not adrenal production of androgens (Dnistrian et al., Citation1985; Dowsett & Richner, Citation1991; Rose & Davis, Citation1980). In contrast, our focus was specifically on ovarian production of androgens and not adrenal, therefore using androstenedione instead of DHEAS. Although intriguing, the limitations of the aforementioned poster study, including the lack of information on the type of malignancy, the small number of patients, and the relatively short follow-up period warrant cautious interpretation.
Our study also indirectly sheds light on the respective adrenal and ovarian contributions to androgen synthesis. Indeed, and quite surprisingly, little biological evidence is available in women regarding the respective androgen synthesis in these 2 organs, which are traditionally considered to be almost equivalent (Abraham, Citation1974; Davison & Davis, Citation2003). Although a potential chemotherapy-induced alteration of adrenal function cannot be ruled out, our results suggest that ovaries account for approximately two thirds of androstenedione synthesis. Interpreting serum levels of total testosterone levels is a little but more speculative, as Body Mass Index and body fat percentage can impact both peripheral conversion by adipocytes and SHBG levels. However, our results suggest that testosterone synthesis follows approximately the same pattern than androstenedione during and after chemotherapy. It should be noted that mean body mass index slightly increased in patients throughout the study, but the relevance of this evolution is likely to be negligible with regard to androgen peripheral conversion. Although the respective part of adrenal, ovarian and peripheral synthesis of testosterone cannot be precisely derived from our results, it can be speculated that not only ovarian compartment is significantly affected by chemotherapy, but also peripheral and/or adrenal compartments.
We believe that this study boasts several strengths. First, this homogeneous cohort comprises a substantial number of reproductive age patients, allowing robust statistical analysis. The 2-year follow-up provides insights into the long-term hormonal profile evolution. The use of automated assays for androgen measurements, coupled with centralized analysis, mitigates the risk of inter-center technical or analytical variation on results.
However, we acknowledge potential limitations. First, the use of an automated testosterone assay instead of the reference method based on isotope dilution mass spectrometry raises the issue of analytical sensitivity and imprecision at low concentrations. Second, a 2-year follow-up might be insufficient to observe the final evolution of androgen serum levels. Third, these results are relevant for younger women exposed to ovarian damage induced by chemotherapy, but may not be extrapolated to older postmenopausal women. Fourth, premenopausal women have high hormonal fluctuations during the menstrual cycle, calling for caution when interpreting these data. Lastly, a longitudinal follow-up of body fat mass in addition to weight could have provided a deeper understanding of the evolution of testosterone levels.
The findings of our study might carry significant implications for both clinical practice and future research. In clinical practice, understanding the dynamics of serum androgen levels during and after chemotherapy can inform healthcare professionals of potential side effects related to hormonal fluctuations and aid in the development of strategies to mitigate these effects, thereby enhancing the overall quality of life for young women undergoing breast cancer treatment. Additionally, the study’s results may prompt further research into the intricate relationship between chemotherapy and ovarian function and contribute to the advancement of fertility preservation. Future investigations could explore the long-term impact of these hormonal changes on patients’ health outcomes.
Conclusions
In conclusion, this study reports the evolution of 2 serum androgens during chemotherapy and 2-year follow-up in a large sample of young women treated for breast cancer. It also brings new insight into the physiology of androgen synthesis, suggesting that ovarian theca cells are responsible for the synthesis of approximately 2 thirds of androstenedione and total testosterone in women of reproductive age.
Supplemental Material
Download MS Word (81.5 KB)Supplemental Material
Download TIFF Image (15.4 KB)Acknowledgments
The authors of this work are extremely grateful to the members of the RESOVA investigators’ group: M Campone (Saint Herblain, France), C Lemanski (Montpellier, France), L Vanlemmens (Lille, France), L Mignot (Paris, France), T Delozier & C Levy (Caen, France), C Bendavid (Rennes, France), M Debled (Bordeaux, France), T Bachelot (Lyon, France), C Jouannaud (Reims, France), C Loustalot (Dijon, France), MA Mouret-Reynier (Clermont-Ferrand, France).
