ABSTRACT
Despite the ambitious temperature goal of the 2015 Paris Agreement, the pace of reducing global CO2 emissions remains sluggish. This creates conditions in which the idea of temperature ‘overshoot and peak-shaving’ is emerging as a possible strategy to meet the Paris goal. An overshoot and peak-shaving scenario rests upon the ‘temporary’ use of speculative solar radiation management (SRM) technologies combined with large-scale carbon dioxide removal (CDR). Whilst some view optimistically the strategic interdependence between SRM and CDR, we argue that this strategy comes with a risk of escalating ‘climate debt’. We explain our position using the logic of debt and the analogy of subprime mortgage lending. In overshoot and peak-shaving scenarios, the role of CDR and SRM is to compensate for delayed mitigation, placing the world in a double debt: ‘emissions debt’ and ‘temperature debt’. Analogously, this can be understood as a combination of ‘subprime mortgage’ (i.e. large-scale CDR) and ‘home-equity-line-of-credit’ (i.e. temporary SRM). With this analogy, we draw some important lessons from the 2007–2009 US subprime mortgage crisis. The analogy signals that the efficacy of temporary SRM cannot be evaluated in isolation of the feasibility of large-scale CDR and that the failure of the overshoot promise will lead to prolonged peak-shaving, masking an ever-rising climate debt. Overshoot and peak-shaving scenarios should not be presented as a secured feasible investment, but rather as a high-risk speculation betting on insecure promises. Obscuring the riskiness of such scenarios is a precipitous step towards escalating a climate debt crisis.
Key policy insights
The slow progress of mitigation increases the attraction of an ‘overshoot and peak-shaving’ scenario which combines temporary SRM with large-scale CDR
Following the logic of debt, the role of CDR and SRM in this scenario is to compensate for delayed mitigation, creating a double debt of CO2 emissions and global temperature
Using the analogy of subprime lending, this strategy can be seen as offering a combination of subprime mortgage and open-ended ‘line-of-credit’
Because the ‘success’ of peak-shaving by temporary SRM hinges critically on the overshoot promise of large-scale CDR, SRM and CDR should not be discussed separately
Introduction
In the wake of the 2015 Paris Agreement, the debate about the role of solar geoengineering (or solar radiation management, SRM) and negative emissions (or carbon dioxide removal, CDR) is changing quickly, specifically whether and how these technologies should be situated within a portfolio of climate policy responses.
Before Paris, SRM and CDR were often grouped together under the common rubric ‘geoengineering’ (Royal Society, Citation2009). But now, many experts agree that a clear separation between SRM and CDR is needed for sensible science-policy discussions (Boucher et al., Citation2014; Lomax, Workman, Lenton, & Shah, Citation2015; Minx et al., Citation2018). While SRM is still largely ignored in the mainstream debate (Parker & Geden, Citation2016), CDR is increasingly considered a crucial policy option necessary to meet the Paris Agreement’s goal of limiting global temperature rise to well below 2°C, or even 1.5°C, above the pre-industrial level (Gasser, Guivarch, Tachiiri, Jones, & Ciais, Citation2015; Minx et al., Citation2018; Peters & Geden, Citation2017; Smith et al., Citation2016). The Intergovernmental Panel on Climate Change (IPCC) Special Report on 1.5°C somewhat endorsed this position, where the risks and potentials of CDR were extensively analysed across different mitigation pathways, but SRM was kept off the table as a viable policy option (IPCC, Citation2018).
However, the slow progress in global CO2 emissions reduction (with a rise in 2018; Le Quéré et al., Citation2018) is increasing the likelihood of ‘overshoot’ scenarios in which warming temporarily exceeds the 1.5–2°C level, before eventually returning to this target through reliance on large-scale deployment of CDR technologies (Ricke, Millar, & MacMartin, Citation2017). This increased likelihood of temperature overshoot is changing the context of debate, opening up the possibility of temporarily using SRM to avoid such overshoot—so-called ‘peak-shaving’ (Sugiyama, Arino, Kosugi, Kurosawa, & Watanabe, Citation2018). It is indeed argued by some that SRM could be used as a stop-gap measure as part of a holistic climate strategy to meet the Paris temperature target (Long, Citation2017; MacMartin, Ricke, & Keith, Citation2018).
In this article, we argue that this emerging idea of temperature ‘overshoot and peak-shaving’ is not a secured investment strategy for meeting the Paris target. Rather, it should better be understood as a high-risk speculation, betting on the insecure promises of both solar geoengineering and negative emissions. We demonstrate this using the logic of debt and the analogy of subprime mortgage lending.
Temperature ‘overshoot and peak-shaving’ scenario
The Paris Agreement has brought the feasibility and desirability of large-scale CDR deployment under the spotlight (Anderson & Peters, Citation2016; Field & Mach, Citation2017; Lenzi, Lamb, Hilaire, Kowarsch, & Minx, Citation2018; Williamson, Citation2016). This is because nearly all the emissions scenarios in the IPCC Fifth Assessment Report that are likely to keep warming below 2°C require net negative CO2 emissions in the second half of this century. This outcome is mostly achieved through the presumed extensive use of CDR technologies, such as bioenergy with carbon capture and storage (BECCS) (Fuss et al., Citation2014). All the scenarios in the IPCC Special Report on 1.5°C presume the use of some form of CDR technology, even if not BECCS (IPCC, Citation2018; see also Holz, Siegel, & Johnston, Citation2018; Obersteiner et al., Citation2018). It is now recognized that CDR is a necessity to meet, at a minimum, the net-zero emissions target by compensating for ‘residual CO2 emissions’ that are near impossible to mitigate (Gasser et al., Citation2015; Geden, Peters, & Scott, Citation2019; Rogelj et al., Citation2015).
