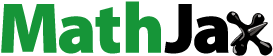
ABSTRACT
Constructing satisfied small-diameter vascular graft (diameter less than 6 mm) remains an unsolvable challenge in vascular tissue engineering. This study described the fabrication of electrospun polyurethane/polycaprolactone (PU/PCL) membranes chemically grafted with various densities of conjugated linoleic acid (CLA) – an antithrombotic fatty acid – for making small-diameter blood vessel. Differences in mechanical, antithrombotic properties and biocompatibility of the membranes resulting from the CLA-grafting procedure were the focus of the study. Investigation of mechanical properties relevant to vascular graft application revealed that these properties of the membranes remained unaffected and satisfied clinical criteria following the CLA graft. Blood–membrane interaction assays showed that the CLA-grafted membranes mitigated the adhesion of blood cells, as well as preventing blood coagulation. These effects were also commensurate with increasing density of CLA, suggesting an effective approach to improve antithromboticity. Cellular tests suggested that CLA has an optimal density at which it promoted cell proliferation on the surface of the membranes; however, excessive presence of CLA might cause undesirable inhibition on cells. In conclusion, PU/PCL membrane grafted with CLA could be a prospective material for vascular tissue engineering with further development and investigation.
Graphical Abstract

1. Introduction
On a global scale, cardiovascular diseases were, are and will still be one of the leading cause of death for years to come [Citation1]. Although dietary, lifestyle modifications and pharmaceutical intervention are prospective treatments for cardiovascular diseases, surgery is still considered the last resort and the most effective solution for severe cases [Citation2]. Similar to the grafting procedures of other tissues, autologous blood vessels are still the current gold standard for vascular graft. However, besides the usual drawbacks of autologous harvest such as limited availability and morbidity at surgery site, these vessels are troubling in quality [Citation3,Citation4]. On the other hand, xenografts suffer from other arising issues due to their unstable sources and poor controllability on their physical and mechanical properties [Citation5,Citation6].
After decades of development, current large-diameter (>8 mm) and medium-diameter (6–8 mm) synthetic vessels have shown adequate efficiency both in short term and long term [Citation7,Citation8]. However, challenge remains in the fabrication of usable small-diameter (<6 mm) vessels [Citation9]. As the typical dimension of coronary arteries, infrainguinal arteries and infrageniculate arteries, this small cross-section makes the graft prone to re-occlusion problem associated with atherosclerosis, thrombosis, aneurysm and intimal hyperplasia [Citation5,Citation10], which eventually lead to rejection and recurring vascular diseases. In a material perspective, maintaining mechanical properties whilst reducing diameter of a vessel is also challenging.
Thus, the situation demands a composite vessel with a small cross-sectional diameter that can satisfy all requirements of a tissue-engineered vascular graft. It must be able to immediately provide reasonable mechanical functions. In addition, other physicochemical properties and biocompatibility of the scaffold should be relatively similar to the target extracellular matrix [Citation11]. The grafts should also be antithrombotic and eventually allows the proliferation of an antithrombotic lumen (autologous endothelium) [Citation12].
Large varieties of materials and methods have been studied to achieve these goals. Among them, many scientists have fabricated artificial blood vessels using electrospinning method due to its simplicity and controllability [Citation11,Citation13]. Regardless of the superiority of electrospinning, the challenges in fabricating artificial electrospun blood vessels remained in the selection and manipulation of base materials and antithrombotic approach. This study takes into focus two FDA-approved polymers which are common electrospinning material and prospective candidate for artificial blood vessels: polyurethane (PU) and polycaprolactone (PCL) [Citation14,Citation15]. PU provides excellent strength, elasticity and biocompatible with blood and tissue [Citation16]; and is favorable to the proliferation of endothelial cells [Citation17]. On the other hand, PCL is biodegradable, biocompatible and possesses adequate mechanical properties [Citation18]. Previous study showed that its combination with PU enhanced the mechanical properties and positively impacted the proliferation of seeded endothelial cells [Citation19,Citation20]. However, these studies have not investigated the antithrombotic properties of the fabricated PU/PCL vessels.
Previously, our group attempted to improve the antithrombotic properties of PU/PCL vessels by mixing the electrospinning polymer solution with conjugated linoleic acid (CLA) [Citation21]. This unsaturated fatty acid is a drug known for antithrombosis application [Citation22]. This activity of CLA has been investigated considerably in the fabrication of hemodialysis membrane from polysulfone, polyacrylonitrile and cellulose acetate [Citation23–Citation25]. However, its application as an antithrombotic approach for artificial vascular graft is still underdeveloped. The mentioned study [Citation21] has yielded positive results regarding the antiplatelet adhesion properties of the material by introducing CLA into the composite. However, the direct loading of CLA into the polymeric fibers hampered the activity of CLA and weakened the structural integrity and mechanical properties of the membrane. Therefore, other methods to introduce CLA onto the surface of PU/PCL membrane are required to grasp its antithrombotic potential without compromising other properties.