Disclosure statement
The authors report there are no competing interests to declare.
Data availability statement
Data can be made available upon reasonable request, after evaluation by the corresponding author.
Additional information
Funding
References
- Abraham, G. E. (1974). Ovarian and adrenal contribution to peripheral androgens during the menstrual cycle. The Journal of Clinical Endocrinology and Metabolism, 39(2), 340–346. https://doi.org/10.1210/jcem-39-2-340
- Anderson, R. A., Brewster, D. H., Wood, R., Nowell, S., Fischbacher, C., Kelsey, T. W., & Wallace, W. H. B. (2018). The impact of cancer on subsequent chance of pregnancy: a population-based analysis. Human Reproduction (Oxford, England), 33(7), 1281–1290. https://doi.org/10.1093/humrep/dey216
- Blumenfeld, Z. (2012). Chemotherapy and fertility. Best Practice & Research. Clinical Obstetrics & Gynaecology, 26(3), 379–390. https://doi.org/10.1016/j.bpobgyn.2011.11.008
- Burger, H. G. (2002). Androgen production in women. Fertility and Sterility, 77 (Suppl 4), S3–S5. https://doi.org/10.1016/s0015-0282(02)02985-0
- Cameron, K., Sammel, M. D., Prewitt, M. M., Lynch, M. E., & Gracia, C. (2016). Impact of chemotherapy on androgen and follicular measures of ovarian reserve in female cancer survivors. Fertility and Sterility, 106(3), e128. https://doi.org/10.1016/j.fertnstert.2016.07.383
- Chung, M., Chang, H. R., Bland, K. I., & Wanebo, H. J. (1996). Younger women with breast carcinoma have a poorer prognosis than older women. Cancer, 77(1), 97–103. https://doi.org/10.1002/(SICI)1097-0142(19960101)77:1<97::AID-CNCR16>3.0.CO;2-3
- Cronin, K. A., Lake, A. J., Scott, S., Sherman, R. L., Noone, A.-M., Howlader, N., Henley, S. J., Anderson, R. N., Firth, A. U., Ma, J., Kohler, B. A., & Jemal, A. (2018). Annual Report to the Nation on the Status of Cancer, part I: National cancer statistics. Cancer, 124(13), 2785–2800. https://doi.org/10.1002/cncr.31551
- Davison, S. L., & Davis, S. R. (2003). Androgens in women. The Journal of Steroid Biochemistry and Molecular Biology, 85(2-5), 363–366. https://doi.org/10.1016/s0960-0760(03)00204-8
- Davison, S. L., Bell, R., Donath, S., Montalto, J. G., & Davis, S. R. (2005). Androgen Levels in Adult Females: Changes with Age, Menopause, and Oophorectomy. The Journal of Clinical Endocrinology and Metabolism, 90(7), 3847–3853. https://doi.org/10.1210/jc.2005-0212
- Dewailly, D., Andersen, C. Y., Balen, A., Broekmans, F., Dilaver, N., Fanchin, R., Griesinger, G., Kelsey, T. W., La Marca, A., Lambalk, C., Mason, H., Nelson, S. M., Visser, J. A., Wallace, W. H., & Anderson, R. A. (2014). The physiology and clinical utility of anti-Mullerian hormone in women. Human Reproduction Update, 20(3), 370–385. https://doi.org/10.1093/humupd/dmt062
- Dezellus, A., Barriere, P., Campone, M., Lemanski, C., Vanlemmens, L., Mignot, L., Delozier, T., Levy, C., Bendavid, C., Debled, M., Bachelot, T., Jouannaud, C., Loustalot, C., Mouret-Reynier, M. A., Gallais-Umbert, A., Masson, D., & Freour, T. (2017). Prospective evaluation of serum anti-Müllerian hormone dynamics in 250 women of reproductive age treated with chemotherapy for breast cancer. European Journal of Cancer (Oxford, England: 1990), 79, 72–80. https://doi.org/10.1016/j.ejca.2017.03.035