These emissions scenarios are developed by integrated assessment models (IAMs) which are built around finding policy pathways that enable long-term cost optimization, incorporating assumptions about technological change, energy systems, population growth, etc. (Knutti & Rogelj, Citation2015). Such cost-optimal pathways determined in IAMs are strongly influenced by the choice of discount rate (Stanton, Ackerman, & Kartha, Citation2009). The models usually apply a relatively high discount rate (van Vuuren, Hof, van Sluisveld, & Riahi, Citation2017), which in turn places less weight on the future cost of BECCS than on the present cost of deep conventional mitigation (Anderson & Peters, Citation2016). Importantly, the inclusion of BECCS and other CDR technologies yields emissions scenarios in which global temperature can temporarily exceed the threshold before falling back to the target level—so-called ‘overshoot’ scenarios (Huntingford & Lowe, Citation2007)—a technically and economically feasible option. The concept of overshoot is politically appealing because it can ease the burden of near-term mitigation, while still keeping alive the promise of achieving long-term stabilization targets (Geden & Löschel, Citation2017).
However, temperature overshoot does nothing to defuse the inherent risks associated with increased warming. Depending on the magnitude and duration of overshoot, these risks might include serious, irreversible ecological and social changes (O’Neill et al., Citation2017; Ricke, Moreno-Cruz, Schewe, Levermann, & Caldeira, Citation2016). Anxieties about such climate-driven risks could heighten the attraction of using SRM to avoid overshoot because SRM technologies such as releasing sulphate aerosols into the stratosphere can in theory cool the Earth very quickly (Irvine, Kravitz, Lawrence, & Muri, Citation2016). Despite uncertainty over its adverse side-effects, this quick-cooling effect of SRM makes it a potential means to reduce some of the serious impacts of climate change (Keith, Parson, & Morgan, Citation2010), except non-climatic impacts such as ocean acidification. In climate model simulations, SRM is conceived as a temporary intervention to shave off the peak warming (‘peak-shaving’) caused by excess emissions, so that global temperature can be kept approximately constant during the overshooting period (MacMartin et al., Citation2018; Niemeier & Tilmes, Citation2017; Smith & Rasch, Citation2013; Sugiyama et al., Citation2018; Tilmes, Sanderson, & O’Neill, Citation2016).
Following Paris, if policymakers take the 1.5–2°C goal seriously, the concept of ‘overshoot and peak-shaving’ scenarios as a possible delivery strategy could attract more attention than ever before. Rogelj et al. (Citation2016) estimate that global temperature will likely rise to around 3°C or more by 2100 if the same level of mitigation efforts made under the Paris Agreement’s nationally determined contributions (NDCs) continues after 2030. While deep emissions cuts before 2030 can avoid overshoot, and hence future reliance on large-scale CDR (IPCC, Citation2018), the sluggish pace of reducing global CO2 emissions (UNEP, Citation2018) appears to make overshoot scenarios more likely. Peak-shaving by temporary SRM might therefore be conceived as a necessary complement to keep warming below 1.5–2°C (MacMartin et al., Citation2018; MacMartin & Kravitz, Citation2019; Parker & Geden, Citation2016). Following this reasoning, a combined deployment of temporary SRM and large-scale CDR may politically become more attractive.
The logic of debt – borrowing emissions and temperature
In overshoot and peak-shaving scenarios, SRM and CDR are in a mutually dependent strategic relationship—SRM is used temporarily for controlling temperature before large-scale CDR can be realized, whereas CDR is deployed as a longer-term exit strategy to reduce atmospheric CO2 concentration to the point where SRM is no longer needed (Long & Shepherd, Citation2014). Because of this reciprocity, orchestrating SRM and CDR in combination might sound like—to the ears of systems engineers—a ‘symphony for harmonized actions’ (Long, Citation2017). However, rather than composing a symphony, their combination might alternatively commit the world to a high-risk gamble (cf. Anderson & Peters, Citation2016; Shue, Citation2017).
To understand this gamble, the notion of debt is crucial. Insomuch as debt rests on a promise to pay back in the future (Adkins, Citation2017), it weakens the responsibilities of the present. In overshoot and peak-shaving scenarios, the role of SRM and CDR is to compensate for delayed mitigation. SRM buys time for mitigation (and CDR) by offsetting ongoing warming due to excess emissions (Keith & MacMartin, Citation2015; Wigley, Citation2006); CDR accommodates slow rates of mitigation by promising that delays can be compensated later through net negative emissions (Anderson & Peters, Citation2016; Meadowcroft, Citation2013). We can therefore read overshooting and peak-shaving alike as acts of ‘borrowing’ or ‘creating debt’. Overshooting is borrowing carbon emissions (an ‘emissions debt’ or ‘carbon debt’ (Carton, Citation2019; Geden, Citation2016)) to avoid near-term emissions reduction, a debt which is to be paid back later by CDR. Peak-shaving is borrowing global temperature (a ‘temperature debt’ (Oschlies, Citation2018)) by deploying SRM to cancel warming above the target threshold until the point when no further borrowing, of either sort, is needed. Either way, the world is placed in debt: whether emissions debt and/or temperature debt ().
