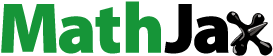
ABSTRACT
The breath figure method, which is used to form porous films from water droplet templates, has attracted considerable interest because it is simple and applicable to a wide variety of materials. Research on breath figures took off after the 2000s, accompanied by new polymer synthesis methods, fabrication methods, and a wide variety of applications. There are several comprehensive reviews of the applications of the porous films, which are usually called ‘honeycomb films’ because their hexagonally packed porous structure resembles honeycombs. However, new materials, progress in preparation technologies for controlling nano- and microstructures, and large-area fabrication are still areas that require further research. Furthermore, new applications of honeycomb films have emerged. In this review, the recent development of honeycomb films prepared by the breath figure techniques and their numerous applications are summarized. The production of honeycomb films can be performed on an industrial scale, greatly broadening their possible applications in areas such as optics, photonics, surface science, biotechnology, and regenerative medicine. Present problems and future perspectives are also discussed.
Graphical Abstract
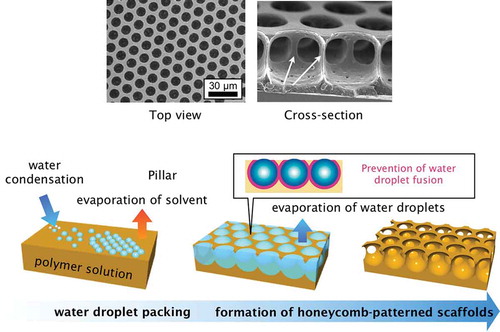
1. Introduction
The breath figure method, which is used to form porous films from water droplet templates, has attracted considerable interest because it is simple and applicable to a wide variety of materials. Breath figures are formed by water microdroplets condensed on a cool surface from warm, humid air like breath. The size of the condensed water droplets is usually on the micrometer scale, and they turn the surface of glass opaque through light scattering. Breath figures have been used for preparing porous films, and it is now a common bottom-up fabrication method. The standard breath figure procedure is as follows () [Citation1]. First, a polymer solution is cast onto a substrate, and then humid air is used to evaporate the solvent and condense water droplets on the solution surface. During the solvent evaporation, the water droplets pack closely owing to the lateral capillary force and form hexagonal lattices. After complete evaporation of solvent, porous polymer films containing hexagonally arranged pores are formed. François and co-workers first used this technique to prepare porous polymer films with rod-coil block copolymers [Citation2]. In the 1990s, groups independently reported early research on preparing porous films by using breath figures [Citation3,Citation4].
Figure 1. Schematic illustration of honeycomb film formation via the breath figure technique, and scanning electron microscopy (SEM) and cross-sectional SEM images of polystyrene honeycomb films.
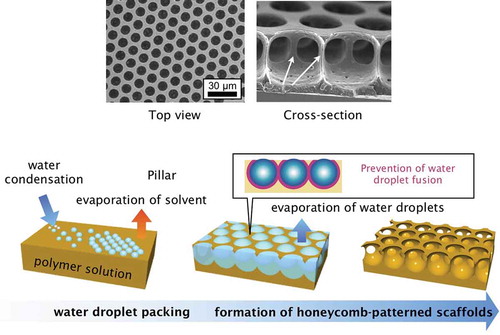
After the 2000s, research on breath figures took off, accompanied by new polymer synthesis methods, fabrication methods, and a wide variety of applications. There are several comprehensive reviews of the applications of the porous films [Citation5–Citation8], which are usually called ‘honeycomb films’ because their hexagonally packed porous structure resembles honeycombs. However, new materials, progress in preparation technologies for controlling nano- and microstructures, and large-area fabrication are still areas that require further research. Furthermore, new applications of honeycomb films have emerged.
In this review, we examine progress in fabrication techniques for honeycomb films by the breath figure method and their emerging applications. outlines the structure of this review. First, we introduce the materials for fabricating honeycomb films, and then we review recent developments in fabrication technologies. We discuss post-modifications for functionalizing and transforming the arrangement of honeycomb films. Next, I review the applications of honeycomb films and honeycomb film-based nanostructures. Finally, I present a summary and discuss future perspectives.
2. Development of materials for honeycomb film preparation
2.1. Polymers
Various kinds of polymers have been used to prepare honeycomb films (). The amphiphilicity and functionality of the polymers should be chosen based on the intended function. Amphiphilic polymers stabilize the template water droplets, and should satisfy the various conditions shown in Section 3.2. Amphiphilic polymers, which have both hydrophobic and hydrophilic moieties, are suitable for fabricating honeycomb films because interfacial tension is an important factor in controlling the uniformity and shapes of pores. Furthermore, functional polymers suitable for the applications of the honeycomb films should be chosen. Polymer architectures are also important for controlling the structures and properties of honeycomb films. Homopolymers, random copolymers, block copolymers, and other polymers with unique architectures have been reported.
Figure 3. Overview of polymers used for fabricating honeycomb films. CTA stands for cellulose triacetate, CAB for cellulose acetate butyrate, LC for liquid crystal, and ATRP for atom transfer radical polymerization.
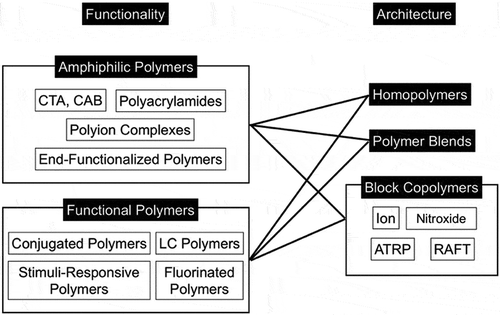
Many reported materials combine these polymer properties and architectures. For example, amphiphilic polymers have been used to fabricate uniform honeycomb films. Cellulose derivatives, including cellulose triacetate and cellulose acetate butylate [Citation9], are widely used polymeric materials, which are amphiphilic because of the hydrophilic polysaccharide main chains and hydrophobic butyrate groups and are soluble in hydrophobic organic solvents. Govor et al. used cellulose derivatives to prepare honeycomb films, and then converted the films to carbon networks via calcination [Citation10,Citation11].
Shimomura and co-workers synthesized amphiphilic polyacrylamide derivatives by free radical polymerization of acrylamide monomers with hydrophobic and hydrophilic side chains [Citation12]. The amphiphilicity of these polyacrylamides was easily controlled by changing the copolymerization ratio of the two types of monomers, and the functionalization was altered by swapping the side chains. End-functionalized hydrophobic polymers have also been used as amphiphilic polymers for honeycomb films. Srinivasarao et al. reported multilayered porous film formation from carboxylic acid-functionalized polystyrene [Citation13]. Billon et al. also used a polystyrene ionomer to fabricate uniform honeycomb films [Citation14]. Polysulfone and sulfonated polysulfone have also been used to form honeycomb films [Citation15,Citation16], and the three-dimensional structure of the film could be controlled by changing the degree of sulfonation.
Various amphiphilic polyion complexes, which consist of polyelectrolytes and counter ionic amphiphiles, have also been reported [Citation1,Citation17,Citation18].
One advantage of using polyion complexes for honeycomb film preparation is that they solubilize water-soluble polyelectrolytes in the water-immiscible organic solvents used to prepare films. For example, a polyion complex of heparin or dextran sulfate, which are biomacromolecules containing anionic sulfate groups, and dihexadecyl dimethylammonium form honeycomb films [Citation17]. Furthermore, honeycomb films of thermally durable polyimides can be prepared from polyion complexes of polyamic acids, which are precursors of polyimides, and cationic amphiphiles. The honeycomb structure was formed from a chloroform solution of the polyamic acid/amphiphile polyion complex, and then imidized to convert the polyamic acid to a polyimide [Citation18].
Functional polymers also form honeycomb films. Erdogan et al. showed that the poly(para-phenylenevinylene) derivative forms uniform honeycomb films [Citation19]. Liquid crystalline polyacetylene derivatives produced by Yabu and co-workers also formed uniform honeycomb structures [Citation20]. Honeycomb films prepared from stimuli-responsive polymers have been also reported. Nygard et al. synthesized block copolymers containing poly(N-isopropyl acrylamide), which is a common thermoresponsive polymer, by reversible addition-fragmentation transfer (RAFT) polymerization, and used the copolymers to prepare honeycomb films [Citation21]. The pose size of the obtained honeycomb films could be reversibly changed between two states by changing the temperature of the aqueous media. Honeycomb films containing photochromic compounds have also been prepared. The color of polymers containing azobenzene [Citation22] or spiropyran [Citation23] is changed by exposure to UV or visible light. Fluorinated polymers, which have low surface free energies, are also used to prepare honeycomb films for creating superhydrophobic surfaces [Citation24]. Two- and three-dimensional porous structures have been formed from oligomers containing ferrocene that exhibit an electrochemical response [Citation25,Citation26]. Furthermore, fluorine-based rod-coil block copolymers form honeycomb films that show blue fluorescence and high water repellency [Citation27].
Polymers with well-defined architectures synthesized by living radical polymerization methods, such as atom-transfer radical polymerization [Citation28] and RAFT polymerization [Citation29], have been used for fabricating honeycomb films. Well-defined multi-block copolymers [Citation30], star-shaped polymers [Citation31], hyperbranched polymers [Citation32], and other unique architectures [Citation33] have been used to fabricate honeycomb films. The review by Stenzel et al. summarizes this area of polymer architecture and honeycomb films preparation [Citation34]. Nanogels prepared from poly(vinyl acetate) crosslinked with divinyl acetate have also been used to form honeycomb films; however, the uniformity of the porous structure was low [Citation35].