The CLA molecule is smaller compared to other usual antithrombotic agents (e.g. heparin), which might facilitate its grafting on a polymeric surface using chemical means due to low steric hindrance. Based on this background, this study investigated the chemical grafting of CLA on the surface of PU/PCL electrospun membrane for the purpose of artificial vascular graft. CLA is hypothesized to be bonded with the membrane via esterification using carbodiimide coupling agent. The center of the research is the alteration of the membrane caused by the whole grafting process and the density of CLA. As such, the project investigated the physicochemical properties, blood – material interaction and cell – material interaction (using fibroblasts and endothelial cells) of the CLA-grafted membranes, to reveal the effect of this combination. It is expected that the grafting can improve antithrombotic activities and biocompatibility of the membranes without compromising their mechanical strength.
2. Materials and methods
2.1. Fabrication of CLA-grafted PU/PCL membrane
2.1.1. Material
Polycaprolactone, poly [4,4′-methylenebis(phenyl isocyanate)-alt-1,4-butanediol/di(propylene glycol)/polycaprolactone] (PU), N,N-dimethyl formamide (DMF), and tetrahydrofuran (THF) were purchased from Sigma-Aldrich (USA). Conjugated linoleic acid (CLA), glutaraldehyde and the condensing agent N-(3-Dimethylaminopropyl)-N′-ethyl carbodiimide (EDC) were also purchased from Sigma-Aldrich. Absolute ethanol was bought from Xilong manufacturer. All other chemicals are analytical graded.
2.1.2. Electrospinning procedure
12% w/w polymer solution was prepared by dissolving PU/PCL pellets (1:1 w/w) into mixed DMF: THF (1:1 v/v). The mixture was stirred at room temperature overnight. The solution was then put into a glass syringe, attached to a peristaltic pump (Harvard Apparatus, Infusion Syringe Pump 980638). The needle tip was supplied with a 20 kV voltage and fibers were collected on a stationary disk at 12 cm distance.
2.1.3. CLA grafting procedure
summarizes the scheme to graft CLA on the surface of PU/PCL membrane. To optimize the grafted-CLA density on PU/PCL membrane, the concentration of EDC were varied. CLA was diluted in absolute ethanol to obtained CLA solution at the molar concentration of 0.4 mM.
Firstly, PU/PCL membranes were plasma-treated under low pressure. Then, 1 × 1 cm membrane was immersed in 500 µL of absolute ethanol containing 0.4 mM CLA and varied concentration (4 mM, 8 mM or 12 mM) of EDC for 24 h at 4°C. After the immersion, the membranes were washed twice with absolute ethanol and then three times with distilled water. The experiments were conducted in dark room. Finally, the samples were vacuum-dried. Fabrication parameters of the six samples tested in this study are summarized in . As a comparison, a membrane was immersed in CLA solution without EDC and was labeled as C2-E0.
Table 1. Fabrication parameters of the samples and experimental density of CLA on surface of PU/PCL. Data presented as average ± S.D
2.2. Surface characterization
2.2.1. Quantification of the CLA density on surface of PU/PCL membranes
CLA grafted on the membrane surface was removed using a reported de-esterification method with modifications [Citation26]. Briefly, a 0.5 M solution of HCl was prepared in absolute ethanol – distilled water mixture (9:1 v/v). Then, the CLA grafted membranes were immersed in this solution and incubated at 37°C for 3 h under agitation to de-esterify and remove CLA from the surface. After the incubation period, the supernatants were transferred to a 96-well plate and their concentrations of CLA were determined using UV-Vis spectroscopy at wavelength of 233 nm based on a previously built calibration curve [Citation27]. The amount of CLA in the washing solution was determined as the total amount of CLA on the membrane.
2.2.2. Surface morphology of PU/PCL membranes
Dried membranes (1 × 1 cm) were sputter-coated with gold. Then, a scanning electron microscope (SEM, JSM-IT100, JEOL, Japan) with an accelerating voltage of 10 kV was used to acquire the images of the membranes.
2.2.3. Surface wettability of PU/PCL membranes
Water contact angle measurements were performed at room temperature. Water droplets were dispensed from the needle of 1 mL syringe onto the membranes. The change of the droplet shape over time was recorded using a DSLR camera (Canon) and analyzed using ImageJ software (National Institutes of Health, USA) to determine the contact angle.
2.3. Characterization of mechanical properties
The tensile strength and suture retention strength of the membranes were evaluated using the Texture Analyser (TA.XTplus, Stable Micro Systems, UK). Specimens of 10 × 50 mm were prepared for each measurement.
The suture retention strength of the membranes was evaluated according to ISO 7198:2016. Since the membranes were aimed for vascular graft application, polypropylene 6–0 thread were used. The thread was passed through the membrane using the provided needle; the suture bite was centered with respect to the specimen width and was separated by at least 2 mm from the nearest edge.
2.4. Investigation on blood–material interaction
The study was conducted in compliance with the ethical regulation of the Institutional Review Board of Biomedical Engineering Department. The facilities for the complete blood count assay and blood coagulation assay were supported by the Clinical Laboratory of European Medical Center (Ho Chi Minh city). Fresh human whole blood was donated by three informed consented volunteers. Fresh blood was immediately put into tubes containing 3.2% w/v sodium citrate solution (blood: sodium citrate v/v ratio = 9:1) or tubes containing ethylenediaminetetraacetic acid (EDTA) to prevent blood clotting. The blood was used maximally 2 h after drawing to ensure efficiency.
