ABSTRACT
The young history of sputtering onto a liquid features great achievements in the green production of various metal and metal oxide nanoparticles (NPs) and nanoclusters (NCs). Studies on how the sputtering parameters affect the properties of NPs and NCs have elucidated their formation mechanism and marked a crucial role of liquid matrix in the nucleation and growth of particles, controlling their various properties. Current research has been devoted to making alloy bi- and trimetallic NPs with a high level of control over the NP structure, composition, and size via well-designed target systems, sputtering steps, and liquid matrix materials. In this minireview, we discuss the recent advances in the use of various types of targets to prepare different bi- and trimetallic NPs. In single target sputtering, systems with alternative configurations of metals, alloy targets, monometallic targets in sequence, and a combination of sputtering and chemical reactions have been developed. On the other hand, a double head system was introduced to widen the range of controllable sputtering parameters yielding more versatility in particle composition and fine structure. The synergistic and tuneable properties exhibited by the multi-metallic components in small NPs and NCs for their use in catalysis and optical applications are discussed.
GRAPHICAL ABSTRACT
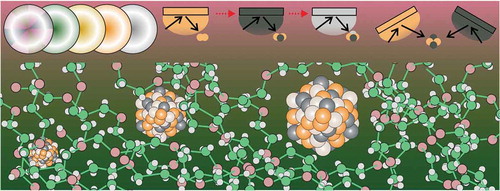
1. Brief history and background of sputtering onto a liquid
Vacuum sputtering is a well-known top-down technique to fabricate thin films based on the bombardment of a target with energetic gas ions formed using Ar, O2, N2 or H2, etc. [Citation1–Citation4]. The bombardment results in the ejection of atoms or clusters from the target, which can then deposit onto a substrate inserted into the vacuum sputtering chamber. The energetic gas ions are generated via the collision of electrons and carrier gas in vacuum using direct current (DC), radio frequency (RF), or magnetron sputtering [Citation4]. The sputtering technique, therefore, is widely used for thin film deposition, surface coating, and surface etching applications [Citation3,Citation4]. As a conventional approach for producing thin film and multiple layers of metals, metal alloys, and metal oxides the method still received significant studies until now [Citation5–Citation16]. To some extent, the technique has been also used for immobilizing nanoparticles (NPs) on a solid substrate [Citation17–Citation22], or decorating nanoparticles on other metal/oxides nano-micron particles as the supporters [Citation23–Citation33]. In order to have NPs instead of big aggregate or thin film very short sputtering time, that is in order of few to several tens of second [Citation23,Citation27], or low pressure [Citation24,Citation25,Citation27] or post heat-treatment [Citation29,Citation30] has been used. The NPs can be sandwiched in two solid layer via alternative sputtering of two or more targets to prevent their growth and attain larger numbers of nanoparticles dispersed in solid [Citation34]. So far with the use of a solid substrate, it restricts the further processing of nanoparticles in a form of liquid dispersion as well as the size that the particles were produced. In 1996, Ye et al. published the very first study of a sputtering process using a liquid as the substrate [Citation35]. They used silicone oil as the liquid substrate in vacuum sputtering to investigate the silver film formed on the liquid. This, however, serves as the first step for a very new research field wherein a low vapour pressure liquid is typically used to capture the nanoclusters (NCs) produced by the technique, resulting in the growth of nanoparticles (NPs) in the liquid (). This in turn has opened up great opportunities for chemists and materials scientists to work with different liquid substrates and tailor their physicochemical properties for obtaining high-quality NPs and NCs with advanced properties. This research field has become highly interdisciplinary with the combination of a physical top-down technique to produce highly pure NCs and chemical methods to capture, stabilize, control the size of, and surface functionalize NPs in the liquid medium, such as processing with a synthesis in a colloidal solution [Citation36–Citation38]. Thus, the succeeding phenomena and results obtained from such combinations are highly interesting. Moreover, because there is no necessity of using toxic reducing agents and organic solvents, the method can be considered as a green approach to generate NPs/NCs in liquids.
In 1999, Wagener and Günther obtained magnetic Fe and Ni NPs of 9 nm and Ag NPs of 5–15 nm by magnetron sputtering metal targets onto a running liquid, i.e., silicone oil [Citation39]. Later on, it was recognized that the NPs dispersed in silicone oil exhibited low colloidal stability, which was attributed to the fact that silicone lacks a functional group to strongly anchor the particle surfaces and stabilize the NPs [Citation40]. The high viscosity of the liquid allows NPs to be dispersed in it for a long time before collisions and aggregation occur. Hence, the nature of the liquid is an important factor for stabilizing the obtained NPs. In 2006, Torimoto et al. introduced an ionic liquid (IL) for the first time as the substrate in vacuum sputtering [Citation41]. An IL is composed of poorly coordinated ions, where one ion has delocalized charge and one is organic, which allows it to stay in liquid form without forming a stable crystal lattice [Citation42–Citation44]. ILs are advantageous because they remain in a liquid state at sufficiently low pressures without evaporation, creating an electrostatic shell around the produced NPs to stabilize them. As a result, Torimoto et al. and other research groups successfully produced noble metal NPs, i.e., Au, Ag, Pd, Pt, with high colloidal stability [Citation41,Citation45–Citation47]. Later, the effects of the sputtering conditions and the properties of the ionic liquids on the NPs formed were further investigated [Citation48–Citation52]. These findings shed light on the formation of NPs in this process. The surface tension and IL composition affect particle growth on the liquid surface, while the viscosity and temperature of the IL regulate particle collision and further agglomeration and growth [Citation52]. Vanecht et al. demonstrated the diffusion limit growth of NPs after sputtering based on the observation that the particle size is as a function of the viscosity of various ILs placed in different cavities under identical sputter conditions wherein less viscous ILs allowed fast growth of particle [Citation52]. The growth was stopped when particles reached a certain size and were stabilized with a surface liquid layer. This was in good agreement with the step-by-step nucleation and growth of Au NPs via reduction in an IL in which addition of Au precursor into the IL containing excessive reducing agent resulted in the stepwise growth of Au NPs [Citation53]. Based on the temperature-dependent viscosity of ILs, stopping the aggregation and growth of Ag NPs after sputtering onto an IL was achieved using low temperature storage, that is below glass transition temperature of IL [Citation54]. The anion and cation effect and the stabilization capability of ionic liquid for NPs have been also investigated and discussed elsewhere [Citation55–Citation57]. On the other hand, liquid monomers, which can be polymerized in the succeeding step, or liquid polymers were then used as a capture medium in sputtering [Citation58–Citation63]. This resulted in a composite of NPs embedded in a polymer matrix, which functions as a solid material [Citation58,Citation59,Citation62]. In this field, research groups led by Hatakeyama and Yonezawa have pioneered practical applications of the resulting materials in optics. In comparison with IL, that is 1-butyl-3-methylimidazolium hexafluorophosphate, [BMI][BF4], Nishikawa group observed similar particle size and size variation depended on liquid viscosity (temperature) when polyethylene glycol (PEG) was used [Citation60]. This reconfirmed that one of the most important factors regulating the NP growth is the collision frequency determined by the diffusion velocity which in turn was determined by the liquid viscosity. However, the authors also recognized that the size and size variation of Au NPs produced in PEG were larger than that in [BMI][BF4] though PEG is more viscous than the IL [Citation60]. Moreover, the NPs in PEG experienced further aggregation and growth in a week after sputtering. This led them to deduce that the stabilization capability of PEG via weak coordination of O and metal surface and alkyl backbone stabilizer for the sputtered NPs is less than that of the IL. Molten salt [Citation64], vegetable oil [Citation65,Citation66], biomolecules [Citation67], fatty acids [Citation68], multifunctional group ligand-containing liquids [Citation69,Citation70], polyol [Citation71] and others have been examined as candidates for liquid sputtering to offer different surface functionality for the particles and to understand the influence of the liquid medium on particle growth. Recently, there has been great progress in particle size control on a scale from sub-nano to a few nanometres via the addition of thiol functionalized protecting agents to the liquid matrix [Citation72–Citation77]. Consequently, fluorescent metal NCs and NCs hybridized in polymer resins were obtained. Readers interested in these hot topics are recommended to refer to some recent publications and reviews [Citation78,Citation79]. Some liquids, ligands and supporters used in sputtering onto liquids are showed in .
Currently, alloy formation has been observed at the nanoscale regime even for immiscible bi- and trimetallic systems [Citation80–Citation88]. As bi-, tri-, and multi-elemental metallic alloys offer new properties or tuneable characteristics due to the presence and interaction of different elements, synthesizing alloy NPs has become a new tool for NP design [Citation80–Citation89]. It has been demonstrated that synthetic Pd-Ru alloy nanoparticles exhibited pseudo Rh catalytic activity [Citation80], CuNi alloy showed efficient photocatalytic activity for H2 generation as compared with conventional Pt catalyst [Citation32], and synergistic catalytic performance was widely observed for alloy catalysts [Citation17,Citation18,Citation23–Citation26,Citation31,Citation32]. In chemical synthesis, the order of generating metal atoms is highly dependent on the reduction potentials of metal salts or decomposition temperatures of the metal complexes [Citation80,Citation81,Citation83–Citation88,Citation90,Citation91]. Plasma-in-liquid process can also be used to reduce metal ions to generate metal atoms [Citation87,Citation88]. Thus, it is challenging to create conditions favourable for co-reduction and alloying rather than for heterogeneous structure formation. Sputtering techniques, on the other hand, can produce atoms or clusters of any metal at once by the physical bombardment of energetic gas ions onto metal targets [Citation17–Citation33]. This can allow for the production of alloy NPs of various bimetallic systems. In this mini-review, we will discuss the bi- and trimetallic alloy NPs obtained via sputtering onto different liquid matrices by using single target sputtering and by co-sputtering, as well as their structures, properties, and applications ().
2. Multi-metallic NPs by single target sputtering
2.1. Direct sputtering of binary metal targets
In 2008, Torimoto et al. reported the use of an IL, [BMI][PF6], as the liquid substrate in sputtering to produce Au/Ag alloy NPs for the first time [Citation92]. In this study, a fan-shaped Au/Ag foil binary target was sputtered at a sputtering current of 40 mA for 5 min under an Ar pressure of 20 Pa. The surface fraction of the two metals in this configuration was determined by the ratio of the two metal elements in the target, thus enabling the preparation of bimetallic alloy NPs with different compositions. The surface plasmon resonance peak position of the obtained bimetallic NPs increased linearly with increasing Au content. This is evidence of alloy formation, as Au/Ag alloy NPs exhibit a compositionally dependent plasmon peak position, which is also observed for chemically synthesized Au/Ag alloy NPs [Citation93,Citation94].
Subsequently, they expanded on the method, using a similar target set-up to produce Au/Pt (in 2012, ) [Citation95], Au/Pd (in 2013) [Citation96], and Au/Cu (in 2016) alloy NPs [Citation97] via sputtering onto ILs. The obtained NPs exhibited enhanced oxidation catalytic properties compared with single metallic NPs prepared in a similar way. An important finding of these studies is the ability of the method to create bimetallic alloy NPs at room temperature for various bimetallic systems, including immiscible bimetallic systems such as Au/Pt or intermetallic systems such as Au/Cu. This shows the advantage of sputtering onto ILs to produce alloy NPs, since the formation of these alloys by chemical synthesis via co-reduction of metal salts at elevated temperature is thermodynamically unfavourable.
Figure 1. Vacuum sputtering onto different liquids to produce metal NPs/NCs. The design of target and the sputter sequence are used for obtaining bimetallic/trimetallic NPs/NCs with desired fine structures. The liquids and solid additives/ligands, for example MUTAB, allow for trapping and stabilizing NPs/NCs in the liquids.
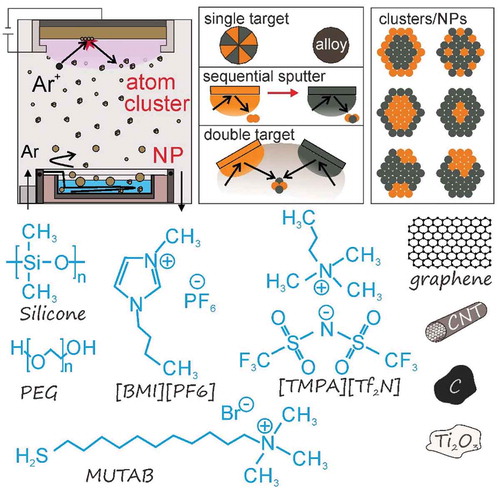
Figure 2. (a) Schematic illustrations of Au/Pt foil binary targets having various f Au, f Au = AAu/(AAu + APt), where AAu and APt are total areas of Au and Ag parts exposed on the targets, respectively. (b) Relationship between the proportion of Au (%) in NPs obtained by sputter deposition on IL and f Au of the binary targets. Reproduced with permission from Ref. [Citation95], copyright 2012 The Royal Society of Chemistry.
![Figure 2. (a) Schematic illustrations of Au/Pt foil binary targets having various f Au, f Au = AAu/(AAu + APt), where AAu and APt are total areas of Au and Ag parts exposed on the targets, respectively. (b) Relationship between the proportion of Au (%) in NPs obtained by sputter deposition on IL and f Au of the binary targets. Reproduced with permission from Ref. [Citation95], copyright 2012 The Royal Society of Chemistry.](/cms/asset/111a2235-8e56-4e7f-93ae-630221f3a9c8/tsta_a_1542926_f0002_b.gif)
2.2. Monometallic target sputtering combined with chemical reduction
In 2009, a combination of sputtering and galvanic reaction was demonstrated to be capable of creating bimetallic alloy NPs [Citation98]. In particular, a less noble metal, that is Ag, was sputtered onto the IL [BMI][PF6] containing a metal salt of a more noble metal, that is HAuCl4. Consequently, sputtered silver atoms/clusters and/or NPs acted as reducing agents to reduce gold ions via a galvanic reaction. The subsequent alloying of Au and Ag occurred and resulted in Au/Ag alloy NPs of similar sizes, ca. 6.4–6.9 nm (). Therefore, the surface plasmon resonance of Au/Ag alloy NPs was tuneable based on the alloy composition, which in turn was manipulated simply by varying the concentration of HAuCl4 in the IL (). Because the galvanic reaction is general for any pair of metals with different reactivities, this approach can be applied to various bimetallic systems. Besides, sputtering Au onto a cubic Ag NPs allowed for the formation of a cubic Au/Ag composite. The Au/Ag composite formed in the IL was useful as an intermediate template for creating Au NPs of desired morphologies, such as nano-frames via selective chemical etching of Ag () [Citation99]. Furthermore, core@shell Au@Pt NPs were prepared via the sputtering of Au NPs onto an IL, then impregnating the Au NPs with carbon black and finally chemically reducing a Pt salt on the Au NPs [Citation100]. This indicated that in combination with a chemical method, sputter deposition onto ILs has become a versatile way of building up interesting nanostructures.
Figure 3. Au/Ag NPs via vacuum sputtering in combination with chemical reduction: UV-Vis spectra of Au/Ag NPs with different concentration of HAuCl4 in IL 0 (a), 50 (b), 65 (c), 100 (d) µmol dm−3. Inset is the relation between UV-Vis peak position and concentration of HAuCl4. TEM images (e) and (f) show Au/Ag NPs with UV-Vis spectra in (c) and (d) respectively. (g) Au/Ag composite prepared in sputtering and used as intermediate for controlling structure of Au nanoframe. SAM stands for self-assembled monolayer, ODT for 1-octadecanethiol and AMP for 4-amino-2-mercaptopyrimidine. Reproduced with permission from (a-f) Ref. [Citation98], copyright 2009 The Electrochemical Society of Japan, and (g) Ref. [Citation99], copyright 2011 The Chemical Society of Japan.
![Figure 3. Au/Ag NPs via vacuum sputtering in combination with chemical reduction: UV-Vis spectra of Au/Ag NPs with different concentration of HAuCl4 in IL 0 (a), 50 (b), 65 (c), 100 (d) µmol dm−3. Inset is the relation between UV-Vis peak position and concentration of HAuCl4. TEM images (e) and (f) show Au/Ag NPs with UV-Vis spectra in (c) and (d) respectively. (g) Au/Ag composite prepared in sputtering and used as intermediate for controlling structure of Au nanoframe. SAM stands for self-assembled monolayer, ODT for 1-octadecanethiol and AMP for 4-amino-2-mercaptopyrimidine. Reproduced with permission from (a-f) Ref. [Citation98], copyright 2009 The Electrochemical Society of Japan, and (g) Ref. [Citation99], copyright 2011 The Chemical Society of Japan.](/cms/asset/173ca261-7354-4632-9a3b-71b6626b1067/tsta_a_1542926_f0003_b.gif)
2.3. Sequential sputtering with monometallic targets
Sputter deposition onto IL surfaces was further developed with sequential sputtering to form either bimetallic alloy NPs or a monolayer of NPs with a metal core coated with an alloy shell structure [Citation101–Citation106]. Wang et al. first sputtered Ag for certain time and subsequently sputtered a second metal target such as Au and Pd onto [BMI][BF4] (1-butyl-methylimidazolium tetrafluoroborate) containing graphene [Citation101]. The resulting NPs have a core@shell structure, of which the core is Ag and the shell composes of small sized Au NPs with various crystallographic orientation and local alloying of Ag and Au at the interface. The authors also reported similar results for Ag@Pd core@shell NPs. It can be observed in the transmission electron microscopy (TEM) and high-resolution TEM (HR-TEM) images () that some monometallic Pd NPs coexisted with the core@shell NPs meanwhile the particle sizes and fine control of the shell structure, thickness, and smoothness have not been completely achieved. Using the same approach, in 2015, the group claimed that instead of a core@shell structure they obtained Pd/Au alloy NPs with tuneable composition on graphene by sputtering a Pd target followed by sputtering an Au target onto a graphene/[BMI][BF4] suspension [Citation102]. The obtained crystalline structure, high angle annular dark field scanning transmission electron microscopy (HAADF-STEM) line profile, and electronic structure suggest the alloying of Au and Pd after sequential sputtering ()). In particular, they found evidence of electron transfer from Pd to Au in X-ray photoelectron spectroscopy (XPS) and X-ray absorption near edge structure (XANES) spectra, the presence of both elemental component in single NPs with HAADF-STEM line profiles, and the shift of X-ray diffraction (XRD) peaks of bimetallic NPs between that of the two monometallic ones. Extended X-ray absorption fine structure (EXAFS) measurements revealed that Au/Pd NPs were non-homogeneous alloy based on comparing the ratios of the number of Pd and Au atoms near an Au atom with Pd:Au composition [Citation106]. The composition of the obtained alloy NPs was tailored by varying the sputtering time in each step [Citation102]. By introducing graphene into the liquid used for sputtering, the authors were able to effect the separation and purification of the NPs from the liquid matrix and the immobilization of active NPs on the catalytic supports [Citation101,Citation102]. As a result, they successfully hybridized Pd/Au NPs on graphene, which exhibited synergistic catalytic activity for both the reduction of 4-nitrophenol and the oxidation of cis-cyclooctene [Citation102]. The group also demonstrated that a similar approach, where the sputtering order of the Au and Pd targets is reversed, can be used to produce TiO2-supported Au/Pd bimetallic NPs [Citation103]. Only partial alloying likely occurred, since a sharp feature of Au was present in the XRD patterns of the Au/Pd bimetallic NPs. Thus, in sequential sputtering, it is easy to vary the particle composition, whereas the sputtering order and choice of the metal couples can influence the structure of the final bimetallic NPs.