Figure 1. Schematic illustration of carbon dioxide emissions and global mean temperature change up to 2100. (a) Emissions debt results from the near-term excess of emissions in the overshoot compared to the non-overshoot scenario. (b) Temperature debt results from the temporary masking of warming committed by excess emissions and which is over and above the target temperature. All curves are merely illustrative.
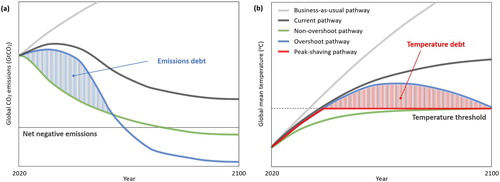
Macdougall, Zickfeld, Knutti, and Matthews (Citation2015) find that, in their climate model simulations, ‘overshoot net carbon budget’ (i.e. net cumulative CO2 emissions following exceedance of and return to a warming target) is smaller than the conventional carbon budget consistent with achieving a warming target without overshoot.Footnote1 In other words, if cumulative emissions exceed a particular warming target, restoring that warming target requires that more carbon will need eventually to be removed from the atmosphere than the actual amount by which the original carbon budget was exceeded (Macdougall et al., Citation2015; Zickfeld, Macdougall, & Matthews, Citation2016). This means that CDR has to pay back the emissions debt with ‘interest’, i.e. the difference between net-overshoot and conventional carbon budgets, where the size of the interest payment depends on the magnitude of overshoot.
In overshoot and peak-shaving scenarios, the role of CDR is to repay a debt. In contrast, SRM acts only to create a debt—this is why SRM is by itself ‘imperfect’ (Keith et al., Citation2010). Temperature debt is created by the temporary masking of warming by SRM. This debt can only be written-off when CDR eventually succeeds in paying off the emissions debt. In this sense, peak-shaving can be seen as a form of ‘debt financing’ or ‘financial leverage’ that uses the temperature debt to facilitate ‘investment’ in masking the excess warming. This ‘investment’ keeps the global temperature constant. If successful, such leveraging can generate a great return, namely the write-off of the temperature debt. However, if the emissions debt is not paid back, the temperature debt will have to be extended indefinitely for continuing leveraged investment in warming-offset above the target temperature. Or else, the debtor will be forced to file for ‘bankruptcy’, i.e. a sudden termination of SRM with the associated dangerous spike in warming (Matthews & Caldeira, Citation2007). The sudden failure of such leveraged investment would then result in exceptionally severe losses and damage that otherwise would not have occurred (Jones et al., Citation2013; McCusker, Armour, Bitz, & Battisti, Citation2014).
The analogy with subprime mortgage lending
The nature of indebtedness accompanied by overshoot and peak-shaving scenarios—and the interdependence between SRM and CDR—can be illustrated further by using the analogy of subprime mortgage lending. We analogize CO2 emissions and global temperature to a ‘mortgage’ and a ‘home equity’ respectively—future emissions reduction equates to the mortgage repayment, while a temperature increase equates to a decline in home equity (see ).
Figure 2. Analogical illustration of temperature overshoot and peak-shaving scenarios with mortgage and home equity. (a) Different ‘mortgage repayment’ schedules for overshoot and non-overshot scenarios (corresponding to CO2 emissions pathway with a relative difference from current pathway as a baseline case). (b) Different approaches to managing ‘home equity’ of global temperature (corresponding to temperature pathway with a relative change from the 2020 levels). The categorization of six approaches (business-as-usual, mitigation, CDR, SRM, adaptation, impacts and suffering) is based on Long and Shepherd (Citation2014).
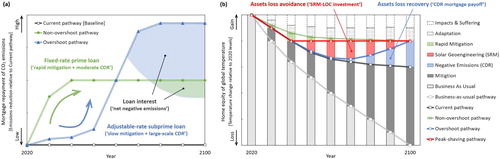
Imagine that you plan to buy a new house and promise your child inheritors that you will maintain its value as best you can for their benefit. However, being short of savings, you secure a mortgage from the bank and promise to pay it back by the date of inheritance transfer. The bank then securitizes your mortgage and sells it as a mortgage-backed security to your children who become the ultimate lenders of your mortgage loan. Nevertheless, you remain financially constrained and so not only are repayments delayed, but you also under-maintain the house so its value diminishes. You therefore leverage additional funds (second-lien loansFootnote2) from the bank to retain your house’s equity value under these delayed mortgage repayment conditions. In this deal on second lien, the bank makes a special arrangement so that if you duly completed your mortgage repayment and there were no outstanding first-mortgage loans by the due date, the leveraged second-lien loans could be written-off. This deal is made possible only when house price desired by the child investors is realized at the date of inheritance transfer.
Here, we can read the Paris Agreement as the imaginary contract of transferring a house between parents and children at the agreed house price (i.e. 1.5–2°C). To secure this contract, the bank may arrange different mortgage lending schemes (i.e. emissions scenarios) for prime or subprime borrowers, depending on their credit scores. Importantly, since the bank securitizes the mortgage and then sells it to the child investors, children play a dual role both as mortgage lenders and as heirs to the house. (For a schematic illustration of our analogy with mortgage lending and securitization, see .)
Figure 3. Simple schematic diagram of mortgage lending and securitization. During the process, financial intermediaries such as banks play an important role as mortgage originators and/or security underwriters. Investors in mortgage-backed security (MBS) become the ultimate lenders of mortgage loans. In the real financial market, investors would often decide which mortgage bonds to buy, based on the information provided by credit rating agencies.
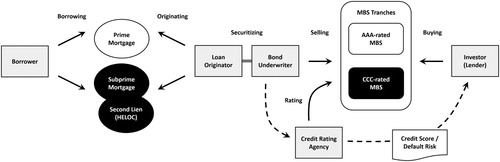
The different emissions pathways between overshoot and non-overshoot scenarios indicate differences to the loan scheme and the repayment schedule ((a)). Non-overshoot scenarios are somewhat similar to prime loan lending. Here, debtors are obligated to repay aggressively in the short term, but the rate of repayment is largely flat in the long run so that planned repayment can be assured. In contrast, overshoot scenarios are the adjustable-rate loan for subprime borrowers. Because the borrower’s current income level is not high enough, they are allowed low-rate or minimum repayments for a little while, but are then expected to repay later with a far higher rate facilitated by assumed income growth. As we analogize emissions reductions to mortgage repayments, crossing the line into the ‘net negative emissions’ (or ‘emissions below zero’) territory can be interpreted as making an ‘interest payment’ on top of the ‘principal repayment’. Here, the size of net-negative emissions can be seen as the ‘interest’ on the loan.Footnote3 As subprime loans come with much higher interest payments than do prime loans, overshoot scenarios require a larger volume of net negative emissions than do non-overshoot scenarios.Footnote4 We liken overshoot scenarios to subprime loans because their repayment depends crucially on the unproven promise of large-scale CDR deployment (Anderson & Peters, Citation2016; Field & Mach, Citation2017; Fuss et al., Citation2014; Lenzi et al., Citation2018; Williamson, Citation2016). Large-scale CDR has no assurance of solvency, so its credit rating must be scored accordingly.
Meanwhile, the resulting temperature pathways suggest different approaches to managing home equity ((b)). For example, if no mitigation actions are taken (the ‘business-as-usual’ case), the house value will greatly diminish. In contrast, rapid and deep mitigation can prevent such a sharp decline in value, although some degree of depreciation is unavoidable as temperature rises to at least 1.5–2°C. In this respect, peak-shaving by temporary SRM is borrowing a ‘home-equity-line-of-credit’ (HELOC) as a form of leverage for house renovation so that the house value can be retained. Since HELOCs allow borrowers to withdraw funds as they need at any time during the draw period (up to the credit limit), temporary SRM can conveniently be used to adjust the amount of cooling through changing net radiative forcing (see Keith & MacMartin, Citation2015). Furthermore, HELOCs tend to have a small payment requirement during the draw period; the cost of SRM deployment is likewise cheap (Smith & Wagner, Citation2018). The key difference between HELOC and ‘SRM-line-of-credit’ (SRM-LOC) is that HELOCs must be repaid after the draw period ends, whereas there is no repayment period for SRM-LOCs. SRM-LOCs cannot be repaid on their own terms, but only written-off when the ‘emissions-mortgage’ has been paid off by simultaneous CDR. If these emissions ‘repayments’ become delinquent then the SRM ‘credit line’ needs to be extended accordingly. Because lenders are also inheritors, and rely on that inheritance, lenders have no option of foreclosure, but instead must extend the term and keep leveraging—otherwise, the result would be a collapse in the value of the house (i.e. a sudden termination of SRM), which would heavily damage a property that they are supposed to inherit (Jones et al., Citation2013; McCusker et al., Citation2014).
Drawing the above arguments together we suggest that, by analogy, temperature overshoot and peak-shaving is based on a combination of a subprime mortgage (i.e. an emissions debt) and a HELOC (i.e. a temperature debt). With this analogy, some important lessons can be drawn from the US subprime mortgage crisis in 2007–2009.Footnote5 One of them is that the opaquely structured mortgage securities were far too complex for investors to know the true riskiness of the underlying subprime mortgages (Gorton, Citation2009; Purnanandam, Citation2011). Substantial ratings inflation by credit rating agencies (Bolton, Freixas, & Shapiro, Citation2012; White, Citation2010) and widespread mortgage fraud by loan originators and security underwriters (Griffin & Maturana, Citation2016; Piskorski, Seru, & Witkin, Citation2015) were tragically responsible for obscuring their default risks, a situation conducive to creating subprime credit boom. As such, misreporting the default risk or inflating the credit rating of ‘overshoot’ scenarios is—intentionally or not—a precipitous step towards creating an analogous risk of a climate debt crisis.
Furthermore, the US subprime mortgage crisis was aggravated by excessive leverage through home-equity borrowings, such as HELOCs (Khandani, Lo, & Merton, Citation2013; Mian & Sufi, Citation2011). The risk of such aggressive leverage was unnoticed during periods of rising house prices. When house prices fell, however, because homeowners could not easily ‘deleverage’, the rate of loan defaults significantly increased. In the case of climate change, insofar as global temperature is kept constant regardless of the ability to reduce CO2 emissions, peak-shaving obscures the progress of emissions reductions, i.e. whether mortgage repayments are being met as planned or else being significantly delayed. This means that, by maintaining house prices, leverage via temporary SRM masks the risk of major default by subprime borrowers, that is, non-deployment of large-scale CDR technologies (cf. Demyanyk & van Hemert, Citation2011). As a consequence, far from complementing mitigation, SRM may actually risk further delaying serious mitigation (cf. McLaren, Citation2016), that is, sinking deeper into climate debt. Crucially, temporary SRM is impossible to be deleveraged gradually unless large-scale CDR were rolled out very swiftly and significant progress in conventional mitigation were made. Thus, the combined use of temporary SRM and large-scale CDR (i.e. aggressive household leverage with subprime mortgage) may very well cause a similar risk of systemic failure, putting the world on the verge of a hidden climate debt crisis.
Conclusion
To meet the Paris climate goal, the idea of an ‘overshoot and peak-shaving’ scenario is emerging. The logic of debt (Adkins, Citation2017) helps to deepen understanding of this scenario, which uses CDR and SRM as a temporary strategy to compensate for delayed mitigation. Overshooting is borrowing emissions from the future to defer mitigation in the present by promising to pay off later through large-scale CDR; peak-shaving is borrowing temperature through temporary SRM to mask—but not erase—the warming from excess emissions, thereby buying time for mitigation and CDR. Paying off the debt of delayed mitigation will eventually translate into the social and ecological costs of deploying large-scale CDR technologies (Field & Mach, Citation2017; Williamson, Citation2016). This can be considered unjust and unsustainable for future generations because it might seriously harm efforts to meet their own human needs by increasing the risks of hunger, biodiversity loss and water stress (Dooley & Kartha, Citation2018; Shue, Citation2017).
Furthermore, the analogy with subprime loans signals to us that, because of the mutually dependent strategic relationship between the two ‘geoengineering’ technologies in overshoot and peak-shaving scenarios, SRM and CDR should not be discussed separately. The efficacy of temporary SRM cannot be evaluated in isolation of the feasibility of large-scale CDR. When the concept of temporary SRM is proposed by its advocates, it sounds like a modest and plausible idea (Keith & MacMartin, Citation2015). However, the ‘success’ of temporary SRM hinges critically on the promise of massively scaling-up CDR within reasonably short periods of time. Any failure of this overshoot promise would necessitate prolonged—potentially endless—peak-shaving. SRM would then become not a temporary but a permanent ‘line of credit’. The risk of incessant SRM (i.e. obscuring an ever-rising climate debt), together with the risk of unplanned termination (Parker & Irvine, Citation2018), must therefore be fully assessed and communicated before its deployment is considered seriously. In our view, framing SRM as temporary usage by counting on the unproven promise of large-scale CDR is reckless because it is by definition a means of debt financing. Crucially, SRM cannot be deleveraged on its own.
Some may argue that the strategy of temperature overshoot and peak-shaving can offer a safe passage away from dangerous climate change (see Steffen et al., Citation2018). However, given the unreliable promise of debt repayments, it is unwise and irresponsible to present this speculative scenario as a secured feasible investment strategy for the world. We have already seen the real-world consequences of poor decision-making in respect of the US subprime mortgage crisis. Such a strategy might help manage some of the risks associated with future climate change, just as borrowing helps people to buy a house or to maintain its value. But it may also very likely place the world in a situation of escalating ‘climate debt’. The failure to communicate properly the riskiness of an overshoot and peak-shaving strategy is the dangerous first step towards being trapped in this potential climate debt crisis, as happened in the failure to appropriately rate the riskiness of subprime mortgage bonds in the run-up to the US housing market crisis. Overshoot and peak-shaving scenarios should be seen as gambling future sustainability against a set of insecure promises.
Acknowledgements
We are grateful to Rob Bellamy, Holly Buck, Oliver Geden, Anders Hansson and Nils Markusson for their thoughts on an early version of the article. We also thank three anonymous reviewers and the journal editor Joanna Depledge for their careful readings of our paper and helpful comments and suggestions.
Disclosure statement
No potential conflict of interest was reported by the authors.
ORCID
Shinichiro Asayama http://orcid.org/0000-0001-6817-3862
Mike Hulme http://orcid.org/0000-0002-1273-7662
Additional information
Funding
Notes
1 This difference in the size of carbon budgets is caused by the lagged response of temperature to CO2 decline during the transition from positive emissions to negative emissions, which is mainly due to ocean thermal inertia (Macdougall et al., Citation2015).
2 Lien is the legal right that a creditor can have a claim to seize the debtor’s collateral property when a debtor fails to meet the obligations of a loan. A home-equity loan or line-of-credit is often considered a ‘second mortgage’ or ‘second-lien loan’ using home as a collateral, a debt that places its holders second in line to be repaid in the case of default.
3 Note that the interest on ‘mortgage loans’ defined here is not the same as the interest associated with the ‘emissions debt’ discussed previously. This is because the definition of ‘principal’ in each case is different. For emissions debt, the principal is the ‘excess emissions over the target’s carbon budget’. For ‘mortgage loans’, since we use the current pathway as our baseline case (see (a)), the principal is the ‘cumulative net-positive emissions gap with current pathway’. The difference in the total mortgage repayments (including the loan interest) between the overshoot and non-overshoot scenarios is then equal to the amount of the interest on the emissions debt, i.e. the difference between the net-overshoot and conventional carbon budgets.
4 There are always ‘residual non-CO2 emissions’ from some greenhouse gas sources (e.g. CH4, N2O), which require to some degree net-negative CO2 emissions to compensate for non-CO2 forcing and stabilize temperature, even in non-overshoot scenarios (Rogelj et al., Citation2015; Holz et al., Citation2018; Obersteiner et al., Citation2018).
5 Of course, the reasons why the subprime mortgage crisis in the US housing market triggered the global financial crisis are rather more complex (see, for example, Acharya & Richardson, Citation2009; Gorton, Citation2009). Note that our analogy is not intended to capture the full picture of either crisis.
References
- Acharya, V., & Richardson, M. (2009). Causes of the financial crisis. Critical Review, 21(2–3), 195–210. doi: 10.1080/08913810902952903
- Adkins, L. (2017). Speculative futures in the time of debt. Sociological Review, 65(3), 448–462. doi: 10.1111/1467-954X.12442
- Anderson, K., & Peters, G. (2016). The trouble with negative emissions. Science, 354(6309), 182–183. doi: 10.1126/science.aah4567
- Bolton, P., Freixas, X., & Shapiro, J. (2012). The credit ratings game. Journal of Finance, 67(1), 85–111. doi: 10.1111/j.1540-6261.2011.01708.x
- Boucher, O., Forster, P. M., Gruber, N., Ha-Duong, M., Lawrence, M. G., Lenton, T., … Vaughan, N. E. (2014). Rethinking climate engineering categorization in the context of climate change mitigation and adaptation. Wiley Interdisciplinary Reviews: Climate Change, 5(1), 23–35. doi: 10.1002/wcc.261
- Carton, W. (2019). ‘Fixing’ climate change by mortgaging the future: Negative emissions, spatiotemporal fixes, and the political economy of delay. Antipode, 51(3), 750–769. doi: 10.1111/anti.12532
- Demyanyk, Y., & van Hemert, O. (2011). Understanding the subprime mortgage crisis. Review of Financial Studies, 24(6), 1848–1880. doi: 10.1093/rfs/hhp033
- Dooley, K., & Kartha, S. (2018). Land-based negative emissions: Risks for climate mitigation and impacts on sustainable development. International Environmental Agreements: Politics, Law and Economics, 18(1), 79–98. doi: 10.1007/s10784-017-9382-9
- Field, C. B., & Mach, K. J. (2017). Rightsizing carbon dioxide removal. Science, 356(6339), 706–707. doi: 10.1126/science.aam9726
- Fuss, S., Canadell, J. G., Peters, G., Tavoni, M., Andrew, R. M., Ciais, P., … Yamagata, Y. (2014). Betting on negative emissions. Nature Climate Change, 4(10), 850–853. doi: 10.1038/nclimate2392
- Gasser, T., Guivarch, C., Tachiiri, K., Jones, C. D., & Ciais, P. (2015). Negative emissions physically needed to keep global warming below 2°C. Nature Communications, 6, 7958. doi: 10.1038/ncomms8958
- Geden, O. (2016). The Paris Agreement and the inherent inconsistency of climate policymaking. Wiley Interdisciplinary Reviews: Climate Change, 7, 790–797. doi: 10.1002/WCC.427
- Geden, O., & Löschel, A. (2017). Define limits for temperature overshoot targets. Nature Geoscience, 10, 881–882. doi: 10.1038/s41561-017-0026-z
- Geden, O., Peters, G., & Scott, V. (2019). Targeting carbon dioxide removal in the European Union. Climate Policy, 19(4), 487–494. doi: 10.1080/14693062.2018.1536600
- Gorton, G. B. (2009). The subprime panic. European Financial Management, 15(1), 10–46. doi: 10.1111/j.1468-036X.2008.00473.x
- Griffin, J. M., & Maturana, G. (2016). Who facilitated misreporting in securitized loans? Review of Financial Studies, 29(2), 384–419. doi: 10.1093/rfs/hhv130
- Holz, C., Siegel, L. S., & Johnston, E. (2018). Ratcheting ambition to limit warming to 1.5°C–trade-offs between emission reductions and carbon dioxide removal. Environmental Research Letters, 13, 064028. doi: 10.1080/14693062.2017.1397495
- Huntingford, C., & Lowe, J. (2007). ‘Overshoot’ scenarios and climate change. Science, 316(5826), 829. doi: 10.1126/science.316.5826.829b
- IPCC. (2018). Global Warming of 1.5°C. Geneva: World Meteorological Organization.
