ABSTRACT
The agricultural sector is a major contributor to greenhouse gas emissions (GHG) in Ethiopia, as it is the basis of the economy and the primary source of employment. This study investigated the implementation of mitigation and adaptation practices in smallholder farms in Ethiopia, estimated GHG emissions associated with mitigation practices, and identified potential mitigation options and barriers and enabling factors for implementation. Twenty-five smallholder farmers were selected by a local development agency and interviewed in the field about their land use and land management practices and the Mitigation Options Tool (MOT) was used to estimate GHG emissions, to identify mitigation options and co-benefits, and as a platform for promoting learning and knowledge exchange across different types of stakeholders. All farmers interviewed in the field acknowledged changes in the climate, but only some were implementing adaptation practices to cope with such changes, namely, crop rotations, planting new crop types, and the early sowing of crops. Fewer mitigation practices were implemented, namely reduced tillage and application of manure in cereal crops and potatoes. These practices were mainly implemented because of their benefits for soil conservation (e.g. fertility, soil water holding capacity, yield stabilization, erosion avoidance) rather than for mitigation (carbon sequestration) purposes. Greenhouse gas emissions from the application of synthetic fertilizer to crops, and from livestock production varied widely across farmers depending on the amount of fertilizer applied and the number and type of livestock raised. Tenancy rights and extension services were identified as potential enablers of the adoption of climate change mitigation and adaptation practices by smallholder farmers in Ethiopia, and competing uses for straw was a potential barrier for the incorporation of residues in the soil. Barriers and enabling factors should be assessed thoroughly through further engagement with farmers as well as data on the amount of organic matter added to the soil, as these practices have co-benefits in terms of soil conservation, which are especially relevant for climate change adaptation in semi-dry climates. The MOT could be used in the future as a facilitator for knowledge exchange between researchers and practitioners in Ethiopia, and in other developing countries where data availability is low, to support the identification of effective climate change mitigation and adaptation actions.
Key policy insights
Climate change mitigation practices in agriculture can provide co-benefits for adaptation and food security, including the stabilization of crop yields, especially in semi-dry climates; more evidence about these co-benefits is needed.
The systematic collection of data on manure and crop residues should be supported as a priority as well as the identification of implementation barriers for mitigation and adaptation practices.
Smallholder farmers need to be engaged throughout any assessment of climate change mitigation potential to raise awareness, identify co-benefits of possible actions, and to identify and address barriers for implementation.
Tenancy rights and extension services are potential enabling factors for the adoption of climate change mitigation and adaptation measures by smallholder farmers in Ethiopia.
In developing countries, user-friendly tools such as the MOT can promote learning and knowledge exchange across diverse stakeholder groups about the impact of land use and management options on climate change mitigation and adaptation.
1. Introduction
Human-induced climate change is already affecting regions across the globe with observed extreme weather events including heatwaves, heavy precipitation, droughts, and tropical cyclones (IPCC, Citation2021). This is already contributing to rapid, broad-scale ecosystem changes (Dasgupta, Citation2021). Global warming of 1.5°C and 2°C are projected to be exceeded during the twenty-first century unless drastic reductions in carbon dioxide (CO2) and other greenhouse gas (GHG) emissions occur in the coming decades. Many African countries, despite having contributed little to climate change and having low GHG emissions, face exponential collateral damage that poses systemic risks to their economies, to their infrastructure investments, and to their water and food systems, public health, agriculture, and livelihoods (African Development Bank, Citation2021; Burke et al., Citation2018). Agriculture, which greatly contributes to the gross domestic product (GDP) of many African nations and provides a major source of employment, is highly sensitive to temperature and precipitation variations (African Development Bank, Citation2019). In East Africa, climate change is projected to increase median temperature by 1.4–5.5°C and median precipitation by −2–20% by the end of the twenty-first century (Adhikari et al., Citation2015), causing an overall decrease in yields of staple crops (Challinor et al., Citation2014) through the shortening of the growing season length, the amplification of water stress and the increased incidence of pest and disease outbreaks (Niang et al., Citation2014). Climate change is becoming the most threatening phenomenon that affects the livelihoods of subsistence farmers (Ali, Citation2021). Ethiopia, located in East Africa, is one of the world’s most drought-prone countries and faces numerous development challenges that exacerbate its vulnerability to climate change (African Development Bank, Citation2019; USAID, Citation2016). These challenges include low level of economic development, heavy dependence on rain fed agriculture and high population growth (Conway & Schipper, Citation2011; Eshetu, Citation2014). The major adverse impacts of climate change in Ethiopia include food insecurity (from droughts and floods), disease outbreaks (namely malaria, dengue fever, water borne diseases (e.g. cholera, dysentery) related to floods, and respiratory diseases related to droughts), land degradation due to heavy rainfall, and damage to communication, roads and other infrastructure by floods (Gezie, Citation2019). Agriculture is the basis of Ethiopia's economy and the primary source of employment for its population (Deressa et al., Citation2009). Ethiopian agriculture contributes to about 44% of the country’s GDP, about 70% of the export earnings, and approximately 80% of all employment (FAO, Citation2014; Zerihun et al., Citation2016). Irrigation use in Ethiopia is extremely low, with an estimated 97% of food crops grown in rain fed agriculture (Bachewe et al., Citation2015). Most projections of future climate in Ethiopia agree that the frequency of hot days and nights will substantially increase, and about 15–29% of days will be considered hot by 2060 (USAID, Citation2016). It is anticipated that climate change will mostly impact smallholder farmers across the world due to their low adaptive capacity (Harvey et al., Citation2014). On the other hand, agriculture, and more specifically livestock-related activities, is also a major contributor of GHG emissions in the country (Berhanu et al., Citation2019). Data from the United Nations Food and Agriculture Organization (UN FAO) show that agricultural emissions have been steadily increasing in Ethiopia from about 53 million tonnes of carbon dioxide equivalent (MtCO2eq) in 1993, to around 117 MtCO2eq in 2019 (FAOSTAT, Citation2021) ().
Figure 1. Greenhouse gas emissions from agriculture in Ethiopia in MtCO2eq. (Source: FAOSTAT, Citation2021).
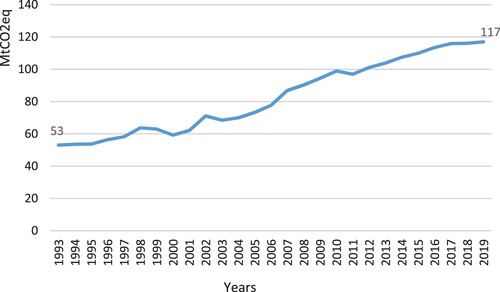
After COP21 in 2015, the Nationally Determined Contributions (NDCs) to the Paris Agreement (the legally binding international treaty on climate change), have become the main instrument for guiding policy responses to climate change in the African continent (World Meteorological Organization, Citation2020). The Government of Ethiopia, one of the 196 country signatories of the Paris Agreement, submitted its NDC in 2020, stating that the country plans to reduce the economy-wide GHG emissions by 220.59 MtCO2eq by 2030 (−53.5% of the country’s total GHG emissions in relation to the 2010 Business-as-Usual (BAU) scenario) (Government of Ethiopia, Citation2020; Redda, Citation2015). These plans include emission reduction targets and mitigation options for all sectors of the economy and are linked to the 2011 Climate-Resilient Green Economy (CRGE) Strategy, which aims at keeping GHG emissions low, and building climate resilience, while achieving middle-income status by 2025 (Teklewold et al., Citation2018). Specific objectives include reducing agricultural emissions, protecting and expanding forests, expanding renewable electricity generation, and adopting energy efficient technologies in transport, industry and the built environment (Climate Action Tracker, Citation2019). A new CRGE is being prepared for the period 2021–2030, which updates the first NDC submitted in 2017, and presents the methodology used to identify and prioritize mitigation and adaptation contributions, as well as the resources required for the implementation of the enhanced NDC (Government of Ethiopia, Citation2020). The Government of Ethiopia has also since submitted an NDC update (Government of Ethiopia, Citation2021). It was submitted in July 2021. Despite being at the frontline of climate policy for low-income countries, the reach of the Ethiopian state to implement programmes in the rural sector remains limited (Paul & Weinthal, Citation2019).
The UNFCCC recognizes that ‘not all developing countries have sufficient capacities to deal with many of the challenges brought by climate change’ (UNFCCC, Citation2021). Therefore, the Paris Agreement emphasizes the need for climate-related capacity-building for developing countries and appeals to all developed countries to increase their support for actions in developing countries (UNFCCC, Citation2021). One way to undertake capacity-building in developing countries is through the use of practical tools that can support interested stakeholders to better understand the problem and identify actions to solve the problem. To identify climate change mitigation actions and practices in the land use sector (agriculture, forestry and other land uses), numerous carbon emission accounting tools have been developed, including the Mitigation Options Tool (MOT).Footnote1 The MOT can conduct context-specific analysis needed to make evidence-based decisions on the prioritization of climate-smart practices, with the objective of reducing GHG emissions. Carbon emission accounting tools can also be used as an interface between science, policy and practice (Whittaker et al., Citation2013), as they are useful to communicate the scale and severity of the climate change challenge; they can, therefore, contribute to the planning and design of national, regional and local level actions that will help countries to accomplish their NDCs (Denef et al., Citation2012; Krueger et al., Citation2012; Peters et al., Citation2017).
In fact, behaviour change literature has identified a need for more inclusive and engaging media to better communicate climate change science (Lorenzoni et al., Citation2007; Moser & Dilling, Citation2007). There is also a growing requirement for tool developers to engage with potential users and experts to improve the quality, acceptance and integration of the tools in different country settings (Hare, Citation2011) as these can facilitate collaborative learning about climate change mitigation (Basco-Carrera et al., Citation2017; Hare, Citation2011). Continuous use and testing of carbon emission accounting tools by stakeholders can be helpful to track how much people learn, while also supporting tool development. In addition, stakeholders can share their concerns and perspectives with tool developers and jointly improve their problem-solving skills (Krueger et al., Citation2012). This study contributes to the wider literature on the accounting of GHG emissions and carbon sequestration, and on the identification of climate change mitigation options with co-benefits for adaptation and food security (e.g. increased/stabilized yields) in small-scale agriculture. At the same time, it explores the ability of the MOT to work as an interface between researchers, stakeholders and farmers for the promotion of effective learning and knowledge exchange about the contribution of land management to climate change. Further, Altieri and Nicholls (Citation2017) show that the climate change mitigation research community clearly needs to engage with small farmers regarding agriculture mitigation and adaptation potential, in order to jointly plan and design enabling conditions that can support the resilience of traditional agriculture and ensure food security. This could impact on the measurement and development of actions that reduce emission reductions and, thus, contribute to the achievement of countries’ NDCs. This study aims to understand the management practices in smallholder farms in Ethiopia, the GHG emissions associated with these practices, and the adoption of mitigation and adaptation options in smallholder farms. The study also aims to test the ability of the MOT to facilitate stakeholder learning about land management and its implications for climate change mitigation and adaptation.
2. Methods and materials
2.1 The Mitigation Options Tool (MOT)
The MOT was used in Ethiopia to undertake rapid assessments of the contribution of land and livestock management practices to GHG emissions and carbon sequestration, and to serve as an interface between research and practice for improved knowledge exchange and learning. The MOT was developed to support farmers, policy advisors and agricultural extension services in choosing management practices that reduce GHG emissions without risking crop yields (Feliciano et al., Citation2017). It is an Excel-based tool, freely available onlineFootnote2, that encompasses several empirical models to estimate GHG emissions in rice, cropland and livestock systems, and agroforestry, and it provides information about the most effective mitigation options. GHG emissions are estimated in terms of kilograms of carbon dioxide equivalent per hectare (kg CO2eq ha−1) and kilograms of carbon dioxide equivalent per unit of product (kg CO2eq kg−1). Baseline management practices are chosen by the user and a set of mitigation options are ranked according to their mitigation potential. The MOT allows different levels of input to be specified from a preliminary to a more comprehensive level, depending on the objectives of the users, who might have different backgrounds, and on the availability of input data. The MOT undertakes farm-level specific assessments of GHGs and mitigation potentials to be made without the need for expert knowledge or for lengthy model set-up and calibration. The methods used to estimate GHG emissions from the application of synthetic fertilizer, from animal enteric fermentation, manure management and animal feed, as well as mitigation options and potential, especially for the incorporation of manure in agricultural soils, are described in Feliciano et al. (Citation2017). The MOT does not include information about barriers that farmers face to implement mitigation options. These barriers can be farm-level barriers (for example, land tenure, access to infrastructure and complementary inputs (such as irrigation) or farmers’ education level), national level barriers (such as governmental taxes and incentives), or global barriers (such as the commodity markets or climate change).
2.2. Study site
Data were collected in two different locations (5 km apart) in the Tula catchment region located in the Doyogena district, Kembata Tembaro Zone, Southern Nations, Nationalities, and Peoples’ Region of Ethiopia (SNNPR) (). Geographically, it is positioned between 7° 16′ 48″ to 7° 19′ 1″ North-latitude and 37° 45′ 36″ to 37° 47′ 2″ East-longitude. The altitude of the region varies from 2620 to 2740 m above sea level, with the mean annual minimum and maximum temperature of 12.6°C and 20°C, respectively, and mean annual rainfall between 1000 and 1400 mm. The climate is tepid sub-humid mid-highland. According to the FAO soil classification, the watershed soils are Chromic Luvisols. These soils have high clay content in the sub-soil and are susceptible to erosion on steep slopes. The agriculture sector plays a central role in the community’s livelihoods. The major farming system of the area is mixed enset or false banana (Ensete ventricosum) with cereals and livestock production. The major cereal crops include wheat, barley, potato, beans, and peas. Livestock production includes cattle, sheep and poultry. There are 345 households in the Tula watershed and most people are subsistence farmers with an average land size of 0.5 hectares. The main source of income is the marketing of wheat, pulses, potatoes, livestock and livestock products, and rural and urban off-farm labour. According to the local wealth ranking in this region, 21% of the households are rich, 50% are medium income and 29% are poor.
2.3. Field data collection
A questionnaire was designed to collect relevant field data from farmers on farm management practices and perceptions of climate change (Appendix 1, Supplementary Material). This data served as input to the MOT for estimation of GHG emissions and for the identification of mitigation options. The questionnaire included five groups of questions about: (1) the field site, (2) crops, (3) agroforestry and livestock management practices, (4) the socio-economic characteristics of the farmers (e.g. age, education level, off-farm activities), and (5) farmer’s perceptions of climate change and practices already being implemented to adapt to climate change.
Twenty-five farmers (20 male and 5 female) aged between 25 and 70 years old were engaged in the study. Thirteen farmers had no formal education, and 12 farmers were educated from the 2nd to the 10th grade. Farmers were recruited by the non-governmental French organization Inter AideFootnote3, which has an office and technicians in the Tula catchment. Each individual interaction lasted about 35 minutes and fieldwork lasted one day in total. Two fieldwork teams of six people each (PhD and MSc students and lecturers), together with dedicated translators, asked the questions to the farmers individually at the two field locations. Before the start of each interaction, farmers were informed about the aims of the study and their consent to participate in the study was sought. After acceptance, farmers were informed that their names would not be associated with responses to the interview questions and that they could withdraw anytime they wanted.
2.4. GHG emissions estimation and mitigation option identification
Thirty-six stakeholders, selected by the International Livestock Research Institute (ILRI), attended a workshop organized by the research team in Addis Ababa to estimate GHG emissions from agricultural activities (more specifically, GHG emissions from synthetic fertilizer application in wheat, barley and potato production, and GHG emissions from livestock production) and to identify potential mitigation options. Stakeholders included university students, researchers from national universities and from the Ethiopian Institute of Agricultural Research (EIAR), practitioners from non-governmental organizations (NGOs), and civil servants working at the Environment, Forest and Climate Change Commission (EFCCC) and the Ethiopian Ministry of Agriculture (MoA). shows the number of participants per stakeholder type represented at the workshop. In order to promote stakeholder learning, the workshop structure followed the learning strategy approach suggested by Harris (Citation2014), namely to ‘reflect’, ‘select’ and ‘rewrite’ the information. The workshop was facilitated by two interdisciplinary researchers who are specialists in participatory action research. This approach followed Haider et al. (Citation2018), who indicate that skills in facilitation, namely participatory approaches and the ability to see ‘the big picture’, are valuable skills in sustainability science.
The workshop was structured in four parts. First, the research team presented the concepts of climate change and climate smart agriculture, and introduced the MOT, in plenary. Second, groups of 5–6 stakeholders used the MOT to estimate GHG emissions from synthetic fertilizer application to wheat, barley and potatoes, and from livestock production with the data collected in the field; the MOT was also used to identify options for GHG emissions reduction and carbon sequestration, i.e. climate change mitigation options. As these mitigation options were not presented back to farmers to ask if they would be willing to implement them, stakeholders at the workshop were encouraged to discuss possible barriers to implementing the mitigation options. Third, stakeholders presented the results of the practical exercises and group discussion in the plenary. Fourth, they discussed the improvements needed in the MOT and the effectiveness of the MOT to provide useful information on climate change mitigation in agriculture. At the end of the workshop, stakeholders were asked to answer a questionnaire (Appendix 2, Supplementary Material) in order to individually evaluate the ability of the MOT to generate relevant knowledge for climate change planning purposes.
3. Results
3.1. Farmer’s perceptions of climate change and adaptation practices
All farmers in the study (n = 25) mentioned they have noticed changes in weather patterns. They specifically mentioned decreased rainfall, increased temperature, decreased cloudiness and increased sunshine hours. They also mentioned increased weather unpredictability and changing patterns. Some farmers also identified impacts of climate change, such as lack of water and disappearance of water springs, increased crop diseases (including insect-related diseases), decreased crop yields, and delays in the start of the crop season. Nine farmers considered that warmer and sunnier days, rather than colder and cloudier days, were positive changes. One farmer further considered that the rainfall amount in the previous cropping season was optimum for crop production and that climate change was creating better growing and living conditions for crops and humans, respectively.
Eighteen farmers mentioned they were implementing agricultural practices to adapt to changes in climate. Practices included crop rotations, the planting of new crop types (e.g. Irish potatoes, change from barley to wheat), early sowing of crops, adoption of improved wheat varieties, introduction of terraces, increase area of enset plots (or increase number of trees), boundary planting, introduction of desho grass (Pennisetum pedicellatum) strips as cover crops, chemical use for crop diseases and increase fertilizer application to improve crop yield. They also mentioned changes in livestock management, such as adoption of local breeds and reduction of livestock numbers to manageable herd sizes. Other practices include undertaking off-farm activities, i.e. livelihood diversification, storing products in the good years to compensate for the bad years, and seeking information and advice from government agricultural officers and development agents. Seven farmers stated they are not implementing any practices to adapt to changing climate and weather conditions, apart from praying to God.
3.2. Estimation of GHG emissions from crop and livestock production and identification of mitigation options using the MOT
3.2.1. Input data for the MOT
Information collected in the field included data on land management practices, for example, the application of synthetic and organic fertilizer application or crop residues, on the different crops produced, namely wheat, barley and potato. Information also included livestock numbers and type (e.g. cow, donkey and ox), livestock management practices, and agroforestry types and management practices. Only some farmers, however, provided the amount of manure and compost applied to soils; therefore, GHG emissions and soil carbon sequestration due to this practice could not be quantified comprehensively. In opposition, the amount of synthetic fertilizer per type of crop was provided by the farmers and its contribution to GHG emissions was estimated. shows a summary of the information collected in the field. This information was used as input data for the MOT to estimate GHG emissions from crop and livestock production during one workshop with stakeholders (Section 2.4). Therefore, the effects of land management practices applied by the smallholder farmers in the field were estimated using the MOT. A more comprehensive database of the information collected is provided as Supplementary Material.
Table 1. Summary of field data collected on climate, land use, land use and land cover change, management practices applied in cropland, livestock and agroforestry, and farm products.
3.2.2. GHG emissions from synthetic fertilizer application and livestock production
show the distribution of GHG emissions per hectare across the 25 farmers visited. In wheat, GHG emissions from synthetic fertilizer varied from 0.53 and 2.6 t CO2eq ha−1, in barley from 0.3 to 3.6 t CO2eq ha−1 and in potatoes from 0.55 to 8.3 t CO2eq ha−1. GHG emissions from the application of synthetic fertilizer application in potatoes were higher than for barley and wheat ().
Figure 4. Greenhouse gas emissions per hectare from synthetic fertilizer application in wheat, barley and potato in t CO2eq ha−1 (N = 25).
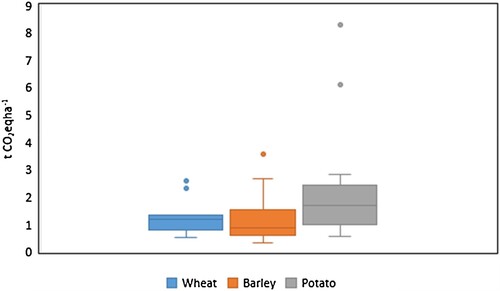
GHG emissions sources from livestock production include animal enteric fermentation, manure management, and production of livestock feed. The animals considered were cows, oxen and sheep, which were the main types of livestock raised by the farmers visited in mixed farming systems. Estimated GHG emissions from livestock ranged between 1.5 and 7.8 t CO2eq across the 25 farmers visited. As the average area managed by each farmer was 0.25 ha, this corresponds to between 6.1 and 31.4 t CO2eq ha−1 ().
3.2.3. Identification of mitigation options and mitigation potential using the MOT
The MOT was used by the workshop participants to identify mitigation options for the 25 farmers. The example of Farmer 1 is described: Farmer 1, for example, reported a yield of 1600 kg ha−1 for wheat and crop management practices such as reduced tillage, no cover crops, no manure addition, compost and crop residues not incorporated in the soil, application of 400 kg ha−1 of urea fertilizer and of 400 kg ha−1 of di-ammonium phosphate, both without nitrogen inhibitors. By inserting these inputs in the MOT, it identified mitigation options that could be independently implemented by farmer 1, namely optimum timing of nitrogen (N) with the best technology, optimum nitrogen (N) application, planting cover crops, implementation of no-tillage, addition of manure to the soil, compost application to the soil, addition of straw in the soil or returning at least 50% of crop residues to the soil, application of fertilizer with nitrogen inhibitor and application of polymer-coated fertilizer. shows a screenshot taken from the online MOT with the mitigation options ranked from the highest mitigation potential to the lowest mitigation potential for the specific case of farmer 1.
Figure 6. Screenshot of the MOT presenting a suggested list of mitigation options and mitigation potential (in kg CO2eq ha−1 yr−1) for farmer 1.
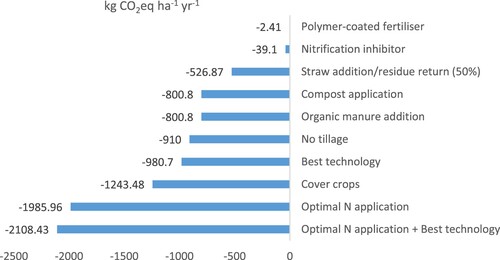
The total mitigation potential of the measure ‘addition of manure’ in wheat across the 25 farmers in this study could be obtained by multiplying the mitigation potential of manure addition provided by the MOT () by the number of farmers who do not add manure to the soil ().
Table 2. Total mitigation potential of manure application in wheat production across the 25 farmers.
In terms of barriers to the implementation of mitigation options, only one was identified by farmers who mentioned that wheat straw and enset leaves were used for animal feed, and therefore the measure ‘straw addition/residue return (50%)’ was not feasible.
3.3. Stakeholders’ assessment of the MOT
During the workshop, stakeholders were invited to reflect on the ability of the MOT to promote effective learning. Stakeholder quotes during the group presentations to the audience revealed that ‘Participants quickly understood the [Mitigation Options] tool and were capable of using it all the way from independent data entry to analysis and interpretation’ and ‘The Mitigation Options Tool is an easy way to learn about GHG emissions and carbon sequestration in agroecosystems’. Stakeholders’ answers to the questionnaire also provided an assessment of the MOT. These are presented in Appendix 3 (Supplementary Material – Appendices) together with their views on the usefulness of the MOT. Many stakeholders indicated they would use the MOT in the future for research projects, PhD research, scientific articles, teaching lectures at university, training purposes, to provide technical advice and consultancy, and for reporting on the NDC. Nevertheless, stakeholders suggested developments in the MOT with the objective to improve the performance and usefulness of the MOT in Ethiopia and for countries with low-data availability. They suggested to contextualize the MOT to the Ethiopian context by integrating specific crops such as teff (Eragrostis tef) and enset (Ensete ventricosum), and the GHG emission factors related to enset processing and fermentation or the inclusion of oxen ploughing as a soil tillage option. They also suggested to increase visibility of practices that sequester carbon, to include soil and water conservation practices or to include cost–benefit analysis for climate mitigation options. A more comprehensive list of improvement suggestions is provided in Appendix 4 (Supplementary Material – Appendices). Stakeholders mentioned their willingness to collaborate and suggested the implementation of large-scale national level projects for data collection and database creation, and for further development of the MOT to the Ethiopian context.
4. Discussion
4.1. Smallholder farmers’ perceptions of climate change impacts in Ethiopia
In this study, farmers mentioned they were noticing changes in the weather and were adapting to those changes. Perceived weather changes included less rainfall, higher temperatures, less cloudy weather, increased sunshine hours, less water in streams and boreholes, increased crop diseases and insect-related human diseases, decreased crop yields, and delays in the start of the crop season. These perceptions coincide with farmers’ perceptions in Ethiopia as reported by Paul and Weinthal (Citation2019) and Hameso (Citation2018). Increasing temperature and decreasing rainfall trends were also perceived by farmers in the goat based agro-pastoral livelihood zone in Ethiopia (Mihiretu et al., Citation2021). Th perception amongst smallholder farmers of changes in temperature and rainfall in recent years has also been documented elsewhere in Africa, notably in West Africa (Ali, Citation2021).
4.2. The contribution of crop and livestock production to GHG emissions
Stakeholders at the workshop estimated the effects of specific land and livestock management practices on GHG emissions using the MOT. GHG emissions from synthetic fertilizer application varied between 0.5 and 2.6 t CO2eq ha−1 in wheat, 0.3 and 3.6 t CO2eq ha−1 in barley and 0.5 and 8.3 t CO2eq ha−1 in potatoes. As a point of comparison, in South Africa, where farm inputs are expected to be higher, GHG emissions due to the application of synthetic fertilizer and crop residues in wheat and barley production were estimated with the Agriculture and Land Use National Greenhouse Gas Inventory (ALU) SoftwareFootnote4; they amounted 1.99 t CO2eq ha−1 and 0.41 t CO2eq ha−1 for wheat and barley, respectively (Tongwane et al., Citation2016). Estimates from the ALU Software are thus within the ranges provided by the MOT (however the ALU Software requires a higher level of detail than the MOT and was thus not used in Ethiopia) (MacSween & Feliciano, Citation2018).
GHG emissions from livestock production estimated by stakeholders at the workshop with the MOT varied between 1.5 and 7.9 t CO2eq per farmer or between 6.1 and 31.4 t CO2eq ha−1 (the average area per farmer in Ethiopia is 0.25 ha), reflecting the different number of animals kept per farmer. Such a wide range also reflects the diversity of values across the farmers surveyed. Balehegn et al. (Citation2020) reveals that in most small-scale production systems in Africa, animals are fed with whatever is available to fill their rumen, without due consideration to the feed quality and animal requirement. In Ethiopia, Berhe et al. (Citation2020) found a higher share of GHG emissions from urban livestock production systems when compared to mixed crop/livestock and pastoral production systems in Ethiopia. The differences were due to the use of fossil fuels for feed production and processing, the use of grain as feed, the use of fertilization for feed production, and transportation of inputs in the urban production system. With African livestock already a significant contributor to GHG emissions and with numbers expected to increase in the future, GHG emissions as well as other negative environmental impacts of livestock will increase (Balehegn et al., Citation2021). Therefore, monitoring of livestock GHG emissions coupled with the identification of feasible mitigation and adaptation measures is essential. The MOT can support policy-makers and practitioners in this process.
4.3. Implementation of adaptation practices
In this study, most farmers mentioned they were changing practices to adapt to climate change; only few farmers stated they were not adapting to climate change. In the area visited, adaptation strategies included adoption of local livestock breeds, reduction of livestock numbers, increase in fertilizer application, planting barley instead of wheat, the early sowing of crops, boundary planting, introduction of desho grass strips, introduction of new crop types, introduction of terraces, and livelihood diversification. Bryan et al. (Citation2009) , however, reported that a large percentage of farmers surveyed in Ethiopia (∼60%) did not adjust their farming practices, despite having perceived changes in temperature and rainfall. More recently, Paul and Weinthal (Citation2019) reported that about 40% of their surveyed households in Ethiopia knew how to adapt to temperature and precipitation change while around 68% only had ‘some’ or ‘no ability’ to respond to future climate change. The use of resistance and high yielding varieties, crops and livestock integration, soil and water conservation practice, the use of organic fertilizer, and adjustment of sowing time are the most adopted farming practices. These findings are consistent with Gezie (Citation2019), who also found that Ethiopian farmers adopt many strategies in response to climate change, including the use of improved crop varieties, agroforestry practices, crop diversification, soil conservation practices, tree planting, off-farm activities, irrigation practices, adjusting planting dates, selling of assets, request of food aid, and permanent and temporary migration in search of employment. Farmers in the goat based agro-pastoral livelihood zone in Ethiopia who have perceived weather changes have implemented adaptation measures related to livestock, crop and non-agriculture strategies to ensure their livelihoods will endure climate change (Mihiretu et al., Citation2021).
Tenure security of the farmers visited in this study might explain the wider adoption of sustainable agricultural practices such as adaptation and mitigation. Teklewold et al. (Citation2018) found that in the Nile Basin in Ethiopia, the number of adaptation practices adopted was higher on owner-cultivated plots than on rented plots. Tarfasa et al. (Citation2017) also found that secured land tenancy rights play a critical role in Ethiopian landholder’s decision to invest in soil management technologies.
Most farmers also mentioned that they seek advice from government officers and international development agents, which reveals the existence of a network of agricultural extension services covering the territory visited. The availability of extension services in the area might be an enabling factor for the adoption of adaptation practices. Paul and Weinthal (Citation2019) found that in Ethiopia, extension services are possibly the only guidance farmers have on adaptation practices. Several authors (e.g. Mutyasira et al., Citation2018; Paul & Weinthal, Citation2019; Teklewold et al., Citation2018) found in Ethiopia that exposure to agricultural extension services positively influences the adoption of sustainable agricultural practices and the implementation of climate-related programmes, with the lack of access to extension services acting as a main barrier to adapt to climate change and variability (Gezie, Citation2019) and as a constraint that hampers farmers from taking any action in response to climate change (Mihiretu et al., Citation2021). Findings here are consistent with those from another study undertaken in Northern Togo, where the support of extension services to farmers was also found to be a key factor in enhancing farm household adaptability to climate change (Ali, Citation2021).
4.4. Implementation of mitigation options
Stakeholders used the MOT to identify potential mitigation options for smallholder farms during the workshop in Ethiopia. Mitigation practices suggested by the MOT include planting cover crops, no-tillage or reduced tillage, addition of organic fertilizer (e.g. manure and compost), optimum nitrogen application, addition of straw in the soil and application of fertilizer with nitrogen inhibitor. MacSween and Feliciano (Citation2018) previously identified the co-benefits of these mitigation options. Cover crops (e.g. with desho grass) increases soil organic carbon (SOC) storage in rotations with medium inputs (Ogle et al., Citation2005). Cover crops can also improve soil health, which can subsequently increase yields (Ogle et al., Citation2005; Olson et al., Citation2014). Cover crops can be planted before the main crops are planted, or in between established crops, with the objective of improving the physical, biological, and chemical properties of the soil. No-tillage can increase carbon sequestration potential through storage of soil organic matter in cropland (van Kessel et al., Citation2013). The application of manure increases soil organic matter content, which leads to improved soil water holding capacity and infiltration, drainage, aeration and biological activity, which enhances water use efficiency; at the same time that it removes atmospheric CO2 thus establishing agricultural soils as carbon sinks (Altieri & Nicholls, Citation2017; Marenya & Barrett, Citation2007). Other studies have also shown that the addition of organic manure can increase crop yield. Wang et al. (Citation2017) found that organic manure can be used when growing maize in dryland agriculture to improve soil environment and water productivity while increasing yield. Dordas et al. (Citation2008) report that synthetic fertilizer can be replaced by organic manure in maize production in northern Greece without a loss of yield. Applying an optimum amount of nitrogen fertilizer and optimizing the placement and time of application during the growing season could enhance efficiency and reduce associated nitrous oxide (N2O) emissions (Moran et al., Citation2011). Returning crop residues into the soil has the potential to increase SOC, to return essential nutrients such as nitrogen (N), phosphorous (P) and potassium (K) into the soil and this, in turn, can increase crop yield (Smith et al., Citation1997; Stagnari et al., Citation2014). The promotion of grasslands for animal feeding could be considered in order to release crop residues used from house construction to be available for soil incorporation. In livestock production, Berhe et al. (Citation2020), found that complementary feeding of maize grain to livestock, together with improvement of manure management and of herd productive and reproductive parameters (e.g. through breed improvement), applied either separately or in combination in the reduction of GHG emissions, more specifically enteric CH4 emissions.
However, a multitude of potential barriers exists that may prevent farmers from implementing climate change mitigation practices (Wreford et al., Citation2017). Failure by research and development organizations to take into consideration farmers’ preferences for soil and water conservation practices has resulted in low adoption of these technologies (Adimassu et al., Citation2013). It is crucial, therefore, to understand what the barriers for implementation of mitigation options are. For example, this study found that farmers mentioned crop residues are used both for livestock feed and for construction so the incorporation of crop residues in the soil is unlikely to be implemented as a mitigation option in small-scale farming. It is also important to understand the context in which practices are to be implemented, especially because some practices are highly site- and context-specific, and some practices that may act positively in one context may have adverse effects in another (Feliciano et al., Citation2017). Crop productivity in the Ethiopian highlands is critically affected by land degradation and climate change (Adego et al., Citation2018). Land degradation is a major socio-economic and environmental concern in the Ethiopian highlands where it has left enormous areas of fertile land unproductive (Adimassu et al., Citation2013). Mitigation options that can increase crop yields and soil water holding efficiency, such the addition of organic manure to the soil, are thus especially important in semi-arid environments, since this can both reduce land degradation and improve climate change adaptation alongside delivery of carbon sequestration mitigation benefits. For Smith et al. (Citation2007), the challenge for successful agricultural GHG mitigation is to remove barriers by implementing creative policies that take into account economic, social and environmental co-benefits such as improved food security (e.g. increased/stabilized yields). For example, Ali (Citation2021) showed that access to credit can increase the probability of the adoption of organic fertilizer as it can help farmers pay for the transportation costs to bring the organic fertilizer to their agricultural fields.
4.5. Effectiveness of the MOT to promote learning about the impacts of agricultural practices on climate change
The MOT was used as an interface between science, policy and practice during the workshop in Ethiopia with the objective to promote stakeholders’ learning on the impact of agricultural practices on climate change and on the potential of agricultural practices to mitigate climate change. According to Brown and Everard (Citation2015), researchers have the important role of deciphering local contexts and communicating their findings with decision-makers so that policies can be successfully designed and implemented. Fortmann and Ballard (Citation2011) believe that collaboration between scientists and stakeholders produces more relevant policy knowledge than the sole collaboration of scientists, without the inclusion of people affected by the policies at stake.
Data collection in the field, guided by the requirements of the MOT, as well as the workshop with a wide range of stakeholders, provided a conducive environment and a memorable experience for stakeholders, in comparison to only listening to oral presentations or reading and analysing results. Experiential learning was maximized in the activities in Ethiopia through the undertaking of field visits to a farming region, on-site interaction with smallholder farmers, interaction with a wide range of stakeholders, reflection on the process, and feedback to the research team, similar to the method developed by Feliciano et al. (Citation2019). A wide diversity of stakeholders from several Ethiopian regions, including both men and women, were involved in the activities. Fazey et al. (Citation2005) acknowledge the importance of experiential knowledge for effective environmental-related research and practice. Behrendt and Franklin (Citation2014) consider that experiential learning is authentic, first-hand and sensory-based. Atieno (Citation2009) considers that a good approach to understand a phenomenon is by viewing it in its context.
The workshop activities undertaken during the study allowed stakeholders to exchange ideas continuously, to provide feedback to the research team, and to adjust the activities to respond to the feedback provided. This contributed to effective stakeholder learning. The workshop also allowed stakeholders to learn through group exchanges and self-reflection on the knowledge gained during the preparation and delivery of presentations to the plenary, and through written feedback to the research team, respectively. For Bonwell and Eison (Citation1991) active learning happens when people ‘are actively or experientially involved in the learning process and where there are different levels of active learning, depending on [their] involvement’. Harris (Citation2014) believes that summarizing information improves learning since it makes individuals rewrite the main points or ideas in their own words, and it generates reflection about the information obtained as well as its relevance.
Feedback provided by stakeholders at the workshop leads to the conclusion that the MOT can generate effective learning. Additionally, all stakeholders indicated they would use the MOT in the future. One example given by stakeholders of where they would use the MOT is the implementation and monitoring of Ethiopian NDC and therefore contributing to national climate policy processes. Another use is teaching, with the capacity to expand the number of users in Ethiopia, and therefore further contribute to stakeholders’ learning. Additionally, the MOT can help stakeholders to identify synergies between mitigation and adaptation, taking into account co-benefits such as food security (e.g. increased/stabilized yields) and land and water management. If farmers are unable to identify any benefits to changing their practices, they will be unwilling to implement the measures without top-down policies (Wreford et al., Citation2017).
Stakeholders noted that the MOT was not specific to the Ethiopian context and suggested several changes that should be implemented to make it more suitable to the Ethiopian context (Appendix 4, Supplementary Material). In fact, the MOT was developed to be dynamic and iterative so that users in different countries can change emission factors and management options according to their needs (Feliciano et al., Citation2017). Many stakeholders indicated their willingness to form partnerships to develop the MOT in order to overcome this limitation; this would have the potential to improve shared knowledge and consequently co-learning about the relationship between agriculture and climate change. Funding to undertake further research to developing the MOT for specific countries should then be pursued. The activities undertaken with the MOT in Ethiopia can be reproduced in other developing countries with similar land use systems, and can contribute to trigger discussions on the impact of land management practices on GHG emissions, including the identification of potential mitigation options and the adaptation co-benefits, and the barriers related to the implementation of sustainable agricultural practices.
4.6. Further research and application of the MOT
In this study, smallholder farmers did not provide the amount of organic manure to soils comprehensively. Future studies on the assessment of GHG emissions in smallholder farming should thoroughly collect information about the amount of manure added to soils by farmers as this option is relevant for both soil carbon sequestration (mitigation) and increasing water holding capacity to stabilize yields (adaptation). Therefore, the method for data collection in the field should be improved with continuous engagement with farmers.
Stakeholders at the workshop suggested several improvements to the MOT, namely to contextualize it to Ethiopia by integrating specific crops such as teff (Eragrostis tef) and enset (Ensete ventricosum), having oxen ploughing as a soil tillage option, and including GHG emission factors related to enset processing and fermentation. They also suggested to increase visibility of practices that sequester carbon, to include soil and water conservation practices or to include cost–benefit analysis for climate mitigation options. Further research should contemplate these suggestions.
Follow-up studies should also include a comprehensive assessment of barriers to the implementation of mitigation options identified by the MOT by engaging with farmers in the field. In fact, when asked during the workshop, stakeholders did not manage to identify barriers to the implementation of the mitigation options in the field. This highlights the importance of working with farmers in order to have an effective design and implementation of climate change policies in the Global South. As pointed out by the United Nations (UN, Citation2019), smallholder farmers urgently require greater support and increased investment in infrastructure and technology for sustainable agriculture implementation. Adimassu et al. (Citation2013) also claim the need to strengthen farmers’ participation in the planning and development of best future alternatives. In addition, an assessment of the effectiveness of the mitigation options identified by the MOT in improving household food security should be also be pursued by engaging with farmers who would implement those options and asking whether they could benefit from the implementation in terms of increased or stabilized crop yields and improved access to a sufficient quantity of nutritious food.
A programme of training activities with agricultural extension agents, international development agents and farmers could be undertaken in Ethiopia using the MOT as a facilitator for knowledge exchange between science and practice. The agents could then use the increased knowledge acquired to base their advice to farmers on the adoption of climate change mitigation practices. Additionally, the MOT could be used in training activities with policy-makers and could, thus, prompt the creation of financial or non-financial incentives to support the adoption of mitigation practices.
Finally, a full assessment of the effectiveness of the MOT in promoting stakeholder learning about the relationship between land management and climate change is suggested by further contacting practitioners and policy-makers who participated in the workshop to ask if they are still using the MOT, how they are using it and for what purpose. Using the MOT within process of planning, design and monitoring of specific actions for the achievement of the NDC in the country would be a good indication of its effectiveness.
5. Conclusion
Management practices implemented by smallholder farmers in Ethiopia as mitigation measures were reduced tillage and incorporating manure in the soils. These practices are also beneficial for adaptation as they have the potential to sequester carbon through the storage of soil organic matter, which contributes to increased soil water holding capacity that is essential in drier climates and consequently stabilizes crop yields. The implementation of adaptation practices was much more common and included adoption of local livestock breeds, reduction of livestock numbers, increase in fertilizer application, planting barley instead of wheat, boundary planting, introduction of desho grass strips, early sowing of crops and livelihood diversification. This possibly reflects the changes in weather patterns noticed by all farmers in the study. GHG emissions from synthetic fertilizer application varied between 0.5 and 2.6 t CO2eq ha−1 in wheat, 0.3 and 3.6 t CO2eq ha−1 in barley and 0.5 and 8.3 t CO2eq ha−1 in potatoes, and GHG emissions from livestock production varied between 6.1 and 31.4 t CO2eq ha−1 across the farmers surveyed. In order to improve estimates, fieldwork planning should consider more time for detailed data collection, including barriers for implementation of mitigation and adaptation options through engagement with farmers.
In this study, feedback revealed that the activities undertaken with the MOT were successful at promoting a lively engagement with a diversity of stakeholders from several regions as well as the continuous exchange of ideas. User-friendly tools, such as the MOT, are essential to support transparency and learning across diverse stakeholder groups, especially in developing countries where the data available for analysis is low. This study tested with good results the applicability of the MOT to systematise data collection on land management practices and data analysis, as well as the ability of the MOT to serve as an interface between, science, policy and practice and to contribute to stakeholder learning about land management and climate change mitigation and adaptation in Ethiopia.
The MOT can be used in similar activities in other countries with the objective of generating discussion about the contribution of land management practices to climate change, the potential of the land use sector to mitigate climate change, as well as the co-benefits for climate change adaptation. The capacity of the MOT to influence change in land management at larger scale depends on the level of engagement and the effectiveness of the knowledge exchange activities (e.g. group fieldwork, capacity building workshops, discussions, classes and focus groups). These activities should create awareness among all stakeholders, including policy-makers, of the sources of emissions, practices to reduce emissions, the co-benefits of those practices, the barriers to implementation, and data gaps. Building on the MOT experience and results, and continuing with such knowledge sharing activities in future, could increase Ethiopia’s capacity and effectiveness to implement climate change mitigation programmes in the rural land use sector with co-benefits for adaptation and food security.
Supplemental Material
Download Zip (153.8 KB)Disclosure statement
No potential conflict of interest was reported by the author(s).
Additional information
Funding
Notes
References
- Adego, T., Simane, B., & Woldie, G. A. (2018). Sustainability, institutional arrangement and challenges of community based climate smart practices in northwest Ethiopia. Agriculture and Food Security, 7(1), 56. https://doi.org/10.1186/s40066-018-0206-0
- Adhikari, U., Nejadhashemi, A. P., & Woznicki, S. A. (2015). Climate change and Eastern Africa: A review of impact on major crops. Food Energy Security, 4(2), 110–132. https://doi.org/10.1002/fes3.61
- Adimassu, Z., Gorfu, B., Nigussie, D., Mowo, J., & Hilemichael, K. (2013). Farmers’ preference for soil and water conservation practices in Central highlands of Ethiopia. African Crop Science Journal, 21(s3), 781–790
- African Development Bank. (2019). Climate change impacts on Africa’s economic growth. Retrieved November 15, 2021, from https://www.afdb.org/sites/default/files/documents/publications/afdb-economics_of_climate_change_in_africa.pdf
- African Development Bank. (2021). Climate change in Africa. Retrieved November 11, 2021, from https://www.afdb.org/en/cop25/climate-change-africa
- Ali, E. (2021). Farm households’ adoption of climate-smart practices in subsistence agriculture: Evidence from Northern Togo. Environmental Management, 67(5), 949–996. https://doi.org/10.1007/s00267-021-01436-3
- Altieri, M. A., & Nicholls, C. I. (2017). The adaptation and mitigation potential of traditional agriculture in a changing climate. Climatic Change, 140(1), 33–45. https://doi.org/10.1007/s10584-013-0909-y
- Atieno, O. P. (2009). An analysis of the strengths and limitation of qualitative and quantitative research paradigms. Problems of Education in the 21st Century, 13, 13–18
- Bachewe, F. N., Berhane, G., Minten, B., & Taffesse, A. S. (2015). Agricultural growth in Ethiopia (2004-2014): Evidence and drivers. ESSP II Working Paper 81. Washington, D.C.: International Food Policy Research Institute (IFPRI). Retrieved June 30, 2021, from http://ebrary.ifpri.org/cdm/ref/collection/p15738coll2/id/129782
- Balehegn, M., Duncan, A., Tolera, A., Ayantunde, A. A., Issa, S., Karimou, M., Adesogan, A. T., Zampaligré, N., André, K., Gnanda, I., Varijakshapanicker, P., Kebreab, E., Dubeux, J., Boote, K., Minta, M., & Feyissa, F. (2020). Improving adoption of technologies and interventions for increasing supply of quality livestock feed in low and middle-income countries. Global Food Security, 26, 100372. https://doi.org/10.1016/j.gfs.2020.100372
- Balehegn, M., Kebreab, E., Tolera, A., Hunt, S., Erickson, P., Crane, T. A., & Adesogan, A. T. (2021). Livestock sustainability research in Africa with a focus on the environment. Animal Frontiers, 11(4), 47–56. https://doi.org/10.1093/af/vfab034
- Basco-Carrera, L., Warren, D., van Beek, E., Jonoski, A., & Giardino, A. (2017). Collaborative modelling or participatory modelling? A framework for water resources management. Environmental Modelling & Software, 91, 95–110. https://doi.org/10.1016/j.envsoft.2017.01.014
- Behrendt, M., & Franklin, T. (2014). A review of research on school field trips and their value in education. International Journal of Environmental and Science Education, 9(3), 235–245. https://doi.org/10.12973/ijese.2014.213a
- Berhanu, Y., Olav, L., Nurfeta, A., Angassa, A., & Aune, J. (2019). Methane emissions from ruminant livestock in Ethiopia: Promising forage species to reduce CH4 emissions. Agriculture, 9(6), 130. https://doi.org/10.3390/agriculture9060130
- Berhe, A., Bariagabre, S. A., & Balehegn, M. (2020). Estimation of greenhouse gas emissions from three livestock production systems in Ethiopia. International Journal of Climate Change Strategies and Management, 12(9), 669–685. https://doi.org/10.1108/IJCCSM-09-2019-0060
- Bonwell, C., & Eison, J. (1991). Active learning: Creating excitement in the classroom AEHE-ERIC higher education report No. 1. Washington, D.C.: Jossey-Bass.
- Brown, I., & Everard, M. (2015). A working typology of response options to manage environmental change and their scope for complementarity using an ecosystem approach. Environmental Science & Policy, 52, 61–73. https://doi.org/10.1016/j.envsci.2015.05.006
- Bryan, E., Deressa, T. T., Gbetibouo, G. A., & Ringler, C. (2009). Adaptation to climate change in Ethiopia and South Africa: Options and constraints. Environmental Science and Policy, 12(4), 413–426.
- Burke, M., Davis, W. M., & Diffenbaugh, N. S. (2018). Large potential reduction in economic damages under UN mitigation targets. Nature, 557(7706), 549–553. https://doi.org/10.1038/s41586-018-0071-9
- Challinor, A. J., Watson, J., Lobell, D. B., Howden, S. M., Smith, D. R., & Chhetri, N. (2014). A meta-analysis of crop yield under climate change and adaptation. Nature Climate Change, 4(4), 287–291. https://doi.org/10.1038/nclimate2153
- Climate Action Tracker. (2019). Retrieved June 30, 2021, from https://climateactiontracker.org/countries/ethiopia/
- Conway, D., & Schipper, E. L. F. (2011). Adaptation to climate change in Africa: Challenges and opportunities identified from Ethiopia. Global Environmental Change, 21(1), 227–237. https://doi.org/10.1016/j.gloenvcha.2010.07.013
- Dasgupta, P. (2021). The economics of biodiversity: The Dasgupta review. Abridged version. HM Treasury.
- Denef, K., Paustian, K., Archibeque, S., Biggar, S., & Pape, D. (2012). Report of greenhouse gas accounting tools for agriculture and forestry sectors. Interim report to USDA under Contract No. GS23F8182H.
- Deressa, T., Hassan, R., Ringler, C., Alemu, T., & Yesuf, M. (2009). Determinants of farmers’ choice of adaptation methods to climate change in the Nile Basin of Ethiopia. Global Environmental Change, 19(2), 248–255. https://doi.org/10.1016/j.gloenvcha.2009.01.002
- Dordas, C., Lithourgidis, A., Matsi, T., & Barbayiannis, N. (2008). Application of liquid cattle manure and inorganic fertilizers affect dry matter, nitrogen accumulation, and partitioning in maize. Nutrient Cycling in Agroecosystems, 80(3), 283–296. https://doi.org/10.1007/s10705-007-9143-1
- Eshetu, A. A. (2014). Microfinance as a pathway out of poverty and viable strategy for livelihood diversification in Ethiopia. Journal of Business Management and Economics, 5(6), 142–151
- FAO. (2014). Country fact sheet on food and agriculture policy trends. Retrieved December 27, 2021, from https://www.fao.org/3/i4181e/i4181e.pdf
- FAOSTAT. (2021). Retrieved June 30, 2021, from http://www.fao.org/faostat/en/#data/GT
- Fazey, I., Fazey, J. A., & Fazey, D. M. A. (2005). Learning more effectively from experience. Ecology and Society, 10(2), 4. https://doi.org/10.5751/ES-01384-100204
- Feliciano, D. M., Blagojević, D., Boehling, K., Hujala, T., Lawrence, A., Lidestav, G., Ludvig, A., Turner, T., Weiss, G., & Zivojinovic, I. (2019). Learning about forest ownership and management issues in Europe while travelling: The travellab approach. Forest Policy and Economics, 99, 32–42. https://doi.org/10.1016/j.forpol.2018.09.005
- Feliciano, D., & MacSween, K. (2018). Using the Mitigation Options Tool (MOT): Case studies. CCAFS info note. CCAFS Policy Briefs, CGIAR Research Program for Climate Change, Agriculture and Food Security (CCAFS), The Netherlands. Retrieved June 30, 2021, from https://ccafs.cgiar.org/resources/publications/using-mitigation-options-tool-ccafs-mot-case-studies
- Feliciano, D., Nayak, D. R., Vetter, S. H., & Hillier, J. (2017). MOT - A tool for farmers, extension services and policy-advisors to identify mitigation options for agriculture. Agricultural Systems, 154, 100–111. https://doi.org/10.1016/j.agsy.2017.03.006
- Fortmann, L., & Ballard, H. (2011). Sciences, knowledges, and the practice of forestry. European Journal of Forest Research, 130(3), 467–477. https://doi.org/10.1007/s10342-009-0334-y
- Gezie, M. (2019). Farmer’s response to climate change and variability in Ethiopia: A review. Cogent Food & Agriculture, 5(1), 1613770. https://doi.org/10.1080/23311932.2019.1613770
- Government of Ethiopia. (2020). Ethiopia's NDC update summary 2020. Retrieved June 30, 2021, from https://www4.unfccc.int/sites/ndcstaging/PublishedDocuments/Ethiopia%20First/Ethiopia%27s%20NDC%20update%20summary%202020.pdf
- Government of Ethiopia. (2021). Updated nationally determined contribution. