ABSTRACT
Introduction
Obesity is recognized as a major healthcare challenge. Following years of slow progress in discovery of safe, effective therapies for weight management, recent approval of the glucagon-like peptide 1 receptor (GLP-1R) mimetics, liraglutide and semaglutide, for obesity has generated considerable excitement. It is anticipated these agents will pave the way for application of tirzepatide, a highly effective glucose-dependent insulinotropic polypeptide receptor (GIPR), GLP-1R co-agonist, recently approved for management of type 2 diabetes mellitus.
Areas covered
Following promising weight loss in obese individuals in Phase III clinical trials, liraglutide and semaglutide were approved for weight management without diabetes. Tirzepatide has attained Fast Track designation for obesity management by the US Food and Drug Association. This narrative review summarizes experimental, preclinical, and clinical data for these agents and related GLP-1R/GIPR co-agonists, prioritizing clinical research published within the last 10 years where possible.
Expert Opinion
GLP-1R mimetics are often discontinued within 24 months meaning long-term application of these agents in obesity is questioned. Combined GIPR/GLP-1R agonism appears to induce fewer side effects, indicating GLP-1R/GIPR co-agonists may be more suitable for enduring obesity management. After years of debate, this GIPR-biased GLP-1R/GIPR co-agonist highlights the therapeutic promise of including GIPR modulation for diabetes and obesity therapy.
1. Introduction
According to their most recently published global figures in 2016, the World Health Organization (WHO) estimates that there are over 1.9 billion adults currently overweight, while 650 million are considered clinically obese [Citation1]. Given the uncurbed prevalence of excess body weight gain, it is likely that these figures will have been exceeded at the time of writing. Indeed, according to more recent estimates from the World Obesity Atlas 2022, it is predicted that over 1 billion people will be clinically obese by 2030 [Citation2], equating to 1 in 5 women and 1 in 7 men globally. More recent regional WHO data for Europe indicate a rise in overweight and obesity prevalence in children and adolescents during the COVID-19 pandemic, which have been compounded by decreases in physical activity and increases in the consumption of foods high in fat, sugar, and salt [Citation3]. Importantly, the report indicates that obesity will surpass smoking as the major risk factor for preventable cancer in many European countries in the coming decades [Citation3].
1.1. Pharmacological management of obesity
While recognized as a major risk factor for stroke and cardiovascular disease [Citation4], type 2 diabetes mellitus (T2DM) [Citation5] and now increasingly implicated as an environmental driver of cognitive decline in the development Alzheimer’s Disease [Citation6,Citation7], the American Medical Association and National Institutes of Health officially recognize obesity as a complex, chronic disease in its own right [Citation8,Citation9]. As such, pharmacological intervention for obesity is becoming increasingly accepted.
However, it is important to note that lifestyle intervention, namely, increasing physical activity and reducing calorie intake, remains the first-line option for managing patients with obesity [Citation10]. While bariatric surgery can also be employed in more extreme cases [Citation11], it is usually reserved for patients with a BMI of 40 kg/m2 or more or patients with a BMI of 35 kg/m2 or above and a comorbidity such as type 2 diabetes or high blood pressure [Citation12]. Thus, pharmacological intervention is a more universally applicable option if lifestyle intervention fails. Unfortunately, prescribing options have remained remarkably limited for obesity, owing to a chequered past of many small molecule agonists previously employed for the disease. Agents such as sibutramine, lorcaserin, and rimonabant have been withdrawn in the vast majority or regions, due to safety concerns such as increased cardiovascular risk and adverse behavioral effects [Citation13–15]. Until relatively recently, the lipase inhibitor orlistat has been the only universal mainstay in obesity management [Citation16]. However, the well-documented unpleasant gastrointestinal (GIT) side-effects of orlistat limit patient uptake and compliance. Thus, there has been real optimism following the recent demonstrated effectiveness and approval of glucagon-like peptide-1 (GLP-1) mimetics, liraglutide, and semaglutide [Citation17,Citation18], for management of obesity, with hope that the genuine success of these peptide agents in type 2 diabetes (T2DM) can be translated to obesity.
2. Glucagon-like peptide-1 mimetics
GLP-1 is a gut-derived, 29 amino acid residue hormone, released post-prandially from intestinal L-cells, particularly following meals rich in fat and carbohydrate [Citation19,Citation20]. GLP-1 secretion is biphasic, with an early phase occurring 10–15 min after meal ingestion and a second, more prolonged, phase occurring 30–60 min post meal [Citation21]. The primary biologically active form of the peptide is GLP-1(7-36)-amide, generated by tissue-specific post-translational processing of the proglucagon gene by prohormone convertase enzymes [Citation22]. Upon binding to the GLP-1 receptor (GLP-1 R) on pancreatic beta-cells, GLP-1 promotes glucose-dependent insulin secretion via stimulation of intracellular cAMP-mediated events and also encourages glucose-induced biosynthesis of insulin, resulting in replenishment of insulin stores within beta cells [Citation21, Citation23; ]. Furthermore, there is evidence to suggest that GLP-1 R activation enhances pancreatic beta-cell growth and survival, at least in rodents [Citation24, Citation25; ]. In addition, GLP-1 is also known to suppress glucagon secretion, with some debate as to whether this is related to a direct effect on alpha cells or mediated indirectly through increased somatostatin secretion from islet delta-cells [Citation26]. As a result of these combined positive effects on glucose modulating islet-derived hormones, sustained activation of GLP-1 R has seen successful clinical application in the management of T2DM.
Figure 1. An overview of the biological actions of endogenous glucagon-like peptide-1 (GLP-1) and glucose-dependent insulinotropic peptide (GIP) in man. Tissue-specific effects of GLP-1 and GIP are provided for relevant organs in green or blue, respectively.
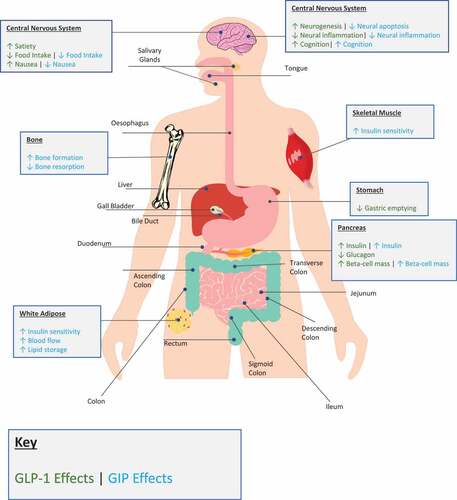
2.1. GLP-1 mimetics in type 2 diabetes
Endogenous GLP-1 is subject to extremely rapid N-terminal degradation by the ubiquitous serine protease dipeptidyl peptidase-4 (DPP-4) [Citation27], which cleaves an N-terminal dipeptide to generate GLP-1(9–36), a weak GLP-R antagonist. Ultimately, this means that the insulinotropic effects of the native peptide are rapidly lost in the circulation, rendering it unsuitable for therapeutic utilization.
In order to combat this rapid enzymatic inactivation, various long-acting GLP-1 analogs have been developed for the management of T2DM. The first such analog was exendin-4, originally isolated from the saliva of the venous Gila monster (Heloderma suspectum) lizard [Citation28]. Exendin-4 is a potent agonist for mammalian GLP-1 Rs, effectively bringing about GLP-1 R-mediated benefits on glycemia [Citation28,Citation29], and importantly also possesses inherent resistance to DPP-4 inactivation [Citation30]. Synthetic exendin-4, exenatide, attained clinical approval for T2DM in 2005 (Byetta), and has since become a widely prescribed second- and third-line agent for this disease. Subsequent medicinal chemistry and drug formulation innovation have seen the successful development of liraglutide, which is a stable once-daily GLP-1 mimetic based on the structure of human GLP-1, and even longer-acting GLP-1 analogs that allow for once weekly delivery. These second-generation drugs include exenatide extended-release as well as semaglutide and dulaglutide analogs that are based on the sequence of human GLP-1 [Citation31–33]. While all these GLP-1 mimetics are injectable agents, it is noteworthy that an oral version of semaglutide (Rybelsus) formulated with sodium N-(8-[2-hydroxybenzoyl] amino) caprylate to protect against stomach degradation is now available as a once-daily tablet [Citation34], but requires extremely high doses of semaglutide to provoke effective glucose-lowering actions.