Figure 4. Sequential sputtering of metal targets A and B onto an ionic liquid containing support materials, i.e., graphene to produce bimetallic NPs. The resulting NPs with Ag core and Au or Pd shell are shown in (a–f). TEM images (a–c) of graphene supported Ag@Au core@shell NPs. The lattice spacing shown in (c) corresponds to (111) of Au and Ag. TEM images (d,e) and HR-TEM image (f) of Ag@Pd core@shell NPs. The presence of both Ag and Pd on the shell indicates the local alloying of Ag and Pd. Reproduced with permission from Ref. [Citation101], copyright 2013 The American Chemical Society.
![Figure 4. Sequential sputtering of metal targets A and B onto an ionic liquid containing support materials, i.e., graphene to produce bimetallic NPs. The resulting NPs with Ag core and Au or Pd shell are shown in (a–f). TEM images (a–c) of graphene supported Ag@Au core@shell NPs. The lattice spacing shown in (c) corresponds to (111) of Au and Ag. TEM images (d,e) and HR-TEM image (f) of Ag@Pd core@shell NPs. The presence of both Ag and Pd on the shell indicates the local alloying of Ag and Pd. Reproduced with permission from Ref. [Citation101], copyright 2013 The American Chemical Society.](/cms/asset/c38a1285-1cd1-42ca-82a7-f3dd655e653b/tsta_a_1542926_f0004_oc.jpg)
Figure 5. Graphene-supported Pd/Au alloy NPs by sequential sputtering of Pd followed by Au: (a) TEM image and EDX spectrum, (b) HR-TEM image, (c) HAADF and crossline profile, (d) UV-Vis spectra of Pd/Au with different Pd-to-Au ratios, and (e) bimetallic composition tuning of Pd/Au NPs. (f) Sequential sputtering of Au followed by Pt to form monolayer of Au core@Au/Pt alloy shell NPs functionalized on IL. Reproduced with permission of (a-e) Ref. [Citation102], copyright 2015 The Royal Society of Chemistry, and (f) Ref. [Citation104], copyright 2016 The American Chemical Society.
![Figure 5. Graphene-supported Pd/Au alloy NPs by sequential sputtering of Pd followed by Au: (a) TEM image and EDX spectrum, (b) HR-TEM image, (c) HAADF and crossline profile, (d) UV-Vis spectra of Pd/Au with different Pd-to-Au ratios, and (e) bimetallic composition tuning of Pd/Au NPs. (f) Sequential sputtering of Au followed by Pt to form monolayer of Au core@Au/Pt alloy shell NPs functionalized on IL. Reproduced with permission of (a-e) Ref. [Citation102], copyright 2015 The Royal Society of Chemistry, and (f) Ref. [Citation104], copyright 2016 The American Chemical Society.](/cms/asset/569c5f66-afd6-43d6-9def-813579bdd139/tsta_a_1542926_f0005_oc.jpg)
In 2016, Torimoto’s group developed a sequential sputtering process of Au and Pt for tailoring NPs with a well-defined metal core@metal alloy shell structure as well as assembling them into a monolayer ()) [Citation104]. They chose an IL, 1-(2-hydroxyethyl)-3-methylimidazolium tetrafluoroborate [HyEMI][BF4], containing hydroxyl functionalized cations to achieve a monolayer of 4.2 nm Au NPs on the liquid surface by first sputtering an Au target. The monolayer of Au NPs was formed via selective nucleation and growth of Au clusters trapped by hydroxyl groups on the surface of the IL. Subsequently, they sputtered Pt onto the Au NP monolayer for 2 h. This resulted in NPs composed of an Au core coated with an Au/Pt alloy shell structure, whereas any single metallic Pt particles were separated out by falling down into the IL via the gaps between Au NPs. In addition, the method allowed for control over the Pt content (0–44 at% Pt) and particle size (4.2–4.8 nm) by changing the sputtering time of the Pt target. Though the formation mechanism of the Au/Pt alloy shell has not been fully understood, the presence of the Au/Pt alloy shell was confirmed with XRD, energy dispersive X-ray spectroscopy (EDX) line profile, and XPS analyses, whereas Pt rich shells or non-homogeneous alloy layers were present in the sputtered NPs.
2.4. Sputtering using an alloy target
As another approach, Wang et al. used a bimetallic alloy target instead of a fan shaped binary target to create alloy NPs via sputtering onto an IL [Citation107–Citation109]. They reported that Au/Pd alloy NPs produced by sputtering an Au/Pd alloy target onto [BMI][BF4] containing multi-walled carbon nanotubes (CNTs) [Citation107] and graphene [Citation108]. They observed Au/Pd NPs of ca. 2.1–2.6 nm decorated on graphene, which can be separated from the liquid matrix by means of centrifugation. Alloy targets with various compositions of Au and Pd were sputtered to obtain Au/Pd NPs with different compositions (). Similarly, the group studied Pt/Ni alloy NPs on multi-walled CNTs produced by sputtering a Pt/Ni alloy target onto an IL, that is [BMI][BF4] [Citation109]. The results were promising for applications in catalysis. Compared with using an alloy target, a fan-shape binary target and sequential sputtering were advantageous and versatile for finely tailoring particle composition, for example via varying the surface area of different metal sectors on the target or the sputtering time of each step, respectively. Further, it was noticed that in immiscible bi- and trimetallic systems, an alloy target of the desired composition may not be available for sputtering of bi- and trimetallic NPs.
Figure 6. HAADF-STEM images and profiles of elemental composition for graphene-supported AuPd alloy NPs prepared using single targets and different Au:Pd ratios: (a) for 3:1; (b) for 1:1; and (c) for 1:3. Reprinted with permission from Ref. [Citation108], copyright Elsevier 2016.
![Figure 6. HAADF-STEM images and profiles of elemental composition for graphene-supported AuPd alloy NPs prepared using single targets and different Au:Pd ratios: (a) for 3:1; (b) for 1:1; and (c) for 1:3. Reprinted with permission from Ref. [Citation108], copyright Elsevier 2016.](/cms/asset/28b0dc8e-b38b-4605-a53d-5152f5634f24/tsta_a_1542926_f0006_oc.jpg)
2.5. An alloy target in combination with sequential sputtering
In an effort to produce trimetallic NPs of an immiscible Au/Pt/Pd system, Wang et al. combined sequential sputtering of a PdAu alloy target and a Pt target onto an IL containing CNTs as shown in [Citation110]. They detected electron transfer from Au and/or Pd to Pt with XPS and XANES, while XRD peak shift and broadening and EDX mapping suggested that 3–4 nm of trimetallic PdAu/Pt alloys of several compositions were created. The formation of ternary noble metal alloy NPs on CNTs shows that sputtering has made great progress in achieving versatile control of the structure and composition of small-sized metal NPs for advanced applications.
Figure 7. (a) Sequential sputtering of PdAu alloy and Pt targets onto room-temperature (RT) IL, [BMI][BF4], containing a carbon nanotube (CNT) to form PdAu/Pt trimetallic NPs-decorated CNT, (b) the HAADF and elemental mapping of CNTs-supported PdAu/Pt trimetallic NPs, (c) Pt L 3 edge XANES spectra of CNTs-supported PdAu/Pt trimetallic NPs with different Pd/Au/Pt ratios, Au/Pt and Pd/Pt bimetallic NPs, and Pt NPs. Reproduced with permission from Ref. [Citation110], copyright 2017 Springer.