2.2. Supramolecular assemblies of low-molecular weight compounds
Several kinds of supramolecular assemblies of low-molecular weight amphiphilic molecules have been used for honeycomb film preparation. For a long time, small compounds were not used for honeycomb film preparation, except as additives to polymer solutions, because it is difficult for low-molecular compounds to maintain the mesoscopic honeycomb structure after solvent evaporation. Recently, low-molecular weight gelators, based on supramolecular assemblies formed via intermolecular hydrogen bonding, have allowed porous structures to maintain their shape after evaporation of solvent and template water droplets during honeycomb film preparation. Kim and co-workers showed that amphiphilic photocrosslinkable supramolecular gelators form porous structures and demonstrated photopatterning of the films [Citation36] (). Triazole and guanosine derivatives, which form multivalent hydrogen bonds and are common supramolecular gelators, have also been used to form honeycomb films [Citation37]. Amphiphilic calix[4]arenes [Citation38] also form uniform honeycomb films.
Figure 4. Supramolecular assembly of low-molecular weight compounds for forming honeycomb structures. Top panel shows the chemical structure of the used compound. Bottom panels present SEM images of a dried gel (left) and honeycomb film (right), respectively. Reproduced with permission from [Citation36] (Copyright 2009, Wiley).
![Figure 4. Supramolecular assembly of low-molecular weight compounds for forming honeycomb structures. Top panel shows the chemical structure of the used compound. Bottom panels present SEM images of a dried gel (left) and honeycomb film (right), respectively. Reproduced with permission from [Citation36] (Copyright 2009, Wiley).](/cms/asset/8c41c2c9-8cb8-4b70-9daa-ffd9b5095f33/tsta_a_1528478_f0004_b.gif)
2.3. Nanoparticles and low-dimensional materials
Inorganic nanoparticles are promising materials for functionalizing porous materials. Russell and co-workers have reported forming porous films from solutions of metal nanoparticles and polymers [Citation39] (). Metal nanoparticles functioned as compatibilizers for polymer blends because they are amphiphilic, and thus they stabilized the template water droplets and polymer solution during film formation. Porous films with pores covered with metal nanoparticles were obtained. Silica and other nanoparticles have also been used to stabilize template water droplets [Citation40,Citation41–Citation43]. These results indicate that metal and inorganic nanoparticles can be used as amphiphiles to stabilize water–solution interfaces, and hierarchic structures of nanoparticles and polymers can be formed.
Figure 5. Schematic and cross-sectional transmission electron microscopy (TEM) images of polymer–nanoparticle composite honeycomb films. TOPO stands for trioctylphosphine oxide. Reproduced with permission from [Citation39] (Copyright 2010, Nature Publishing Group).
![Figure 5. Schematic and cross-sectional transmission electron microscopy (TEM) images of polymer–nanoparticle composite honeycomb films. TOPO stands for trioctylphosphine oxide. Reproduced with permission from [Citation39] (Copyright 2010, Nature Publishing Group).](/cms/asset/a4926e82-6d1d-47c4-ba07-4f59eb99ba39/tsta_a_1528478_f0005_oc.jpg)
Monolithic inorganic porous films have been fabricated from nanoparticles by using the breath figure technique. Yonezawa et al. formed a uniform honeycomb Ag structure by casting a hydrochlorofluorocarbon solution of Ag nanoparticles stabilized with fluorinated alkyl thiols under humid conditions [Citation44]. Han and co-workers used a dispersion of dodecanethiol-stabilized gold nanoparticles to obtain porous gold films [Citation45]. They formed ellipsoidal pores by tilting the airflow direction at different angles during the solidification process. They explained the formation of ellipsoidal pores in terms of interfacial tension; however, because such structures are rare in polymers, other parameters, including dispersion viscosity, may affect the pore formation. Zhang et al. reported the formation of honeycomb films from metal oxide nanoparticles, which can be used for fuel cells stabilized with lipids [Citation46]. Saito et al. reported that dispersions of inorganic nanoparticles stabilized with amphiphilic copolymers containing catechol groups could be used to form inorganic hierarchic porous films [Citation47,Citation48] (). When TiO2 and other oxide nanoparticles with diameters on a scale of tens of nanometers were stabilized with an amphiphilic copolymer, they dispersed as secondary aggregates. The hierarchic porous films contained micrometer-sized pores formed by the breath figure technique and calcination, and nanoscale pores among the nanoparticles. Hierarchic porous films have a high relative surface area and they can be used as electrodes for dye-sensitized photovoltaic devices.
Figure 6. Illustrations of a film (a) before and (b) after sintering. Surface and cross-sectional SEM images of honeycomb-patterned porous films composed of (c)–(f) SiO2, (g)–(j) TiO2, (k)–(n) Al2O3, (o)–(r) ZnO, (s)–(v) anisotropic small ZnO (short rods), and (w)–(z) anisotropic large ZnO nanoparticles (long rods) before and after heating at 600 °C in air. Reproduced with permission from [Citation47,Citation48] (Copyright 2013, Wiley).
![Figure 6. Illustrations of a film (a) before and (b) after sintering. Surface and cross-sectional SEM images of honeycomb-patterned porous films composed of (c)–(f) SiO2, (g)–(j) TiO2, (k)–(n) Al2O3, (o)–(r) ZnO, (s)–(v) anisotropic small ZnO (short rods), and (w)–(z) anisotropic large ZnO nanoparticles (long rods) before and after heating at 600 °C in air. Reproduced with permission from [Citation47,Citation48] (Copyright 2013, Wiley).](/cms/asset/cb8495d9-be3d-4128-a08b-eb5b53b297d8/tsta_a_1528478_f0006_oc.jpg)
Kimizuka and co-workers fabricated honeycomb structures with three-dimensional networks from a one-dimensional complex of amphiphiles and metal ions by the breath figure technique [Citation49]. Low-dimensional carbon materials, such as graphene, are attractive for highly conductive electrodes. Kim and co-workers prepared honeycomb films from graphene oxide, and then reduced the film to obtain a conductive material [Citation50]. Wakamatsu et al. also reported single-walled carbon nanotubes (SWCNTs) and graphene oxide honeycomb films. SWCNT honeycomb films prepared on a polyethylene terephthalate (PET) substrate have high near-infrared transparency and conductivity [Citation51].
3. Progress on preparation technologies
In this section, we summarize the many factors controlling the three-dimensional structures of the films fabricated by the breath figure method.
3.1. Solvent
A suitable solvent should be chosen for honeycomb film preparation. Ideally, the solvent should be water-immiscible so that the water droplets do not mix with the polymer solution and cause polymer precipitation. For example, when water miscible tetrahydrofuran (THF) was used as a solvent, heterogeneous sized pores were formed at a high polymer concentration [Citation9], although uniform sized polymer particles precipitated in the water after complete evaporation of THF [Citation52].
The pore size of honeycomb films is equal to the size of the water droplets; thus, controlling the size of the water droplets is important. Various parameters control the pore size of water droplets, including evaporation time [Citation53], interfacial tension between water and solution, and solvent boiling point, which affects evaporation time [Citation54,Citation55]. When the boiling point of the solvent is high, the solvent evaporates slowly, and evaporative cooling is not sufficient to condense water droplets from the atmosphere; there must be a temperature difference between the solution surface and the dew point of the atmosphere for condensation occur. Even though some water droplets form on the surface, there are not enough to form a hexagonal lattice, and only small pores are obtained. When solvents with lower boiling points are used, the difference between the surface temperature of the solution and the dew point increases, and more water condenses on the solution surface.
3.2. Interfacial tension between water and solution
Interfacial tension between the water droplets and solution governs the shape of pores. Govor et al. reported that the shapes of the template water droplets changed from spherical to ellipsoidal by varying the polymer in solution [Citation56]. The pores were larger for a poly(para-phenylenevinylene) precursor than for a poly(3-octylthiophene) precursor. Because the interfacial tension among the air, solution, and water should be balanced, the water droplets sank in the solution when the interfacial tension between polymer solution and water was low. Because the interfacial tension between the water droplets and solution also depended on the polymer, the position of the droplets at the interface also changed, altering the apparent pore sizes of the honeycomb film.
Fukuhira and co-workers reported that phospholipids could control the interfacial tension between the polymer solution and water, and they determined the range of interfacial tension suitable for preparing honeycomb films [Citation57]. Various phospholipids were used to form honeycomb films; however, only a chloroform solution of poly(D,L-lactic acid) and dioleoylphosphatidylethanolamine, which has an interfacial tension against water of around 16 mN/m, formed uniform honeycomb structures. Other phospholipids decreased the interfacial tension between the solution and water further, and the template water droplets spread at the air–solution interface, fusing together. Using an amphiphilic copolymer instead of the phospholipids also made the water droplets spread. Since surfactants should be soluble in organic solvents in the breath figure technique, only a few water-soluble surfactants, which can be dissolved in water-immiscible organic solvents, can be used. This is the reason why only long alkyl chain phospholipids have been employed for honeycomb film fabrication. Kojima et al. reported that the size of the polymer frames decreased with decreasing interfacial tension, which depended on the number of hydrophilic moieties in the amphiphilic copolymers [Citation58]. Because the water droplets deformed from spherical to ellipsoidal as the interfacial tension decreased, the overlap between water droplets increased, decreasing the size of the polymer frame. Wan et al. reported a similar experiment for characterizing the shapes of the pores [Citation59].
3.3. Substrates
Honeycomb films can be prepared on hydrophilic substrates, including glass, metals, and other inorganic substrates that are not eroded by solvents, because water-immiscible organic solvents are used. In addition, organic solid substrates, including PET, which is a stable, inert material, are good candidates.
Honeycomb films can be fabricated on various curved or structured substrates because the liquid thin film follows the shape of the surfaces. For example, honeycomb films can be formed on bas-relief structures [Citation60], and Qiao and co-workers reported the fabrication of honeycomb films on structured substrates [Citation61,Citation62]. Honeycomb films have been formed on substrates with many different shapes [Citation63]. By using line-patterned substrates, the position and arrangement of the honeycomb film formation can be controlled [Citation64]. Honeycomb films have been formed by vertical loading of a solid substrate with a polymer solution [Citation65], and a micropipette has been used as a film substrate [Citation66].
Liquids have also been used as substrates. Nishikawa and co-workers reported a honeycomb film formed at the air–water interface [Citation12]. Because the substrate water readily fused with the template water droplets, pores that passed through the film from top to bottom were created. Ice has also been used as a substrate [Citation67]. These substrates can be removed easily at room temperature, improving the handling of the film.
3.4. Processing
There are various breath figure fabrication processes for obtaining uniform honeycomb films. Conventionally, a polymer solution is cast onto a glass dish because the polymer solution has low viscosity and is difficult to keep on a substrate, and then humid air flows over the solution surface vertically. This technique has already been well reviewed in the literature. Spin casting [Citation9], vertical drawing [Citation65,Citation68], and other film forming techniques [Citation69] have been used to fabricate honeycomb films. However, these films still contain defects and heterogeneous pores owing to factors such as inhomogeneous nucleation and over- or under-nucleation of the condensed water droplets, turbulence in the humid airflow, and convection in the polymer solution during solvent evaporation.
Before developing a fabrication process for honeycomb films, it is important to understand the dynamic condensation, assembly of water droplets, and drying processes in the breath figure technique. For the rapid evaporation of solvent, the smallest condensed water droplet was about 20 nm, which is identical to the thermodynamically estimated critical size of water droplets [Citation70]. Karthaus et al. reported that the size and order of water droplets gradually increased during solvent evaporation observed by in situ light diffraction experiments. They also observed the rearrangement of water droplets to form well-ordered honeycomb structures by optical microscopy [Citation71]. Wang and co-workers also observed rearrangements of water droplets during solvent evaporation [Citation72]. Hypergravity experiments revealed the effect of gravity on the uniformity of honeycomb structures through thermal convection during solvent evaporation [Citation73].
Yamazaki et al. reported a method for the continuous fabrication of honeycomb films on solid substrates, including PET films, and the formation of defectless honeycomb films [Citation74] (). The solid substrate is moved from right to left and the temperature of the substrate is controlled by a Peltier device. After casting the polymer solution on the surface of the solid substrate, humid air is applied along the direction that the substrate is moving in. Furthermore, they found that the number of water droplets should be controlled to form a densely packed honeycomb lattice covering the whole surface of the polymer solution. If the number of water droplets is greater or less than that required to form the densely packed lattice, the film forms cracks or multilayered defects on its surface, respectively. Their technique controlled the number of water droplets and produced densely packed, defect-free honeycomb films.
Figure 7. (a) Schematic illustration of continuous fabrication of honeycomb films and (b) a photograph of an A4 size honeycomb film prepared on a PET film. The inset image shows a laser diffraction pattern obtained from the film. Reproduced with permission from [Citation74] (Copyright 2013, Royal Society of Chemistry).
![Figure 7. (a) Schematic illustration of continuous fabrication of honeycomb films and (b) a photograph of an A4 size honeycomb film prepared on a PET film. The inset image shows a laser diffraction pattern obtained from the film. Reproduced with permission from [Citation74] (Copyright 2013, Royal Society of Chemistry).](/cms/asset/eb2076f9-5f2f-4f23-8e8a-c7bc69e332fd/tsta_a_1528478_f0007_oc.jpg)
4. Post-modifications
4.1. Deposition of inorganic materials
Metals can be deposited on honeycomb films by physical or chemical techniques to produce thermally and electrically conductive films. Physical techniques include sputtering or thermal deposition of metals [Citation75,Citation76]. Discharge sputtering is a simple method of forming thin metal layers on organic materials, and has been used to obtain highly conductive honeycomb films coated with metals.
Chemical deposition techniques include plating, where metal ions are reduced by reducing agents in solution, depositing metals on the surface of the substrate. We reported on simple electroless deposition of metals to form metal layers on the surface of honeycomb films. In particular, when the substrate surface was functionalized with a Pt or Pd catalyst, reduction occurred selectively on the catalyst surfaces () [Citation77]. Selective plating was used to metalize the interior of pores [Citation78]. Furthermore, photochromic amphiphilic polymers containing a spiropyran side chain were used for photoselective deposition of metals [Citation79] (). Connal and co-workers fabricated metal-containing honeycomb films from spiropyran polymers [Citation80]. Zinc oxide thin films were constructed on the surface of honeycomb films by depositing zinc on the surface of honeycomb films followed by oxidation [Citation81,Citation82]. A hydrothermal method was also used to form ZnO crystals on honeycomb film surfaces [Citation83]. Electrostatic adhesion of TiO2 nanoparticles onto honeycomb films comprising poly(styrene-co-maleic anhydride) are also examined [Citation84]. Since nanopatterned surface is useful for catalytic applications [Citation85,Citation86], these oxide semiconductor honeycomb films can be applicable to photocatalytic applications.
Figure 8. Photopatterning of metallic honeycomb films fabricated from a spiropyran-containing amphiphilic polymer. Bottom images show photographs and SEM images of prepared honeycomb film (left), photo-patterned honeycomb film (center), and plated honeycomb film (right), respectively. Reproduced with permission from [Citation77] (Copyright 2010, Royal Society of Chemistry).
![Figure 8. Photopatterning of metallic honeycomb films fabricated from a spiropyran-containing amphiphilic polymer. Bottom images show photographs and SEM images of prepared honeycomb film (left), photo-patterned honeycomb film (center), and plated honeycomb film (right), respectively. Reproduced with permission from [Citation77] (Copyright 2010, Royal Society of Chemistry).](/cms/asset/031739cd-8817-4bba-bcf8-b14d43bf97c3/tsta_a_1528478_f0008_oc.jpg)
4.2. Pattern transferring
Honeycomb films have spherical pores that reflect the structure of the template water droplets. By using this unique structure, microlens arrays can be formed by using the honeycomb films as templates [Citation87,Citation88]. The porous structures of a polystyrene honeycomb film are filled with a polydimethylsiloxane (PDMS) elastomer prepolymer and then cured. The PDMS microlens arrays were obtained by removing the template honeycomb films with chloroform. This process is robust and suitable for a wide variety of materials that do not dissolve the template honeycomb films. Microlens arrays have been formed from metals, polyvinylpyrrolidone, and other materials, and then used as a template again to produce hexagonally arranged microdimple structures.
Inorganic porous materials can also be prepared by pattern transferring. This process is called breath figure lithography. Haupt et al. fabricated Si cylinder arrays with a high aspect ratio [Citation89] () by forming a positive mold of the honeycomb structures of a copolymer containing methylmethacrylate (MMA) and fluorinated MMA on a Si substrate by depositing gold on the bottom of the pores followed by dry etching. The cylinder array can be used as an optical cutoff filter in the infrared region. Hirai et al. reported Si nanoscale pincushion arrays covered with fluorinated polymers, obtained by dry etching the Si substrate with a pillar structure template created by peeling off the top layer of a honeycomb film with adhesive tape () [Citation90]. The Si substrate was sequentially etched with SF6 gas and protection gas by using the Bosch method. Because the protection gas contained fluorinated carbon, fluorinated hydrocarbon layers formed on the pincushion array. The Si pincushion arrays showed high water repellency and low light scattering. Li co-workers also showed that honeycomb films can be used as templates for forming porous structures on Si and glass substrates [Citation91].
Figure 9. Pattern transfer onto a Si surface by metal deposition and dry etching. The right SEM image shows a pillar structure obtained after etching. Reproduced with permission from [Citation89] (Copyright 2004, American Society of Physics).
![Figure 9. Pattern transfer onto a Si surface by metal deposition and dry etching. The right SEM image shows a pillar structure obtained after etching. Reproduced with permission from [Citation89] (Copyright 2004, American Society of Physics).](/cms/asset/55c3d8ba-767d-4999-b5ee-e36e9c063ecb/tsta_a_1528478_f0009_b.gif)
Figure 10. Schematic and SEM images of pattern transfer of a pillar film onto Si substrates by dry etching. Reproduced with permission from [Citation90] (Copyright 2011, Royal Society of Chemistry).
![Figure 10. Schematic and SEM images of pattern transfer of a pillar film onto Si substrates by dry etching. Reproduced with permission from [Citation90] (Copyright 2011, Royal Society of Chemistry).](/cms/asset/fa7b09dd-6d17-42b3-89ae-3ab0b5f02be4/tsta_a_1528478_f0010_oc.jpg)
Wet etching is also used to form inorganic porous structures. Saito and Yabu demonstrated wet etching of aluminum, titanium, and other inorganic substrates by using honeycomb films as templates [Citation92]. In wet etching, adhesion between honeycomb films and inorganic substrates is important because the wet etching solution is often strongly alkaline or acidic; it can easily disrupt adhesion, which usually occurs via van der Waals forces, hydrogen bonding, and electrostatic interactions, and remove the template from the inorganic substrate. To prevent the removal of the film, it can be coated with an adhesive, such as polyvinylalcohol (PVA), or adhesive functional groups can be introduced on the surface of honeycomb films. PVA is a polymeric amphiphilic adhesive that can adhere to both hydrophobic honeycomb films and hydrophilic inorganic substrates; however, PVA is water soluble. Saito et al. introduced catechol groups, which are the adhesive functional groups in mussel adhesive, on the surface of honeycomb films. The honeycomb films adhered strongly on the surface of inorganic and organic substrates, even in strong alkaline etchant solution [Citation93]. These adhesion techniques allow the surface topography of honeycomb films to be transferred onto inorganic materials.
Another simple pattern transfer method involves inducing buckling of the PDMS surface by oxidation though the pores of honeycomb films. When thin rigid oxidized layers form on the surface of PDMS, buckling occurs and forms microwrinkles. When the size of the pores of the honeycomb films is smaller than that of the wavelength of the microwrinkles, the porous structure is imprinted on the PDMS surface as dimples [Citation94].
4.3. Mechanical deformation
Honeycomb films prepared by elastic or viscoelastic polymers can be deformed by stretching or shrinking. Nishikawa et al. reported that mechanically stretching honeycomb films of poly(ε-caprolactone) (PCL), a viscoelastic biodegradable polymer, deformed their microporous structures along the stretching directions [Citation95]. The deformed shapes of the porous structures were characterized by the stretching ratio and relation between the lattice vector of the honeycomb structure and the direction of stretching. Lattice vectors of honeycomb lattices were determined in the same way as Brillouin zones of crystals. When the stretching was along the K-G direction, the apexes of the honeycomb lattices were stretched, and the stretched lattices maintained their hexagonal periodicities. However, rectangular pores were formed in the stretching direction due to the deformation of the hexagonal structures.
Shrinking honeycomb films also deforms the porous structure. Honeycomb films of elastic 1,2-polybutadiene (PB) on thermally shrinkable film substrates were mechanically shrunk by heating () [Citation96]. As with stretching, the surface structures of honeycomb films after shrinking depend on the shrinking ratio and direction of shrinking. Nanosized porous structures can be obtained from micrometer pores by multiple shrinking processes.
Figure 11. SEM images of shrunk PB honeycomb films on thermally shrinkable substrates. Reproduced with permission from [Citation96] (Copyright 2008, Wiley).
![Figure 11. SEM images of shrunk PB honeycomb films on thermally shrinkable substrates. Reproduced with permission from [Citation96] (Copyright 2008, Wiley).](/cms/asset/05ba5210-b289-4a4d-9bf8-452b40c513fb/tsta_a_1528478_f0011_b.gif)
Because the water droplet templates are spherical, honeycomb films have double-layered structures connected with pillars that are thin in the center. Peeling off the top layer of the film with adhesive tape breaks the pillars at their thinnest points, creating pillared surface structures [Citation97].
4.4. Crosslinking
Although robust polyimide honeycomb films have been reported, crosslinking improves the thermal and chemical stability of films. Bunz and co-workers reported the formation of stable honeycomb films from an azide-functionalized poly(para-phenyleneethynylene) derivative [Citation19]. The azide group was cross-linked with a Pt catalyst to form rigid, stable films. Liquid epoxy resins, which can be cross-linked by a photosensitive initiator, have also been used to prepare robust honeycomb films [Citation98]. Liquid epoxy resins with a UV light-sensitive initiator were dissolved in chloroform, and then cured under UV light in humid conditions during solvent evaporation. Honeycomb films remained after complete evaporation of solvent owing to the crosslinking of epoxy resins. Because the resin was liquid, the double-layered film structure collapsed, and a single-layered honeycomb structure was formed [Citation99]. To maintain the structure of original honeycomb structures, crosslinking after formation of the honeycomb structure has been investigated. Long and co-workers synthesized poly(D,L-lactide)s with cross-linkable side chains, and used them for honeycomb film formation [Citation100]. The film structure was fixed by photocrosslinking the side chains. Although the structure of the film was not uniform, a robust porous structure was obtained. Karthaus and co-workers crosslinked poly(maleic anhydride) and alkyl diamines after forming honeycomb structures [Citation101]. They also reported photocrosslinking of polycinnamates to form solvent-resistant honeycomb films ()) [Citation102]. Other photocrosslinking methods have been used to form stable honeycomb films. Qiao and co-workers crosslinked star polymers through dimerizing the anthracene side chains [Citation63]. Furthermore, honeycomb structures can also be fixed by photocrosslinking PB, a well-known synthetic rubber, by UV irradiation, and photopatterning can also be performed ()) [Citation103,Citation104]. Thus, hierarchic structures can be produced by the bottom-up breath figure technique and top-down photopatterning.
Figure 12. SEM image of a photocrosslinked honeycomb film of polycinnamate (a) and SEM images of photopatterned PB honeycomb films (b)–(e). Reproduced with permission from [Citation102,Citation103] (Copyright 2010, Royal Society of Chemistry).
![Figure 12. SEM image of a photocrosslinked honeycomb film of polycinnamate (a) and SEM images of photopatterned PB honeycomb films (b)–(e). Reproduced with permission from [Citation102,Citation103] (Copyright 2010, Royal Society of Chemistry).](/cms/asset/9b9755cf-bd63-4fb4-8934-26dd9a64f2c5/tsta_a_1528478_f0012_b.gif)
4.5. Surface modification
To change the surface properties of honeycomb films, several kinds of surface modification have been examined. Honeycomb films show strong water repellency because of their surface structures and the hydrophobic polymers from which they are prepared.
Surface modifications have been used to make honeycomb films hydrophilic. Treating the surface of polystyrene honeycomb films with UV-ozone decreased the water contact angle with increasing treatment time, and the film surface eventually became easy to wet with water because of the oxidation of the surface hydrocarbons. After this treatment, the holes in the film could be filled with an aqueous dispersion of particles [Citation105].
Layer-by-layer (LbL) techniques have been used to modify the surface of honeycomb films. Wan and co-workers reported the surface modification of polystyrene and poly(N,N-dimethylaminoethyl methacrylate) amphiphilic block copolymers by the LbL technique [Citation106,Citation107]. The water contact angles decreased as the number of layers increased.
Chemical modification of the film surface has been used to introduce more specific chemical moieties. In particular, immobilizing bioactive ligands has attracted intense interest. Nishida et al. reported biotin-functionalized honeycomb films fabricated from amphiphilic copolymers with biotin side chains. Avidin was immobilized on the biotin moieties, and gelatin, which is a useful ligand for cell adhesion, was immobilized on the modified film surface [Citation108]. Stenzel and co-workers selectively introduced streptavidin on the surface of the pores () [Citation109]. They used polystyrene-b-PAA to form honeycomb films with acrylic acid groups on the surface of the pores that were then coupled with biotinamidohexanoic acid hydrazide to immobilize biotin, which selectively interacts with streptavidin. Streptavidin was immobilized in the pores by immersing the film in a streptavidin solution. A similar approach exploiting the avidin–biotin interaction has been reported by Ke et al. [Citation110] and Nyström et al. [Citation111]. Click-chemistry has also been used to immobilize bioactive ligands [Citation112]. Honeycomb films bearing a dye that could be displaced by sugar were fabricated by hydroboration of polymers containing phenylboronic acids, and these films could be used in glucose sensors [Citation113]. Surface modification with polyaniline, to increase the electrical conductivity of honeycomb films, was also examined [Citation114].
Figure 13. Schematic of the immobilization of proteins in honeycomb pores. Reproduced with permission from [Citation109] (Copyright 2008, Wiley).
![Figure 13. Schematic of the immobilization of proteins in honeycomb pores. Reproduced with permission from [Citation109] (Copyright 2008, Wiley).](/cms/asset/ecb01851-c1bd-492f-9401-452b27e830cf/tsta_a_1528478_f0013_b.gif)
5. Potential applications of honeycomb films
5.1. Optical and photonics applications
Honeycomb-patterned porous films are likely to have major applications in optics and photonics. Owing to the highly ordered honeycomb array of spherical pores, photonic crystal applications have attracted great interest. Haupt and co-workers prepared a honeycomb film from a rod-coil block copolymer on a Si substrate, and used the film as a template for dry etching the Si substrate to obtain pillar arrays, which functioned as photonic crystals in the infrared region [Citation89]. Kurono et al. prepared honeycomb films from PCL and an amphiphilic copolymer, and used a similar technique to prepare an infrared region stop band [Citation115].
Owing to the unique double-layered structure of honeycomb films, transmittance through the film strongly depends on the incident angle [Citation116]. Light irradiated in the normal direction to the metalized double-layered honeycomb films passed though the pores in the upper and bottom surfaces with high transmittance. However, the polymer rims of bottom layers blocked light falling at oblique angles. Consequently, the metalized honeycomb films had a limited viewing angle, even in thin films. This property may be useful for improving privacy in mobile devices.
Nanospike structures have been formed by using a pillar template, which was created by peeling off the top layer of a honeycomb film, for dry etching a Si wafer [Citation117]. Because the nanospikes have edges smaller than the wavelength of visible light, they can be used for anti-reflection surfaces, known as moth-eye structures. The gradual change in refractive index at the air–Si interface prevented light reflection, and the surface of the patterned Si wafer looked black. The reflectance of the Si wafer was less than 1% in the visible light range. This property is expected to raise the efficiency of Si photovoltaic cells by increasing the effective absorption of light by the cell.
Metal nanopillar structures obtained by sputtering pillar structures can be used for surface-enhanced Raman scattering (SERS) substrates () [Citation118]. Because the triangular pillars formed from template water droplet arrays had nanosized sharp edges, the electromagnetic field was strongly enhanced at the metallic edges. The metallized pillar structures have been used to detect molecules dispersed in aqueous media by SERS, demonstrating that these pillar structures can be used as high-sensitivity sensors for analyzing molecular structures.