2.4.1. Complete blood count assay
Change in whole blood cell count induced by CLA-grafted PU/PCL membranes was tested as followed. For each sample, 1 × 1 cm membrane was immersed in 2 mL of EDTA whole blood and incubated at 37°C for 1 h. After the incubation period, the sample was removed and the hematological parameters of the exposed blood were determined using an automated hematology analyzer (SYSMEX, XN-1000).
2.4.2. Blood clotting assay
The anticoagulant properties of the membranes were investigated using the method described previously [Citation28,Citation29]. 20 μL of recalcified blood (citrated whole blood contained 0.04 M Ca2+) was dropped on the surface of the samples. Glass coverslip, as it strongly initiates blood coagulation, was used as control. After predetermined periods of incubation time (5, 10, 20, 30, 40, 50 and 60 min) at 37°C, the specimens were immersed in 5 mL of distilled water for 10 min to lyse the uncoagulated erythrocytes and release hemoglobin. The concentration of free hemoglobin in distilled water (refer below as supernatant) was quantified by measuring its absorbance at 540 nm. The absorbance of 20 μL citrated whole blood in 5 mL distilled water was set to be 100 as a reference value. The anti-clotting index (ACI) of the samples was calculated as followed:
2.4.3. Anti-platelet adhesion property of CLA-grafted PU/PCL membrane
To obtain platelet-rich plasma (PRP), citrated whole blood was centrifuged at 1200 rpm for 5 min at room temperature without brake. Part of the PRP was then centrifuged at 3500 rpm for 10 min to get platelet-poor plasma (PPP). The platelet density in the suspension was adjusted to 105 cells/µl by mixing PRP and PPP in appropriate ratio.
Then for each sample, 200 µl (platelet number: 2 × 107) of the platelet suspension was loaded on its surface and incubated for 2 h at 37°C in static condition. After the incubation period, plasma and the weakly adhered platelets were rinsed off the membranes with phosphate-buffered saline (PBS) (pH 7.4). The rinsed samples with adhered platelets were fixed by immersion in 2.5 wt.% glutaraldehyde for 2 h at 37°C. Then PBS, 50% PBS, and distilled water were consecutively used to rinse the fixed samples. Finally, the samples were air-dried, and sputter-coated with gold prior to observation by SEM.
2.5. Investigation on cell–material interaction
2.5.1. Cell culture
Murine fibroblast L929 cells and Bovine artery endothelial cell (BAEC) were cultured in DMEM with 10% fetal bovine serum and 1% penicillin/streptomycin. The cells were maintained at 37°C with 5% CO2 atmosphere and subcultured when confluence reached 85-90%.
2.5.2. Cytotoxicity assay
Cytotoxicity of the extract of the membranes was evaluated on L929 cells by resazurin assay based on ISO 10993. Firstly, the cells were seeded at a density of 1 × 105 cells/1 mL and cultured for 24 h. Next, the extract solutions were prepared by immersing 6 cm2 sample/mL of media and incubated for 24 h at 37°C. The 100% extract and its dilutions were added to the cell-seeded wells and cultured for 24 h. Then, they were removed and the culture medium with resazurin (0.02 mg/ml) was added to each well and incubated for another 4 h. The fluorescence of the solutions was excited at 530 nm and the emitted signal was measured at 590 nm using microplate reader (VarioskanTM, ThermoScientific, USA). Signal of cells cultured on tissue culture plate was considered as 100% viability. Each sample was replicated 4 times.
2.5.3. Cell proliferation assay
Cell proliferation assays were conducted separately with two cell lines: L929 and BAEC. For each experiment, cells were seeded at a density of 2 × 104 cells/mL on the surface of 1 × 1 cm membranes then incubated at 37°C for four predetermined culture times (1, 3, 5 and 7 days).
For resazurin colorimetric assay, the membranes were moved to a new plate after its incubation period. Then, 750 μL of culture medium contained resazurin (0.02 mg/mL) was added to each well with the membranes and incubated for an additional 4 h. The solutions after the incubation were excited at a wavelength of 530 nm and their fluorescence signals were measured at 590 nm. For quantification purpose, the fluorescence intensity of the PU/PCL sample after day 1 was considered as 100% cell viability.
For SEM observation, the cellular constructs were collected and fixed using glutaraldehyde. Briefly, the constructs were rinsed thrice with PBS and subsequently fixed in 4% glutaraldehyde for 20 min. Next, they were washed twice with PBS to remove glutaraldehyde then dehydrated through a series of graded EtOH. Finally, cell-seeded membrane samples were air-dried at room temperature before SEM observation. Dry cellular constructs were sputter-coated with gold and studied by SEM (JSM-IT100, JEOL, Japan).
2.6. Statistical analysis
All experiments were done in triplicate, unless stated otherwise. Data were presented as average ± standard deviation and analyzed using SPSS Statistics software (IBM). Student’s t-test was used to compare means between two groups. For comparison between three or more groups, one-way ANOVA followed by Tukey-Kramer post-hoc test. Differences were regarded as significant if the p-value was <0.05.
3. Results
3.1. Effect of CLA grafting on physicochemical properties of PU/PCL membranes
3.1.1. Density of grafted-CLA
In this study, the density of CLA on the surface of membranes was quantified using de-esterification and UV-Vis spectroscopy. As shown in , variation in concentration of EDC leads to notable difference in CLA density. C2-E0 sample had negligible amount of CLA (less than 4 nmol/cm2). In carbodiimide-treated samples, the CLA densities were significantly higher than that of the physical coated samples, and they increased accordingly to the EDC amount. These densities of CLA were also similar to other studies that quantified CLA using other methods [Citation23,Citation24].