2.2. GLP-1 mimetics in obesity
In addition to the positive effects of GLP-1 on insulin secretion and beta-cell survival [Citation21, Citation23; ], the hormone has long been understood to play an important role in delaying gastric transit and promoting satiety []. These actions are linked to activation of GLP-1 Rs at central and peripheral enteric neurons to slow gastric emptying and intestinal transit, as well as suppress appetite at the level of the hypothalamus, together influencing a mechanism termed the ‘ileal brake’ [Citation35; ]. It is clear that a complex gut-brain relationship is at play given the presence of releasable stores of GLP-1 and its target receptors in both peripheral and central locations [Citation35,Citation36; ]. Indeed, satiety is influenced by activation of GLP-1 R present in both the central nervous system, while the gut is further modulated by vagal-derived cholinergic signals [Citation36]. It has been suggested that centrally, modulation of the anorexigenic proopiomelanocortin (POMC) neurons is responsible for satiating effects, given they possess GLP-1 Rs and are present in the nucleus tractus solitarius (NTS), the site of GLP-1 production in the brain [Citation36]. This complex interplay likely explains why an intact vagus nerve is required for the satiating effects of exogenous GLP-1 when delivered by intraperitoneal injection, but not when delivered intravenously [Citation35,Citation36]. Promotion of satiety has been demonstrated to impart significant weight loss in T2DM patients receiving GLP-1 analogs [Citation36], hence enthusiasm in repurposing of GLP-1 analogs as anti-obesity agents, in the absence of T2DM.
Such exploitation was realized in 2020 following the approval of liraglutide (marketed as Saxenda for obesity) as the first GLP-1 mimetic to gain regulatory consent for management of obesity, without concurrent diabetes [Citation17]. Related phase III clinical trials (Satiety and Clinical Adiposity – Liraglutide Evidence in non‐diabetic and diabetic individuals; SCALE) demonstrated a sustained 2-year weight loss of 5–10% in non-diabetic participants receiving liraglutide, when employed in addition to patient education on increased physical activity and some restriction of food intake [Citation37; ]. The increased dose of liraglutide for obesity as compared to diabetes, 1. 8 mg vs. 3.0 mg, respectively, has been a topic of some concern [Citation17,Citation38]. As such, the SCALE trials indicated that when liraglutide was employed at a dose of 3.0 mg it was subject to largely the same GIT side-effects as the 1.8 mg dose used in T2DM but the incidence of severe adverse effects was increased [Citation17]. Moreover, the trial confirmed that any positive effects in terms of decrease in body weight are lost upon drug discontinuation, necessitating long-term therapy. In this respect, in T2DM as many as 70% of patients in the US receiving GLP-1 therapies discontinue within 24 months, largely due to GIT related side effects [Citation39]. In good agreement, a related study indicated that over 60% of patients cite feelings of nausea or GIT discomfort as the primary reason for discontinuation of GLP-1 mimetics [Citation40]. In that regard, it will be interesting to track how this adverse effect profile affects translation of longer-term GLP-1 benefits on obesity in the real-world setting, especially since both liraglutide and semaglutide are used at higher doses in obesity than for T2DM.
Table 1. A summary of GLP-1 R mimetics and GLP-1/GIPR co-agonists studied in obesity management.
Following the successful approval of liraglutide for obesity, Phase III clinical trials (Semaglutide Treatment Effect in People with obesity; STEP) demonstrated that once weekly semaglutide induced an average weight loss of 14.9% following 68 weeks treatment in obese and overweight adults, with an acceptable adverse effect profile linked to anticipated GIT actions [Citation18; ]. Previous studies had already indicated that semaglutide elicited superior weight loss than liraglutide [Citation41]. Injectable semaglutide gained FDA approval for obesity in 2021 (marketed as Wegovy), with subsequent approval by the MHRA and EMA following in 2022. As with liraglutide, the dose for obesity of 2.4 mg is higher than the 2.0 mg employed in T2DM [Citation42]. However, an 18-week titration window with injectable semaglutide may help partially alleviate discontinuation issues, by potentially reducing troublesome GIT adverse effects. Additionally, oral semaglutide has now been approved for T2DM and demonstrates promising body weight reductions and tolerability in the PIONEER 8 trials in T2DM patients [Citation43]. Phase III clinical trials in obese individuals are set to be conducted in 2023, investigating the effects of 50 mg of oral semaglutide daily over a 68-week period [Citation44; ]. The significant dose increase from T2DM to obesity is notable but relates to the poor oral bioavailability of oral semaglutide, reported to be in the region of 0.8% [Citation45].
3. GIP mimetics
Glucose-dependent insulinotropic polypeptide (GIP) is a 42-amino acid peptide hormone secreted from intestinal K-cells of the duodenum and proximal jejunum [Citation46]. Like GLP-1 [Citation19, Citation20; ], GIP is released in response to macronutrient load in the gut, working together with GLP-1 to induce the ‘incretin-effect.’ As such, GIP enhances glucose-stimulated insulin secretion following binding to GIP receptors (GIP-R) on the beta-cell [Citation47; ], augmenting adenyl cyclase signal transduction pathways [Citation48], similar in many respects to GLP-1 [Citation49]. Further overlap exists with GLP-1, as GIP is also subject to rapid degradation by DPP-4, generating the inactive GIP(3–42) metabolite [Citation50]. Indeed, this DPP-4 degradation fragment may even function as a weak GIPR antagonist, especially at high concentrations [Citation51; Citation52]. Additionally, GIP and its receptor have been evidenced within the brain, with GIPR expression in the hypothalamus being implicated in the modulation of feeding, particularly within arcuate, paraventricular, and dorsomedial nuclei, hypothalamic regions associated with energy balance [Citation53, Citation54; ]; however, the exact mechanisms at play within these regions are yet to be confirmed. Moreover, the peripheral actions of GIP in improving insulin sensitivity in adipocytes and modulate overall lipid metabolism positively influence overall energy balance and can benefit weight loss [Citation54]. Thus, given the complementary actions of these hormones, the development of a dual-acting GIPR/GLP-1 R agonist is entirely logical.
Figure 2. A peptidic structure analysis of glucose-dependent insulinotropic peptide (GIP) (1–42), glucagon-like peptide-1 (GLP-1) (7–36) and tirzepatide, a dual GIP/GLP-1 receptor co-agonist. Amino acid residues are provided as their single-letter abbreviations. Residues shared with GIP(1–42) are shaded in blue, shared with GLP-1(7–36) are shaded in green, residues shared with both GLP-1 and GIP are indicated in Orange and those unique to tirzepatide are shaded in gray. The fatty acid modification of tirzepatide is indicated in yellow. Receptor affinities are also indicated based on values reported in the literature [Citation101] .
![Figure 2. A peptidic structure analysis of glucose-dependent insulinotropic peptide (GIP) (1–42), glucagon-like peptide-1 (GLP-1) (7–36) and tirzepatide, a dual GIP/GLP-1 receptor co-agonist. Amino acid residues are provided as their single-letter abbreviations. Residues shared with GIP(1–42) are shaded in blue, shared with GLP-1(7–36) are shaded in green, residues shared with both GLP-1 and GIP are indicated in Orange and those unique to tirzepatide are shaded in gray. The fatty acid modification of tirzepatide is indicated in yellow. Receptor affinities are also indicated based on values reported in the literature [Citation101] .](/cms/asset/f5cad5fd-81c7-47c5-ad98-ca318254bcdc/ieop_a_2192865_f0002_oc.jpg)
In that respect, the antidiabetic efficacy of several first-generation GIP compounds with N-terminal modifications at positions Tyr1 or Ala2 were documented some 20 years previously [Citation55]. These GIP analogs were highly effective in the preclinical setting using animal models of obesity and diabetes [Citation56–58]. Furthermore, second-generation acylated versions of these GIP analogs with extended biological half-lives also exhibited impressive antidiabetic profiles in animal models of diabetes [Citation59–62]. Moreover, advocation of GIPR agonist therapies in T2DM is supported by early studies that confirm GIP as the major incretin hormone [Citation63,Citation64]. However, concerns over a postulated lack of bioactivity of GIP in human T2DM-hindered clinical progression [Citation65].