- Irvine, P. J., Kravitz, B., Lawrence, M. G., & Muri, H. (2016). An overview of the Earth system science of solar geoengineering. Wiley Interdisciplinary Reviews: Climate Change, 7(6), 815–833. doi: 10.1002/wcc.423
- Jones, A., Haywood, J. M., Alterskjær, K., Boucher, O., Cole, J. N. S., Curry, C. L., … Yoon, J. H. (2013). The impact of abrupt suspension of solar radiation management (termination effect) in experiment G2 of the geoengineering model Intercomparison Project (GeoMIP). Journal of Geophysical Research Atmospheres, 118, 9743–9752. doi: 10.1002/jgrd.50762
- Keith, D. W., & MacMartin, D. G. (2015). A temporary, moderate and responsive scenario for solar geoengineering. Nature Climate Change, 5, 201–206. doi: 10.1038/nclimate2493
- Keith, D. W., Parson, E., & Morgan, M. G. (2010). Research on global sun block needed now. Nature, 463(28), 426–427. doi: 10.1038/463426a
- Khandani, A. E., Lo, A. W., & Merton, R. C. (2013). Systemic risk and the refinancing ratchet effect. Journal of Financial Economics, 108(1), 29–45. doi: 10.1016/j.jfineco.2012.10.007
- Knutti, R., & Rogelj, J. (2015). The legacy of our CO2 emissions: A clash of scientific facts, politics and ethics. Climatic Change, 133(3), 361–373. doi: 10.1007/s10584-015-1340-3
- Lenzi, D., Lamb, W. F., Hilaire, J., Kowarsch, M., & Minx, J. C. (2018). Weigh the ethics of plans to mop up carbon dioxide. Nature, 561, 303–305. doi: 10.1038/d41586-018-06695-5
- Le Quéré, C., Andrew, R. M., Friedlingstein, P., Sitch, S., Hauck, J., Pongratz, J., … Zheng, B. (2018). Global carbon budget 2018. Earth System Science Data, 10, 2141–2194. doi: 10.5194/essd-10-2141-2018
- Lomax, G., Workman, M., Lenton, T., & Shah, N. (2015). Reframing the policy approach to greenhouse gas removal technologies. Energy Policy, 78, 125–136. doi: 10.1016/j.enpol.2014.10.002
- Long, J. C. S. (2017). Coordinated action against climate change: A new world symphony. Issues in Science and Technology, 33(3), 78–82.
- Long, J. C. S., & Shepherd, J. G. (2014). The strategic value of geoengineering research. In B. Freedman (Ed.), Global Environmental Change (pp. 757–770). Dordrecht: Springer doi: 10.1007/978-94-007-5784-4_24
- Macdougall, A. H., Zickfeld, K., Knutti, R., & Matthews, H. D. (2015). Sensitivity of carbon budgets to permafrost carbon feedbacks and non-CO2 forcings. Environmental Research Letters, 10, 125003. doi: 10.1088/1748-9326/10/12/125003
- MacMartin, D. G., & Kravitz, B. (2019). Mission-driven research for stratospheric aerosol geoengineering. Proceedings of the National Academy of Sciences, 116(4), 1089–1094. doi: 10.1073/pnas.1811022116
- MacMartin, D. G., Ricke, K. L., & Keith, D. W. (2018). Solar geoengineering as part of an overall strategy for meeting the 1.5°C Paris target. Philosophical Transactions of the Royal Society A, 376, 20160454. doi: 10.1098/rsta.2016.0454
- Matthews, H. D., & Caldeira, K. (2007). Transient climate-carbon simulations of planetary geoengineering. Proceedings of the National Academy of Sciences, 104(24), 9949–9954. doi: 10.1073/pnas.0700419104
- McCusker, K. E., Armour, K. C., Bitz, C. M., & Battisti, D. S. (2014). Rapid and extensive warming following cessation of solar radiation management. Environmental Research Letters, 9, 024005. doi: 10.1088/1748-9326/9/2/024005
- McLaren, D. (2016). Mitigation deterrence and the “moral hazard” of solar radiation management. Earth's Future, 4, 596–602. doi: 10.1002/2016EF000445
- Meadowcroft, J. (2013). Exploring negative territory carbon dioxide removal and climate policy initiatives. Climatic Change, 118(1), 137–149. doi: 10.1007/s10584-012-0684-1
- Mian, A., & Sufi, A. (2011). House prices, home equity-based borrowing, and the US household leverage crisis. American Economic Review, 101(5), 2132–2156. doi: 10.1257/aer.101.5.2132
- Minx, J. C., Lamb, W. F., Callaghan, M. W., Fuss, S., Hilaire, J., Lenzi, D., … del Mar Zamora, M. (2018). Negative emissions—Part 1: Research landscape, ethics and synthesis. Environmental Research Letters, 13, 063001. doi: 10.1088/1748-9326/aabf9b
- Niemeier, U., & Tilmes, S. (2017). Sulfur injections for a cooler planet. Science, 357(6348), 246–248. doi: 10.1126/science.aan3317
- Obersteiner, M., Bednar, J., Wagner, F., Gasser, T., Ciais, P., Forsell, N., … Schmidt-Traub, G. (2018). How to spend a dwindling greenhouse gas budget. Nature Climate Change, 8, 7–10. doi: 10.1038/s41558-017-0045-1
- O’Neill, B. C., Oppenheimer, M., Warren, R., Hallegatte, S., Kopp, R. E., Pörtner, H. O., … Yohe, G. (2017). IPCC reasons for concern regarding climate change risks. Nature Climate Change, 7(1), 28–37. doi: 10.1038/NCLIMATE3179
