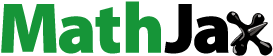
ABSTRACT
Poly(3-hexylthiophene) (P3HT) is a typical conducting polymer widely used in organic thin-film transistors, polymer solar cells, etc., due to good processability and remarkable charging carrier and hole mobility. It is known that the ordered structure assembled by π-conjugated P3HT chains could promote the performance of electronic devices. Interfacial and confined molecular-assembly is one effective way to generate an ordered structure by tuning surface geometry and substrate interaction. Great efforts have been made to investigate the molecular chain assembly of P3HT on a curved surface that is confined to different geometry. In this report, we review the recent advances of the interfacial chain assembly of P3HT in a flat or curved confined space and its application to organic electronic devices. In principle, this interfacial assembly of P3HT at a nanoscale could improve the electronic properties, such as the current transport, power conversion efficiency, etc. Therefore, this review on interfacial and confined assembly of P3HT could give general implications for designing high-performance organic electronic devices.
1. Introduction
Chain orientation and packing of π-conjugated polymers greatly affect the properties of functional electronic devices. Poly(3-hexylthiophene) (P3HT) is a common conducting polymer and has been widely used in function electronic devices [Citation1–12]. The main backbone of P3HT is composed of thiophene rings and the side group is short alkyl chains. Two main forms exist in thiophene polymers (form I, a = 1.6 nm, b = 0.77 nm, c = 0.76 nm, α =β = 90°, γ = 87°; form II, a = 1.076 nm, b = 0.777 nm, c = 0.944 nm, α = γ = 90°, β = 64.66°) [Citation13,Citation14].
Confinement is one of the efficient methods to manipulate the chain-assembly at a molecular level. Both the geometry of confined space and interfacial interaction plays an important role in assembling the ordered structure of functional molecular chains [Citation15–30]. A lot of research have reported novel nanostructures like nanotwin or coherent crystal branching and so on could be produced under cylindrical confinement. Meanwhile, the π-π stacking orientation of a conducting polymer is dependent on the interaction between the conductive polymer and the substrate. Furthermore, P3HT could be fabricated into several kinds of nanostructures, such as nanotubes, nanowires, thin films and 2D-sandwich nanocrystals [Citation31–49]. It has been found that in the ordered and well-aligned chain-assembling structure of P3HT nano-devices, the hole and the charge carrier mobility transport is more efficient [Citation50–60].
In this report, we review the recent advances in interfacial and confined chain-assembly of P3HT nanostructure in different types of substrate and its application in functional and organic electronic devices, such as organic field effect transistors (OFET), organic photovoltaic (OPV), organic light-emitting diodes (OLED) and etc [Citation57,Citation61–80]. The effect of temperature, confined spatial geometry, substrate surface, and interface on the functional P3HT nanomaterial has been described below:
2. π-π stacking and chain orientations of P3HT on the substrate
According to Agbolaghi et al., there are three main π–π stacking orientations of the lamellar in P3HTgenerated on the substrate as shown in : the edge-on orientation (the thiophene-backbone plane is vertical to the substrate), the face-on orientation (the thiophene-backbone plane is parallel to the substrate), the flat-on orientation (the thiophene-backbone plane, as well as the molecular chain orientation, are vertical to the substrate) [Citation81–83].
3. Interfacial assembly of P3HT and its application in functional electronic devices
3.1. The rubbing effect on the lamellar orientation of P3HT in thin film
Hamidi-Sakr et al. investigated the crystal orientation of regioregular P3HT thin films prepared by mechanical rubbing at a temperature in the range 144–217℃ [Citation82]. display the alternated black and white line morphology of thin films of P3HT prepared by mechanically rubbing at 144℃ and 217℃, respectively, indicating that periodic lamellar morphology is formed and becomes more regular at high temperatures. The lamellar period, l, turns to be bigger when increasing the rubbing temperature. L improved from 13 to 26 nm when the temperature changes from 144℃ to 217℃. The selected-area electron diffraction patterns of transmission electron microscopy (TEM) of P3HT thin film crystals prepared at 144℃ and 217℃ are displayed in , respectively. According to the literature report, the (020) reflection at the equator stands for the edge-on lamellae orientation, while the equatorial reflection of (h00) represents the face-on orientation. The schematic illustration of the crystal orientation change in rubbed P3HT film with respect to the temperature is shown in . Below 98℃, the films exhibited a highly strained smectic-like phase with the thiophene backbones extending along the rubbing direction, implying the face-on orientation has been formed. But when the rubbing temperature was increased from 140 to 217℃, both the reflections and the 020 reflection are located at the equator. Therefore, both face-on and edge-on orientations coexist in the 144–217℃.
Figure 2. TEM bright-field images of the oriented P3HT films prepared by mechanically rubbing at (a) 144 ℃ and (b) 217 ℃, respectively. ED patterns of P3HT films when rubbed at (c) 144 ℃, (d) 217 ℃; (e) the curves of hole mobility in bottom-gate/bottom-contact OFETs made by rubbing P3HT films. μ// and μ⊥ represents the hole mobility is measured by parallel or perpendicular to the rubbing direction; (f) schematic illustration of the lamellar orientation’s changes with respect to the temperature. Reprinted from Ref. [Citation82] with permission. Copyright 2016.
![Figure 2. TEM bright-field images of the oriented P3HT films prepared by mechanically rubbing at (a) 144 ℃ and (b) 217 ℃, respectively. ED patterns of P3HT films when rubbed at (c) 144 ℃, (d) 217 ℃; (e) the curves of hole mobility in bottom-gate/bottom-contact OFETs made by rubbing P3HT films. μ// and μ⊥ represents the hole mobility is measured by parallel or perpendicular to the rubbing direction; (f) schematic illustration of the lamellar orientation’s changes with respect to the temperature. Reprinted from Ref. [Citation82] with permission. Copyright 2016.](/cms/asset/460fba58-ddbf-4abf-aa2f-8910ec9ae2d9/tsta_a_2125826_f0002_oc.jpg)
Kajiya and Saitow et al. have investigated the effect of rubbing on the photoconductivity of three P3AT-based compounds: P3DDT, P3HT and P3BT with different alkyl side chain length which are –C12H25, –C6H13 and –C4H9 respectively. It is obvious that the film rubbing has significantly improved the out-of-plane mobility of the three P3AT-based samples that were identified by polarized electronic absorption spectroscopy and 2D grazing-incidence X-ray diffraction [Citation84,Citation85]. On the other hand, when the P3HT was applied in organic solar cell, they found that the out of plane mobility was 8 orders enhanced by rubbing in low regularity P3HT samples. This is because the more amount of face-on orientation has been formed during the rubbing process and thus more π−π stacking orientation of P3HT inclined to be the out-of-plane direction instead of in-plane direction.
In summary, the lamellar stacking orientation of P3HT can be manipulated by mechanically rubbing the films at different temperatures. Furthermore, besides the temperature impact of the packing structure, other factors such as molecular weight, the solvent used for the film preparation and etc. also affect the lamellar orientation [Citation78,Citation83,Citation86–91].