4. Tirzepatide, a GLP-1 R/GIPR co-agonist
Considerable excitement has surrounded Eli Lilly & Co’s next-generation peptide therapeutic for T2DM, namely tirzepatide (Mounjaro). Not unlike some of the above-mentioned therapies, tirzepatide is a synthetic, linear peptide containing 39 amino acids, also incorporating a C20 diacid fatty acid that directly contributes to a half-life of approximately 5 days [Citation66; ], allowing for weekly dosing. However, tirzepatide sets itself apart from earlier peptide therapies through a dual-acting biological profile, functioning as an agonist at both GLP-1 and GIP receptors to elicit its biological actions [Citation66]. Indeed, the structure of tirzepatide has strong parallels with GIP, together with sequence modifications to also encourage GLP-1 R binding and activation [], thus building on early preclinical work with GIP therapeutics [Citation67].
In that regard, tirzepatide attained US Food and Drug Association (FDA) approval for the management of T2DM in May 2022 following impressive Phase III (SURPASS-3) clinical trial data, where the peptide-induced superior HbA1c reductions than dulaglutide (2.4–2.8% compared to 1.3%) following 52 weeks treatment [Citation68; ]. Interestingly, HbA1c reductions were noted to be largely independent of age [Citation69]. However, in this regard, it is important to note that while over 1200 participants in the SURPASS program achieved HbA1c levels of below 5.7%, these individuals tended to be both younger and have had a shorter duration of disease [Citation70]. Doses of 5, 10, and 15 mg were employed in the SURPASS trials and the current recommended dose is 5 mg daily which can be titrated to a maximum dose of 15 mg in 2.5 mg increments [Citation71].
Beyond glycemic benefits, tirzepatide has been demonstrated to induce profound reductions in body weight. Phase II clinical trials in T2DM patients indicated highly impressive body weight reductions of 5–10% alongside substantial reductions in waist-circumference following 12 weeks administration [Citation72]. Tirzepatide-induced reductions of body weight were over three times greater than with dulaglutide, being attributed to complementary benefits of GIPR/GLP-1 R modulation on inhibition of appetite and gastric emptying, as well as the ability of GIPR to curb GLP-1-induced emesis, leading to improved overall effectiveness and tolerability [Citation66]. It is important to note that appetite reduction was measured as a self-reported score, hence further study will be required to elucidate synergistic mechanisms between GLP-1/GIP centrally and in the periphery in regulating appetite. Such findings are supported by data arising from Phase III clinical trials (SURPASS-3) that demonstrated a 7.6–11.2% weight loss in participants receiving tirzepatide, in comparison with 5.7% induced by semaglutide alone [Citation72,Citation73]. Hence, tirzepatide is claimed to be a potential game changer in terms of pharmacological interventions for obesity. Indeed, on 6 October 2022, the FDA granted Fast Track designation for tirzepatide in obesity management, with the results of a current Phase III clinical trial [SURMOUNT-MMO; 75], anticipated to be completed in April 2023, being the primary factor relating to how expedited this process will actually be.
Given the strong similarity of tirzepatide with the amino acid sequence of GIP [], it is not unsurprising that in vitro mechanistic studies reveal strong bias toward the GIPR, activating this receptor with equipotency to native GIP whilst having 5-fold weaker affinity than native GLP-1 at GLP-1 R [Citation74; ]. This poses a question as to the importance of each receptor interaction in driving the pro-glycemic and weight-loss effects of tirzepatide. When investigated in murine pancreatic islets lacking GLP-1 R’s, tirzepatide-stimulated insulin secretion was abolished when co-cultured with a GIPR antagonist [Citation66]. Additionally, in islets from GIPR knock-out (KO) mice, tirzepatide-stimulated insulin secretion is completely blocked by GLP-1 R antagonism [Citation66], indicating the importance of both receptors for beneficial effects of the peptide in relation to insulin secretion. Although species-specific activity of GIP amino acid sequences [Citation75], that is not observed with GLP-1, may make it difficult to interpret and translate exact receptor selectivity importance of tirzepatide from the rodent setting to humans. In addition, additive or even synergistic benefits of combined GLP-1 R/GIPR signaling would not be fully quantifiable when using receptor KO models of individual receptor pathways, since signaling pathway interactions are unable to be examined. A similar paradigm has been noted when attempting to understand the additive benefits of GLP-1 alongside other related gut-derived hormones, such as cholecystokinin or gastrin [Citation76,Citation77]. This could be particularly important when it comes to the side-effect profile of tirzepatide and long-term tolerability in patients. For example, it has been suggested that anti-emetic effects GIPR agonism combined with GLP-1 R agonism improves tolerability of tirzepatide when compared to GLP-1 mimetic monotherapy [Citation78], especially given the higher doses employed for GLP-1 mimetic monotherapy in obesity management. That said, the incidence of GIT side-effects were only modestly reduced in tirzepatide receiving subjects than in those receiving semaglutide [Citation73]. Although in this study, semaglutide was employed at a lower dose than is now recommended for obesity, meaning tirzepatide may have a competitive edge in this respect.
5. Further GLP-1 R/GIPR co-agonists in development
While representing the first major success in terms of the clinical application of GLP-1/GIP-R co-agonism, it is important to note that tirzepatide builds on a considerable body of previous work exploring this combination. Indeed, the first chimeric GLP-1/GIP chimeric peptides were first described over 25 years ago [Citation79], with other related dual-acting peptides only being characterized several years after this [Citation80–82].
In this regard, preclinical studies in genetically obese-diabetic (ob/ob) mice indicated that combination therapy with a stable, N-terminally acylated GIP analog, N-AcGIP, with the GLP-1 R agonist exendin-4, elicited superior improvements in glucose tolerance and insulin sensitivity than exendin-4 alone [Citation83]. Notably, no body weight reduction was observed over the sub-chronic, 14-day study with combined N-AcGIP and exendin-4 therapy [Citation84]. However, the relatively short treatment period could be a factor in terms of lack of effect of the GIPR and GLP-1 R agonist combination therapy on adiposity in the ob/ob mice. Indeed, the effectiveness of this approach was further endorsed through later studies utilizing administration of an acylated GLP-1 and GIP preparations in the same mouse model [Citation85]. The initial promise of GIPR and GLP-1 R combination therapies led to the generation of a unimolecular molecule, termed N-Ac(d-Ala2)GIP/GLP-1-exe, combining a N-AcGIP molecule with exendin-4 [Citation82], which brought about significant body weight reduction in addition to improved glucose handling and insulin sensitivity in diet-induced obese (DIO) mice. Although, the receptor balance profile of N-Ac(d-Ala2)GIP/GLP-1-exe has not yet been fully explored, making it difficult to determine the relative impact of GIPR and GLP-1 R signaling in these observed metabolic improvements [Citation83,Citation84].
Intriguingly, a balanced GLP-1/GIPR unimolecular co-agonist has been described in the literature, with development appearing to run largely in tandem with that of tirzepatide. Denominated in the literature as RG7697, or NNC0090-2746, this molecule was designed on a glucagon-based core, with residues from native GIP, GLP-1 and also exendin-4 incorporated into the sequence, to produce a balanced GIPR/GLP-1 R binding profile [Citation80; ], although modest affinity for the glucagon receptor (GCGR) was retained. The molecule progressed as far as Phase II clinical trials [Citation85; ], with comparison against liraglutide (1.8 mg) noting similar reductions in HbA1c following 12 weeks administration in subjects with T2DM, although body weight reductions were significantly improved over liraglutide [Citation81]. While both molecules were employed at 1.8 mg, it is important to note that this is considerably lower than 3.0 mg currently utilized for liraglutide in obesity management [Citation17]. It is unclear why RG7697 has not progressed further, but higher rates of discontinuation with RG7697 as compared to the liraglutide may be a factor [Citation81], as well as requirement for once daily injection [Citation66,Citation86,Citation87]. In addition, the combined impact of GIPR, GLP-1 R and GCGR activation on the cardiovascular system, particularly in relation to elevated heart rate [Citation73], could also be a factor that requires consideration. Moreover, it appears that Novo Nordisk is currently attempting to emulate their success with oral semaglutide, through the development of an oral GLP-1/GIPR agonist, reported to be undergoing Phase I trials [Citation88,Citation89], but information on this molecule is sparse at the time of writing.