3.1.2. Effect on morphological properties and wettability of PU/PCL membrane
SEM images of the plasma-treated and CLA-grafted membranes are shown in ). Overall, there was no significant difference between the membranes in terms of fiber morphology and size. Similar to the initial PU/PCL membrane, all the samples had non-woven matrix of uniform nanofibers (diameter less than 1 µm). Thus, the result suggested that the plasma treatment and chemical graft had no notable effect to the morphology of the membrane as well as on the morphology of individual fibers.
Figure 2. (a) SEM images of the membranes. (b) Contact angle of the water droplet on the surface of the membranes. The images illustrate the contact angle closest to the mean value of its sample. *: p < 0.05
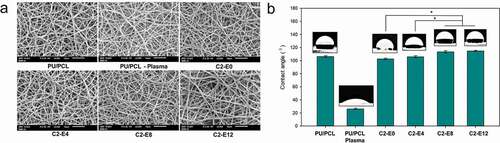
Wettability of the membranes was investigated by water contact angle assays ()). The PU/PCL sample was considerably hydrophobic with a contact angle of 106.6°. The plasma treatment effectively increased the hydrophilicity of the PU/PCL Plasma surface. Among the CLA-contained membranes, a slight rising trend in hydrophobicity was found along with the increase in CLA graft density. Contained the highest density of CLA, C2-E12 appeared to be the most hydrophobic sample with a contact angle of 114.7°.
3.1.3. Effect on mechanical properties of PU/PCL membrane
Mechanical behavior of the samples – including stress-strain behavior, tensile strength, yield stress and suture retention strength – were tested and compared to detect any alteration occurred after the plasma treatment and CLA graft ().
Table 2. Mechanical properties of membranes. Data were presented as average ± SD (n = 3)
These parameters of the saphenous vein and the internal mammary artery, the two blood vessels most commonly used as autologous graft in coronary artery bypass graft, were provided as reference. The difference between PU/PCL and treated membranes were negligible in all categories (p > 0.05). For instance, all membranes retained their elasticity against a stress up to 9 MPa. When exceeded this limit, the membranes demonstrate plastic behavior and deform irreversibly (Figure S1). The membranes withstand peak stress higher than 20 MPa before being torn, considerably higher than the autologous vessels. In terms of strain, the membranes were torn when they were deformed approximately 240% of their original length.
In clinical practice, an alternative blood vessel or bypass graft were usually attached to existing vessels using anastomosis techniques with suture. Thus, the suture retention strength of the material is also a critical factor deciding its usability in surgical intervention. As shown in , when tested with 6–0 polypropylene thread – suture specialized for vascular graft surgery, the samples displayed a suture retention strength of approximately 2.05 N and there was insignificant difference (p > 0.05) between treated and non-treated samples.
3.2. Effect of CLA grafting on blood–material interaction of PU/PCL membranes
3.2.1. Changes in hematological parameters after exposure to the membranes
Counting the main hematological parameters of human whole blood – before and after its exposure to the membranes – help determine whether blood cells adheres to the surface. ) presents the percentage of blood cells retained in whole blood after exposure to the membranes compared to unexposed blood. There were no significant differences in the amount of white blood cells and red blood cells after the samples were immersed in blood (p > 0.05). In contrast, a definite difference was detected in the changes of platelets. Human whole blood lost approximately 5% of its platelets after being subjected to the non-grafted membrane and C2-E0 whereas C2-E8 and C2-E12 had negligible effect on the platelets of exposed blood. The differences in this parameter between these two samples and PU/PCL and C2-E0 were statistically significant (p < 0.05).
3.2.2. Clotting behavior of blood exposed to the membranes
Result of the conducted blood clotting test reflects the antithrombotic activity of the target biomaterial. ACI value of 100 – inferred from fresh whole blood in distilled water – is defined as no clotting. A larger ACI value represents an improvement in anticoagulation.
) shows the change of ACI value of each sample with respect to sample-blood contact time. Overall, sample with higher density of CLA generated slower decline of ACI, or slower blood clotting. As a coagulation-initiating surface, glass surface exhibited a rapid reduction of ACI. C2-E0 displayed antithrombotic activity similar to the activity of the plain PU/PCL membrane. After 5 min of exposure, both samples demonstrated notable thrombogenicity, with ACI of 64.4 and 67.1, respectively. In comparison, membranes grafted with CLA effectively mitigated the clotting of blood exposed to their surface with ACI higher than 90.
After that, ACI values of the samples decreased gradually with the incubation time as the blood clotting occurred. After 50 min, blood initiated by glass was clotted significantly with an ACI of 8.2. The minor CLA content of C2-E0 has a negligible effect on blood clotting after extended period, as the ACI of C2-E0 and PU/PCL converged to comparable values of approximately 36.5. The index of the three CLA-grafted samples decreased accordingly to the decreasing order of CLA density (C2-E12 > C2-E8> C2-E4). Notably, C2-E8 and C2-E12 preserved blood in liquid state effectively as their ACI were higher than 75 after 50 min of incubation. Thus, it can be deduced that CLA grafting is a promising approach to lower blood clotting rate.
3.2.3. Anti-platelet adhesion properties of the membranes
Interaction of the membranes with platelet in platelet-rich-plasma was investigated under static condition. As seen in ), there were activated platelets adhered to the surface of PU/PCL. A few inactivated platelets (marked by dashed circle) in their spherical shape were also identified.
By contrast, activated platelets were not present on the surface of samples contained CLA. An abundance of platelets adhered to surface fibers of C2-E0, but they retained their dormant spherical shape. Compared to these two samples, the CLA-grafted membranes indicated superior anti-adhesion properties against platelets. Adhered thrombocytes were virtually not detected on all three samples C2-E4, C2-E8 and C2-E12. However, unidentified fibrous materials, which can be attributed to the formation of fibrin, were observed on the surface of C2-E4, as well as on the surface of PU/PCL and C2-E0.
3.3. Effect of CLA grafting on cell–material interaction of PU/PCL membranes
3.3.1. In vitro biocompatibility of the membranes
Biocompatibility of the membranes was examined based on the guidelines of ISO 10993–5. ) shows the percentage of cell viability of all samples according to the extract concentration. All samples reached viability of 100%, indicated a highly biocompatible material. As suggested by ISO, a material that yielded cell viability higher than 70% at 100% concentration of extract is biocompatible and can be investigated for further application in human.
Figure 4. (a) Cell viability in different concentrations of extract solution of PU/PCL membranes. (b) SEM images of seeded cells proliferated on the surface of PU/PCL and C2-E8 after 7 days of incubation, (c) Proliferation of L929 fibroblast cells on the surface of PU/PCL membranes. (d) Proliferation of BAEC cells on the surface of PU/PCL membranes., #, a: Label of statistical significance (p < 0.05). Difference between samples with the same label is not statistically significant
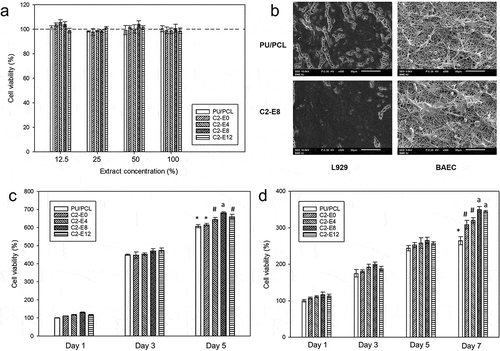
3.3.2. Proliferation of fibroblasts and endothelial cells on surface of PU/PCL membranes
As two main types of cell in the structure of blood vessels, direct interaction of fibroblast (located in the adventitia layer of natural blood vessel) and endothelial cells (intima layer) with the material surface is critical in vascular tissue engineering. Therefore, this study cultured and investigated the proliferation of murine fibroblasts and bovine arterial endothelial cells directly on the electrospun membranes.
Viability of fibroblasts cultured on the surface of the membranes after 1, 3 and 5 days was shown in ). In general, all samples were favorable for proliferation of fibroblasts. On day 3, all samples yielded similar cell viabilities of higher than 450%, illustrated significant growths in cell confluence. After 5 days of culturing, the cells proliferated extensively. From C2-E0 to C2-E8, the viability value increased with the increase in the CLA density, with C2-E8 yielded 681%. However, this trend discontinued as the viability of C2-E12 dropped to approximately 660%. Data of day 7 were omitted since the cells reached maximum confluence and stop proliferating.
Due to difference in cell mitosis cycle, the BAEC proliferated at a slower pace compared to the murine fibroblasts ()). From day 1 to day 5, cells on all samples proliferate gradually, reaching viability of approximately 180% after 3 days and 250% on day 5. Moreover, there was no clear dissimilarity between the samples. However, the obtained viability values differed notably after 7 days of culturing. Similar to the proliferation of L929 cell lines, there was also an increase in cell proliferation according to the increase of CLA content. C2-E8 demonstrated a viability of 349%, significantly higher than which of C2-E0 and C2-E4. Similar to the proliferation of L929 cells, the viability value of C2-E12 was lower, interrupted the rising trend.
In accordance with the prior quantitative data, the SEM images demonstrated the same trend where the presence of CLA enhanced cell proliferation ()). After 7 days, L929 fibroblasts proliferated strongly to completely mask the surface of CLA-grafted membranes. In PU/PCL membrane, the cells also proliferated, but there were still uncovered areas on the surface. With BAEC, the difference between the samples was less significant. At day 7, there were slight differences in the number of cells whereas the cells exhibited comparable morphology. The slower proliferation of BAEC was also observed with SEM.
4. Discussion
In this study, we prepared a membrane from PU/PCL using electrospinning then chemically grafted it with CLA. As an anticoagulant fatty acid with complex bioactivity, the presence of CLA is expected to improve the antithrombotic property of the membranes – a crucial factor to prevent occlusion in vascular graft. From the result, it is suggested that the grafting was effective and the density of CLA increased with the concentration of EDC. With the highest amount of EDC used in this study, C2-E12 reached a CLA density of approximately 15% of the hypothetical maximum. This constraint might arise from the esterification rate and the limit of introduced hydroxyl -OH group. Moreover, as C2-E0 has a low amount of CLA, we deduced that CLA could physically adsorbed to the surface of PU/PCL membranes. Owing to its activity, the variation in CLA density could considerably alter the other properties of the membranes. Thus, this quantification is the basis of this research and it was used to rationalize later findings.