3.2. Interfacial interaction and confinement effect on the molecular assembly on the (reduced graphene oxide) RGO surface
Agbolaghi et al. investigated that the thiophene rings of P3HT interact with the bared surface of RGO, causing a conventional face-on orientation. In this case, the molecular chains of P3HT are parallel to the RGO surface. For comparison, the functionalized-RGO could interact with the side chains of P3HT and therefore generate edge-on orientations. Meanwhile, the researchers also noticed that with increasing the crystallization time, the fibrils of P3HT tend to be parallel to the bared-RGO surface. And under these circumstances, the chain orientations are perpendicular to the RGO surface normal [Citation92].
describe the TEM bright-field (BF) image of P3HT crystals grown on the RGO surface for 6 h and 18 h, respectively. display the [010] and [100] zone electron diffraction patterns of P3HT crystals that are grown on the RGO surface, respectively. show the grazing-incidence wide-angle X-ray diffraction (GIWAXD) patterns of P3HT crystals isothermally crystallized on the RGO surface for 6 hrs and 18 hrs, respectively. Both electron diffraction (ED) patterns and GIWAXD patterns show that b-axis ((010) direction) in the face-on assemblies is oriented normal to the RGO surface when the P3HT is crystallized on the RGO surface in a shorter time (6 hours). On the other hand, a-axis ((100) direction) in the edge-on assemblies is perpendicular to the RGO surface when the P3HT is crystallized on the RGO surface for a longer time (18 hours). The reason for the change of lamellar orientation in P3HT with respect to the crystallization time can be described as follows: At the initial stage of crystallization of P3HT on the RGO surface, the interactions between the thiophene-rings and phenyl-rings of RGO favor both the plane of thiophene-ring and molecular chains inP3HT are parallel to the RGO surface. Therefore, the π-π stacking orientations are directed along the surface normal and face-on orientation is generated. However, for the later stage (longer time) in crystallization, the 1D confinement imposed by the thin film’s thickness on the RGO surface prevails, and the fast growth direction (the b-axis) of P3HT fibrils is aligned perpendicular to the surface normal and thus induces an edge-on orientation. In this case, the plane of the thiophene-ring is perpendicular to the RGO surface.
Figure 3. TEM BF images for P3HT crystals grown on the graphene in toluene about (a) 6 h, (b) 18 h; (c) selected-area electron diffraction pattern of the P3HT crystals (face-on) grown on the graphene about 6 h; and (d) selected-area electron diffraction pattern of the P3HT crystals (edge-on) grown on the graphene about18 h; GIWAXDpatterns of P3HT on grown intoluene about (e) 6 h, (f) 18 h; schematic illustration of P3HT crystals with (g) face-on and (h) edge-on orientation on the graphene substrate. Reprinted from Ref. [Citation92] with permission. Copyright 2018.
![Figure 3. TEM BF images for P3HT crystals grown on the graphene in toluene about (a) 6 h, (b) 18 h; (c) selected-area electron diffraction pattern of the P3HT crystals (face-on) grown on the graphene about 6 h; and (d) selected-area electron diffraction pattern of the P3HT crystals (edge-on) grown on the graphene about18 h; GIWAXDpatterns of P3HT on grown intoluene about (e) 6 h, (f) 18 h; schematic illustration of P3HT crystals with (g) face-on and (h) edge-on orientation on the graphene substrate. Reprinted from Ref. [Citation92] with permission. Copyright 2018.](/cms/asset/06926483-b370-49a8-979a-f22912ff6ec4/tsta_a_2125826_f0003_oc.jpg)
Another important issue is that the interfaction interaction between the conjugated polymers and substrate could affect the chain conformations and its packing structure in P3HT aggregation. It is known that for conjugated polymers, H- and J-aggregates are composed of the extended chain and folded chain, respectively. And the microstructure of the lamellar aggregates could affect the hole mobility and thus the device performance [Citation93,Citation94]. Normally, the aggregate of conjugated polymer is a mixture of H- and J-aggregate. Recently, an ultrapure 99.8% H-aggregate polymer can be obtained by casting a droplet of P3HT solution on a low-wettability substrate at room temperature. The hole mobility of nearly pure H-aggregate is a 6-fold increase compared to a common P3HT film with a mixture of H- and J-aggregate.
In addition, when using the solution crystallization process to prepare P3HT films, the solvent selection of the solution is an important factor affecting the film structure. Recently, many studies have reported the effect of solvent on the molecular orientation of conjugated thin films [Citation95,Citation96]. It has been found that the molecular chain orientation of P3HT in perovskite films for solar cells can be manipulated by using organic solvents with specific volatile properties.
3.3. P3HT chains/nanosheets self-assembling at the air/liquid interface
To achieve highly efficient charge transport in organic electronic devices, the long-range ordering structure of π-conjungated polymer crystals is desirable (). Chaudhary et al. prepared a uniform thin film of P3HT/MoS2 via surface-tension gradient and compressive force. The thin film is formed by casting the solution containing the ultrasonicated P3HT/MoS2 nanocomposite onto the liquid surface of a glycerol and ethylene glycol mixture shown in [Citation97]. The phase morphology of pristine P3HT () and P3HT/MoS2 (1%) nanocomposite () has been studied by atomic force microscopy (AFM). It is obvious that compared to the phase image of pristine P3HT, long fibril crystals are formed and aligned almost in one direction as shown in the phase images of P3HT/MoS2 (1%) nanocomposite and MoS2 nanosheets are also included in the thin film. The well-aligned and ordered structure of P3HT fibril crystals and MoS2 nanosheets are generated by the surface tension gradient between the polymer solution and liquid substrate ()).
Figure 4. AFM phase image of (a) pristine P3HT and (b) P3HT/MoS2 (1%); (c) powder XRD and (d) GIWAXD pattern of pure P3HT and P3HT/MoS2 with various ratios (1%, 2% and 3%); (e) transfer characteristics of pristine P3HT, sonicated P3HT and P3HT/MoS2 (1%, 2% and 3%) OFETs with standard deviations; (f) schematic illustration of OFET devices made by P3HT/nanosheets; (g) fabrication process of P3HT/MoS2 nanocomposite under ultrasonic treatment; (h) edge-on orientation of P3HT/MoS2 nanocomposite. Reprinted from Ref. [Citation97] with permission. Copyright 2020.
![Figure 4. AFM phase image of (a) pristine P3HT and (b) P3HT/MoS2 (1%); (c) powder XRD and (d) GIWAXD pattern of pure P3HT and P3HT/MoS2 with various ratios (1%, 2% and 3%); (e) transfer characteristics of pristine P3HT, sonicated P3HT and P3HT/MoS2 (1%, 2% and 3%) OFETs with standard deviations; (f) schematic illustration of OFET devices made by P3HT/nanosheets; (g) fabrication process of P3HT/MoS2 nanocomposite under ultrasonic treatment; (h) edge-on orientation of P3HT/MoS2 nanocomposite. Reprinted from Ref. [Citation97] with permission. Copyright 2020.](/cms/asset/2bdf5d48-a642-4d61-a6e4-fec93b7c9bbd/tsta_a_2125826_f0004_oc.jpg)
The ordered structure of P3HT/MoS2 (1%) nanocomposite has been examined through GIWAXD and X-ray powder diffraction experiments ()). The equatorial (020) reflection represents the edge-on orientation of P3HT crystals formed on the substrate in P3HT/MoS2 (). As for the charge transport properties in the P3HT/nanocomposites, the typical drain current/back-gate voltage (IDS-VDS) curves indicate it has a p-type charge transport (). The drain current of P3HT/MoS2 is larger than that of pristine P3HT. The P3HT/MoS2 (1%) possessed the maximum degree of electronic mobility because the edge-on orientation brings in the lowest exciton bandwidth and longest conjugation length compared to other samples. In addition, when increasing the amount of MoS2, the electron mobility decreases. Because a large number of nanosheets prohibits the growth of P3HT fibril crystals and thus, the length of fibril crystals is reduced [Citation98,Citation99].
Furthermore, according to the literature, the hole mobility and density of Si-nanocrystal/P3HT hybrid film have been greatly increased about 50- and 12-fold of the pristine P3HT, respectively, due to higher crystallinity of hybrid P3HT film induced by homogeneous distribution Si-nanocrystals. The hole moves to be parallel to the out-of-plane direction of Si-nanocrystal/P3HT film or film surface normal. Meanwhile, the inorganic Si-nanocrystals can improve film stability [Citation100].
The Langmuir-Blodgett (LB) method is another important way to fabricate the highly ordered film of conjugated polymers at liquid/air interface. And the stable and well-defined LB film on conjugate polymer can be transferred onto the hydrophobic substrate and thus can be applied for constructing organic devices, such as FET and etc [Citation101–103]. For the uniform LB film of regioregular P3HT, in most cases, the backbone of P3HT is parallel to the substrate surface and the preferred molecular orientation is in an edge-on orientation. The high-filed effect mobility (2.2 × 10−4 and 4.4 × 10−4 cm2 V−1 s−1) for mixed regioregular P3HT were obtained and used as the semiconducting film in the OFET.
3.4. 1D confined chain-assembly of P3HT in the 2D polyhedral oligomeric silsesquioxanes (POSS)-layered nanocrystals
Two-dimensional materials can be designed to improve the charge-carriers mobilities, mechanical, electronic, and optical properties. Taoet al. found a layered 2D nanocrystals of POSS-P3HT can be applied to the organic thin film transistor. The POSS-P3HT synthesized with click chemistry contains one P3HT molecular chain covalently linked with one POSS molecule [Citation104,Citation105]. The TEM bright-field morphology is displayed in and the selected-area electron diffraction experiments corresponding to the crystal in are shown in . The simulated pattern with the [12] zone of the 2D crystal on POSS-P3HT is displayed in . The 2D POSS-P3HT crystals own a hexagonal unit cell with a = b = 1.606 nm, and c = 1.714 nm with a symmetry group of P6. Based on the electron diffraction and wide-angle X-ray diffraction results of 2D crystals, we established the molecular packing model of 2D crystals()). The P3HT chains interdigitated between two crystalline layers of POSS [Citation106]. The sandwich 2D crystal structures could be also confirmed by the one layer’s thickness (26.7 nm) measured by atomic force microscopy. It is worth mentioning that the power conversion efficiency of the POSS-P3HT 2D crystal is 40% higher than that of the pristine P3HT mixed with [6,6]-phenyl-C61-butyric acid methyl ester (PCBM) ()).
Figure 5. (a) TEM BF morphology of the 2D crystals (POSS-P3HT); (b) selected-area electron diffraction pattern of POSS-P3HT single crystal; (c) calculated diffraction pattern with the [12] zone; (d) ab-plane projection of 2D crystals of POSS-P3HT; (e) schematic illustration of the molecular packing of the 2D crystals (POSS-P3HT); (f) hierarchical structure of the 2D crystals (POSS-P3HT); (g)IDS versus VGcurves of thin flim transisitors prepared by 2D nanocrystals (POSS-P3HT), pristine P3HT and Phenyl-P3HT J – V characteristics of thin film transistors preparedfrom 2DPOSS-P3HT nanocrystals, pristine P3HT and Phenyl-P3HT thin films (h) with and (i) without white illumination. Reprinted from Ref. [Citation106] with permission. Copyright 2019.
![Figure 5. (a) TEM BF morphology of the 2D crystals (POSS-P3HT); (b) selected-area electron diffraction pattern of POSS-P3HT single crystal; (c) calculated diffraction pattern with the [121ˉ] zone; (d) ab-plane projection of 2D crystals of POSS-P3HT; (e) schematic illustration of the molecular packing of the 2D crystals (POSS-P3HT); (f) hierarchical structure of the 2D crystals (POSS-P3HT); (g)IDS versus VGcurves of thin flim transisitors prepared by 2D nanocrystals (POSS-P3HT), pristine P3HT and Phenyl-P3HT J – V characteristics of thin film transistors preparedfrom 2DPOSS-P3HT nanocrystals, pristine P3HT and Phenyl-P3HT thin films (h) with and (i) without white illumination. Reprinted from Ref. [Citation106] with permission. Copyright 2019.](/cms/asset/b4124876-8bb9-47b2-a47b-aab89e86df14/tsta_a_2125826_f0005_oc.jpg)
3.5. Solution-Floating interfacial-assembly of P3HT nanowires
It is known that the evenness and morphology of P3HT thin films play a key role in the properties of functional electronic devices. Kim et al., invented a solution-floating self-assembly method to prepare the thin film of P3HT. P3HT samples are first dissolved into a toluene solution [Citation107]. A droplet of the solution was subsequently cast onto the water surface and a thin film of P3HT could be generated once the toluene was completely evaporated. The morphology of P3HT nanostructures can be tuned by changing the different types of solvents. Compared to the spin-coating methods for thin film preparation, the solution-floating assembling methods have more advantages and could be used to form a more ordered structure with high crystallinity.
The nanostructure and morphology generated in the thin film deeply rely on the solvent type for polymer and must be water-immiscible. Using the solution-floating methods, P3HT can self-assemble into a highly uniform thin film made up of nanowires. The GIWAXD results of the P3HT film prepared by toluene spin-cast, toluene solution-floating, and CHCl3 solution-floating are shown in ), respectively. The equatorial 010 and meridian h00 reflections manifest an edge-on orientation in the thin films of P3HT nanowires. Based on the transfer curves (ID–VG) of the OFET device, more ordered nanostructure and morphology could enhance the electronic properties of the organic electronic device. And the OFET device prepared by self-assembling P3HT via solution floating method has at least 3.4 times mobility value (0.086 cm2 V−1s−1) than that of assembling molecular chains of P3HT with spin-coating method (0.025 cm2 V−1s−1).