![Figure 7. (a) Sequential sputtering of PdAu alloy and Pt targets onto room-temperature (RT) IL, [BMI][BF4], containing a carbon nanotube (CNT) to form PdAu/Pt trimetallic NPs-decorated CNT, (b) the HAADF and elemental mapping of CNTs-supported PdAu/Pt trimetallic NPs, (c) Pt L 3 edge XANES spectra of CNTs-supported PdAu/Pt trimetallic NPs with different Pd/Au/Pt ratios, Au/Pt and Pd/Pt bimetallic NPs, and Pt NPs. Reproduced with permission from Ref. [Citation110], copyright 2017 Springer.](/cms/asset/422d2548-628b-42f1-9bab-b47b5fcfbe89/tsta_a_1542926_f0007_oc.jpg)
3. Multi-metallic NPs by using double-target sputtering
Most publications on bimetallic alloy NPs formed via sputtering onto a liquid are mainly concerned with a single alloy target or sequential sputtering. However, the alloy set-up requires fabricating different targets with different compositions in order to vary the composition of the resulting NPs while it is difficult for creating a complete alloy of any two metals based on sequential sputtering. Using a double head system, on the other hand, can help overcome these issues, for example alloying via co-sputtering and varying the sputter parameters to tailor particle composition [Citation111]. From a fundamental point of view, the combination of sputtered metal atoms/clusters from two targets allows for versatility in forming binary structures of NPs below several nanometres. Hence, this method can offer more room to create alloy/bimetal NPs of various compositions and fine structures.
In 2014, Ludwig et al. [Citation111] reported on binary alloy NPs obtained by double head sputtering onto an IL, that is 1-butyl-3-methylimidazolium bis(trifluoromethylsulfonyl)imide, [BMI][Tf2N]. In their experiment, two elemental Cu and Au targets were positioned opposite each other with an inclination angle of 28° to the substrate normal (). The substrate was an array of cavities containing the IL positioned below these targets in the sputtering chamber. As the size of the cavities is very small compared with the target distance, the composition of the particles in a cavity can be considered uniform. The use of two targets separated at a distance resulted in the formation of Au/Cu NPs in the cavities with location-dependent compositions, i.e., creating a linear composition gradient over the substrate’s so-called Au/Cu NP libraries. NPs formed with a wide range of alloy compositions from Au rich to equimolar Au/Cu to Cu rich, depending on the relative distance of the cavities with respect to the targets. This is an advantage of the method: the obtained alloy NPs form a library of tuneable compositions in a single synthesis batch. The resulting NPs were uniform with a narrow size distribution but exhibited compositionally dependent particle size, that is particle size increases with an increase in Cu content. In the report, the lattice constant of Au/Cu NPs calculated from XRD peak position, which was found to vary linearly with particle composition, indicated the formation of an Au/Cu solid solution.
Figure 8. Upper left: schematic (not to scale) of the combinatorial co-deposition from two sputter targets into a cavity array substrate filled with IL. Upper right: Schematic of the proposed formation process of NPs in IL. Lower left: photo of a 4-in cavity array substrate used for the fabrication of NP libraries synthesized by method described in the upper left Figure. The red dots mark the measurement points whereas the red arrow shows the direction in which the EDX line scan analysis was performed. Lower right: results of EDX screening for the four materials libraries. Adapted from Ref. [Citation111]. Copyright 2014 John Wiley & Sons Ltd.
![Figure 8. Upper left: schematic (not to scale) of the combinatorial co-deposition from two sputter targets into a cavity array substrate filled with IL. Upper right: Schematic of the proposed formation process of NPs in IL. Lower left: photo of a 4-in cavity array substrate used for the fabrication of NP libraries synthesized by method described in the upper left Figure. The red dots mark the measurement points whereas the red arrow shows the direction in which the EDX line scan analysis was performed. Lower right: results of EDX screening for the four materials libraries. Adapted from Ref. [Citation111]. Copyright 2014 John Wiley & Sons Ltd.](/cms/asset/5e07363b-c738-4a7a-a584-8d12dac54cbf/tsta_a_1542926_f0008_oc.jpg)
ILs are believed to have lower vapour pressure than mostly other non-ILs liquids having been used in sputtering whereas they can absorb and stabilize NPs via electrostatic repulsion and steric stabilization [Citation55–Citation57,Citation112–Citation114]. In this sense, ILs are favourable for sputtering under vacuum conditions and particles in ILs likely have higher colloidal stability compared to other non-ILs. However, ILs are relatively expensive for practical applications and relatively strong adsorption of ILs on particle surfaces [Citation43] can lead to some difficulties in complete removal of absorbed IL from active particle surfaces where catalytic reactions occur. Nishikawa et al. [Citation60] introduced the liquid polymer PEG (molecular weight 600) as a more economical, green, and biocompatible liquid with sufficiently low vapour pressure for vacuum sputtering. NPs are less stable in PEG than in ILs due to the lack of electrostatic forces and functional groups in PEG with a strong affinity for metal particles [Citation60]. However, the weak bonding of PEG to particle surfaces can be beneficial for the catalytic performance of sputtered NPs due to more complete removal of the polymer, resulting in clean active surfaces. Thus, Sung et al. produced carbon-supported Pt/Ni alloy NPs via co-sputtering of Pt and Ni targets onto PEG for an hour at 100 W and 300 W, respectively [Citation115]. The shorter Pt-Pt distance in Pt3Ni compared with that in pure Pt NPs, as deduced from XAFS and photoelectron spectroscopy (PES) results, suggested alloy formation.
When fabricating sputtered NPs for bio-applications such as bio-imaging or labelling, the biocompatibility of PEG is highly advantageous [Citation116]. In 2016, Nguyen et al. also used PEG as liquid medium to perform co-sputtering of Au and Ag using a double-target system [Citation117]. The targets were tilted at an angle of 60° to the substrate normal and facing each other so that the collision of ejected metal clusters could form alloy NPs. They varied the sputtering currents of the two targets to finely tailor the composition of the alloy NPs over a wide range from Au rich to equimolar to Ag rich ()). Average particle sizes were in range of ca. 4.2–5.9 nm for NPs of different Au/Ag compositions. Thus, the linear relationship between plasmon resonance peak position and particle composition suggested the formation of a bimetallic Au/Ag alloy, as observed in Au/Ag NPs prepared using a binary target. They provided elemental mapping of single NPs as direct evidence to further confirm their statement that they had created complete solid solution Au/Ag NPs with tuneable compositions ()). Further, the authors demonstrated that the method can be used to create Au/Cu alloy NPs of various controllable compositions at room temperature [Citation118]. This is interesting because Au/Cu is commonly known to form intermetallic compounds in the bulk state, but forms random alloys in double-target sputtering ()). Their recent progress highlighted the successful creation of Au/Ag NCs with fluorescence emission dependent on the alloy composition [Citation119]. They realized the quantum confinement effect when the particle size was decreased by adding cationic capping ligands to the PEG, i.e., 1-mercaptoundecyl-N,N,N-trimethylammonium bromide (MUTAB), to protect the newly formed metal alloy clusters from further growth in the liquid, preventing them from reaching larger sizes with plasmonic properties.
Figure 9. (a) LSPR peak maxima versus Au content measured by EDX in alloy NPs synthesized by independently varying the sputter current of each metal target. Numbers in the figure indicate the sputtering current for each target in mA. (b) EDX mapping for several single plasmonic Au/Ag alloy NPs. (c) EDX mapping for Au/Cu NPs prepared using double-target sputtering. Reproduced with permission from (a and b) Ref. [Citation117], copyright 2016 Elsevier, and (c) Ref. [Citation118], copyright 2017 The American Chemical Society.
![Figure 9. (a) LSPR peak maxima versus Au content measured by EDX in alloy NPs synthesized by independently varying the sputter current of each metal target. Numbers in the figure indicate the sputtering current for each target in mA. (b) EDX mapping for several single plasmonic Au/Ag alloy NPs. (c) EDX mapping for Au/Cu NPs prepared using double-target sputtering. Reproduced with permission from (a and b) Ref. [Citation117], copyright 2016 Elsevier, and (c) Ref. [Citation118], copyright 2017 The American Chemical Society.](/cms/asset/613d20cd-539a-4afc-a8a2-53aae4f53bb3/tsta_a_1542926_f0009_oc.jpg)
4. Applications of sputtered multi-metallic NPs/NCs
With two or three metal elements in single NPs/NCs in the form of an alloy or a segregated structure with sizes from sub-nano to several nanometres, multi-metallic NPs are promising candidates for high performance catalysts, tuneable plasmonics, and new photoluminescent materials with composition and structure dependent properties [Citation79–Citation96]. Compared with single metallic NPs formed by sputtering onto liquids, we noticed that bimetallic NPs exhibited smaller sizes than single metallic ones [Citation84–Citation86,Citation92,Citation94,Citation96,Citation111]. Thus, bimetallic NPs have higher surface area, exposing more active species to the surface. A large number of publications in this field have demonstrated the composition dependent catalytic properties of sputtered metal alloys and their enhanced activity compared with monometallic NPs for ethanol/methanol oxidation, hydrogen reduction reactions, etc. as summarized in . Torimoto’s group found that after immobilization of sputtered Au/Pd NPs on highly oriented pyrolytic graphite (HOPG) substrates, Au/Pd with an Au fraction of 0.61 is optimal for ethanol electro-oxidation ()) [Citation96]. Further, simultaneous sputtering and loading of binary alloy NP catalysts onto TiO2 co-catalysts [Citation103], graphene [Citation108], CNTs [Citation109,Citation110] and amorphous carbon [Citation115], which dispersed in liquid media, allowed for uniform hybridization of the active metal catalyst on the support and aided separation and purification to remove the liquid matrix. Among graphene coated Au/Pd alloy NPs formed via sputtering of AuPd alloy targets with different compositions [Citation108], Wang et al. found that Au/Pd NPs with an Au: Pd molar ratio of 1:3 exhibited superior catalytic activity and stability (500 cycle test) for ethanol electro-oxidation compared with monometallic Pd/graphene or Au/Pd NPs with other compositions ()). The catalytic effect was attributed to the synergistic effect of the Au/Pd alloy and the graphene support. Recently, they found that trimetallic PdAu/Pt NPs [Citation110] resulted in much higher catalytic activity in methanol oxidation than monometallic or bimetal alloy NPs (). On the other hand, as a catalyst for 4-nitrophenol reduction reaction, graphene-supported Au/Pd (Au:Pd = 1:1 mol/mol, diameter 2.3 ± 0.7 nm) bimetallic NPs prepared by using sequential sputtering of Au and Pd target onto [BMI][BF4] ionic liquid exhibited the highest activity among other Au or Pd rich NPs [Citation102,Citation106]. The bimetallic Au/Pd NPs with enhanced catalytic activity for adsorption on catalytic surface was a result of the diluting effect that is Pd, active site, was isolated in Au, less active component, as an increase in Au-to-Pd ratio whereas Au at the adsorption site weaken the interaction of adsorbates to the NP surface [Citation102,Citation106].
Table 1. Summary of multi-metallic NPs obtained via sputtering onto a liquid.
Figure 10. (a) Cyclic voltammograms of AuPd nanoparticle-immobilized HOPG electrodes (solid lines: the positive potential scan, dotted lines: the negative potential scan). The value of fAu used for the preparation of AuPd particles is shown in panel a. (b and c) Plots of peak current density (b) and onset potential (c) observed in the positive potential scan as a function of fAu. The values for a bulk Pt electrode are shown by dashed lines. (d) enhanced stability and ethanol oxidation electro-catalytic activity of AuPd alloy with Au: Pd = 1: 3 mol/mol compared with other compositions and single metallic Pd. Reproduced with permission from (a–c) Ref. [Citation96], copyright The Royal Society of Chemistry 2013 and (d) Ref. [Citation108], copyright Elsevier 2016.
![Figure 10. (a) Cyclic voltammograms of AuPd nanoparticle-immobilized HOPG electrodes (solid lines: the positive potential scan, dotted lines: the negative potential scan). The value of fAu used for the preparation of AuPd particles is shown in panel a. (b and c) Plots of peak current density (b) and onset potential (c) observed in the positive potential scan as a function of fAu. The values for a bulk Pt electrode are shown by dashed lines. (d) enhanced stability and ethanol oxidation electro-catalytic activity of AuPd alloy with Au: Pd = 1: 3 mol/mol compared with other compositions and single metallic Pd. Reproduced with permission from (a–c) Ref. [Citation96], copyright The Royal Society of Chemistry 2013 and (d) Ref. [Citation108], copyright Elsevier 2016.](/cms/asset/eff0e558-16a4-4f22-ac8d-1271264d8ce7/tsta_a_1542926_f0010_oc.jpg)
Figure 11. CV curves for the methanol oxidation reaction using: (a) CNTs-PdAu/Pt (3:1:2) NPs, Pd/Pt and Au/Pt bimetallic NPs, and a commercial Pt/C catalyst, and (b) CNTs-PdAu/Pt trimetallic NPs with different Pd/Au/Pt ratios. The durability (500 cycles) of the oxidation peak current for (c) samples shown in a; and (d) samples shown in b. Reproduced with permission from Ref. [Citation110], copyright 2017 Springer.
![Figure 11. CV curves for the methanol oxidation reaction using: (a) CNTs-PdAu/Pt (3:1:2) NPs, Pd/Pt and Au/Pt bimetallic NPs, and a commercial Pt/C catalyst, and (b) CNTs-PdAu/Pt trimetallic NPs with different Pd/Au/Pt ratios. The durability (500 cycles) of the oxidation peak current for (c) samples shown in a; and (d) samples shown in b. Reproduced with permission from Ref. [Citation110], copyright 2017 Springer.](/cms/asset/5b992c52-3b7d-4dab-b25f-8f89f8870c20/tsta_a_1542926_f0011_oc.jpg)
As for the influence of the liquid medium used on the catalytic activity of the sputtered NPs, it was found that the Pt3Ni alloy NPs obtained in PEG showed a higher oxidation electro-catalytic activity compared to the Pt NPs, which in turn showed a higher activity than the Pt NPs produced using an IL. This was attributed to the synergistic effect from the alloying of Pt and Ni combined with a clean surface after the removal of PEG. The authors suggested that the shorter Pt-Pt distance in Pt3Ni compared to that in pure Pt NPs is the origin of the enhanced catalytic properties obtained via alloying [Citation115]. The short Pt-Pt distance induced by Pt-Ni alloying resulted in the overlapping of Pt d-bands, weakening the binding forces between the NPs and oxygen species [Citation115]. Thus, carbon supported Pt3Ni led to the enhanced oxidation catalytic performance. It is noted that a short Pt-Pt distance as the origin of catalytic activity in Au/Pt NPs had also been reported based on K-edge X-ray absorption fine structure (XAFS) by Asakura et al. [Citation100].
In addition to catalytic activity, alloy NPs/NCs were found to show tuneable optical properties, for example localized surface plasmon resonance (LSPR) peak position, by varying the composition of the produced NPs [Citation92,Citation97,Citation111,Citation117]. Recently, Corpuz et al. [Citation119] reported a novel photoluminescence emission due to the inclusion of Ag into Au/Ag bimetallic NCs with diameters of ca. 1.3–1.5 nm (). These positively charged, fluorescent Au/Ag bimetallic NCs are highly attractive for applications in bio-imaging.
Figure 12. Correlation between the emission energies and the atomic percentage of Ag in positively charged Au/Ag NCs determined by XPS. Reproduced with permission from Ref. [Citation119], copyright 2017 The American Chemical Society.
![Figure 12. Correlation between the emission energies and the atomic percentage of Ag in positively charged Au/Ag NCs determined by XPS. Reproduced with permission from Ref. [Citation119], copyright 2017 The American Chemical Society.](/cms/asset/ec7d6c1f-fdce-4343-84fb-0ecceba2dffd/tsta_a_1542926_f0012_b.gif)
5. Summary and prospects
We have reviewed the synthesis of bi- and trimetallic NPs via vacuum sputtering onto a liquid medium. ILs have been extensively used, whereas the use of other low-volatility liquids have been limited to liquid polymers such as PEG, which is relatively biocompatible and economical liquid. Alloy NPs prepared using a single alloy target, sectional bimetallic target, sequential sputtering onto ILs, or sputtering in combination with chemical reactions have all been investigated. Several approaches have been studied for tailoring the fine structure (alloy, core@shell, partial alloy) and composition of alloy particles. The use of a double-target system is still at an immature stage, with few publications, but it has opened up new opportunities for creating alloys and manipulating their compositions and structures by tuning various sputtering parameters. Further developments in double-target sputtering include using a liquid with the addition of functional protecting agents for the stabilization of NPs in liquid, creating alloy NCs with new properties based on decreasing particle size and multiple metal compositions, investigating sputtering parameters for creating fine structures of interest, and introducing materials for NP immobilization. The obtained particles are potential candidates for efficient catalysts and highly pure materials for optical applications, bio-imaging, and sensing as well as for energy conversion and storage. Although bi- and trimetallic NPs have been prepared in ILs, PEG, and with addition of capping agents via sputtering, there has been no report so far addressing the impact of the liquid nature on different metal elements in the NPs, on the NP fine structure, and on the alloy formation. This can be an interesting topic for researchers in this field. Besides, the growth of sputtered particles, in particular the bi- and trimetallic ones, by co-sputtering of two or more metal targets is fundamentally important. Thus, understanding the state and interaction of metal atoms/clusters/NPs before landing on the liquid surface, on the surface, and in the bulk liquid during and post-sputtering is highly demanded, but remains an open question. Hence, not only systematic investigations of the influence of various sputter parameters as well as the liquid physicochemical properties in the sputtered particles but also the implementation of in situ analysis in combination with simulation are recommended for the future studies. Introducing reactive gas and sputter systems, which allow manipulation of energies and chemical state of the sputtered atoms/clusters, for doping multiple metal NPs and for synthesizing desired compounds and structures can be also considered for sputtering onto liquids towards advanced nanomaterials. Regarding production of metal, metal alloy and hybrid nanoparticles with wide variety of morphologies, higher ordered structure, doping and functionalization, we have demonstrated that our solution synthesis approaches based on chemical reduction, plasma in liquid process and stabilizing agents are very versatile [Citation120–Citation126] though in many cases toxic chemicals and by-products are present as they are also expected in other reports. Hence, we encourage and welcome other researchers join investigating this new vacuum sputtering onto liquid method, take all of its great advantages as the green technique for pure nanoparticles and unexpected structures, as well as devote new ideas for achieving more versatile control of the sputtered NPs.