Figure 14. Schematic illustration (a) and SEM images (b)–(d) of silver pillar structure and SERS signals from rhodamine 6G measured on flat and silver pillar structures (e). Reproduced with permission from [Citation118] (Copyright 2010, Royal Society of Chemistry).
![Figure 14. Schematic illustration (a) and SEM images (b)–(d) of silver pillar structure and SERS signals from rhodamine 6G measured on flat and silver pillar structures (e). Reproduced with permission from [Citation118] (Copyright 2010, Royal Society of Chemistry).](/cms/asset/cfd6122b-b83b-4460-b7b6-f2dc948a76d9/tsta_a_1528478_f0014_oc.jpg)
The micrometer-sized pores in honeycomb films can be served as a template for microlens arrays (Section 4.2) [Citation87,Citation119]. Microlens arrays can be used as light collectors or scattering screens for liquid crystal projectors, and for enhancing outcoupling of light from organic light-emitting diodes [Citation120].
5.2. Applications of surface properties
Because of their hydrophobic nature and unique surface structures, honeycomb films and their derivative structures have been used for various applications. A common application is superhydrophobic surfaces. Because the wettabilities of structured surfaces increased with the roughness and porosity of the surface, as in lotus leaves [Citation121], micro and nanostructured surfaces are suitable for fabricating superhydrophobic surfaces. shows the water contact angles of honeycomb and pincushion structures prepared from a fluorinated polymer [Citation122] (). Because the honeycomb film has a porous structure, the surface is a hybrid surface consisting of the fluorinated polymer and air. The Cassie-Baxter model (Equation (1)) has been used to explain the contact angles on these surfaces.
Figure 15. Schematic of the fabrication of superhydrophobic, superhydrophilic, and omniphobic surfaces by using honeycomb films as templates. Reproduced with permission from [Citation112,Citation126,Citation127] (Copyright (a) 2005, (b) 2014 American Chemical Society, (c) 2014 Wiley).
![Figure 15. Schematic of the fabrication of superhydrophobic, superhydrophilic, and omniphobic surfaces by using honeycomb films as templates. Reproduced with permission from [Citation112,Citation126,Citation127] (Copyright (a) 2005, (b) 2014 American Chemical Society, (c) 2014 Wiley).](/cms/asset/82e56a7b-a4ed-45c3-8a40-7670131b5504/tsta_a_1528478_f0015_oc.jpg)
Here, θ is the apparent contact angle, A is the polymer surface fraction, and θp is the contact angle on the flat polymer surface. The black line in indicates the water contact values obtained from Equation (1), and the experimental values matched with the calculated values well.
Post-modifications of honeycomb films have produced unique surface properties. For example, superhydrophobic surfaces with strong water droplet adhesion properties were created by combining electroless plating and pillar formation [Citation123,Citation124]. Thermoresponsive wettability control from the superhydrophobic to the wetting state has been achieved by using amphiphilic copolymers containing thermoresponsive moieties [Citation125]. Superhydrophilic surfaces prepared by surface oxidation of the polymer honeycomb and pincushion structures have also been reported, and they showed high bubble repellency [Citation126]. Furthermore, biomimetic omniphobic lubricated surfaces with on-demand liquid transportation properties were produced by combining fluorinated PB pincushion surfaces with fluorinated lubricants [Citation127]. The fluorocarbon was immobilized on the surface of PB honeycomb films by the ene-thiol reaction, and the pincushion structures were formed by peeling off the top film layer. The surface was then filled with fluoroether lubricant to obtain the omniphobic surface. Liquid transportation on the surface PB honeycomb films can be controlled by stretching and patterning by photocrosslinking.
Honeycomb structures were used to control interfacial friction. Hexagonally arranged dimple patterns with no burrs were formed on metal surfaces by wet etching with honeycomb films as templates [Citation92]. The friction coefficient of the micro dimple arrays was lower than that of a polished surface with fluid lubrication; however, the mechanism of the friction reduction has not been revealed. Fabricating dimple arrays on surfaces could be used to reduce the friction between surfaces in automobiles and other vehicles.
5.3. Electronic applications
Recently, the applications of honeycomb films in electronics have also been investigated. As mentioned in Section 4, metallization of honeycomb films has been realized by sputtering or plating technique. Also, electronic applications of honeycomb films have been done by embedding electroconductive materials in the honeycomb films. Lee and co-workers prepared an organic memory device from tiny carbon fibers formed on the pillars connecting the top and bottom layers of the honeycomb films [Citation128].
5.4. Biomedical applications
Honeycomb-patterned porous films have promising biomedical applications. From the early stages of development, honeycomb-patterned porous films have been used as scaffolds for cell culturing. In regenerative medicine, cells, cytokines, and scaffolds are three components for growing tissues for transplantation. Biologists have changed the conditions of cells and cytokines to control cell behavior; however, the types of scaffolds that are for suitable tissue generation have not been thoroughly investigated. Materials including collagen gels [Citation129] and patterned substrates [Citation130] have been used as scaffolds.
Honeycomb-patterned porous films have some advantages as cell culturing scaffolds, including control of pore size. The surface topology and elasticity of scaffolds strongly affect cell behavior, including adhesion, proliferation, and differentiation; therefore, the control of the topographical and mechanical properties of scaffolds is important. Compared with existing porous scaffolds, which have pores with size distributions, honeycomb-patterned porous films have uniform-sized pores with sizes from the sub-cellular to the super-cellular scale. The pore size can be controlled by controlling the template water droplets from sizes of several tens of nanometers to tens of micrometers. Endothelial cells [Citation131], fibroblasts [Citation132], hepatocytes [Citation133], and other cells [Citation109,Citation134–Citation139] have been cultured and suitable pore sizes for cell growth and function have been reported (). When neural stem cells and human mesenchymal stem cells [Citation140] were cultured on honeycomb-patterned porous scaffolds, the differentiation of these stem cells changed [Citation141,Citation142].
Figure 16. Honeycomb films for cell culturing substrates. PLA stands for polylactic acid. Reproduced with permission from [Citation131] (Copyright American Chemical Society and Elsevier).
![Figure 16. Honeycomb films for cell culturing substrates. PLA stands for polylactic acid. Reproduced with permission from [Citation131] (Copyright American Chemical Society and Elsevier).](/cms/asset/81b68483-ed57-43b5-9fa4-92c8ed0589da/tsta_a_1528478_f0016_oc.jpg)
The mechanical properties of honeycomb-patterned porous films, such as elasticity, can also be controlled by changing the film materials. For example, poly(L-lactic acid) is a well-known biodegradable hard polymer [Citation143], whereas PCL is an elastic biodegradable polymer. These properties also affect cell behavior. For example, the anisotropic arrangement of cardiac myocytes was achieved by elongating PCL honeycomb-patterned scaffolds, and the anisotropic pulsating of the cells was observed [Citation95]. This is a good example of the combination of the topography and mechanical properties of the scaffold affecting cell behavior. Furthermore, photocrosslinking of PB can be used to control the surface elasticity of honeycomb-patterned porous scaffolds [Citation144]. Fibroblast adhesion on soft honeycomb-patterned scaffolds was suppressed compared with hard scaffolds crosslinked by UV light () [Citation145].
Figure 17. Mechanical regulation of cellular adhesion on elastomeric honeycomb films. Reproduced with permission from [Citation145] (Copyright 2013, American Chemical Society).
![Figure 17. Mechanical regulation of cellular adhesion on elastomeric honeycomb films. Reproduced with permission from [Citation145] (Copyright 2013, American Chemical Society).](/cms/asset/d71abd22-7faf-406c-846d-40abcb00abb5/tsta_a_1528478_f0017_oc.jpg)
Furthermore, Uraki and co-workers cultured bacteria that produced highly crystalline cellulose [Citation146]. When negative PDMS honeycomb films molds were used as culture substrates, bacteria moved around the valleys in the microlens dimples, producing honeycomb-like cellulose.
PLA honeycomb films can be used as an adhesion barrier to prevent postoperative adhesion between organs [Citation147]. Honeycomb films with pores on the front surface and a flat back surface can hold fluids on the surface of organs and stick to the surface. The film prevents leaking of fibroblasts and the adhesion of other organs.
These studies indicate that the microstructures and mechanical properties of honeycomb films strongly affect the adhesion, proliferation, and differentiation of various cells.
6. Summary and future prospects
In this review, I have summarized the recent development of honeycomb films prepared by the breath figure techniques and their many applications. The production of honeycomb films can be performed on an industrial scale, greatly broadening their possible applications in areas such as optics, photonics, surface science, biotechnology, and regenerative medicine.
However, there are still problems to be solved in the production and applications of honeycomb films. For example, the pore sizes of honeycomb films can be controlled from the sub-micrometer to the tens of micrometers scale. However, the critical size of water droplets at room temperature is normally about 20 nm [Citation148], and water droplet arrays on the tens of nanometers scale could be realized by controlling the fabrication process. Pores larger than 50 µm are also challenging because the lateral capillary force is not strong enough to attract the large template water droplets and gravity affects the template water droplet shapes. Microdroplet manipulation techniques will help to align water droplets of these sizes [Citation149].
Continuous fabrication of honeycomb films on a large surface area is also a problem. Companies have attempted to solve this problem and have improved productivity [Citation150]. This industrial development is important for understanding the breath figure technique and for widening the applications of honeycomb films.
Liquid or solid templates made from materials other than water will also allow the fabrication of porous films with unique, and more controlled and complex shapes. Alcohols have been used to from honeycomb films with high aspect ratio pores arising from the low interfacial tension between the alcohol and polymer solution [Citation151]. Low-molecular weight compounds that can be sublimed are also a candidate for condensable templates.