- Oschlies, A. (2018). Temperature debt of solar geoengineering. Nature, 554, 423. doi: 10.1038/d41586-018-02203-x
- Parker, A., & Geden, O. (2016). No fudging on geoengineering. Nature Geoscience, 9(12), 859–860. doi: 10.1038/ngeo2851
- Parker, A., & Irvine, P. J. (2018). The risk of termination shock from solar geoengineering. Earth’s Future, 6, 456–467. doi: 10.1002/2017EF000735
- Peters, G., & Geden, O. (2017). Catalysing a political shift from low to negative carbon. Nature Climate Change, 7, 619–621. doi: 10.1038/nclimate3369
- Piskorski, T., Seru, A., & Witkin, J. (2015). Asset quality misrepresentation by financial intermediaries: Evidence from the RMBS market. Journal of Finance, 70(6), 2635–2678. doi: 10.1111/jofi.12271
- Purnanandam, A. (2011). Originate-to-distribute model and the subprime mortgage crisis. Review of Financial Studies, 24(6), 1881–1915. doi: 10.1093/rfs/hhq106
- Ricke, K. L., Millar, R. J., & MacMartin, D. G. (2017). Constraints on global temperature target overshoot. Scientific Reports, 7, 14743. doi: 10.1038/s41598-017-14503-9
- Ricke, K. L., Moreno-Cruz, J. B., Schewe, J., Levermann, A., & Caldeira, K. (2016). Policy thresholds in mitigation. Nature Geoscience, 9, 5–6. doi: 10.1038/ngeo2607
- Rogelj, J., den Elzen, M., Höhne, N., Fransen, T., Fekete, H., Winkler, H., … Meinshausen, M. (2016). Paris Agreement climate proposals need boost to keep warming well below 2°C. Nature, 534, 631–639. doi: 10.1038/nature18307
- Rogelj, J., Schaeffer, M., Meinshausen, M., Knutti, R., Alcamo, J., Riahi, K., & Hare, W. (2015). Zero emission targets as long-term global goals for climate protection. Environmental Research Letters, 10, 105007. doi: 10.1088/1748-9326/10/10/105007
- Royal Society. (2009). Geoengineering the Climate: Science, Governance and Uncertainty. London: Royal Society.
- Shue, H. (2017). Climate dreaming: Negative emissions, risk transfer, and irreversibility. Journal of Human Rights and the Environment, 8(2), 203–216. doi: 10.4337/jhre.2017.02.02
- Smith, P., Davis, S. J., Creutzig, F., Fuss, S., Minx, J., Gabrielle, B., … Yongsung, C. (2016). Biophysical and economic limits to negative CO2 emissions. Nature Climate Change, 6, 42–50. doi: 10.1038/nclimate2870
- Smith, S. J., & Rasch, P. J. (2013). The long-term policy context for solar radiation management. Climatic Change, 121(3), 487–497. doi: 10.1007/s10584-012-0577-3
- Smith, W., & Wagner, G. (2018). Stratospheric aerosol injection tactics and costs in the first 15 years of deployment. Environmental Research Letters, 13, 124001. doi: 10.1088/1748-9326/aae98d
- Stanton, E. A., Ackerman, F., & Kartha, S. (2009). Inside the integrated assessment models: Four issues in climate economics. Climate and Development, 1(2), 166–184. doi: 10.3763/cdev.2009.0015
- Steffen, W., Rockström, J., Richardson, K., Lenton, T., Folke, C., Liverman, D., … Schellnhuber, H. J. (2018). Trajectories of the Earth system in the Anthropocene. Proceedings of the National Academy of Sciences, 11(33), 8252–8259. doi: 10.1073/pnas.1810141115
- Sugiyama, M., Arino, Y., Kosugi, T., Kurosawa, A., & Watanabe, S. (2018). Next steps in geoengineering scenario research: Limited deployment scenarios and beyond. Climate Policy, 18(6), 681–689. doi: 10.1080/14693062.2017.1323721
- Tilmes, S., Sanderson, B. M., & O’Neill, B. C. (2016). Climate impacts of geoengineering in a delayed mitigation scenario. Geophysical Research Letters, 43, 8222–8229. doi: 10.1002/2016GL070122
- UNEP. (2018). Emissions Gap Report 2018. Nairobi: United Nations Environment Programme.
- van Vuuren, D. P., Hof, A. F., van Sluisveld, M. A. E., & Riahi, K. (2017). Open discussion of negative emissions is urgently needed. Nature Energy, 2, 902–904. doi: 10.1038/s41560-017-0055-2
- White, L. J. (2010). Markets: The credit rating agencies. Journal of Economic Perspectives, 24(2), 211–226. doi: 10.1257/jep.24.2.211
- Wigley, T. M. L. (2006). A combined mitigation/geoengineering approach to climate stabilization. Science, 314, 452–454. doi: 10.1126/science.1131728
- Williamson, P. (2016). Scrutinize CO2 removal methods. Nature, 530, 153–155. doi: 10.1038/530153a
- Zickfeld, K., Macdougall, A. H., & Matthews, H. D. (2016). On the proportionality between global temperature change and cumulative CO2 emissions during periods of net negative CO2 emissions. Environmental Research Letters, 11, 055006. doi: 10.1088/1748-9326/11/5/055006