Beyond obesity and diabetes, it is worth documenting that GLP-1/GIPR co-agonism is also being explored in neurodegenerative diseases []. This is noteworthy given that obesity is becoming increasingly recognized as a risk factor for neurodegenerative disorders [Citation6,Citation7]. A unimolecular GLP-1/GIPR co-agonist, DA5-CH, which crosses the blood-brain barrier (BBB) [Citation90], was shown to improve cognition, memory retention and motor function in a superior manner to exendin-4, liraglutide, or semaglutide in rodent models of Alzheimer’s and Parkinson’s disease [Citation91,Citation92]. While no metabolic data was published for DA5-CH within these studies, it would be interesting to assess potential benefits in relation to glycemia and weight loss, particularly given the increasing role assigned to obesity in the pathophysiology of neurodegenerative disorders [Citation6,Citation7,Citation90]. In support of this, work with DA5-CH actually builds on findings relating to the neurological benefits of the aforementioned N-Ac(d-Ala2)GIP/GLP-1-exe dual acting peptide, that was demonstrated to augment recognition memory, hippocampal neurogenesis, synapse formation, and reduce neuronal oxidative stress in DIO mice, alongside benefits on metabolism [Citation82]. In good harmony, earlier work had already confirmed cognitive benefits of up-regulation of either GIPR [Citation93; ] or GLP-1 R [Citation94; ] signaling in obese mice with impaired memory and cognition.
Finally, there are established benefits of GIP on bone formation in both animal models and humans with T2DM [Citation95, Citation96; ], with a suggestion that GLP-1 may also positively impact bone composition [Citation97]. Indeed, similar to the picture with neurodegenerative disorders, obesity is also noted to have a negative impact on the osteocyte network and overall bone quality [Citation98]. Thus, dual GIPR/GLP-1 R activation may also lead to improvements in bone homeostasis as documented with DPP-4 inhibitors [Citation99], which is relevant since T2DM is linked with increased bone fragility [Citation100].
6. Conclusion
The approval of semaglutide and liraglutide for weight management has been met with considerable excitement [Citation17,Citation18]. Conversely, given high discontinuation rates observed in T2DM [Citation39], application of these medications for long-term management of obesity remains unclear. Importantly however, their successful clinical application has likely paved the way for use of the first GLP-1 R/GIP-R co-agonist, tirzepatide, in obesity [Citation66,Citation101], following recent approval of this molecule in T2DM. Moreover, the success of this GIPR-biased molecule has finally highlighted the potential of GIPR-modulation for the treatment of obesity and T2DM [Citation66], after decades of debate. Once considered the poorer relative of GLP-1, these new and exciting observations with GIPR modulation confirm the longstanding belief that GIP possess translatable benefits for human disease [Citation67].
7. Expert opinion
Amid many years of stagnation in the approval of new, safe, and effective therapies for obesity, the considerable excitement surrounding the recent approval of GLP-1 R mimetics liraglutide and semaglutide in 2021[] for the management of the condition is understandable. Indeed, such has been the popularity of these therapies for weight loss that global shortages of semaglutide have been witnessed in 2022, likely compounded by prior off-label use of the GLP-1 R mimetic before regulatory approval of semaglutide in 2021 [Citation42,Citation102]. In addition, the increased dose requirement for obesity, as well as for oral application in T2DM, has likely compounded semaglutide peptide supply issues. Furthermore, it is important to note that application of GLP-1 therapies for obesity is in relative infancy, with considerable intrigue surrounding the longevity of these agents, given the already high drug discontinuation rates in T2DM coupled with elevated doses employed for weight management [Citation18,Citation37,Citation39]. In this respect, a reduced GIT side-effect profile with tirzepatide in T2DM is encouraging [Citation73], suggesting prolonged treatment within the confines of obesity may be more achievable with tirzepatide than GLP-1 monotherapy counterparts.
Ethnic differences may play an important role in the universal application of GLP-1 mimetics for obesity. As such, in relation to T2DM management, concern over the use of GLP-1 R mimetics has been noted in Asian populations who appear to be at increased risk of sarcopenia [Citation103], while the same could also be true for the elderly. Thus, GLP-1-induced weight-loss has thus far been primarily reported as a crude percentage of body weight or BMI reduction, rather than specific fat mass loss [Citation18,Citation36]. In addition, the WHO BMI classifications have been defined as inappropriate for Asian populations [Citation104]. Thus, the relative ‘quality’ of GLP-1 mimetic-induced weight loss, i.e. fat mass over lean or muscle mass reductions, may be pertinent in this regard. More positively, a recent study in rodents indicated semaglutide specifically reduced the accumulation of intramuscular fat, promoted muscle protein synthesis, increased skeletal muscle proportion and improved muscle function in DIO mice [Citation105], although translation to the human setting is still required.
As highlighted above, the recent approval of GLP-1 R mimetics for management of obesity will likely pave the way for application of tirzepatide in weight management. Assumed uptake of tirzepatide in the diabetes and obesity clinic may also lead to realization of more complex unimolecular agonists, that modulate pathways beyond that of only GLP-1 R and GIPR. In that respect, multiagonism more closely emulates the hormonal changes demonstrated following Roux-en-Y gastric bypass (RYGB) surgery, following which numerous gut hormones are upregulated and are becoming more recognized as major dictators of the T2DM remission and profound weight loss seen post-surgery [Citation106]. Indeed, the therapeutic potential of other unimolecular peptidic agents has been reviewed recently [Citation107]. Nonetheless, it appears that after many years of debate, tirzepatide has endorsed the therapeutic promise of GIPR modulation for diabetes. In addition, it is well recognized that obesity is as a major risk factor for stroke and cardiovascular disease as well as certain kinds of malignancy, thus GLP-1 mimetics or related dual agonists such as tirzepatide will likely improve outcomes in this respect. In keeping with this, GLP-1 R mimetics are known to improve cardiovascular risk in T2DM, largely independent of their antihyperglycemic actions [Citation108].
Interestingly, the discovery of GIP was made over a decade prior to that of GLP-1 [Citation109,Citation110], hence it poses the question as to why its therapeutic application has taken this long to be realized? The answer is probably two-fold and relates to a documented inefficacy of GIP in patients with T2DM [Citation65], although this phenomenon appears to be reversible when glycemia is returned to more normal values [Citation111]. Secondly, a continuing debate remains as to whether GIPR agonism or antagonism is of most benefit in T2DM and obesity? In this regard, GIP was once referred to as an ‘obesity hormone,’ due to direct actions on GIPR present on adipocytes that promote lipid accumulation [Citation112]. Additionally, GIPR KO mice were reported to be resistant to the adipogenic effects of a high fat diet [Citation113], but this is also true for GLP-1 R KO mice [Citation114]. Conversely, the hormone has been demonstrated to also possess a lipolytic effect [Citation115], albeit in in vitro settings. Either way, it is clear that GIP exerts important direct actions on lipid metabolism that merit further investigation [Citation116,Citation117].
Taken together, modulation of GIP action presents something of a conundrum, in that antidiabetic and anti-obesity benefits of GIPR agonism and antagonism have been demonstrated. Indeed, obesity and hyperphagia are attenuated by central and/or peripheral injection of a GIPR-neutralizing antibodies and GIPR antagonist peptides [Citation118–121], while in preclinical studies, benefits of sustained GIPR blockade have been demonstrated alone and in combination GLP-1 R mimetics. Thus, the partial GIPR antagonist, (Pro3)GIP, has originally been demonstrated to improve obesity-related diabetes, reducing islet hypertrophy and improving insulin sensitivity [Citation122], while also eliciting 8% weight loss following administration alone in obese mice [Citation123]. These observations have been largely confirmed by others employing GIPR monoclonal antibodies [Citation124]. Interestingly, there is a suggestion that the metabolic benefits of chronic GIPR agonism are related to desensitization of the GIPR, thus mimicking GIPR antagonism [Citation125]. Although this GIPR desensitization theory still requires significant further investigation and confirmation.