Control of the properties of honeycomb film materials and uses for their unique microstructures are important for expanding their practical applications. For example, refractive index control of honeycomb films is crucial for using honeycomb films in optics and photonics applications. In the field of regenerative medicine, biodegradable materials, which have controlled degradation periods, should be developed for using honeycomb films as implantable materials. Because honeycomb films have interconnected pores, the side pores could be used to separate or regulate the diffusion of dispersed substances in liquid media. Honeycomb films made from elastomers can be stretched by mechanical stretching, which allows deformation of pores and selective separation depending on the size of materials [Citation152]. These developments will pave the way for developing bottom-up fabrication technologies and unique applications.
Disclosure statement
No potential conflict of interest was reported by the author.
Additional information
Funding
References
- Shimomura M, Koito T, Maruyama N, et al. Photonic and electronic applications of mesoscopic polymer assemblies. Mol Cryst Liquid Cryst Sci Technol Sect A Mol Cryst Liquid Cryst. 1998;322:305–312.
- Widawski G, Rawiso M, François B. Self-organized honeycomb morphology of star-polymer polystyrene films. Nature. 1994;369:387–389.
- Maruyama N, Karthaus O, Ijiro K, et al. Mesoscopic pattern formation of nanostructured polymer assemblies. Supramol Sci. 1998;5:331–336.
- Jenekhe SA. Self-assembly of ordered microporous materials from rod-coil block copolymers. Science. 1999;283:372–375.
- Bunz UHF. Breath figures as a dynamic templating method for polymers and nanomaterials. Adv Mater. 2006;18:973–989.
- Bai H, Du C, Zhang A, et al. Breath figure arrays: unconventional fabrications, functionalizations, and applications. Angew Chem Int Ed. 2013;52:12240–12255.
- Escalã P, Rubatat L, Billon L, et al. Recent advances in honeycomb-structured porous polymer films prepared via breath figures. Eur Polym J. 2012;48:1001–1025.
- Hernández-Guerrero M, Stenzel MH. Honeycomb structured polymer films via breath figures. Polym Chem. 2012;3:563–577.
- Park M, Kim J. Breath figure patterns prepared by spin coating in a dry environment. Langmuir. 2004;20:5347–5352.
- Bashmakov IA, Govor LV, Solovieva LV, et al. Preparation of self assembled carbon network structures with magnetic nanoparticles. Macromol Chem Phys. 2002;203:544–549.
- Govor LV, Parisi J. Hopping charge transport in honeycomb carbon network structures. Zeitschrift Fur Naturforschung Sect A J Phys Sci. 2002;57:757–779.
- Nishikawa T, Nishida J, Ookura R, et al. Web-structured films of an amphiphilic polymer from water in oil emulsion: fabrication and characterization. Langmuir. 2000;16:1337–1342.
- Srinivasarao M, Collings D, Philips A, et al. Three-dimensionally ordered array of air bubbles in a polymer film. Science. 2001;292:79–83.
- Billon L. Tailoring highly ordered honeycomb films based on ionomer macromolecules by the bottom-up approach. Macromolecules. 2009;42:345–356.
- Mansouri J, Yapit E, Chen V. Polysulfone filtration membranes withisoporous structures prepared by a combination of dip-coating and breath figure approach. J Memb Sci. 2013;444:237–251.
- Lu Y. Fabrication of conducting polyaniline composite film using honeycomb ordered sulfonated polysulfone film as template. Thin Solid Films. 2008;516:6365–6370.
- Nishikawa T. Honeycomb-patterned thin films of amphiphilic polymers as cell culture substrates. Mater Sci Eng C. 1999;8–9:495–500.
- Yabu H, Tanaka M, Ijiro K, et al. Preparation of honeycomb-patterned polyimide films by self-organization. Langmuir. 2003;19:6297–6300.
- Erdogan B. Permanent bubble arrays from a cross-linked poly(para-phenyleneethynylene): picoliter holes without microfabrication. J Am Chem Soc. 2004;126:3678–3679.
- Yabu H, Akagi K, Shimomura M. Micropatterning of liquid crystalline polyacetylene derivative by using self-organization processes. Synth Met. 2009;159:762–764.
- Nygard A, Davis TP, Barner-Kowollik C, et al. A simple approach to micro-patterned surfaces by breath figures with internal structure using thermoresponsive amphiphilic block copolymers. Aust J Chem. 2005;58:595–599.
- Jung GH, Kim JK, Thinh PX, et al. Photo-controlled fabrication of honeycomb-patterned films in poly(N-vinylcarbazole/azobenzene) copolymer. J Photochem Photobiol A Chem. 2013;272:6–17.
- Kojima M, Nakanishi T, Hirai Y, et al. Photo-patterning of honeycomb films prepared from amphiphilic copolymer containing photochromic spiropyran. Chem Commun. 2010;46:3970.
- Yabu H, Shimomura M. Surface properties of self-organized honeycomb-patterned films. Mol Cryst Liquid Cryst. 2006;445:125–129.
- Dong R, Yan J, Ma H, et al. Dimensional architecture of ferrocenyl-based oligomer honeycomb-patterned films: from monolayer to multilayer. Langmuir. 2011;27:9052–9056.
- Dong R, Ma H, Yan J, et al. Tunable morphology of 2D honeycomb-patterned films and the hydrophobicity of a ferrocenyl-based oligomer. Chemistry. 2011;17:7674–7684.
- Chiu Y-C, Kuo -C-C, Lin C-J, et al. Highly ordered luminescent microporous films prepared from crystalline conjugated rod-coil diblock copolymers of PF-b-PSA and their superhydrophobic characteristics. Soft Matter. 2011;7:9350.
- Lin C-L, Tung P-H, Chang F-C. Synthesis of rod-coil diblock copolymers by ATRP and their honeycomb morphologies formed by the ‘breath figures’ method. Polymer. 2005;46:9304–9313.
- Hao X, Stenzel MH, Barner-Kowollik C, et al. Molecular composite materials formed from block copolymers containing a side-chain liquid crystalline segment and an amorphous styrene/maleic anhydride segment. Polymer. 2004;45:7401–7415.
- Li Q-Z. Well-defined polyolefin/poly(ε-caprolactone) diblock copolymers: new synthetic strategy and application. J Polym Sci Polym Chem. 2010;49:511–517.
- Connal LA, Vestberg R, Hawker CJ, et al. Dramatic morphology control in the fabrication of porous polymer films. Adv Funct Mater. 2008;18:3706–3714.
- Dong W. Honeycomb-structured microporous films made from hyperbranched polymers by the breath figure method. Langmuir. 2009;25:173–178.
- Chang C-C. Using a breath-figure method to self-organize honeycomb-like polymeric films from dendritic side-chain polymers. Mater Chem Phys. 2011;128:157–165.
- Stenzel MH, Barner-Kowollik C, Davis TP. Formation of honeycomb-structured, porous films via breath figures with different polymer architectures. J Polym Sci Polym Chem. 2006;44:2363–2375.
- Poly J. Nanogels based on poly(vinyl acetate) for the preparation of patterned porous films. Langmuir. 2011;27:4290–4295.
- Kim JH, Seo M, Kim SY. Lithographically patterned breath figure of photoresponsive small molecules: dual patterned honeycomb lines from a combination of bottom up and top down. Adv Mater. 2009. doi:10.1002/adma.200900868
- Gao Y-F, Huang Y-J, Xu S-Y, et al. Ordered honeycomb microporous films from self-assembly of alkylated guanosine derivatives. Langmuir. 2011;27:2958–2964.
- Nomura E. Self-organized honeycomb-patterned microporous polystyrene thin films fabricated by calix[4]arene derivatives. Langmuir. 2010;26:10266–10270.
- Böker A. Hierarchical nanoparticle assemblies formed by decorating breath figures. Nat Mater. 2004;3:302–306.
- Jiang X. Interfacial effects of in situ-synthesized Ag nanoparticles on breath figures. Langmuir. 2010;26:2477–2483.
- Sun W, Shao Z, Ji J. Particle-assisted fabrication of honeycomb-structured hybrid films via breath figures method. Polymer. 2010;51:4169–4175.
- Ma H, Cui J, Song A, et al. Fabrication of freestanding honeycomb films with through-pore structures via air/water interfacial self-assembly. Chem Commun. 2011;47:1154.
- Ma H, Cui J, Chen J, et al. Self-organized polymer nanocomposite inverse opal films with combined optical properties. Chem Eur J. 2010;17:655–660.
- Yonezawa T, Onoue S, Kimizuka N. Self organized superstructures of fluorocarbon stabilized silver nanoparticles. Adv Mater. 2001;13:140–142.
- Li J. Ordered honeycomb-structured gold nanoparticle films with changeable pore morphology: from circle to ellipse. Langmuir. 2005;21:2017–2021.
- Zhang N, Li J, Ni D, et al. Preparation of honeycomb porous La0.6Sr0.4Co0.2Fe0.8O3Gd0.2Ce0.8O2 composite cathodes by breath figures method for solid oxide fuel cells. Appl Surf Sci. 2011;258:50–57.
- Saito Y, Shimomura M, Yabu H. Breath figures of nanoscale bricks: a universal method for creating hierarchic porous materials from inorganic nanoparticles stabilized with mussel-inspired copolymers. Macromol Rapid Commun. 2014;35:1763–1769.
- Saito Y, Shimomura M, Yabu H. Dispersion of Al2O3 nanoparticles stabilized with mussel-inspired amphiphilic copolymers in organic solvents and formation of hierarchical porous films by the breath figure technique. Chem Commun. 2013;49:6081–6083.