Figure 6. GIWAXD pattern of P3HT thin film prepared by (a) toluene spin-cast; (b) toluene solution-floating; (c) CHCl3 solution-floating; (d) J–V curves of P3HT thin films prepared by the methods as described in (a), (b) and (c); (e) TEM BF images of P3HT thin film made by solvent evaporation; (f) schematic illustration of OFET device fabricated by P3HT thin film with edge-on orientation. Reprinted from Ref. [Citation107] with permission. Copyright 2017.
![Figure 6. GIWAXD pattern of P3HT thin film prepared by (a) toluene spin-cast; (b) toluene solution-floating; (c) CHCl3 solution-floating; (d) J–V curves of P3HT thin films prepared by the methods as described in (a), (b) and (c); (e) TEM BF images of P3HT thin film made by solvent evaporation; (f) schematic illustration of OFET device fabricated by P3HT thin film with edge-on orientation. Reprinted from Ref. [Citation107] with permission. Copyright 2017.](/cms/asset/237b172e-3051-46cb-a0cf-494f4ddb24ff/tsta_a_2125826_f0006_oc.jpg)
A well orientated P3HT film can also be prepared by soft friction transfer methods, which is an easy approach to fabricate an uniaxially oriented P3HT film at room temperature and ambient atmosphere [Citation108]. And the film can be transferred from the glass substrate by using cellulose solution. This novel soft friction transfer method is a promising way to be used in next-generation transistors, sensors and other soft electronic devices.
3.6. Interfacial assembly of molecular chains in scrolled/flat crystals of P3HT
The crystal geometry of P3HT can be tuned by interfacial assembly of molecular chains in P3HT at a curved surface that are covalently linked with poly(ethylene glycol) (PEG) as block copolymer with different crystallization conditions [Citation109].
The half-ring crystals of P3HT shown in were observed in a solution crystallization of the pure P3HT and the P3HT-b-PEG at 30 ℃ without self-seeding. In this case, the sandwiched and curved crystal of P3HT can be formed in the middle. The inner and outerside of scrolled crystals of P3HT-b-PEG are PEG crystals and the packing density of PEG chains in both sides of middle P3HT crystals is not equal, and the unbalanced surface stress between inner and outside crystals results the curved crystal. The thickness of PEG lamellar on both sides was 17.4 (outer) and 15.0 (inner) nm. The scrolled crystals of P3HT are an edge-on orientation, identified by the 002 and 020 electron diffraction spots via TEM selected-area electron diffraction technique () and an 020 equatorial orientation was displayed in the 2D GIWAXD experiments.
Figure 7. (a) TEM BF image and (b) ED pattern of scrolled half-rings crystals of P3HT7000-b-PEG5000 block copolymers isothermally crystallized at 30 °C; (c) TEM BF image and (d) ED pattern of P3HT7000-b-PEG5000 square single crystals seedily grown at Tc = 30 °C for 24 h in amyl acetate.(e) TEM BF image and (f) ED pattern of the fibrillar single crystals of pure P3HT; (g) 2D GIWAXD pattern of cubic P3HT-b PEG crystals isothermally crystallized at 30 °C; (h) Schematic illustration of homopolymer-P3HT fibrillar crystal, P3HT-b-PEG scrolled half-rings crystals and flat-on crystals. Reprinted from Ref. [Citation109] with permission. Copyright 2016.
![Figure 7. (a) TEM BF image and (b) ED pattern of scrolled half-rings crystals of P3HT7000-b-PEG5000 block copolymers isothermally crystallized at 30 °C; (c) TEM BF image and (d) ED pattern of P3HT7000-b-PEG5000 square single crystals seedily grown at Tc = 30 °C for 24 h in amyl acetate.(e) TEM BF image and (f) ED pattern of the fibrillar single crystals of pure P3HT; (g) 2D GIWAXD pattern of cubic P3HT-b PEG crystals isothermally crystallized at 30 °C; (h) Schematic illustration of homopolymer-P3HT fibrillar crystal, P3HT-b-PEG scrolled half-rings crystals and flat-on crystals. Reprinted from Ref. [Citation109] with permission. Copyright 2016.](/cms/asset/ab1fa7ff-d7f5-4d09-ae1f-706170074658/tsta_a_2125826_f0007_oc.jpg)
On the other hand, the square crystals of P3HT shown in can be obtained by a two-step crystallization process by adding the pure PEG seeds formed at 40 ℃ to crystallize P3HT7000-b-PEG5000 solution at 30 ℃ via self-seeding technique. Four strong 120PEG diffraction spots confirmed the single crystals of PEG are generated with the [001] zone (). 020P3HT and 100P3HT diffraction spots in the ED pattern () reflects that P3HT crystals are epitaxially grown at the lamellar surface of PEO crystals. And the c-axis of the P3HT crystals is parallel to the PEG-lamellar surface normal. Therefore, under these circumstances, the P3HT crystals have an edge-on orientation. Generally speaking, the fibrillar crystals of P3HT () can be obtained when crystallizing the pristine P3HT samples. The long-axis of fibrillar crystals is along the b-axis (). Overall, the schematic illustration of P3HT crystals with crystal geometry (fibrillar, scrolled and square) via interfacial assembly are shown in .
3.7. Assembling the molecular chain of P3HT under geometrical (cylindrical) confinement and its application in organic solar cell device
A mold-releasing agent (low molecular weight (MW) polydimethylsiloxane) was used to modify the template surface to release the nanorods easily from the template. Therefore, perfect free-standing nanorods of P3HT without collapsing as shown in can be fabricated by reducing the interaction between P3HT and nanoporous alumina [Citation110]. Compared to the normal 2D confinement without modifying the template surface, the low MW polydimethylsiloxane was used to lower the surface energy of the template, and thus the rods are easily obtained after the template was etched by 5% KOH [Citation111]. displays the J-V curves of the OPV devices fabricated by P3HT nanopillar (50 and 30 nm). Compared to the common thin film device of P3HT, the power conversion efficiency of the P3HT nanorod device can be improved by about 2%. The rod diameter was dependent on the pore diameter of the AAO templates. The P3HT nanorods with the aspect ratio and spacing of 30 nm rod are about 6.6 and 60 nm, respectively. The edge-on orientation of P3HT has been identified in both pure P3HT and P3HT/PCBM nanopillars for 50 nm (. The molecular chains of P3HT are perpendicular to the rod long-axis. The ordered P3HT nanostructure induced by 2D confinement provides a new route to design the high-performance organic electronic device.
Figure 8. Scanning electron micrograph of (a) pure P3HT nanopillars; (b) P3HT/PCBM nanopillars; (c) J-V curves of the organic photovoltaic devices made by P3HT nanopillar; GIWAXD patterns of (d) pure P3HT nanopillars and (e) P3HT/PCBM nanopillars; (f) schematic illustration of organic solar cell devices with P3HT nanopillars; (g) the edge-on orientation of P3HT thin films. Reprinted with permission from Ref. [Citation110]. Copyright 2012.
![Figure 8. Scanning electron micrograph of (a) pure P3HT nanopillars; (b) P3HT/PCBM nanopillars; (c) J-V curves of the organic photovoltaic devices made by P3HT nanopillar; GIWAXD patterns of (d) pure P3HT nanopillars and (e) P3HT/PCBM nanopillars; (f) schematic illustration of organic solar cell devices with P3HT nanopillars; (g) the edge-on orientation of P3HT thin films. Reprinted with permission from Ref. [Citation110]. Copyright 2012.](/cms/asset/25a6669e-96b7-44a6-be31-e8cf40cb7a0a/tsta_a_2125826_f0008_oc.jpg)
It is worth mentioning that the periodic P3HT surface nanostructure can be fabricated by laser radiation with a fluence of about 22~30 mJ/cm2. When this periodic surface structure of P3HT induced by laser radiation is applied in an organic solar cell acting as a bilayer covered by C71-butyric acid methyl ester, an enhancement of about 10% is found in the open-circuit voltages for the solar cell. And the interesting heterogeneous electrical conductivity of the laser-induced nanostructured solar cell does not affect the production of photocurrent [Citation112].
4. Summary and perspective
The interfacial assembly of P3HT and its application in organic electronic devices has been summarized in this review. Four main factors greatly affect the molecular packing of P3HT at the interface: a. the interaction between P3HT and the substrate; b. the geometrical confinement; c. covalently linked with the nanoatoms or other polymer; d. thin film preparation methods. An edge-on orientation of P3HT can be obtained by self-assembling P3HT-based block copolymers, in which P3HT is chemically linked with a nanoatom such as POSS or the other polymer like PEG etc. In the 2D crystals of P3HT-based block copolymers, the P3HT crystals have a flat-on orientation on the substrate of 2D crystals. The geometrical (cylindrical) constraints on the crystallization of P3HT nanorods favor an edge-on orientation. Specific thin film preparation methods like using mechanical force or solution-floating self-assembling nanowire or spinning-coating thin film methods usually generate an edge-on orientation, which could be widely applied in organic field-effect transistors. For comparison, the interaction between thiophene rings and graphene promotes the face-on orientation, which can be used to improve the device efficiency of the organic solar cell.
Overall, understanding the formation mechanism of interfacial molecular self-assembly of P3HT and their relationship associated with the edge-on and face-on orientation is a key issue to manipulate the electron and hole to undergo orderly transporting, which has a deep impact on improving the performance of the organic electronic device.
Acknowledgement
This work was supported by the Natural Science Foundation of SZU (827-000150 and 860-0000021103750) and the Recruitment Program of Guangdong (No.2016ZT06C322).
Disclosure statement
No potential conflict of interest was reported by the author(s).
Additional information
Funding
Notes on contributors
Yan Cao
Yan Cao is currently a Instrument Platform Director of Advanced Institute for Soft Matter Science and Technology (AISMST) at South China University of Technology. She is proficient in solving the crystal structural problem of soft matter via X-ray diffraction and electron diffraction on transmission electron microscopy. She worked as a principle investigator at the Institute of Advanced Study of Shenzhen University (2015–2022). She got a Ph.D. in polymer physics at the department of polymer science at the University of Akron, USA (2007–2012) and have done postdoc research on the confined polymer nanostructural characterization for Japan Science and Technology Agency (JST)—Takahara Soft Interface ERATO project (2012–2014).
References
- Zhang C, Qiao Y, Xiong P, et al. Conjugated microporous polymers with tunable electronic structure for high-performance potassium-ion batteries. ACS Nano. 2019;13(1):745–754.
- Holliday S, Ashraf RS, Wadsworth A, et al. High-Efficiency and air-stable P3HT-based polymer solar cells with a new non-fullerene acceptor. Nat Commun. 2016;7(1):11585–11595.
- Dang MT, Hirsch L, Wantz G. P3HT:PCBM. Best Seller Polym Photovoltaic Res. 2011;23(31):3597–3602.
- Cook S, Furube A, Katoh R. Analysis of the excited states of regioregular polythiophene P3HT. Energy Environ Sci. 2008;1(2):294–299.
- Ahn S-K, Carrillo JM-Y, Keum JK, et al. Nanoporous poly(3-hexylthiophene) thin film structures from self-organization of a tunable molecular bottlebrush scaffold. Nanoscale. 2017;9(21):7071–7080.
- Liu S, Kang L, Henzie J, et al. Recent advances and perspectives of battery-type anode materials for potassium ion storage. ACS Nano. 2021;15(12):18931–18973.
- Chen L, Honsho Y, Seki S, et al. Light-harvesting conjugated microporous polymers: rapid and highly efficient flow of light energy with a porous polyphenylene framework as antenna. J Am Chem Soc. 2010;132(19):6742–6748.
- Jiang J-X, Trewin A, Adams DJ, et al. Band gap engineering in fluorescent conjugated microporous polymers. Chem Sci. 2011;2(9):1777–1781.
- Scheuble M, Goll M, Ludwigs S. Branched terthiophenes in organic electronics: from small molecules to polymers. 2015;36(2):115–137. doi:10.1002/marc.201400525
- Jarosz T, Lapkowski M, Ledwon P. Advances in star-shaped π-conjugated systems: properties and applications. Macromol Rapid Commun. 2014;35(11):1006–1032.
- Handa NV, Serrano AV, Robb MJ, et al. Exploring the synthesis and impact of end-functional poly(3-hexylthiophene). J Polym Sci A Polym Chem. 2015;53(7):831–841.
- Bhatt MP, Magurudeniya HD, Rainbolt EA, et al. Poly(3-hexylthiophene) nanostructured materials for organic electronics applications. J Nanosci Nanotechnol. 2014;14(2):1033–1050.
- Buono A, Son NH, Raos G, et al. Poly(3-butylthiophene): crystal structure and preferred orientation in spherulitic thin films. Macromolecules. 2010;43(16):6772–6781.
- Kayunkid N, Uttiya S, Brinkmann M. Structural model of regioregular poly(3-hexylthiophene) obtained by electron diffraction analysis. Macromolecules. 2010;43(11):4961–4967.
- Zeng X, Zhang S, Zheng N, et al. Diversified Α-phase nanostructure of isotactic polypropylene under cylindrical confinement via cross diffraction analysis. Polymer. 2019;179:121647–121653.
- Liang Z, Zheng N, Ni B, et al. Coherent crystal branches: the impact of tetragonal symmetry on the 2D confined polymer nanostructure. IUCrJ. 2021;8(2):215–224.
- Sohail M, Huang J, Lai Z, et al. Synthesis of flower-like Co9S8/reduced graphene oxide nanocomposites and their photocatalytic performance. J Inorg Organomet Polym Mater. 2020;30(12):5168–5179.
- Zhang W, Cao Y, Lai Z, et al. Hierarchical structure with an unusual honeycomb fullerene scaffold by a fullerene–triphenylene shape amphiphile. Macromolecules. 2020;53(14):6056–6062.
- Wang KC, Lai ZW, Huang JM, et al. Dual effects of interfacial interaction and geometric constraints on structural formation of Poly(Butylene Terephthalate) nanorods. Chin J Polym Sci. 2022;40(6):700–708.
- Lai ZW, Zheng N, Liang ZY, et al. Structural ensemble of molecular chains in isotactic polypropylene under cylindrical confinement. Macromolecules. 2021;54(5):2325–2333.
- Yang C-E, Woo EM, Nagarajan S. Epicycloid extinction-band assembly in Poly(Decamethylene Terephthalate) confined in thin films and crystallized at high temperatures. Polymer. 2021;212:123256–123270.
- Villada-Gil S, Palacio-Betancur V, Armas-Perez JC, et al. Directing the far-from-equilibrium assembly of nanoparticles in confined liquid crystals by hydrodynamic fields. Soft Matter. 2021;17(12):3463–3472.
- Hu S, Nozawa J, Kang K, et al. Structural transformations of growing thin colloidal crystals in confined space via convective assembly. J Colloid Interface Sci. 2021;591:300–306.
- Pitet LM, Chamberlain BM, Hauser AW, et al. Dispersity and architecture driven self-assembly and confined crystallization of symmetric branched block copolymers. Polym Chem. 2019;10(39):5385–5395.
- Chen Y, Huang S, Wang T, et al. Confined self-assembly enables stabilization and patterning of nanostructures in liquid-crystalline block copolymers. Macromolecules. 2019;52(4):1892–1898.
- Heo K, Miesch C, Na JH, et al. Assembly of P3HT/Cdse nanowire networks in an insulating polymer host. Soft Matter. 2018;14(25):5327–5332.
- Istif E, Kagkoura A, Hernandez-Ferrer J, et al. Self-assembled core-shell CdTe/poly(3-hexylthiophene) nanoensembles as novel donor-acceptor light-harvesting Systems. ACS Appl Mater Interfaces. 2017;9(51):44695–44703.
- Uto K, Yamamoto K, Kishimoto N, et al. Characterization of stable, electroactive protein cage/synthetic polymer multilayer thin films prepared by layer-by-layer assembly. J Nanopart Res. 2013;15(4):1516–1526.
- Kim DH, Han JT, Park YD, et al. Single-crystal polythiophene microwires grown by self-assembly. Adv Mater. 2006;18(6):719–723.
- Makamba H, Hsieh YY, Sung WC, et al. Stable permanently hydrophilic protein-resistant thin-film coatings on Poly(dimethylsiloxane) substrates by electrostatic self-assembly and chemical cross-linking. Anal Chem. 2005;77(13):3971–3978.
- Rahimi K, Botiz I, Stingelin N, et al. Controllable processes for generating large single crystals of poly(3-hexylthiophene). Angew Chem Int Ed Engl. 2012;51(44):11131–11135.
- Martín J, Campoy-Quiles M, Nogales A, et al. Poly(3-hexylthiophene) nanowires in porous alumina: internal structure under confinement. Soft Matter. 2014;10(18):3335–3346.
- Shen C, Chai S, Zou S, et al. Crystallization of poly(3-hexylthiophene) on graphitic surfaces with different curvatures. Polymer. 2018;144:168–178.
- Ye Z, Xiubao Y, Cui H, et al. Nanowires with unusual packing of poly(3-hexylthiophene)s induced by electric fields. J Mater Chem C. 2014;2(33):6773–6780.
- Zhai L, Khondaker SI, Thomas J, et al. Ordered conjugated polymer nano- and microstructures: structure control for improved performance of organic electronics. Nano Today. 2014;9(6):705–721.
- Shin H, Lee H, Kim B, et al. Effects of blended poly(3-hexylthiophene) and 6,13-bis(triisopropylsilylethynyl) pentacene organic semiconductors on the photoresponse characteristics of thin-film transistors. Korean J Met Mater. 2022;60(3):198–205.
- Ruffino R, Fichera L, Valenti A, et al. Tuning the randomization of lamellar orientation in poly (3-hexylthiophene) thin films with substrate nano-curvature. Polymer. 2021;230:124071–124079.
- Shin H, Park J, Choi JS. Illumination effect on electrical characteristics of poly(3-hexylthiophene): TIPS-pentacene blend thin-film transistor. J Nanosci Nanotechnol. 2021;21(7):3829–3834.
- Higashihara T, Fukuta S, Ochiai Y, et al. synthesis and deformable hierarchical nanostructure of intrinsically stretchable ABA triblock copolymer composed of poly(3-hexylthiophene) and polyisobutylene segments. Acs Appl Polym Mater. 2019;1(3):315–320.
- Shinohara T, Higaki Y, Hoshino T, et al. “Buried” nano-structure and molecular aggregation state in ordered heterojunction poly(3-hexylthiophene)-based photovoltaics. Jpn J Appl Phys. 2014;53(5):05FH09.
- Liu K, Yang Q, Tang Y, et al. Effects of solvent boiling-point on chain structure and molecular orientation in poly(3-hexylthiophene)thin film. J Funct Polym. 2019;32(2):192–198,218.
- Agbolaghi S. Optical/thermal studies on nanostructures of poly(3-hexylthiophene) and carbon nanotube/graphene precursors. Fuller Nanotub Car Nanostruct. 2019;27(7):572–581.
- Hosseinzadeh N, Agbolaghi S, Abbaspoor S, et al. A delicate maneuver on conjugated rod-rod structures composed of poly(3-hexylthiophene) and polyaniline subtending patched-fibrillar, ringed-fibrillar, double-fibrillar and sandwiched configurations. J Polym Res. 2018;25(8):189–200.
- Hase H, O’Neill K, Frisch J, et al. Unraveling the microstructure of molecularly doped poly(3-hexylthiophene) by thermally induced dedoping. J Phys Chem C. 2018;122(45):25893–25899.
- Abbaspoor S, Agbolaghi S, Mahmoudi M, et al. Supramolecular donor-acceptor structures via orienting predeveloped fibrillar poly(3-hexylthiophene) crystals on bared/functionalized/grafted reduced graphene oxide with novel thiophenic constituents. Organic Electron. 2018;52:243–256.
- Xiang WZ, Sun XL, Ren ZJ, et al. Diameter and thermal treatment dependent structure and optical properties of poly(3-hexylthiophene) nanotubes. J Mater Chem C. 2017;5(32):8315–8322.
- Matsumoto T, Nishi K, Tamba S, et al. Molecular weight effect on surface and bulk structure of poly(3-hexylthiophene) thin films. Polymer. 2017;119:76–82.
- Koch FPV, Rivnay J, Foster S, et al. The impact of molecular weight on microstructure and charge transport in semicrystalline polymer semiconductors poly(3-hexylthiophene), a model study. Progress Polym Sci. 2013;38(12):1978–1989.
- Xie WY, Sun YY, Zhang SB, et al. Structure and sources of disorder in poly(3-hexylthiophene) crystals investigated by density functional calculations with Van Der Waals interactions. Phys Rev B. 2011;83(18):184117–184122.
- Cheval N, Kampars V, Fowkes C, et al. Assembl y of poly-3-hexylthiophene nano-crystallites into low dimensional structures using indandione derivatives. Nanomaterials. 2013;3(1):107–116.
- Heinrich CD, Thelakkat M. Poly-(3-hexylthiophene) bottlebrush copolymers with tailored side-chain lengths and high charge carrier mobilities. J Mater Chem C. 2016;4(23):5370–5378.