Acknowledgements
This work is partially supported by Kakenhi [17K1407207; 18H0182008; 18KK0159] from JSPS. TY thanks a partial financial support from Murata Science Foundation. MTN and TY thank Hokkaido University for partial support. TY also thanks Murata Foundation for a financial support.
Disclosure statement
No potential conflict of interest was reported by the authors.
Additional information
Funding
References
- Kelly PJ , Arnell RD. Magnetron sputtering: a review of recent developments and applications. Vacuum. 2000;56(3):159–172.
- Wender H , Migowski P , Feil AF , et al. Sputtering deposition of metal nanoparticles onto liquid substrates: recent advances and future trends. Coordin Chem Rev. 2013;257(17–18):2468–2483.
- Bräuer G , Szyszka B , Vergöhl M , et al. Magnetron sputtering – milestones for 30 years. Vacuum. 2010;84(12):1354–1359.
- Alexeeva OK , Fateev VN . Application of magnetron sputtering for nanostructured electrocatalysts synthesis. Int J Hydrogen Energ. 2016;41(5):3373–3386.
- Khan W , Wang Q , Jin X . Effect of target composition and sputtering deposition parameters on the functional properties of nitrogenized Ag-permalloy flexible thin films deposited on polymer substrates. Mater. 2018;11(3):439.
- Maurizio C , Michieli N , Kalinic B , et al. Local structure and X-ray magnetic circular dichroism of Au in Au-Co nanoalloys. Appl Surf Sci. 2018;433:596–601.
- Kariuki NN , Khudhayer WJ , Karabacak T , et al. GLAD Pt-Ni alloy nanorods for oxygen reduction reaction. ACS Catal. 2013;3(12):3123–3132.
- Debieu O , Nalini RP , Cardin J , et al. Structural and optical characterization of pure Si-rich nitride thin films. Nanoscale Res Lett. 2013;8:57.
- Figueroa AI , Bartolome J , Garcia LM , et al. Structural and magnetic properties of granular Co-Pt multilayers with perpendicular magnetic anisotropy. Phys Rev B. 2014;90(17):174421.
- Ngene P , Westerwaal RJ , Sachdeva S , et al. Polymer-induced surface modifications of Pd-based thin films leading to improved kinetics in hydrogen sensing and energy storage applications. Angew Chem Int Ed. 2014;53:12081–12085.
- Wang L , Gao T , Yu Y . Effects of Ag addition on FePt L1(0) ordering transition: a direct observation of ordering transition and Ag segregation in FePtAg alloy films. J Appl Phys. 2015;118:235304.
- Janczak-Rusch J , Chiodi M , Cancellieri C , et al. Structural evolution of Ag-Cu nano-alloys confined between AlN nano-layers upon fast heating. Phys Chem Chem Phys. 2015;17(42):28228–28238.
- Manoharan EA , Mankey G , Hong YK . Tuning the magnetic properties of Fe50-xMnxPt50 thin films. J Magn Magn Mater. 2017;438:111–115.
- Brummel O , Waidhas F , Khalakhan I , et al. Structural transformations and adsorption properties of PtNi nanoalloy thin film electrocatalysts prepared by magnetron co-sputtering. Electrochim Acta. 2017;251:427–441.
- Yu JG , Sun XH , Gong HH , et al. Influence of Ag concentration on microstructure, mechanical properties and cytocompatibility of nanoscale Ti-Ag-N/Ag multilayers. Surf Coat Technol. 2017;312:128–133.
- Zhu Y , Yuan M , Deng L , et al. High-efficiency electrochemical hydrogen evolution based on the intermetallic Pt2Si compound prepared by magnetron-sputtering. RSC Adv. 2017;7(3):1553–1560.
- Inoue M , Shingen H , Kitami T , et al. Preparation and physical and electrochemical properties of carbon-supported Pt-Ru (Pt-Ru/C) samples using the polygonal barrel-sputtering method. J Phys Chem C. 2008;112(5):1479–1492.
- Guo H , Kemell M , Al-Hunaiti A , et al. Gold-palladium supported on porous steel fiber matrix: structured catalyst for benzyl alcohol oxidation and benzyl amine oxidation. Catal Commun. 2011;12(13):1260–1264.
- Martinez L , Diaz M , Roman E , et al. Generation of nanoparticles with adjustable size and controlled stoichiometry: recent advances. Langmuir. 2012;28(30):11241–11249.
- Alissawi N , Zaporojtchenko V , Strunskus T , et al. Effect of gold alloying on stability of silver nanoparticles and control of silver ion release from vapor-deposited Ag-Au/polytetrafluoroethylene nanocomposites. Gold Bull. 2013;46(1):3–11.
- Johnson GE , Colby R , Laskin J . Soft landing of bare nanoparticles with controlled size, composition, and morphology. Nanoscale. 2015;7(8):3491–3503.
- Gholamali H , Shafiekhani A , Darabi E , et al. Ag/Au Alloy LSPR engineering by co-deposition of RF-sputtering and RF-PECVD. Appl Orgnomet Chem. 2018;32(5):e4316.
- Pylypenko S , Queen A , Olson TS , et al. Tuning carbon-based fuel cell catalyst support structures via nitrogen functionalization. II. Investigation of durability of Pt-Ru nanoparticles supported on highly oriented pyrolytic graphite model catalyst supports as a function of nitrogen implantation dose. J Phys Chem C. 2011;115(28):13676–13684.
- Guo X , Brault P , Zhi G , et al. Synergistic combination of plasma sputtered Pd-Au bimetallic nanoparticles for catalytic methane combustion. J Phys Chem C. 2011;115(22):11240–11246.
- Hiromi C , Inoue M , Taguchi A , et al. Optimum Pt and Ru atomic composition of carbon-supported Pt-Ru alloy electrocatalyst for methanol oxidation studied by the polygonal barrel-sputtering method. Electrochim Acta. 2011;56(24):8438–8445.
- Kariuki NN , Cansizoglu MF , Begum M , et al. SAD–GLAD Pt–ni@Ni Nanorods as highly active oxygen reduction reaction electrocatalysts. ACS Catal. 2016;6(6):3478–3485.
- Radnoczi G , Bokanyi E , Erdelyi Z , et al. Size dependent spinodal decomposition in Cu-Ag nanoparticles. Acta Mater. 2017;123:82–89.
- Kim SH , Shim GI , Choi SY . Fabrication of Nb-doped ZnO nanowall structure by RF magnetron sputter for enhanced gas-sensing properties. J Alloy Compd. 2017;698:77–86.
- Kunwar S , Pandey P , Sui M , et al. Evolution of ternary AuAgPd nanoparticles by the control of temperature, thickness, and tri-layer. Metal. 2017;7(11):472.
- Zhang H , Wang C , Li H , et al. Physical process-aided fabrication of periodic Au–M (M = Ag, Cu, Ag–cu) alloyed nanoparticle arrays with tunable localized surface plasmon resonance and diffraction peaks. RSC Adv. 2018;8(17):9134–9140.
- Cai RS , Ellis PR , Yin JL , et al. Performance of preformed Au/Cu nanoclusters deposited on MgO powders in the catalytic reduction of 4-nitrophenol in solution. Small. 2018;14(13):1703734.
- Spanu D , Recchia S , Mohajernia S , et al. Templated dewetting–alloying of NiCu bilayers on TiO2 nanotubes enables efficient noble-metal-free photocatalytic H2 evolution. ACS Catal. 2018;8(6):5298–5305.
- Liu Y . Synthesis of AuPd alloy nanoparticles on ZnO nanorod arrays by sputtering for surface enhanced Raman scattering. Mater Lett. 2018;224:26–28.
- Vahl A , Strobel J , Reichstein W , et al. Single target sputter deposition of alloy nanoparticles with adjustable composition via a gas aggregation cluster source. Nanotechnology. 2017;28(17):175703.
- Ye G , Zhang Q , Feng C , et al. Structure and electrical properties of a metallic rough-thin-film system deposited on liquid substrates. Phys Rev B. 1996;54(20):14754–14757.
- Grammatikopoulos P , Steinhauer S , Vernieres J , et al. Nanoparticle design by gas-phase synthesis. Adv Phys X. 2016;1(1):81–100.
- Howes PD , Chandrawati R , Stevens MM . Bionanotechnology. Colloidal nanoparticles as advanced biological sensors. Science. 2014;346(6205):1247390.
- You H , Yang S , Ding B , et al. Synthesis of colloidal metal and metal alloy nanoparticles for electrochemical energy applications. Chem Soc Rev. 2013;42(7):2880–2904.
- Wagener M , Günther B . Sputtering on liquids-a versatile process for the production of magnetic suspension. J Magn Magn Mater. 1999;201:41–44.
- Zhang C , Feng Y . Fabrication of size-controlled gold nanoparticles on silicone oil surface and mechanism for size-controllability. J Phys Soc Jpn. 2015;84:074601 (4 pages).
- Torimoto T , Okazaki K , Kiyama T , et al. Sputter deposition onto ionic liquids: simple and clean synthesis of highly dispersed ultrafine metal nanoparticles. Appl Phys Lett. 2006;89(24):243117.