- Dnistrian, A. M., Greenberg, E. J., Dillon, H. J., Hakes, T. B., Fracchia, A. A., & Schwartz, M. K. (1985). Chemohormonal therapy and endocrine function in breast cancer patients. Cancer, 56(1), 63–70. https://doi.org/10.1002/1097-0142(19850701)56:1<63::aid-cncr2820560111>3.0.co;2-7
- Dowsett, M., & Richner, J. (1991). Effects of cytotoxic chemotherapy on ovarian and adrenal steroidogenesis in pre-menopausal breast cancer patients. Oncology, 48(3), 215–220. https://doi.org/10.1159/000226930
- Drakopoulos, P., Bardhi, E., Scherer, S., Blockeel, C., Verheyen, G., Anckaert, E., Tournaye, H., & Polyzos, N. P. (2021). Androgens and Anti-Müllerian Hormone in Infertile Patients. Reproductive Sciences (Thousand Oaks, Calif.), 28(10), 2816–2821. https://doi.org/10.1007/s43032-021-00539-w
- Early Breast Cancer Trialists’ Collaborative Group (EBCTCG). (2005). Effects of chemotherapy and hormonal therapy for early breast cancer on recurrence and 15-year survival: an overview of the randomised trials. Lancet (London, England), 365(9472), 1687–1717. https://doi.org/10.1016/S0140-6736(05)66544-0
- Eifel, P., Axelson, J. A., Costa, J., Crowley, J., Curran, W. J., Deshler, A., Fulton, S., Hendricks, C. B., Kemeny, M., Kornblith, A. B., Louis, T. A., Markman, M., Mayer, R., & Roter, D. (2001). National institutes of health consensus development conference statement: Adjuvant therapy for breast cancer, November 1-3, 2000. Journal of the National Cancer Institute, 93(13), 979–989. https://doi.org/10.1093/jnci/93.13.979
- Fidler, M. M., Gupta, S., Soerjomataram, I., Ferlay, J., Steliarova-Foucher, E., & Bray, F. (2017). Cancer incidence and mortality among young adults aged 20-39 years worldwide in 2012: a population-based study. The Lancet. Oncology, 18(12), 1579–1589. https://doi.org/10.1016/S1470-2045(17)30677-0
- Ghoncheh, M., Pournamdar, Z., & Salehiniya, H. (2016). Incidence and mortality and epidemiology of breast cancer in the world. Asian Pacific Journal of Cancer Prevention: APJCP, 17(S3), 43–46. https://doi.org/10.7314/apjcp.2016.17.s3.43
- Goldhirsch, A., Glick, J. H., Gelber, R. D., & Senn, H. J. (1998). Meeting highlights: International consensus panel on the treatment of primary breast cancer. Journal of the National Cancer Institute, 90(21), 1601–1608. https://doi.org/10.1093/jnci/90.21.1601
- Jamil, Z., Fatima, S. S., Ahmed, K., & Malik, R. (2016). Anti-mullerian hormone: Above and beyond conventional ovarian reserve markers. Disease Markers, 2016, 5246217–5246219. https://doi.org/10.1155/2016/5246217
- Kim, H.-A., Choi, J., Park, C. S., Seong, M.-K., Hong, S.-E., Kim, J.-S., Park, I.-C., Lee, J. K., & Noh, W. C. (2018). Post-chemotherapy serum anti-Müllerian hormone level predicts ovarian function recovery. Endocrine Connections, 7(8), 949–956. https://doi.org/10.1530/EC-18-0180
- Lambertini, M., Goldrat, O., Toss, A., Azim, H. A., Peccatori, F. A., Ignatiadis, M., Del Mastro, L., & Demeestere, I. (2017). Fertility and pregnancy issues in BRCA-mutated breast cancer patients. Cancer Treatment Reviews, 59, 61–70. https://doi.org/10.1016/j.ctrv.2017.07.001