- Al-Harthi AM, Mahmoud WE. Influence of the fullerene acceptor derivative on the structure, molecular interaction and optoelectronic properties of poly(3-hexylthiophene) blend films: elucidating key factors. Opt Mater. 2022;124:112009–112019.
- Dong WJ, Cho WS, Lee JL. Indium tin oxide branched nanowire and poly(3-hexylthiophene) hybrid structure for a photorechargeable supercapacitor. ACS Appl Mater Interfaces. 2021;13(19):22676–22683.
- Wei RB, Gryszel M, Migliaccio L, et al. Tuning photoelectrochemical performance of poly(3-hexylthiophene) electrodes via surface structuring. J Mater Chem C. 2020;8(31):10897–10906.
- Faria GC, Coutinho DJ, von Seggern H, et al. Doping mechanism in organic devices: effects of oxygen molecules in poly (3-hexylthiophene) thin films. Organic Electron. 2018;57:298–304.
- Xu W, Han H Three-terminal artificial synapse device for realizing analog neural signals, has negative voltage electrical pulse which is configured to cause portion of anion to rapidly collect around poly 3-hexylthiophene (P3HT) ultra-thin Film. CN110277496-A.
- Zenoozi S, Agbolaghi S, Poormahdi E, et al. Verification of scherrer formula for well-shaped poly(3-hexylthiophene)-based conductive single crystals and nanofibers and fabrication of photovoltaic devices from thin film coating. Macromol Res. 2017;25(8):826–840.
- Ahn KS, Jo H, Kim JB, et al. Structural transition and interdigitation of alkyl side chains in the conjugated polymer poly(3-hexylthiophene) and their effects on the device performance of the associated organic field-effect transistor. ACS Appl Mater Interfaces. 2020;12(1):1142–1150.
- Zhang R, Li B, Iovu MC, et al. Nanostructure dependence of field-effect mobility in regioregular poly(3-hexylthiophene) thin film field effect transistors. J Am Chem Soc. 2006;128(11):3480–3481.
- Salleo A. Charge transport in polymeric transistors. Mater Today. 2007;10(3):38–45.
- Couto R, Chambon S, Aymonier C, et al. Microfluidic supercritical antisolvent continuous processing and direct spray-coating of poly-(3-hexylthiophene) nanoparticles for OFET devices. Chem Comm. 2015;51(6):1008–1011.
- Kang YH, Ko SJ, Lee MH, et al. Highly efficient and air stable thermoelectric devices of Poly (3-Hexylthiophene) by dual doping of Au metal precursors. Nano Energy. 2021;82:105681–105691.
- Lan T, Gao ZJ, Barbosa MS, et al. Flexible ion-gated transistors making use of poly-3-hexylthiophene (P3HT): effect of the molecular weight on the effectiveness of gating and device performance. J Electron Mater. 2020;49(9):5302–5307.
- Deng Y, Jiang J, Sun J, et al. Nanowire junction induced high threshold voltage in poly(3-hexylthiophene) mesoscale crystalline thin-film transistors with significantly enhanced mobility. Phys Status Solidi Rapid Res Lett. 2020;14(4):1900723–1900728.
- Rawlings D, Thomas EM, Segalman RA, et al. Controlling the doping mechanism in poly(3-hexylthiophene) thin-film transistors with polymeric ionic liquid dielectrics. Chem Mater. 2019;31(21):8820–8829.
- Park H, Ma BS, Kim J-S, et al. Regioregular-block-regiorandom poly(3-hexylthiophene) copolymers for mechanically robust and high-performance thin-film transistors. Macromolecules. 2019;52(20):7721–7730.
- Luo J, Gao Y, Wang XY, et al. Double in-plane-gate IZO-based thin-film transistors with pea protein gate dielectrics. J Phys D Appl Phys. 2019;52(17):174001–174006.
- Kim M, Ryu SU, Park SA, et al. Donor-acceptor-conjugated polymer for high-performance organic field-effect transistors: a progress report. Adv Funct Mater. 2019;30:1904545–1904569.
- Hou S, Yu J. Self-stratified poly(3-Hexylthiophene)/Polystyrene (P3HT/PS) blends based organic thin-film transistor nitrogen dioxide gas sensors. Abstr Papers Am Chem Soc. 2019;257:10–15.
- Yang J, Xie G, Su Y, et al. Flexible organic thin-film transistors based on poly(3-hexylthiophene) films for nitrogen dioxide detection. Sci China Technol Sci. 2018;61(11):1696–1704.
- Miyane S, Wen H-F, Chen W-C, et al. Synthesis of block copolymers comprised of poly(3-hexylthiophene) segment with trisiloxane side chains and their application to organic thin film transistor. J Polym Sci Part A-Polym Chem. 2018;56(16):1787–1794.
- Mansouri S, Coskun B, El Mir L, et al. Graphene oxide/poly(3-hexylthiophene) nanocomposite thin-film phototransistor for logic circuit applications. J Electron Mater. 2018;47(4):2461–2467.
- Wang J-T, Takshima S, Wu H-C, et al. Stretchable conjugated rod coil poly(3-hexylthiophene)-block-Poly(butyl acrylate) thin films for field effect transistor applications. Macromolecules. 2017;50(4):1442–1452.
- Mun S, Park Y, Lee Y-EK, et al. Highly sensitive ammonia gas sensor based on single-crystal poly(3-hexylthiophene) (P3HT) organic field effect transistor. Langmuir. 2017;33(47):13554–13560.
- Xiao CY, Zhao GY, Zhang AD, et al. High performance polymer nanowire field-effect transistors with distinct molecular orientations. Adv Mater. 2015;27(34):4963–4968.
- Smith BH, Clark MB, Kuang H, et al. Controlling polymorphism in poly(3-hexylthiophene) through addition of ferrocene for enhanced charge mobilities in thin-film transistors. Adv Funct Mater. 2015;25(4):542–551.
- Tiwari S, Takashima W, Nagamatsu S, et al. A comparative study of spin coated and floating film transfer method coated poly (3-hexylthiophene)/poly (3-hexylthiophene)-nanofibers based field effect transistors. J Appl Phys. 2014;116(9):094306–094313.
- Kline RJ, McGehee MD, Toney MF. Highly oriented crystals at the buried interface in polythiophene thin-film transistors. Nat Mater. 2006;5(3):222–228.
- Arif M, Liu JH, Zhai L, et al. Poly(3-hexylthiophene) crystalline nanoribbon network for organic field effect transistors. Appl Phys Lett. 2010;96(24):243304–243306.
- Tian XY, Xu Z, Zhao SL, et al. Vacuum relaxation and annealing-induced enhancement of mobility of regioregular poly(3-hexylthiophene) field-effect transistors. Chin Phys B. 2009;18(11):5078–5083.
- Lilliu S, Agostinelli T, Pires E, et al. Dynamics of crystallization and disorder during annealing of P3HT/PCBM bulk heterojunctions. Macromolecules. 2011;44(8):2725–2734.