- Chang-Soo L, Kimizuka N. Pillared honeycomb nanoarchitectures formed on solid surfaces by the self-assembly of lipid-packaged one-dimensional Pt complexes. Proc Natl Acad Sci USA. 2002;99:4922–4926.
- Lee SH. Three dimensional self assembly of graphene oxide platelets into mechanically flexible macroporous carbon films. Angew Chem Int Ed Engl. 2010;49:10084–10088.
- Wakamatsu N, Takamori H, Fujigaya T, et al. Self-organized single-walled carbon nanotube conducting thin films with honeycomb structures on flexible plastic films. Adv Funct Mater. 2009;19:311–316.
- Yabu H. Creation of functional and structured polymer particles by self-organized precipitation (SORP. Bull Chem Soc Jpn. 2012;85:265–274.
- Xu Y. Effects of drying time on the surface morphology evolution of urushiol–formaldehyde diethylenetriamine polymer microporous films. Appl Surf Sci. 2012;258:5141–5145.
- Xiong X, Lin M, Zou W, et al. Kinetic control of preparing honeycomb patterned porous film by the method of breath figure. React Funct Polym. 2011;71:964–971.
- Tanaka M. Effect of pore size of self-organized honeycomb-patterned polymer films on spreading, focal adhesion, proliferation, and function of endothelial cells. J Nanosci Nanotechnol. 2007;7:763–772.
- Govor L, Reiter G, Bauer G, et al. Formation of low-dimensional close-packed arrays of nanoparticles in a dewetting water layer. Phys Rev E. 2007;76:041609.
- Fukuhira Y, Yabu H, Ijiro K, et al. Interfacial tension governs the formation of self-organized honeycomb-patterned polymer films. Soft Matter. 2009;5:2037.
- Kojima M, Hirai Y, Yabu H, et al. The effects of interfacial tensions of amphiphilic copolymers on honeycomb-patterned films. Polym J. 2009;41:667–671.
- Wan L-S, Ke -B-B, Zhang J, et al. Pore shape of honeycomb-patterned films: modulation and interfacial behavior. J Phys Chem B. 2012;116:40–47.
- Ohzono T, Nishikawa T, Shimomura M. One-step fabrication of polymer thin films with lithographic bas-relief micro-pattern and self-organized micro-porous structure. J Mater Sci. 2004;39:2243–2247.
- Connal LA, Vestberg R, Hawker CJ, et al. Fabrication of reversibly crosslinkable, 3-dimensionally conformal polymeric microstructures. Adv Funct Mater. 2008;18:3315–3322.
- Zhang Z. The behaviour of honeycomb. Polymer. 2013;54:4446–4454.
- Li L. Constructing robust 3-dimensionally conformal micropatterns: vulcanization of honeycomb structured polymeric films. Soft Matter. 2011;7:546.
- Park JS, Lee SH, Han TH, et al. Hierarchically ordered polymer films by templated organization of aqueous droplets. Adv Funct Mater. 2007;17:2315–2320.
- Bormashenko E, Balter S, Pogreb R, et al. Single-step technique allowing formation of microscaled thermally stable polymer honeycomb reliefs demonstrating reversible wettability. Polym Adv Technol. 2010;22:94–98.
- Jiang X. Surfactant-induced formation of honeycomb pattern on micropipette with curvature gradient. Langmuir. 2011;27:5410–5419.
- Cong H, Wang J, Yu B, et al. Preparation of a highly permeable ordered porous microfiltration membrane of brominated poly(phenylene oxide) on an ice substrate by the breath figure method. Soft Matter. 2012;8:8835.
- Bormashenko E, Balter S, Aurbach D. On the nature of the breath figures self-assembly in evaporated polymer solutions: revisiting physical factors governing the patterning. Macromol Chem Phys. 2012;213:1742–1747.
- Zheng Y, Kubowaki Y, Kashiwagi M, et al. Process optimization of preparing honeycomb-patterned polystyrene films by breath figure method. J Mech Sci Technol. 2011;25:33–36.
- Yabu H, Shimomura M. Single-step fabrication of transparent superhydrophobic porous polymer films. Chem Mater. 2005;17:5231–5234.
- Karthaus O. Water-assisted formation of micrometer-size honeycomb patterns of polymers. Langmuir. 2000;16:6071–6076.
- Ma H, Tian Y, Wang X. In situ optical microscopy observation of the growth and rearrangement behavior of surface holes in the breath figure process. Polymer. 2011;52:489–496.
- Yabu H, Hirai Y, Shimomura M, et al. Effects of hypergravity on formation of microporous films by dissipative processes. Jpn J Appl Phys. 2010;49.
- Yamazaki H, Ito K, Yabu H, et al. Formation and control of line defects caused by tectonics of water droplet arrays during self-organized honeycomb-patterned polymer film formation. Soft Matter. 2014;10:2741–2747.
- Hirai Y, Yabu H, Shimomura M. Preparation of metal microdots and microdiscs by using honeycomb template prepared by self organization. Macromol Symp. 2008;267:95–99.
- Yabu H. Spontaneous formation of microwrinkles on metal microdot arrays by shrinkage of thermal shrinkable substrate. ACS Appl Mater Interfaces. 2010;2:23–27.
- Yabu H, Hirai Y, Shimomura M. Electroless plating of honeycomb and pincushion polymer films prepared by self-organization. Langmuir. 2006;22:9760–9764.
- Ishii D, Yabu H, Shimomura M. Selective metal deposition in hydrophobic porous cavities of self-organized honeycomb-patterned polymer films by all-wet electroless plating. Colloids Surf A Physicochem Eng Aspects. 2008;313–314:590–594.
- Nakanishi T, Hirai Y, Kojima M, et al. Patterned metallic honeycomb films prepared by photo-patterning and electroless plating. J Mater Chem. 2010;20:6741.
- Connal LA, Franks GV, Qiao GG. Photochromic, metal-absorbing honeycomb structures. Langmuir. 2010;26:10397–10400.
- Hirai Y, Yabu H, Shimomura M. Electroless deposition of zinc oxide on pincushion films prepared by self-organization. Colloids Surf A Physicochem Eng Aspects. 2008;313–314:312–315.
- Kon K, Brauer CN, Hidaka K, et al. Preparation of patterned zinc oxide films by breath figure templating. Langmuir. 2010;26:12173–12176.
- Li L, Zhong Y, Ma C, et al. Honeycomb-patterned hybrid films and their template applications via a tunable amphiphilic block polymer/inorganic precursor system. Chem Mater. 2009;21(20):4977–4983.
- Kon K, Nakajima K, Karthaus O. polymer honeycomb templated microporous Tio2 films with enhanced photocatalytic activity. e-J Surf Sci Nanotechnol. 2008;6:161–163.
- Charrault E, He M, Muller P, et al. Route to homogeneous high density networks of metal nanoparticles. Langmuir. 2009;25(19):11285.
- Kataoka. S, Takeuchi Y, Endo A. Nanometer-sized domains in Langmuir−Blodgett films for patterning SiO2. Langmuir. 2010;26(9):6161.
- Yabu H, Shimomura M. Simple fabrication of micro lens arrays. Langmuir. 2005;21:1709–1711.
- Bolognesi A. Self-organization of polystyrenes into ordered microstructured films and their replication by soft lithography. Langmuir. 2005;21:3480–3485.
- Haupt M. Breath figures: self-organizing masks for the fabrication of photonic crystals and dichroic filters. J Appl Phys. 2004;96:3065.
- Hirai Y, Yabu H, Matsuo Y, et al. Biomimetic bi-functional silicon nanospike-array structures prepared by using self-organized honeycomb templates and reactive ion etching. J Mater. 2010;20:10804–10808.
- Li L. Breath figure lithography: a facile and versatile method for micropatterning. J Colloid Interface Sci. 2010;342:192–197.
- Saito Y, Yabu H. Bio-inspired low frictional surfaces having micro-dimple arrays prepared with honeycomb patterned porous films as wet etching masks. Langmuir. 2015;31:959–963.
- Saito Y, Kawano T, Shimomura M, et al. Fabrication of mussel-inspired highly adhesive honeycomb films containing catechol groups and their applications for substrate-independent porous templates. Macromol Rapid Commun. 2013;34:630–634.
- Yabu H, Saito Y, Nakamichi Y, et al. Self-assembled porous templates allow pattern transfer to poly(dimethyl siloxane) sheets through surface wrinkling. Polym J. 2012;44:573–578.
- Nishikawa T, Nonomura M, Arai K, et al. Micropatterns based on deformation of a viscoelastic honeycomb mesh. Langmuir. 2003;19:6193–6201.
- Yabu H. Preparation of highly oriented nano-pit arrays by thermal shrinking of honeycomb-patterned polymer films. Adv Mater. 2008;20(21):4200–4204.
- Yabu H, Shimomura M. Mesoscale pincushions microrings, and microdots prepared by heating and peeling of self-organized honeycomb-patterned films deposited on a solid substrate. Langmuir. 2006;22:4992–4997.
- Yabu H, Kojima M, Tsubouchi M, et al. Fabrication of photo-cross linked honeycomb-patterned films. Colloids Surf A Physicochem Eng Aspects. 2006;284–285:254–256.
- Kojima M, Yabu H, Shimomura M. Preparation of microporous and microdot structures from photo-crosslinkable resin by self-organization. Colloids Surf A Physicochem Eng Aspects. 2008;313–314:343–346.
- Karikari AS, Williams SR, Heisey CL, et al. Porous thin films based on photo-cross-linked star-shaped poly(d, l-lactide)s. Langmuir. 2006;22:9687–9693.
- Kabuto T, Hashimoto Y, Karthaus O. Thermally stable and solvent resistant mesoporous honeycomb films from a crosslinkable polymer. Adv Funct Mater. 2007;17:3569–3573.