- Lambertini, M., Olympios, N., Lequesne, J., Calbrix, C., Fontanilles, M., Loeb, A., Leheurteur, M., Demeestere, I., Di Fiore, F., Perdrix, A., & Clatot, F. (2019). Impact of taxanes, endocrine therapy, and deleterious germline BRCA mutations on anti-müllerian hormone levels in early breast cancer patients treated with anthracycline- and cyclophosphamide-based chemotherapy. Frontiers in Oncology, 9, 575. https://doi.org/10.3389/fonc.2019.00575
- Lebbe, M., & Woodruff, T. K. (2013). Involvement of androgens in ovarian health and disease. Molecular Human Reproduction, 19(12), 828–837. https://doi.org/10.1093/molehr/gat065
- Lucis, O. J., Hobkirk, R., Hollenberg, C. H., MacDonald, S. A., & Blahey, P. (1966). Polycystic ovaries associated with congenital adrenal hyperplasia. Canadian Medical Association Journal, 94(1), 1–7.
- Mastro, L. D., Catzeddu, T., & Venturini, M. (2006). Infertility and pregnancy after breast cancer: Current knowledge and future perspectives. Cancer Treatment Reviews, 32(6), 417–422. https://doi.org/10.1016/j.ctrv.2006.05.004
- Morgan, S., Anderson, R. A., Gourley, C., Wallace, W. H., & Spears, N. (2012). How do chemotherapeutic agents damage the ovary? Human Reproduction Update, 18(5), 525–535. https://doi.org/10.1093/humupd/dms022
- Pache, T. D., & Fauser, B. C. (1993). Polycystic ovaries in female-to-male transsexuals. Clinical Endocrinology, 39(6), 702–703.
- Partridge, A. H., & Ruddy, K. J. (2007). Fertility and adjuvant treatment in young women with breast cancer. Breast (Edinburgh, Scotland), 16 Suppl 2, S175–S181. https://doi.org/10.1016/j.breast.2007.07.029
- Rose, D. P., & Davis, T. E. (1980). Effects of adjuvant chemohormonal therapy on the ovarian and adrenal function of breast cancer patients. Cancer Research, 40(11), 4043–4047.
- Rosendahl, M., Andersen, C. Y., la Cour Freiesleben, N., Juul, A., Løssl, K., & Andersen, A. N. (2010). Dynamics and mechanisms of chemotherapy-induced ovarian follicular depletion in women of fertile age. Fertility and Sterility, 94(1), 156–166. https://doi.org/10.1016/j.fertnstert.2009.02.043
- Sklar, C. A., Mertens, A. C., Mitby, P., Whitton, J., Stovall, M., Kasper, C., Mulder, J., Green, D., Nicholson, H. S., Yasui, Y., & Robison, L. L. (2006). Premature menopause in survivors of childhood cancer: a report from the childhood cancer survivor study. Journal of the National Cancer Institute, 98(13), 890–896. https://doi.org/10.1093/jnci/djj243
- Spears, N., Lopes, F., Stefansdottir, A., Rossi, V., De Felici, M., Anderson, R. A., & Klinger, F. G. (2019). Ovarian damage from chemotherapy and current approaches to its protection. Human Reproduction Update, 25(6), 673–693. https://doi.org/10.1093/humupd/dmz027
- Walters, K. A. (2015). Role of androgens in normal and pathological ovarian function. Reproduction, 149(4), R193–R218. https://doi.org/10.1530/REP-14-0517
- Walters, K. A., Rodriguez Paris, V., Aflatounian, A., & Handelsman, D. J. (2019). Androgens and ovarian function: translation from basic discovery research to clinical impact. The Journal of Endocrinology, 242(2), R23–R50. https://doi.org/10.1530/JOE-19-0096