Complications in clinical uses of synthetic vascular grafts can result from mismatch in material behavior [Citation11,Citation32]. Mismatch at the anastomosis, especially in mechanical properties is a contributing factor to formation of thrombus and endothelial hyperplasia. Moreover, surface morphology can have a notable effect on mechanical strength, wettability and blood interaction behavior (especially interaction with platelets) of a material [Citation33–Citation35]. Thus, the ideal antithrombotic approach should not alter the native morphology of the base material. We characterized the physical properties of the membranes to detect undesirable changes. For this purpose, the plasma procedure was previously optimized, and the grafting procedure did not include destructive chemicals. As observed by SEM, there were no significant differences in the morphology between non-treated and treated PU/PCL samples. Moreover, the membranes showed typical electrospun structures with uniform nanofibers and even pores. This architecture was also shown to be advantageous compared with solvent casting morphology in terms of anti-adhesion and anti-aggregation of platelet or endothelial cell interaction [Citation36,Citation37].
CLA is an unsaturated fatty acid with hydrophilic carboxyl head and hydrophobic alkenyl tail. As the hydrophilic head formed ester bond with the surface, the alkenyl tail of CLA was expected to reduce the wettability of the membranes. Result of the water contact angle assay confirmed this assumption, but the effect was negligible. Although the CLA was not grafted on the surface of C2-E0, the minor presence of the fatty acid and multiple wash also diminished the effect of plasma treatment and reduced the wettability of this sample. Since hydrophobicity plays an important role in determining the adhesion of protein and platelets to a surface, this change could also be a contributing factor to the differences between samples in blood-material and cell–material interaction [Citation38].
The mechanical properties of the membrane were not affected after the graft, and they were in accordance or superior to other reported studies on PCL composite vessels [Citation39,Citation40]. Compare to the autologous blood vessels usually used as bypass graft, the electrospun membranes showed higher tensile strength and strain threshold. Moreover, suture retention strength of the membrane against the typical thread satisfied the recommended threshold for clinical application. As recommended by ISO 7198:2016, a strength of 2 N is sufficient to avoid break or tear of the graft during the surgery. There are other essential mechanical parameters that need to be examined such as burst strength and water leakage. However, since the study did not investigate the tubular form of the material, these characteristics were out of its scope.
As mentioned, CLA was grafted on the surface of the membrane to enhance its antithrombotic activity. Thus, coagulating behavior of human whole blood and its components (PRP and PPP) before and after being exposed to the membranes were examined. As a result, the treatment was effective in preventing or hampering blood coagulation. When subjected to human whole blood, the membranes containing CLA effectively prevented the adhesion of platelets and slowed the coagulating kinetic of blood. The deceleration shown by the clotting assay was also commensurate with the CLA amount of each sample, suggesting the antithrombotic activity of CLA on the surface.
When exposed to PRP, membranes contained CLA had less activated and adhered platelets. Thus, it is suggested that the presence of CLA effectively prevents their activation and adhesion to a target surface. However, the antiplatelet mechanism of CLA was not thoroughly understood. As shown by Truitt et al. [Citation41], this effect of CLA might be due to the resemblance of its structure with the structure of arachidonic acid (AA). Thanks to this similarity, CLA could be the competitive inhibitor of AA and stop the cyclooxygenase cascade. Thus, the antiplatelet adhesion property of the PU/PCL could come from both released CLA (in free fatty acid structure) and CLA attached on the surface (in esterified structure) as shown by the studies of Kung et al. [Citation24].
Since the source materials are highly biocompatible, the final grafted membrane showed no sign of cytotoxicity regardless of CLA density. On the other hand, this parameter had a notable effect on the proliferation of cells on the surface of the membranes. The effect was especially significant against endothelial cells, where increasing density of CLA positively support the cells’ growth. However, cells cultured on top of C2-E12 sample demonstrated slower proliferation compared to the cells of C2-E8. This phenomenon was observed consistently through all culture periods, even though the difference between the samples was less notable in earlier days. SEM images also revealed that CLA might have positive effect on adhesion of the cells. The same phenomenon was also observed with L929 fibroblast cell lines as the cells cultured on C2-E8 had the highest proliferative rate. This discontinuity of the rising trend could suggest an optimal density of CLA; a CLA density exceeds this limit could in turn restrain normal behavior of cells, either impede their proliferation or prevent the initial adhesion. CLA is known as an inhibitor toward cancer cells [Citation42,Citation43]. However, other studies regarding the effect of CLA on normal human cells have come to different conclusions. For instance, Belda et al. demonstrated a decrease in proliferation of fibroblasts treated with CLA [Citation44], whereas Donnelly et al. found no significant alteration in treated HeLa cells and fibroblasts [Citation45]. Thus, further investigations are needed to rationalize the effect of CLA on proliferation of cells, considering its release from the surface. In this research, C2-E8 was indicated as the optimal samples with the most supportive cell–material interaction and reasonable antithrombotic activities.
5. Conclusions
This study has reported the electrospinning and the chemical modification of PU/PCL membrane for vascular tissue engineering application. Except a slight decrease in surface wettability, the grafting of CLA on the surface of PU/PCL caused no notable alteration to the surface morphology and mechanical properties of the membranes. Tensile strength, yield stress and suture retention strength of CLA-grafted membranes satisfied the requirements for a clinically applicable artificial vascular graft. When contact directly with whole blood or plasma, membranes grafted with CLA exhibited antithrombotic activity commensurate to its CLA density. Gathered data suggested that the presence of CLA lengthened clotting dynamic of exposed blood, mitigated platelet activation and adhesion, as well as impeded the coagulation cascade on both pathways. The samples had excellent biocompatibility and supported the proliferation of fibroblasts and endothelial cells. However, it was shown that CLA increased cell proliferation up to a certain optimal density; this positive effect diminished when the density of CLA surpassed this limit. To conclude, CLA-grafted PU/PCL membrane is verified to be a biocompatible and actively anti-thrombotic material with satisfying physicochemical properties worthy of investigating further for the purpose of vascular tissue engineering. Deeper examinations of the material in its tubular form and in dynamic condition are the next steps to confirm its potential as a small-diameter vascular graft.
Supplemental Material
Download PDF (69.2 KB)Disclosure statement
No potential conflict of interest was reported by the authors.
Supplementary material
Supplemental data for this article can be accessed here.
Additional information
Funding
References
- Zoghbi WA, Duncan T, Antman E, et al. Sustainable development goals and the future of cardiovascular health: a statement from the global cardiovascular disease taskforce. J Am Coll Cardiol. 2014;64:1385–1387.
- Abdulhannan P, Russell D, Homer-Vanniasinkam S. Peripheral arterial disease: a literature review. Br Med Bull. 2012;104(1):21–39.
- Klinkert P, Post P, Breslau P, et al. Saphenous vein versus PTFE for above-knee femoropopliteal bypass. A review of the literature. Eur J Vasc Endovascular Surg. 2004;27(4):357–362.
- Taylor LM, Edwards JM, Brant B, et al. Autogenous reversed vein bypass for lower extremity ischemia in patients with absent or inadequate greater saphenous vein. Am J Surg. 1987;153(5):505–510.
- McKenna KA, Hinds MT, Sarao RC, et al. Mechanical property characterization of electrospun recombinant human tropoelastin for vascular graft biomaterials. Acta Biomater. 2012;8(1):225–233.
- Steinhoff G, Stock U, Karim N, et al. Tissue engineering of pulmonary heart valves on allogenic acellular matrix conduits: in vivo restoration of valve tissue. Circulation. 2000;102(suppl_3):Iii-50-Iii-55.
- Chlupáč J, Filova E, Bačáková L. Blood vessel replacement: 50 years of development and tissue engineering paradigms in vascular surgery. Physiol Res. 2009;58(Suppl 2):S119–139.
- Brewster DC. Current controversies in the management of aortoiliac occlusive disease. J Vasc Surg. 1997;25(2):365–379.
- Hehrlein F, Schlepper M, Loskot F, et al. The use of expanded polytetrafluoroethylene (PTFE) grafts for myocardial revascularization. J Cardiovasc Surg (Torino). 1984;25(6):549–553.
- Wise SG, Byrom MJ, Waterhouse A, et al. A multilayered synthetic human elastin/polycaprolactone hybrid vascular graft with tailored mechanical properties. Acta Biomater. 2011;7(1):295–303.
- Hasan A, Memic A, Annabi N, et al. Electrospun scaffolds for tissue engineering of vascular grafts. Acta Biomater. 2014;10(1):11–25.
- Stegemann JP, Kaszuba SN, Rowe SL. Review: advances in vascular tissue engineering using protein-based biomaterials. Tissue Eng. 2007;13(11):2601–2613.
- Xiang P, Li M, Zhang C, et al. Cytocompatibility of electrospun nanofiber tubular scaffolds for small diameter tissue engineering blood vessels. Int J Biol Macromol. 2011;49(3):281–288.
- Kim J, Kim S. PEO-grafting on PU/PS IPNs for enhanced blood compatibility – effect of pendant length and grafting density. Biomaterials. 2002;23(9):2015–2025.
- Williamson MR, Black R, Kielty C. PCL–PU composite vascular scaffold production for vascular tissue engineering: attachment, proliferation and bioactivity of human vascular endothelial cells. Biomaterials. 2006 Jul 01;27(19):3608–3616.
- Guo H-F, Dai -W-W, Qian D-H, et al. A simply prepared small-diameter artificial blood vessel that promotes in situ endothelialization. Acta Biomater. 2017 May 01;54(Supplement C):107–116.
- Ishihara K, Liu Y, Inoue Y. 11 - Enhancing polyurethane blood compatibility. In: Cooper SL., Guan J, editors. Advances in polyurethane biomaterials. Woodhead Publishing; 2016. p. 319–348.
- de Valence S, Tille J-C, Mugnai D, et al. Long term performance of polycaprolactone vascular grafts in a rat abdominal aorta replacement model. Biomaterials. 2012;33(1):38–47.
- Nguyen T-H, Padalhin AR, Seo HS, et al. A hybrid electrospun PU/PCL scaffold satisfied the requirements of blood vessel prosthesis in terms of mechanical properties, pore size, and biocompatibility. J Biomater Sci Polym Ed. 2013;24(14):1692–1706.
- Abdal-hay A, Bartnikowski M, Hamlet S, et al. Electrospun biphasic tubular scaffold with enhanced mechanical properties for vascular tissue engineering. Mater Sci Eng C. 2018;82:10–18.