- Karthaus O, Hashimoto Y, Kon K, et al. Solvent resistant honeycomb films from photo-crosslinkable polycinnamate. Macromol Rapid Commun. 2007;28:962–965.
- Nakamichi Y, Hirai Y, Yabu H, et al. Fabrication of patterned and anisotropic porous films based on photo-cross-linking of poly(1,2-butadiene) honeycomb films. J Mater. 2011;21(11):3884–3889.
- Yabu H, Nakamichi Y, Hirai Y, et al. Robust anisotropic polymer meshes prepared by stretching and photo-crosslinking of poly(1,2-butadiene) honeycomb films. Phys Chem Chem Phys. 2011;13:4877.
- Yabu H, Inoue K, Shimomura M. Multiple-periodic structures of self-organized honeycomb-patterned films and polymer nanoparticles hybrids. Colloids Surf A Physicochem Eng Aspects. 2006;284–285:301–304.
- Ke -B-B, Wan L-S, Li Y, et al. Selective layer-by-layer self-assembly on patterned porous films modulated by Cassie–Wenzel transition. Phys Chem Chem Phys. 2011;13:4881.
- Ke -B-B, Wan L-S, Chen P-C, et al. Tunable assembly of nanoparticles on patterned porous film. Langmuir. 2010;26:15982–15988.
- Nishida J. Preparation of self-organized micro-patterned polymer films having cell adhesive ligands. Polym J. 2002;34:166–174.
- Min EH, Wong KH, Stenzel MH. Microwells with patterned proteins by a self assembly process using honeycomb structured porous films. Adv Mater. 2008. doi:10.1002/adma.200800569
- Ke -B-B, Wan L-S, Xu Z-K. Controllable construction of carbohydrate microarrays by site-directed grafting on self-organized porous films. Langmuir. 2010;26:8946–8952.
- Nyström D. Biomimetic surface modification of honeycomb films via a ‘Grafting From’ approach. Langmuir. 2010;26:12748–12754.
- Xu WZ, Zhang X, Kadla JF. Design of functionalized cellulosic honeycomb films: site-specific biomolecule modification via ‘click chemistry’. Biomacromolecules. 2012;13:350–357.
- Chen P-C, Wan L-S, Ke -B-B, et al. Honeycomb-patterned film segregated with phenylboronic acid for glucose sensing. Langmuir. 2011;27:12597–12605.
- Karthaus O, Okamoto T, Okajima C. Preparation of electrically conducting self-organized micro-hybrid structures. e-J Surf Sci Nanotechnol. 2015;13:19–22.
- Kurono N, Shimada R, Ishihara T, et al. Fabrication and optical property of self-organized honeycomb-patterned films. Mol Cryst Liquid Cryst. 2002;377:285–288.
- Yabu H, Hirai Y, Matsuo Y, et al. Double layered metal mesh film with limited viewing angle prepared by electroless plating of self organized honeycomb film. Macromol Symp. 2008;267:100–104.
- Hirai Y, Yabu H, Matsuo Y, et al. Biomimetic bi-functional silicon nanospike-array structures prepared by using self-organized honeycomb templates and reactive ion etching. J Mater Chem. 2010;20:10804–10808.
- Hirai Y, Yabu H, Matsuo Y, et al. Arrays of triangular shaped pincushions for SERS substrates prepared by using self-organization and vapor deposition. Chem Commun. 2010;46:2298.
- Galeotti F, Mróz W, Scavia G, et al. Microlens arrays for light extraction enhancement in organic light-emitting diodes: a facile approach. Org Electron. 2013;14:212–218.
- Chari K, Lander CW, Wang JS, et al. P-175: enhanced outcoupling of light from organic light emitting diodes by microlens arrays based on breath figure template. SID Symp Dig Tech Pap. 2007;38:852–855.
- Koch K, Bhushan B, Jung YC, et al. Fabrication of artificial lotus leaves and significance of hierarchical structure for superhydrophobicity and low adhesion. Soft Matter. 2009;5:1386–1393.
- Yabu H, Takebayashi M, Tanaka M, et al. Superhydrophobic and lipophobic properties of self-organized honeycomb and pincushion structures. Langmuir. 2005;21:3235–3237.
- Ishii D, Yabu H, Shimomura M. Novel biomimetic surface based on a self-organized metal−polymer hybrid structure. Chem Mater. 2009;21:1799–1801.
- Ishii D, Shimomura M. Invisible gates for moving water droplets: adhesive force gradients on a biomimetic superhydrophobic surface. Chem Mater. 2013;25:509–513.
- Yabu H, Hirai Y, Kojima M, et al. Simple fabrication of honeycomb- and pincushion-structured films containing thermoresponsive polymers and their surface wettability. Chem Mater. 2009;21:1787–1789.
- Kamei J, Saito Y, Yabu H. Biomimetic ultra-bubble-repellent surfaces based on a self-organized honeycomb film. Langmuir. 2014;30:14118–14122.
- Kamei J, Yabu H. On-demand liquid transportation using bioinspired omniphobic lubricated surfaces based on self-organized honeycomb and pincushion films. Adv Funct Mater. 2015;25:4195–4201.
- Lee B-H. Direct observation of a carbon filament in water-resistant organic memory. ACS Nano. 2015;9(7):7306–7313.
- Guvendiren M, Burdick JA. Biomaterials. Biomaterials. 2010;31:6511–6518.
- Dalby MJ, Gadegaard N, Oreffo ROC. Harnessing nanotopography and integrin-matrix interactions to influence stem cell fate. Nat Mater. 2014;13:558–569.
- Yamamoto S. Effect of honeycomb-patterned surface topography on the adhesion and signal transduction of porcine aortic endothelial cells. Langmuir. 2007;23:8114–8120.
- Sato T. Effect of honeycomb-patterned surface topography on the function of mesenteric adipocytes. J Biomater Sci Polym Ed. 2010;21:1947–1956.
- Tanaka M. Control of hepatocyte adhesion and function on self-organized honeycomb-patterned polymer film. Colloids Surf A Physicochem Eng Aspects. 2006;284–285:464–469.
- Fukuhira Y. Effect of honeycomb-patterned structure on chondrocyte behavior in vitro. Colloids Surf A Physicochem Eng Aspects. 2008;313–314:520–525.
- Arai K, Tanaka M, Yamamoto S, et al. Effect of pore size of honeycomb films on the morphology, adhesion and cytoskeletal organization of cardiac myocytes. Colloids Surf A Physicochem Eng Aspects. 2008;313–314:530–535.
- Birch MA, Tanaka M, Kirmizidis G, et al. Microporous ‘Honeycomb’ films support enhanced bone formation in vitro. Tissue Eng. 2013;19:2087–2096.
- Choi H, Tanaka M, Hiragun T, et al. Non-tumor mast cells cultured in vitro on a honeycomb-like structured film proliferate with multinucleated formation. Nanomed Nanotechnol Biol Med. 2014;10:313–319.
- Yamazaki H. Micropatterned culture of HepG2 spheroids using microwell chip with honeycomb-patterned polymer film. J Biosci Bioeng. 2014;118:455–460.
- Duan S. Osteocompatibility evaluation of poly(glycine ethyl ester- co-alanine ethyl ester)phosphazene with honeycomb-patterned surface topography. J Biomed Mater Res. 2012;101A:307–317.
- Tsukiyama S. Enhanced cell survival and yield of rat small hepatocytes by honeycomb-patterned films. Jpn J Appl Phys. 2008;47:1429–1434.
- Tsuruma A, Tanaka M, Yamamoto S, et al. Control of neural stem cell differentiation on honeycomb films. Colloids Surf A Physicochem Eng Aspects. 2008;313–314:536–540.
- Kawano T, Sato M, Yabu H, et al. Honeycomb-shaped surface topography induces differentiation of human mesenchymal stem cells (hMSCs): uniform porous polymer scaffolds prepared by the breath figure technique. Biomater Sci. 2013;2:52.
- Fukuhira Y, Kitazono E, Hayashi T, et al. Biodegradable honeycomb-patterned film composed of poly(lactic acid) and dioleoylphosphatidylethanolamine. Biomaterials. 2006;27:1797–1802.
- Nakamichi Y, Hirai Y, Yabu H, et al. Fabrication of patterned and anisotropic porous films based on photo-cross-linking of poly(1,2-butadiene) honeycomb films. J Mater Chem. 2011;21:3884.
- Kawano T. Mechanical regulation of cellular adhesion onto honeycomb-patterned porous scaffolds by altering the elasticity of material surfaces. Biomacromolecules. 2013;14:1208–1213.
- Uraki Y. Honeycomb-like architecture produced by living bacteria, Gluconacetobacter xylinus. Carbohydr Polym. 2007;69:1–6.
- Fukuhira Y. Prevention of postoperative adhesions by a novel honeycomb patterned poly(lactide) film in a rat experimental model. J Biomed Mater Res. 2008;86B:353–359.
- Kittel C. Thermal Physics. New York: Wiley; 1969.
- Uchida Y, Takanishi Y, Yamamoto J. Controlled fabrication and photonic structure of cholesteric liquid crystalline shells. Adv Mater. 2013;25:3234–3237.
- Iwanaga H, Shiratsuchi K. Fabrication and application of honeycomb film. Fujifilm Res Dev. 2009;54:43–47.
- Ding J, Zhang A, Bai H, et al. Breath figure in non-aqueous vapor. Soft Matter. 2013;9:506–514.
- Kamei J, Yabu H. One step fabrication of mesh-reinforced hierarchic perforated microporous honeycomb films with tunable filtering property. Soft Matter. 2017;13(43):7834–7839.