- Torimoto T , Tsuda T , Okazaki K , et al. New frontiers in materials science opened by ionic liquids. Adv Mater. 2010;22(11):1196–1221.
- He Z , Alexandridis P . Nanoparticles in ionic liquids: interaction and organization. Phys Chem Chem Phys. 2015;17(28):18238–18261.
- Dupont J . From molten salts to ionic liquids: a “nano” journey. Acc Chem Res. 2011;44(11):1223–1231.
- Oda Y , Hirano K , Yoshii K , et al. Palladium nanoparticles in ionic liquid by sputter deposition as catalysts for suzuki-miyaura coupling in water. Chem Lett. 2010;39(10):1069–1071.
- Hamm SC , Shankaran R , Korampally V , et al. Sputter-deposition of silver nanoparticles into ionic liquid as a sacrificial reservoir in antimicrobial organosilicate nanocomposite coatings. ACS Appl Mater Interfaces. 2012;4(1):178–184.
- Yoshii K , Yamaji K , Tsuda T , et al. Highly durable Pt nanoparticle-supported carbon catalyst for the oxygen reduction reaction tailored by using an ionic liquid thin layer. J Mater Chem A. 2016;4(31):12152–12157.
- Wender H , de Oliveira LF , Migowski P , et al. Ionic liquid surface composition controls the size of gold nanoparticles prepared by sputtering deposition. J Phys Chem C. 2010;114(27):11764–11768.
- Hatakeyama Y , Onishi K , Nishikawa K . Effect of sputtering conditions on formation of gold nanoparticles in sputter deposition technique. RSC Adv. 2011;1(9):1815–1821.
- Hatakeyama Y , Judai K , Onishi K , et al. Anion and cation effects on the size control of Au nanoparticles prepared by sputter deposition in imidazolium-based ionic liquids. Phys Chem Chem Phys. 2016;18(4):2339–2349.
- Hatakeyama Y , Kimura S , Kameyama K , et al. Temperature-independent formation of Au nanoparticles in ionic liquids by arc plascarma deposition. Chem Phys Lett. 2016;658:188–191.
- Vanecht E , Binnemans K , Seo JW , et al. Growth of sputter-deposited gold nanoparticles in ionic liquids. Phys Chem Chem Phys. 2011;13(30):13565–13571.
- Redel E , Walter M , Thomann R , et al. Stop-and-go, stepwise and “ligand-free” nucleation, nanocrystal growth and formation of Au NPs in ionic liquids (ILs). Chem Commun. 2010;46(7):1159–1161.
- Roese S , Kononov A , Timoshenko J , et al. Cluster assemblies produced by aggregation of preformed Ag clusters in ionic liquids. Langmuir. 2018;34(16):4811–4819.
- Janiak C . Inonic liquids for the syntehsis and stabilization of metal nanoparticles. Z Naturforsch. 2013;68b:1059–1089.
- He Z , Alexandridis P . Nanoparticles in ionic liquids: interactions and organization. Phys Chem Chem Phys. 2015;17:18238–18261.
- Wegner S , Janiak C . Metal nanoparticles in ionic liquids. Top Curr Chem (Z). 2017;375:65.
- Shishino Y , Yonezawa T , Udagawa S , et al. Preparation of optical resins containing dispersed gold nanoparticles by the matrix sputtering method. Angew Chem Int Ed. 2011;50(3):703–705.
- Ishida Y , Udagawa S , Yonezawa T . Understanding the primary and secondary aggregation states of sputtered silver nanoparticles in thiolate matrix and their immobilization in resin. Colloids Surf A. 2016;504:437–441.
- Hatakeyama Y , Morita T , Takahashi S , et al. Synthesis of gold nanoparticles in liquid polyethylene glycol by sputter deposition and temperature effects on their size and shape. J Phys Chem C. 2011;115(8):3279–3285.
- Nakagawa K , Narushima T , Udagawa S , et al. Preparation of copper nanoparticles in liquid by matrix sputtering process. J Phys Conf Ser. 2013;417:012038.
- Porta M , Nguyen MT , Yonezawa T , et al. Titanium oxide nanoparticle dispersions in a liquid monomer and solid polymer resins prepared by sputtering. New J Chem. 2016;40(11):9337–9343.
- Deng L , Nguyen MT , Yonezawa T . Sub-2 nm single-crystal Pt nanoparticles via sputtering onto a liquid polymer. Langmuir. 2018;34(8):2876–2881.
- Shishino Y , Yonezawa T , Kawai K , et al. Molten matrix sputtering synthesis of water-soluble luminescent Au nanoparticles with a large Stocks shift. Chem Commun. 2010;46(38):7211–7213.
- Wender H , de Oliveira LF , Feil AF , et al. Synthesis of gold nanoparticles in a biocompatible fluid from sputtering deposition onto castor oil. Chem Commun. 2010;46(37):7019–7021.
- Wender H , Gonçalves RV , Feil AF , et al. Sputtering onto liquids: from thin film to nanoparticles. J Phys Chem C. 2011;115(33):16362–16367.
- Bussamara R , Eberhardt D , Feil AF , et al. Sputtering deposition of magnetic Ni nanoparticles directly onto an enzyme surface: a novel method to obtain a magnetic biocatalyst. Chem Commun. 2013;49(13):1273–1275.
- Fujita A , Matsumoto Y , Takeuchi M , et al. Growth behaviour of gold nanoparticles synthesized in unsaturated fatty acids by vacuum evaporation method. Phys Chem Chem Phys. 2016;18(7):5464–5470.
- Akita I , Ishida Y , Yonezawa T . Ligand effect on the formation of gold nanoparticles via sputtering deposition over a liquid matrix. Bull Chem Soc Jpn. 2016;89(9):1054–1056.
- Ishida Y , Udagawa S , Yonezawa T . Growth of sputtered silver nanoparticles on a liquid mercaptan matrix with controlled viscosity and sputter rate. Colloids Surf A. 2016;498:106–111.
- Staszek M , Siegel J , Polívková M , et al. Influence of temperature on silver nanoparticle size prepared by sputtering into PEG-glycerol system. Mater Lett. 2017;186:341–344.
- Ishida Y , Sumi T , Yonezawa T . Sputtering synthesis and optical investigation of octadecanthiol-protected fluorescent Au nanoparticles. New J Chem. 2015;39(8):5895–5897.
- Ishida Y , Lee C , Yonezawa T . A novel physical approach for cationic-thiolate protected fluorescent gold nanoparticles. Sci Rep. 2015;5:15372.
- Porta M , Nguyen MT , Tokunaga T , et al. Matrix sputtering into liquid mercaptan: from blue-emitting copper nanoclusters to red-emitting copper sulfide nanoclusters. Langmuir. 2016;32(46):12159–12165.
- Porta M , Nguyen MT , Ishida Y , et al. Highly stable and blue-emitting copper nanocluster dispersion prepared by magnetron sputtering over liquid polymer matrix. RSC Adv. 2016;6(107):105030–105034.
- Ishida Y , Nakabayashi R , Corpuz RD , et al. Water-dispersible fluorescent silver nanoparticles via sputtering deposition over liquid polymer using a very short thiol ligand. Colloids Surf A. 2017;518:25–29.
- Corpuz RD , Ishida Y , Yonezawa T . Synthesis of cationically charged photoluminescent coinage metal nanoclusters by sputtering over a liquid polymer matrix. New J Chem. 2017;41(14):6828–6833.
- Ishida Y , Corpuz RD , Yonezawa T . Matrix sputtering method: a novel physical approach for photoluminescent noble metal nanoclusters. Acc Chem Res. 2017;50(12):2986–2995.
- Cha IY , Yoo SJ , Jang JH . Recent progress in nanoparticle synthesis via liquid medium sputtering and its applications. J Electrochem Sci Technol. 2016;7(1):13–26.
- Kusada K , Kobayashi H , Ikeda R , et al. Solid solution alloy nanoparticles of immiscible Pd and Ru elements neighboring on Ru: changeover of the thermodynamic behaviour for hydrogen storage and enhanced CO-oxidizing ability. J Am Chem Soc. 2014;136(5):1864–1871.
- Huang B , Kobayashi H , Yamamoto T , et al. Solid-solution alloying of immiscible Ru and Cu with enhanced CO oxidation activity. J Am Chem Soc. 2017;139(13):4643–4646.
- Zhang Z , Nenoff TM , Huang JY , et al. Room temperature synthesis of thermally immiscible Ag-Ni alloys. J Phys Chem C. 2009;113(4):1155–1159.
- Xu J , Zhao T , Liang Z , et al. Facile preparation of AuPt alloy nanoparticles from organometallic complex precursor. Chem Mater. 2008;20(5):1688–1690.
- Toshima N , Yonezawa T , Kushihashi K . Polymer-protected palladium-platinum bimetallic clusters: preparation, catalytic properties and structural considerations. J Chem Soc Faraday Trans. 1993;89(14):2537–2543.
- Toshima N , Harada M , Yonezawa T , et al. Structural analysis of polymer-protected palladium/platinum bimetallic clusters as dispersed catalysts by using extended x-ray absorpbtion fine structure spectroscopy. J Phys Chem. 1991;95(19):7448–7453.