- Hamidi-Sakr A, Biniek L, Fall S, et al. Precise control of lamellar thickness in highly oriented regioregular poly(3-hexylthiophene) thin films prepared by high-temperature rubbing: correlations with optical properties and charge transport. Adv Funct Mater. 2016;26(3):408–420.
- Brinkmann M, Rannou P. Effect of molecular weight on the structure and morphology of oriented thin films of regioregular poly(3-hexylthiophene) grown by directional epitaxial solidification. Adv Funct Mater. 2007;17(1):101–108.
- Kajiya D, Ozawa S, Koganezawa T, et al. Enhancement Of out-of-plane mobility in P3HT film by rubbing: aggregation and planarity enhanced with low regioregularity. J Phys Chem C. 2015;119(15):7987–7995.
- Kajiya D, Koganezawa T, Saitow K. Enhancement of out-of-plane mobilities of three poly(3-alkylthiophene)s and associated mechanism. J Phys Chem C. 2016;120(41):23351–23357.
- Zen A, Pflaum J, Hirschmann S, et al. Effect of molecular weight and annealing of poly (3-hexylthiophene)s on the performance of organic field-effect transistors. Adv Funct Mater. 2004;14(8):757–764.
- Kline RJ, McGehee MD, Kadnikova EN, et al. Controlling the field-effect mobility of regioregular polythiophene by changing the molecular weight. Adv Mater. 2003;15(18):1519–1522.
- Kline RJ, McGehee MD, Kadnikova EN, et al. Dependence Of regioregular poly(3-hexylthiophene) film morphology and field-effect mobility on molecular weight. Macromolecules. 2005;38(8):3312–3319.
- Chang JF, Sun BQ, Breiby DW, et al. Enhanced mobility of poly(3-hexylthiophene) transistors by spin-coating from high-boiling-point solvents. Chem Mater. 2004;16(23):4772–4776.
- Bao Z, Dodabalapur A, Lovinger AJ. Soluble and processable regioregular poly(3-hexylthiophene) for thin film field-effect transistor applications with high mobility. Appl Phys Lett. 1996;69(26):4108–4110.
- Wang BH, Chen JB, Shen CY, et al. Relation between charge transport and the number of interconnected lamellar poly(3-hexylthiophene) crystals. Macromolecules. 2019;52(16):6088–6096.
- Agbolaghi S, Abbaspoor S, Massoumi B, et al. Conversion of face-on orientation to edge-on/flat-on in induced-crystallization of poly(3-hexylthiophene) via functionalization/grafting of reduced graphene oxide with thiophene Adducts. Macromole Chem Phys. 2018;219(4):1700484–1700498.
- Kajiya D, Saitow K. Ultrapure films of polythiophene derivatives are born on a substrate by liquid flow. ACS Appl Energy Mater. 2018;1(12):6881–6889.
- Sakata T, Saitow K. 4D, microspectroscopy explores orientation and aggregations in π-conjugated polymer films prepared by brush Printing. J Phys Chem Lett. 2022;13(2):653–660.
- Chae S, Yi A, Kim HJ. Molecular engineering of a conjugated polymer as a hole transporting layer for versatile p–i–n perovskite solar cells. Mater Today Energy. 2019;14:10034.
- Chae S, Yi A, Lee HH, et al. Laser-induced orientation transformation of a conjugated polymer thin film with enhanced vertical charge transport. J Mater Chem C. 2018;6(35):9374–9382.
- Chaudhary V, Pandey RK, Sahu PK, et al. MoS2 assisted self-assembled poly(3-hexylthiophene) thin films at an air/liquid interface for high-performance field-effect transistors under ambient conditions. J Phys Chem C. 2020;124(15):8101–8109.
- Kleinhenz N, Persson N, Xue Z, et al. Ordering of poly(3-hexylthiophene) in solutions and films: effects of fiber length and grain boundaries on anisotropy and mobility. Chem Mater. 2016;28(11):3905–3913.
- Kim N, Lee BH, Choi D, et al. Role of interchain coupling in the metallic state of conducting polymers. Phys Rev Lett. 2012;109(10):106405.
- Kajiya D, Saitow K. Si-Nanocrystal/p3ht hybrid films with a 50- and 12-fold enhancement of hole mobility and density: films prepared by successive drop casting. Nanoscale. 2015;7(38):15780–15788.
- Xu G, Bao Z, Groves JT. Langmuir−blodgett films of regioregular poly(3-hexylthiophene) aS field-effect transistors. Langmuir. 2000;16(4):1834–1841.
- Matsui J, Yoshida S, Mikayama T, et al. Fabrication of polymer langmuir−blodgett films containing regioregular poly(3-hexylthiophene) for application to field-effect transistor. Langmuir. 2005;21(12):5343–5348.
- Zhu H, Yamamoto S, Matsui J, et al. Resistive non-volatile memories fabricated with poly(vinylidene fluoride)/poly(thiophene) blend nanosheets. RSC Adv. 2018;8(15):7963–7968.
- Leu C-M, Chang Y-T, Wei K-H. Polyimide-side-chain tethered polyhedral oligomeric silsesquioxane nanocomposites for low-dielectric film applications. Chem Mater. 2003;15(19):3721–3727.
- Elemans JAAW, Lei S, De Feyter S. Molecular and supramolecular networks on surfaces: from two-dimensional crystal engineering to reactivity. Angewandte Chemie. 2009;48(40):7298–7332.
- Zhu T, Zheng LY, Yi C, et al. Two-dimensional conjugated polymeric nanocrystals for organic electronics. Acs Appl Electron Mater. 2019;1(8):1458–1464.
- Kim Y-J, Jung H-T, Ahn CW, et al. Simultaneously induced self-assembly of poly(3-hexylthiophene) (P3HT) nanowires and thin-film fabrication via solution-floating method on a water substrate. Adv Mater Interfaces. 2017;4(19):19.
- Imanishi M, Kajiya D, Koganezawa T, et al. Uniaxial orientation of P3HT film prepared by soft friction transfer method. Sci Rep. 2017;7(1):5141–5150.
- Agbolaghi S, Zenoozi S, Hosseini Z, et al. Scrolled/flat crystalline structures of poly(3-hexylthiophene) and Poly(ethylene glycol) block copolymers subsuming unseeded half-ring-like and seeded cubic, epitaxial, and fibrillar crystals. Macromolecules. 2016;49(24):9531–9541.
- Chen D, Zhao W, Russell TP. P3HT nanopillars for organic photovoltaic devices nanoimprinted by AAO templates. ACS Nano. 2012;6(2):1479–1485.
- Byun J, Kim Y, Jeon G, et al. Ultrahigh density array of free-standing poly(3-hexylthiophene) nanotubes on conducting substrates via solution wetting. Macromolecules. 2011;44(21):8558–8562.
- Cui J, Rodriguez-Rodriguez A, Hernandez M, et al. Laser-induced periodic surface structures on P3HT and on its photovoltaic blend with PC71BM. ACS Appl Mater Interfaces. 2016;8(46):31894–31901.