- Minh HH, Hiep NT, Hai ND, et al. Fabrication of polycaprolactone/polyurethane loading conjugated linoleic acid and its antiplatelet adhesion. Int J Biomater. 2017;2017:1–7.
- Yu L, Adams D, Gabel M. Conjugated linoleic acid isomers differ in their free radical scavenging properties. J Agric Food Chem. 2002;50(14):4135–4140.
- Kung FC, Yang MC. Effect of conjugated linoleic acid grafting on the hemocompatibility of polyacrylonitrile membrane. Polym Adv Technol. 2006;17(6):419–425.
- Kung F-C, Yang M-C. Effect of conjugated linoleic acid immobilization on the hemocompatibility of cellulose acetate membrane. Colloids Surf B Biointerfaces. 2006;47(1):36–42.
- Kung F-C, Yang M-C. The effect of covalently bonded conjugated linoleic acid on the reduction of oxidative stress and blood coagulation for polysulfone hemodialyzer membrane. Int J Biol Macromol. 2006;38(3–5):157–164.
- Aveldano MI, Horrocks LA. Quantitative release of fatty acids from lipids by a simple hydrolysis procedure. J Lipid Res. 1983;24(8):1101–1105.
- van den Berg JJ, Cook NE, Tribble DL. Reinvestigation of the antioxidant properties of conjugated linoleic acid. Lipids. 1995;30(7):599–605.
- Meng Z, Zheng W, Li L, et al. Fabrication and characterization of three-dimensional nanofiber membrance of PCL–MWCNTs by electrospinning. Mater Sci Eng C. 2010;30(7):1014–1021.
- Liu Y, Yang Y, Wu F. Effects of L-arginine immobilization on the anticoagulant activity and hemolytic property of polyethylene terephthalate films. Appl Surf Sci. 2010;256(12):3977–3981.
- Stekelenburg M, Rutten MC, Snoeckx LH, et al. Dynamic straining combined with fibrin gel cell seeding improves strength of tissue-engineered small-diameter vascular grafts. Tissue Eng Part A. 2008;15(5):1081–1089.
- L’Heureux N, Dusserre N, Konig G, et al. Human tissue-engineered blood vessels for adult arterial revascularization. Nat Med. 2006;12(3):361.
- Gong W, Lei D, Li S, et al. Hybrid small-diameter vascular grafts: anti-expansion effect of electrospun poly ε-caprolactone on heparin-coated decellularized matrices. Biomaterials. 2016;76:359–370.
- Szewczyk P, Ura D, Metwally S, et al. Roughness and fiber fraction dominated wetting of electrospun fiber-based porous meshes. Polymers. 2019;11(1):34.
- Zingg W, Neumann A, Strong A, et al. Effect of surface roughness on platelet adhesion under static and under flow conditions. Can J Surg J Canadien De Chirurgie. 1982;25(1):16–19.
- Fidelis MEA, Pereira TVC, Gomes O, et al. The effect of fiber morphology on the tensile strength of natural fibers. J Mater Res Technol. 2013;2(2):149–157.
- Ahmed F, Choudhury NR, Dutta NK, et al. Interaction of platelets with poly (vinylidene fluoride-co-hexafluoropropylene) electrospun surfaces. Biomacromolecules. 2014;15(3):744–755.
- Ahmed F, Roy Choudhury N, Dutta NK, et al. Fabrication and characterisation of an electrospun tubular 3D scaffold platform of poly (vinylidene fluoride-co-hexafluoropropylene) for small-diameter blood vessel application. J Biomater Sci Polym Ed. 2014;25(18):2023–2041.
- Wu Y, Simonovsky FI, Ratner BD, et al. The role of adsorbed fibrinogen in platelet adhesion to polyurethane surfaces: a comparison of surface hydrophobicity, protein adsorption, monoclonal antibody binding, and platelet adhesion. J Biomed Mater Res A. 2005;74(4):722–738.
- Lee SJ, Liu J, Oh SH, et al. Development of a composite vascular scaffolding system that withstands physiological vascular conditions. Biomaterials. 2008;29(19):2891–2898.
- He W, Ma Z, Teo WE, et al. Tubular nanofiber scaffolds for tissue engineered small‐diameter vascular grafts. J Biomed Mater Res A. 2009;90(1):205–216.
- Truitt A, McNeill G, Vanderhoek JY. Antiplatelet effects of conjugated linoleic acid isomers. Biochim Biophys Acta (BBA)-Mol Cell Biol Lipids. 1999;1438(2):239–246.
- Scimeca JA, Thompson HJ. Conjugated linoleic acid. A powerful anticarcinogen from animal fat sources. Cancer. 1994;74(S3):1050–1054.
- Kelley NS, Hubbard NE, Erickson KL. Conjugated linoleic acid isomers and cancer. J Nutr. 2007;137(12):2599–2607.
- Belda B, Heuvel JV. Conjugated linoleic acid effects on adipose differentiation and its potential interactions in breast cancer. AACR. 2007;67(9):2783–2783.
- Donnelly C, Olsen AM, Lewis LD, et al. Conjugated linoleic acid (CLA) inhibits expression of the Spot 14 (THRSP) and fatty acid synthase genes and impairs the growth of human breast cancer and liposarcoma cells. Nutr Cancer. 2008;61(1):114–122.