- Yonezawa T , Toshima N . Polymer- and micelle-protected gold/platinum bimetallic systems. Preparation, application to catalysis for visible-light-induced hydrogen evolution, and analysis of formation process with optical methods. J Mol Catal. 1993;83(1–2):167–181.
- Čempel D , Nguyen MT , Ishida Y , et al. Ligand free green plasma-in-liquid synthesis of Au/Ag Alloy nanoparticles. New J Chem. 2017;42(8):5680–5687.
- Shirai H , Nguyen MT , Čempel D , et al. Preparation of Au/Pd bimetallic nanoparticles by a microwave-induced plasma in liquid process. Bull Chem Soc Jpn. 2017;90(3):279–285.
- Toshima N , Yonezawa T . Bimetallic nanoparticles-novel materials for chemical and physical applications. New J Chem. 1988;22(11):1179–1201.
- Mallin MP , McDonagh AM . Synthesis and optical properties of hybrid and alloy plasmonic nanoparticles. Chem Rev. 2011;111(6):3713–3735.
- Ferrando R , Jellinek J , Johnston RL . Nanoalloy: from theory to applications of alloy clusters and nanoparticles. Chem Rev. 2008;108(3):845–910.
- Okazaki K , Kiyama T , Hirahara K , et al. Single-step synthesis of gold-silver alloy nanoparticles in ionic liquids by a sputter deposition technique. Chem Commun. 2008;(6):691–693.
- Mallin MP , Murphy CJ . Solution-phase synthesis of sub-10 nm Au-Ag nanoparticles. Nano Lett. 2002;2(11):1235–1237.
- Liu S , Chen G , Prasad PN , et al. Synthesis of monodisperse Au, Ag, and Au-Ag alloy nanoparticles with tunable size and surface plasmon resonance frequency. Chem Mater. 2011;23(18):4098–4101.
- Suzuki S , Suzuki T , Tomita Y , et al. Compositional control of AuPt nanoparticles synthesized in ionic liquids by the sputter deposition technique. CrystEngComm. 2012;14(15):4922–4926.
- Hirano M , Enokida K , Okazaki K , et al. Composition-dependent electrocatalytic activity of AuPd alloy nanoparticles prepared via simultaneous sputter deposition into an ionic liquid. Phys Chem Chem Phys. 2013;15(19):7286–7294.
- Suzuki S , Tomita Y , Kuwabata S , et al. Synthesis of alloy AuCu nanoparticles with the L10 structure in an ionic liquid using sputter deposition. Dalton Trans. 2015;44(9):4186–4194.
- Suzuki T , Okazaki K , Kiyama T , et al. A facile synthesis of AuAg alloy nanoparticles using a chemical reaction induced by sputter deposition of metal onto ionic liquids. Electrochem. 2009;77(8):636–638.
- Okazaki K , Sakuma J , Yasui J , et al. Fabrication of nanoframe structures by site-selective assembly of gold nanoparticles on silver cubes in an ionic liquid. Chem Lett. 2011;40(1):84–86.
- Kaito T , Mitsumoto H , Sugawara S , et al. K-edge X-ray absorption fine structure analysis of Pt/Au core-shell electrocatalyst: evidence for short Pt-Pt distance. J Phys Chem C. 2014;118(16):8481–8490.
- Liu CH , Chen XQ , Hu YF , et al. One-pot environmentally friendly approach toward highly catalytically active bimetal-nanoparticle-graphene hybrids. ACS Appl Mater Interfaces. 2013;5(11):5072–5079.
- Liu CH , Liu RH , Sun QJ , et al. Controlled synthesis and synergistic effects of graphene-supported PdAu bimetallic nanoparticles with tunable catalytic properties. Nanoscale. 2015;7(14):6356–6362.
- Chang JB , Liu CH , Liu J , et al. Green-chemistry compatible approach to TiO2-supported PdAu bimetallic nanoparticles for solvent-free 1-phenylethanol oxidation under mild condition. Nano-Micro Lett. 2015;7(3):307–315.
- Sugioka D , Kameyama T , Kuwabata S , et al. Formation of a Pt-decorated Au nanoparticle nanolayer floating on an ionic liquid by the ionic liquid/metal sputtering method and tunable electrocatalytic activities of the resulting monolayer. ACS Appl Mater Interfaces. 2016;8(17):10874–10883.
- Hamada T , Sugioka D , Kameyama T , et al. Electrocatalytic activity of bimetallic Pd-Au particle films prepared by sequential sputter deposition of Pd and Au onto hydroxyl-functionalized ionic liquid. Chem Lett. 2017;46(7):956–959.
- Liu CH , Chen N , Li J , et al. Fingerprint feature of atomic intermixing in supported AuPd nanocatalysts probed by X-ray absorption fine structure. J Phys Chem C. 2017;121(51):8385–28394.
- Liu CH , Liu J , Zhou YY , et al. Small and uniform Pd monometallic/bimetallic nanoparticles decorated on multi-walled carbon nanotubes for efficient reduction of 4-nitrophenol. Carbon N Y. 2015;94:295–300.
- Liu CH , Cai XL , Wang JS , et al. One-step synthesis of AuPd alloy nanoparticles on graphene as a stable catalyst for ethanol electro-oxidation. Int J Hydrogen Energ. 2016;41(31):13476–13484.
- Zhou YY , Liu CH , Liu J , et al. Self-decoration of PtNi alloy nanoparticles on multiwalled carbon nanotubes for high efficient methanol electro-oxidation. Nano-Micro Lett. 2016;8(4):371–380.
- Cai XL , Liu CH , Liu L , et al. Synergistic effects in CNTs-PdAu/Pt trimetallic nanoparticles with high electrocatalytic activity and stability. Nano-Micro Lett. 2017;9:48 (10 pages).
- König D , Richter K , Siegel A , et al. High-throughput fabrication of Au-Cu nanoparticle libraries by a combinatorial sputtering in ionic liquids. Adv Funct Mater. 2014;24(14):2049–2056.
- Heym F , Etzold BJM , Kern C , et al. Analysis of evaporation and thermal decomposition of ionic liquids by thermogravimetrical analysis at ambient pressure and high vacuum. Green Chem. 2011;13(6):1453–1466.
- Kuwabata S , Tsuda T , Torimoto T . Room-temperature ionic liquid. A new medium for material production and analyses under vacuum conditions. J Phys Chem Lett. 2010;1(21):3177–3188.
- Aschenbrenner O , Supasitmongkol S , Marie Taylor M , et al. Measurement of vapour pressures of ionic liquids and other low vapour pressure solvents. Green Chem. 2009;11(8):1217–1221.
- Cha IY , Ahn M , Yoo SJ , et al. Facile synthesis of carbon supported metal nanoparticles via sputtering onto a liquid substrate and their electrochemical application. RSC Adv. 2014;4:38575–38580.
- Knop K , Hoogenboom R , Fischer D , et al. Poly(ethyleneglycol) in drug delivery: pros and cons as well as potential alternatives. Angew Chem Int Ed Engl. 2010;49(36):6288–6308.
- Nguyen MT , Yonezawa T , Wang Y , et al. Double target sputtering into liquid: a new approach for preparation of Ag-Au alloy nanoparticles. Mater Lett. 2016;171:75–78.
- Nguyen MT , Zhang H , Deng L , et al. Au/Cu bimetallic nanoparticles via double-target sputtering onto a liquid polymer. Langmuir. 2017;33(43):12389–12397.
- Corpuz RD , Ishida Y , Nguyen MT , et al. Synthesis of positively charged photoluminescent bimetallic Au-Ag nanoclusters by double-target sputtering method on a biocompatible polymer matrix. Langmuir. 2017;33(36):9144–9150.
- Shirai H , Huang YY , Yonezaw T , et al. Hard-templating synthesis of macroporous platinum microballs (MPtM). Mater Lett. 2016;164:488–492.
- Liao YT , Chen JE , Ishida Y , et al. De novo synthesis of gold-nanoparticle-embedded, nitrogen-doped nanoporous carbon nanoparticles (Au@NC) with enhanced reduction ability. ChemCatChem. 2016;8(3):502–509.
- Čempel D , Nguyen MT , Ishida Y , et al. Au nanoparticles prepared using a coated electrode in plasma-in-liquid process: effect of the solution pH. J Nanosci Nanotechnol. 2016;16(9):9257–9262.
- Juan LMZ , Nguyen MT , Yonezawa T , et al. Structural control parameters for formation of single-crystalline β-Sn nanorods in organic phase. Cryst Growth Des. 2017;17(9):4554–4562.
- Nishimoto M , Yonezawa T , Čempel D , et al. Effect of H2O2 on Au nanoparticle preparation using microwave-induced plasma in liquid. Mater Chem Phys. 2017;193:7–12.
- Shirai H , Nguyen MT , Ishida Y , et al. A new approach for additive-free room temperature sintering of conductive patterns using polymer-stabilized Sn nanoparticles. J Mater Chem C. 2016;4(11):2228–2234.
- Ishida Y , Motokane Y , Tokunaga T , et al. Plasma-induced tungsten doping of TiO2 nanoparticles for enhanced photocatalysis using visible light. Phys Chem Chem Phys. 2015;17(38):24556–24559.