In terms of a combination approach for GIPR antagonism and GLP-1 R agonism, related investigations demonstrate that co-administration of GIPR monoclonal antibody, with various GLP-1 R mimetic therapies, elicits superior weight-loss over monotherapy in obese mice and non-human primates [Citation120]. Similar, but less pronounced benefits of a peptide based GIPR antagonist in combination with liraglutide have subsequently been noted in DIO mice [Citation126]. More recently, the peptidic GIPR antagonist, [Nα-Ac, L14, R18, E21] hGIP(5-31)-K11(γE-C16) has been described as potentiating the weight loss benefits of semaglutide alone, when administered over 28 days in DIO mice [Citation127]. Furthermore, preclinical studies suggest an optimized scheduling to annul GIPR signaling and promote GLP-1 R signaling at specific times during the day may be important for therapeutic benefits, interchanging between GIPR blockade during periods of inactivity and GLP-1 R agonism during the active hours [Citation128]. However, a more recent study has characterized a bi-specific antibody that combines GIPR antagonism and GLP-1 R agonism within the same compound and revealed beneficial weight reducing actions in mice and monkeys [Citation129], arguing against a scheduled daily dosing pattern.
Article highlights
Approval of GLP-1R mimetics liraglutide and semaglutide for weight management paves the way for peptide therapies in obesity.
FDA approval of tirzepatide for type 2 diabetes management has been met with considerable excitement owing to significant glycaemic and weight loss benefits.
It is anticipated tirzepatide will ultimately find clinical application in obesity management. Debate remains as to whether GIPR agonism or antagonism is of most metabolic benefit, but the pendulum is swinging towards agonism.
The therapeutic potential of GIPR modulation may now be realised in management of metabolic disease.
This box summarizes key points contained in the article.
Declaration of interest
All authors are shareholders in Dia Beta Labs Ltd. a spinout company from Ulster University developing peptide-based therapies for management of Type 2 diabetes mellitus. P R Flatt and N Irwin are also named on patents filed by Ulster University for the exploitation of incretin-based drugs and other peptide therapeutics.
Reviewer disclosures
Peer reviewers on this manuscript have no relevant financial or other relationships to disclose.
Additional information
Funding
References
- World Health Organization. Obesity and overweight. Geneva: WHO; 2017.
- World Obesity Federation. World obesity atlas. 2022
- World Health Organization. European regional obesity report 2022. Copenhagen: WHO; 2022
- Hubert HB, Feinleib M, McNamara PM, et al. Obesity as an independent risk factor for cardiovascular disease: a 26-year follow-up of participants in the Framingham heart study. Circulation. 1983;67:968–977.
- Leitner DR, Frühbeck G, Yumuk V, et al. Obesity and type 2 diabetes: two diseases with a need for combined treatment strategies - EASO can lead the way. Obes Facts. 2017;10:483–492.
- Pegueroles J, Jiménez A, Vilaplana E, et al. Obesity and Alzheimer’s disease, does the obesity paradox really exist? A magnetic resonance imaging study. Oncotarget. 2018;9:34691–34698.
- Naderali EK, Ratcliffe SH, Dale MC. Obesity and Alzheimer’s disease: a link between body weight and cognitive function in old age. Am J Alzheimers Dis Other Demen. 2010;24:445–449
- Kyle TK, Dhurandhar EJ, Allison DB. Regarding obesity as a disease: evolving policies and their implications. Endocrinol Metab Clin North Am. 2016;45:511–520
- National Heart, Lung, and Blood Institute (NIH publication 98-4083). The evidence report, clinical guidelines on the identification, evaluation, treatment of overweight and obesity in adults. 1998. Available from: http://www.nhlbi.nih.gov/guidelines/obesity/ob_gdlns.pdf. [cited 2022 Nov 8]
- Baillot A, Romain AJ, Boisvert-Vigneault K, et al. Effects of lifestyle interventions that include a physical activity component in class II and III obese individuals: a systematic review and meta-analysis. PLoS One. 2015;10:e0119017.
- Elder KA, Wolfe BM. Bariatric surgery: a review of procedures and outcomes. Gastroenterology. 2007;132:2253–2271.
- Ahmad A, Laverty AA, Aasheim E, et al. Eligibility for bariatric surgery among adults in England: analysis of a national cross-sectional survey. JRSM Open. 2014;5:2042533313512479.
- Czernichow S, Batty GD. Withdrawal of sibutramine for weight loss: where does this leave clinicians? Obes. Facts. 2010;3(3):155–156.
- Smith SR, Weissman NJ, Anderson CM, et al. Behavioural modification and lorcaserin for overweight and obesity management (BLOOM) study group. Multicentre, placebo-controlled trial of lorcaserin for weight management. N Engl J Med. 2010;363:245–256.
- Blasio A, Iemolo A, Sabino V, et al. Rimonabant precipitates anxiety in rats withdrawn from palatable food: role of the central amygdala. Neuropsychopharmacology. 2013;38:2498–2507.
- Guerciolini R. Mode of action of orlistat. Int J Obes Relat Metab Disord. 1997;21:12–23.
- Kelly AS, Auerbach P, Barrientos-Perez M, et al. A randomized, controlled trial of liraglutide for adolescents with obesity. N Eng J Med. 2020;382:2117–2128.
- Wilding JPH, Batterham RL, Calanna S, et al. Once-weekly semaglutide in adults with overweight or obesity. N Engl J Med. 2021;384:989.
- Buhls T, Thimq L, Kofodll H, et al. Naturally occurring products of proglucagon 11 1-160 in the porcine and human small intestine. J Biol Chem. 1988;263:8621–8624.
- Reimann F, Gribble FM. Glucose-sensing in glucagon-like peptide-1-secreting cells. Diabetes. 2002;51:2757–2763.
- Baggio LL, Drucker DJ. Biology of incretins: GLP-1 and GIP. Gastroenterology. 2007;132:2131–2157.
- Knudsen LB, Pridal L. Glucagon-like peptide-1-(9-36) amide is a major metabolite of glucagon-like peptide-1-(7-36) amide after in vivo administration to dogs, and it acts as an antagonist on the pancreatic receptor. Eur J Pharmacol. 1996;318:429–435.
- Doyle ME, Egan JM. Mechanisms of action of glucagon-like peptide 1 in the pancreas. Pharmacol Ther. 2007;113:546–593.
- Moffett RC, Patterson S, Irwin N, et al. Positive effects of GLP-1 receptor activation with liraglutide on pancreatic islet morphology and metabolic control in C57BL/KsJ db/db mice with degenerative diabetes. Diabetes Metab Res Rev. 2015;31:248–255
- Vasu S, Moffett RC, Thorens B, et al. Role of endogenous GLP-1 and GIP in beta cell compensatory responses to insulin resistance and cellular stress. PLoS One. 2014;9:e101005.
- De Marinis YZ, Salehi A, Ward CE, et al. GLP-1 inhibits and Adrenaline stimulates glucagon release by differential modulation of N- and L-type Ca2+ channel-dependent exocytosis. Cell Metab. 2010;11:543–553.
- Deacon CF, Johnsen AH, Holst JJ. Degradation of glucagon-like peptide-1 by human plasma in vitro yields an N-terminally truncated peptide that is a major endogenous metabolite in vivo. J Clin Endocrinol Metab. 1995;80(3):952–957.
- Eng J, Kleinman WA, Singh L, et al. Isolation and characterization of exendin-4, an exendin-3 analogue, from Heloderma suspectum venom. Further evidence for an exendin receptor on dispersed acini from Guinea pig pancreas. J Biol Chem. 1992;267:7402–7405.
- Edwards CMB, Stanley SA, Davis R, et al. Exendin-4 reduces fasting and postprandial glucose and decreases energy intake in healthy volunteers. Am J Physiol – Endocrinol Metab. 2001;281:155–161
- Kolterman OG, Kim DD, Shen L, et al. Pharmacokinetics, pharmacodynamics, and safety of exenatide in patients with type 2 diabetes mellitus. Am J Heal Pharm. 2005;62:173–181.
- Vilsbøll T, Zdravkovic M, Le-Thi T, et al. Liraglutide, a long-acting human glucagon-like peptide-1 analog, given as monotherapy significantly improves glycemic control and lowers body weight without risk of hypoglycemia in patients with type 2 diabetes. Diabetes Care. 2007;30:1608–1610.
- Lingvay I, Catarig AM, Frias JP, et al. Efficacy and safety of once-weekly semaglutide versus daily canagliflozin as add-on to metformin in patients with type 2 diabetes (SUSTAIN 8): a double-blind, phase 3b, randomised controlled trial. Lancet Diabetes Endocrinol. 2019;7:834–844.
- Jendle J, Grunberger G, Blevins T, et al. Efficacy and safety of dulaglutide in the treatment of type 2 diabetes: a comprehensive review of the dulaglutide clinical data focusing on the AWARD phase 3 clinical trial program. Diabetes Metab Res Rev. 2016;32:776–790
- Pieber TR, Bode B, Mertens A, et al. Efficacy and safety of oral semaglutide with flexible dose adjustment versus sitagliptin in type 2 diabetes (PIONEER 7): a multicentre, open-label, randomised, phase 3a trial. Lancet Diabetes Endocrinol. 2019;7:528–539.
- Zander M, Madsbad S, Madsen JL, et al. Effect of 6-week course of glucagon-like peptide 1 on glycaemic control, insulin sensitivity, and beta-cell function in type 2 diabetes: a parallel-group study. Lancet. 2002;359:824–830.
- Shah M, Vella A. Effects of GLP-1 on appetite and weight. Rev Endocr Metab Disord. 2014;15(3):181–187.
- Davies MJ, Bergenstal R, Bode B, et al. Efficacy of liraglutide for weight loss among patients with type 2 diabetes: the SCALE diabetes randomized clinical trial. J Am Med Assoc. 2015;314:687–699.
- Blonde L, Russell-Jones D. The safety and efficacy of liraglutide with or without oral antidiabetic drug therapy in type 2 diabetes: an overview of the LEAD 1-5 studies. Diabetes Obes Metab. 2009;11(3)26–34.
- Weiss T, Carr RD, Pal S, et al. Real-world adherence and discontinuation of glucagon-like peptide-1 receptor agonists therapy in type 2 diabetes mellitus patients in the United States. Patient Prefer Adherence. 2020;27(14):2337–2345.
- Sikirica MV, Martin AA, Wood R, et al. Reasons for discontinuation of GLP1 receptor agonists: data from a real-world cross-sectional survey of physicians and their patients with type 2 diabetes. Diabetes Metab Syndr Obes. 2017;29(10):403–412.
- Nauck MA, Petrie JR, Sesti G, et al. A phase 2, randomized, dose-finding study of the novel once-weekly human GLP-1 analog, semaglutide, compared with placebo and open-label liraglutide in patients with type 2 diabetes. Diabetes Care. 2016;39:231–241.
- Frías JP, Auerbach P, Bajaj, et al. Efficacy and safety of once-weekly semaglutide 2·0 mg versus 1·0 mg in patients with type 2 diabetes (SUSTAIN FORTE): a double-blind, randomised, phase 3B trial. Lancet Diabetes. Endocrinol 2021;9(9):563–574.
- Zinman B, Aroda VR, Buse JB, et al. Efficacy, safety, and tolerability of oral semaglutide versus placebo added to insulin with or without metformin in patients with type 2 diabetes: the PIONEER 8 trial. Diabetes Care. 2019;42:2262–2271.
- ClinicalTrials.gov Identifier: NCT05132088. Available from: https://clinicaltrials.gov/ct2/show/NCT05132088?term=semaglutide&cond=Obesity&draw=2&rank=7. [cited 2022 Nov 15].
- Overgaard RV, Navarria A, Ingwersen SH, et al. Clinical pharmacokinetics of oral semaglutide: analyses of data from clinical pharmacology trials. Clin Pharmacokinet. 2021;60(10):1335–1348.
- Buchan AMJ, Polak JM, Capella C, et al. Electronimmunocytochemical evidence for the K cell localization of gastric inhibitory polypeptide (GIP) in man. Histochemistry. 1978;56:37–44.
- Pederson RA, Schubert HE, Brown JC. Gastric inhibitory polypeptide. Its physiologic release and insulinotropic action in the dog. Diabetes 1975;24:1050–1056.
- Green BD, Gault VA, Flatt PR, et al. Comparative effects of GLP-1 and GIP on cAMP production, insulin secretion, and in vivo antidiabetic actions following substitution of Ala8/Ala2 with 2-aminobutyric acid. Arch Biochem Biophys. 2004;428(2):136–143.
- Mommsen TP, Mojsov S. Glucagon-like peptide-1 activates the adenylyl cyclase system in rockfish enterocytes and brain membranes. Comp Biochem Physiol B Biochem Mol Biol. 1998;121(1)49–56.
- Mentlein R, Gallwitz B, Schmidt WE. Dipeptidyl‐peptidase IV hydrolyses gastric inhibitory polypeptide, glucagon‐like peptide‐ 1(7–36)amide,peptide histidine methionine and is responsible for their degradation in human serum. Eur J Biochem. 1993;214:829–835.
- Deacon CF, Plamboeck A, Rosenkilde MM, et al. GIP-(3-42) does not antagonize insulinotropic effects of GIP at physiological concentrations. Am J Physiol Endocrinol Metab. 2006;291(3):E468–75.
- Gault VA, Parker JC, Harriott P, et al. Evidence that the major degradation product of glucose-dependent insulinotropic polypeptide, GIP(3-42), is a GIP receptor antagonist in vivo. J Endocrinol. 2002;175(2):525–533.
- Seino Y, Fukushima M, Yabe D. GIP and GLP-1, the two incretin hormones: similarities and differences. J Diabetes Investig. 2010;1:8–23.
- Samms RJ, Coghlan MP, Sloop KW. How may gip enhance the therapeutic efficacy of GLP-1? Trends Endocrinol. Metab. 2020;31(6):410–421.
- Gault VA, Flatt PR, O’Harte FP. Glucose-dependent insulinotropic polypeptide analogues and their therapeutic potential for the treatment of obesity-diabetes. Biochem Biophys Res Commun. 2003;308(2):207–213.
- Hinke SA, Gelling RW, Pederson RA, et al. Dipeptidyl peptidase IV-resistant [D-Ala(2)]glucose-dependent insulinotropic polypeptide (GIP) improves glucose tolerance in normal and obese diabetic rats. Diabetes. 2002;51(3):652–661.
- Gault VA, O’Harte FP, Harriott P, et al. Degradation, cyclic adenosine monophosphate production, insulin secretion, and glycemic effects of two novel N-terminal Ala2-substituted analogs of glucose-dependent insulinotropic polypeptide with preserved biological activity in vivo. Metabolism. 2003;52(6):679–687.
- Gault VA, Flatt PR, Harriott P, et al. Improved biological activity of Gly2- and Ser2-substituted analogues of glucose-dependent insulinotrophic polypeptide. J Endocrinol. 2003;176(1):133–141.
- Irwin N, Green BD, Gault VA, et al. Degradation, insulin secretion, and antihyperglycemic actions of two palmitate-derivitized N-terminal pyroglutamyl analogues of glucose-dependent insulinotropic polypeptide. J Med Chem. 2005;48(4):12440–12450.
- Irwin N, Green BD, Mooney MH, et al. A novel, long-acting agonist of glucose-dependent insulinotropic polypeptide suitable for once-daily administration in type 2 diabetes. J Pharmacol Exp Ther. 2005;314(3):1187–1194.
- Irwin N, O’Harte FP, Gault VA, et al. GIP(Lys16PAL) and GIP(Lys37PAL): novel long-acting acylated analogues of glucose-dependent insulinotropic polypeptide with improved antidiabetic potential. J Med Chem. 2006;49(3):1047–1054.
- Irwin N, Gault VA, Green BD, et al. Antidiabetic potential of two novel fatty acid derivatised, N-terminally modified analogues of glucose-dependent insulinotropic polypeptide (GIP): n-AcGIP(LysPAL16) and N-AcGIP(LysPAL37). Biol Chem. 2005;386(7):679–687.
- Gault VA, Fpm O, Harriott P, et al. Effects of the novel (Pro3)GIP antagonist and exendin(9-39)amide on GIP- and GLP-1-induced cyclic AMP generation, insulin secretion and postprandial insulin release in obese diabetic (ob/ob) mice: evidence that GIP is the major physiological incretin. Diabetologia. 2003;46(2):222–230.
- Parker JC, Irwin N, Lavery KS, et al. Metabolic effects of sub-chronic ablation of the incretin receptors by daily administration of (Pro3)GIP and exendin(9-39)amide in obese diabetic (ob/ob) mice. Biol Chem. 2007;388(2):221–226.
- Nauck MA, Heimesaat MM, Orskov C, et al. Preserved incretin activity of glucagon-like peptide 1 [7-36 amide] but not of synthetic human gastric inhibitory polypeptide in patients with type-2 diabetes mellitus. J Clin Invest. 1993;91(1):301–307.
- Coskun T, Sloop KW, Loghin C, et al. LY3298176, a novel dual GIP and GLP-1 receptor agonist for the treatment of type 2 diabetes mellitus: from discovery to clinical proof of concept. Mol Metab. 2018;18:3–14.
- Irwin N, Gault V, Flatt PR. Therapeutic potential of the original incretin hormone glucose-dependent insulinotropic polypeptide: diabetes, obesity, osteoporosis and Alzheimer’s disease? Expert Opin. Investig Drugs. 2010;19(9):1039–1048.
- Inagaki N, Takeuchi M, Oura T, et al. Efficacy and safety of tirzepatide monotherapy compared with dulaglutide in Japanese patients with type 2 diabetes (SURPASS J-mono): a double-blind, multicentre, randomised, phase 3 trial. Lancet Diabetes Endocrinol. 2022;10(9):623–633.
- Whysham CH, Tofe S, Sapin H, et al. Effect of once-weekly TZP on glycemic control by baseline age in patient subpopulations from the SURPASS trials. Diabetes. 2022;71(1):743.
- Rosenstock J, Del Prato S, Franco DR, et al. Characterization of tirzepatide-treated patients achieving HbA1c < 5.7 % in the SURPASS 1–4 trials. Diabetes. 2022;71(1):90.
- Syed YY. Tirzepatide: first Approval. Drugs. 2022;82(11):1213–1220.
- Frias JP, Nauck MA, Van J, et al. Efficacy and tolerability of tirzepatide, a dual glucose-dependent insulinotropic peptide and glucagon-like peptide-1 receptor agonist in patients with type 2 diabetes: a 12-week, randomized, double-blind, placebo-controlled study to evaluate different dose-escalation regimens. Diabetes Obes Metab. 2020;22:938–946.
- Frías JP, Davies MJ, Rosenstock J, et al. Tirzepatide versus semaglutide once weekly in patients with type 2 diabetes. N Engl J Med. 2021;385:503–515.
- Willard FS, Douros JD, Gabe MBN, et al. Tirzepatide is an imbalanced and biased dual GIP and GLP-1 receptor agonist. J. C. I. Insight. 2020;5:e140532.
- Perry RA, Craig SL, Ng MT, et al. Characterisation of glucose-dependent insulinotropic polypeptide receptor antagonists in rodent pancreatic beta cells and mice. Clin Med Insights Endocrinol Diabetes. 2019;12:1179551419875453
- Tanday N, English A, Lafferty RA, et al. Benefits of sustained upregulated unimolecular GLP-1 and CCK receptor signalling in obesity-diabetes. Front Endocrinol. 2021;14:674704.
- Tanday N, Flatt PR, Irwin N. Amplifying the antidiabetic actions of glucagon-like peptide-1: potential benefits of new adjunct therapies. Diabet Med. 2021;38(12):e14699.
- Borner T, Geisler CE, Fortin SM, et al. GIP receptor agonism attenuates GLP-1 receptor agonist-induced nausea and emesis in preclinical models. Diabetes. 2021;70(11):2545–2553.
- Gallwitz B, Witt M, Morys-Wortmann C, et al. GLP-1/GIP chimeric peptides define the structural requirements for specific ligand-receptor interaction of GLP-1. Regul Pept. 1996;63(1):17–22.
- Finan B, Ma T, Ottaway N, et al. Unimolecular dual incretins maximize metabolic benefits in rodents, monkeys, and humans. Sci Transl Med. 2013;209(5):209ra151.
- Frias JP, Bastyr EJ, Vignati L, et al. The sustained effects of a dual GIP/GLP-1 receptor agonist, NNC0090-2746, in patients with type 2 diabetes. Cell Metab. 2017;26(2):343–352.
- Pathak NM, Pathak V, Gault VA, et al. Novel dual incretin agonist peptide with antidiabetic and neuroprotective potential. Biochem Pharmacol. 2018;155:264–274.
- Irwin N, McClean PL, Cassidy RS, et al. Comparison of the anti-diabetic effects of GIP- and GLP-1-receptor activation in obese diabetic (ob/ob) mice: studies with DPP IV resistant N-AcGIP and exendin(1-39)amide. Diabetes Metab Res Rev. 2007;23(7):572–579.
- Gault VA, Kerr BD, Harriott P, et al. Administration of an acylated GLP-1 and GIP preparation provides added beneficial glucose-lowering and insulinotropic actions over single incretins in mice with Type 2 diabetes and obesity. Clin Sci. 2011;121(3):107–117.
- ClinicalTrials.gov Identifier: NCT02205528. Available from: https://clinicaltrials.gov/ct2/show/NCT02205528?term=NNC0090-2746&draw=2&rank=1. [cited 2021 Nov 19].
- Portron A, Jadidi S, Sarkar N, et al. Pharmacodynamics, pharmacokinetics, safety and tolerability of the novel dual glucose-dependent insulinotropic polypeptide/glucagon-like peptide-1 agonist RG7697 after single subcutaneous administration in healthy subjects. Diabetes Obes Metab. 2017;19(10):1446–1453.
- Schmitt C, Portron A, Jadidi S, et al. Pharmacodynamics, pharmacokinetics and safety of multiple ascending doses of the novel dual glucose-dependent insulinotropic polypeptide/glucagon-like peptide-1 agonist RG7697 in people with type 2 diabetes mellitus. Diabetes Obes Metab. 2017;19(10):1436–1445.
- ClinicalTrials.gov Identifier: NCT05521256. Available from: https://clinicaltrials.gov/ct2/show/NCT05521256?term=Novo+Nordisk&recrs=ab&phase=04&draw=3&rank=3. [cited 2021 Nov 19].
- ClinicalTrials.gov Identifier: NCT05363774. Available from: https://clinicaltrials.gov/ct2/show/NCT05363774?term=Novo+Nordisk&recrs=ab&phase=04&draw=2&rank=5. [cited 2021 Nov 19].
- Zhang LY, Chun L, Zhang Z, et al. DA5-CH and Semaglutide protect against neurodegeneration and reduce α-Synuclein levels in the 6-OHDA Parkinson’s disease rat model. Parkinson’s Dis. 2022;2022:1428817.
- Li C, Liu W, Li X, et al. The novel GLP-1/GIP analogue DA5-CH reduces tau phosphorylation and normalizes theta rhythm in the icv. STZ rat model of AD. Brain Behav. 2020;10(3):e01505.
- Zhang LY, Jin QQ, Hölscher C, et al. Glucagon-like peptide-1/glucose-dependent insulinotropic polypeptide dual receptor agonist DA-CH5 is superior to exendin-4 in protecting neurons in the 6-hydroxydopamine rat Parkinson model. Neural Regen Res. 2021;6(8):1660–1670.
- Porter DW, Irwin N, Flatt PR, et al. Prolonged GIP receptor activation improves cognitive function, hippocampal synaptic plasticity and glucose homeostasis in high-fat fed mice. Eur J Pharmacol. 2011;650(3):688–693.
- Lennox R, Porter DW, Flatt PR, et al. Comparison of the independent and combined effects of sub-chronic therapy with metformin and a stable GLP-1 receptor agonist on cognitive function, hippocampal synaptic plasticity and metabolic control in high-fat fed mice. Neuropharmacology. 2014;86:22–30.
- Vyavahare SS, Mieczkowska A, Flatt PR, et al. GIP analogues augment bone strength by modulating bone composition in diet-induced obesity in mice. Peptides. 2020;125:170207.
- Stensen S, Gasbjerg LS, Krogh LL, et al. Effects of endogenous GIP in patients with type 2 diabetes. Eur J Endocrinol. 2021;185(1):33–45.
- Mansur SA, Mieczkowska A, Flatt PR, et al. The GLP-1 receptor agonist exenatide ameliorates bone composition and tissue material properties in high fat fed diabetic mice. Front Endocrinol. 2019;12(10):51.
- Mabilleau G, Perrot R, Flatt PR, et al. High fat-fed diabetic mice present with profound alterations of the osteocyte network. Bone. 2016;90:99–106.
- Mansur SA, Mieczkowska A, Flatt PR, et al. Sitagliptin alters bone composition in high-fat-fed mice. Calcif Tissue Int. 2019;104(4)437–448.
- Mabilleau G, Chappard D, Flatt PR, et al. Effects of anti-diabetic drugs on bone metabolism. Exp Rev Endocrinol Metab. 2015;10(6):663–675.
- ClinicalTrials.gov Identifier: NCT05556512. Available from: https://clinicaltrials.gov/ct2/show/NCT05556512?term=tirzepatide&draw=2&rank=9. [cited 2021 Nov 16].
- Tchang BG, Aras M, Kumar RB, et al. Pharmacologic treatment of overweight and obesity in adults. In: Feingold KR, Anawalt B, Boyce A, et al., (editors) Endotext [Internet]. South Dartmouth (MA): MDText.com, Inc; 2000. Available from: https://www.ncbi.nlm.nih.gov/books/NBK279038/.
- Usui R, Yabe D, Seino Y. Twincretin as a potential therapeutic for the management of type 2 diabetes with obesity. J Diabetes Investig. 2019;10(4):902–905.
- Lim JU, Lee JH, Kim JS, et al. Comparison of world health organization and Asia-Pacific body mass index classifications in COPD patients. Int J Chron Obstruct Pulmon Dis. 2017;12:2465–2475
- Ren Q, Chen S, Chen X, et al. An effective glucagon-like peptide-1 receptor agonists, semaglutide, improves sarcopenic obesity in obese mice by modulating skeletal muscle metabolism. Drug Des Devel Ther. 2022;16:3723–3735
- Holst JJ. Enteroendocrine secretion of gut hormones in diabetes, obesity and after bariatric surgery. Curr Opin Pharmacol. 2013;13:983–988.
- Lafferty RA, Flatt PR, Irwin N. Is polypharmacy the future for pharmacological management of obesity? Curr. Opin Endo Metab Res. 2022;23:100322
- Bethel MA, Patel RA, Merrill P, et al. Cardiovascular outcomes with glucagon-like peptide-1 receptor agonists in patients with type 2 diabetes: a meta-analysis. Lancet Diabetes Endocrinol. 2018;6(2):105–113.
- Brown JC, Pederson RA, Jorpes E, et al. Preparation of highly active enterogastrone. Can J Physiol Pharmacol. 1969;47:113–114.
- Brown JC, Mutt V, Pederson RA. Further purification of a polypeptide demonstrating enterogastrone activity. J Physiol. 1970;209:57–64.
- Bailey CJ. GIP analogues and the treatment of obesity-diabetes. Peptides. 2020;125:170202.
- Marks V. GIP: the obesity hormone. In: James WPT, Parker SW, editors. Current Approaches: obesity. Southampton (England): Duphar Medical Relations; 1988. p. 13–19.
- Miyawaki K, Yamada Y, Ban N, et al. Inhibition of gastric inhibitory polypeptide signaling prevents obesity. Nat Med. 2002;8(7):738–742.
- Ayala JE, Bracy DP, James FD, et al. Glucagon-like peptide-1 receptor knockout mice are protected from high-fat diet-induced insulin resistance. Endocrinology. 2010;151(10):4678–4687.
- Getty-Kaushik L, Song DH, Boylan MO, et al. Glucose-dependent insulinotropic polypeptide modulates adipocyte lipolysis and reesterification. Obesity. 2006;14(7):1124–1131.
- Yip RG, Boylan MO, Kieffer TJ, et al. Functional GIP receptors are present on adipocytes. Endocrinology. 1998;139(9):4004–4007.
- English A, Craig SL, Flatt PR, et al. Individual and combined effects of GIP and xenin on differentiation, glucose uptake and lipolysis in 3T3-L1 adipocytes. Biol Chem. 2020;401(11):1293–1303.
- Boylan MO, Glazebrook PA, Tatalovic M, et al. Gastric inhibitory polypeptide immunoneutralization attenuates development of obesity in mice. Am J Physiol Endocrinol Metab. 2015;309(12):E1008–18.
- McClean PL, Gault VA, Irwin N, et al. Daily administration of the GIP-R antagonist (Pro3) GIP in streptozotocin-induced diabetes suggests that insulin-dependent mechanisms are critical to anti-obesity-diabetes actions of (Pro3) GIP. Diabetes Obes Metab. 2008;10(4):336–342.
- Killion EA, Wang J, Yie J, et al. Anti-obesity effects of GIPR antagonists alone and in combination with GLP-1R agonists in preclinical models. Sci Transl Med. 2018;10(472):eaat3392.
- Kaneko K, Fu Y, Lin HY, et al. Gut-derived GIP activates central Rap1 to impair neural leptin sensitivity during overnutrition. J Clin Invest. 2019;129(9):3786–3791.
- Gault VA, Irwin N, Green BD, et al. Chemical ablation of gastric inhibitory polypeptide receptor action by daily (Pro3)GIP administration improves glucose tolerance and ameliorates insulin resistance and abnormalities of islet structure in obesity-related diabetes. Diabetes. 2005;54:2436–2446.
- McClean PL, Irwin N, Cassidy RS, et al. GIP receptor antagonism reverses obesity, insulin resistance, and associated metabolic disturbances induced in mice by prolonged consumption of high-fat diet. Am J Physiol Endocrinol Metab. 2007;293(6):1746–1755.
- Chen J, Zheng S, Hu Y, et al. Chronic treatment with anti-GIPR mAb alone and combined with DPP-4 inhibitor correct obesity, dyslipidemia and nephropathy in rodent animals. Life Sci. 2021;269:119038.
- Killion EA, Chen M, Falsey JR, et al. Chronic glucose-dependent insulinotropic polypeptide receptor (GIPR) agonism desensitizes adipocyte GIPR activity mimicking functional GIPR antagonism. Nat Commun. 2020;11(1):4981.
- West JA, Tsakmaki A, Ghosh SS, et al. Chronic peptide-based GIP receptor inhibition exhibits modest glucose metabolic changes in mice when administered either alone or combined with GLP-1 agonism. PLoS One. 2021;16(3):e0249239.
- Yang B, Gelfanov VM, El K, et al. Discovery of a potent GIPR peptide antagonist that is effective in rodent and human systems. Mol Metab. 2022;2022:101638.
- Pathak V, Vasu S, Gault VA, et al. Sequential induction of beta cell rest and stimulation using stable GIP inhibitor and GLP-1 mimetic peptides improves metabolic control in C57BL/KsJ db/db mice. Diabetologia. 2015;58:2144–2153.
- Lu SC, Chen M, Atangan L, et al. GIPR antagonist antibodies conjugated to GLP-1 peptide are bispecific molecules that decrease weight in obese mice and monkeys. Cell Rep Med. 2021;2:100263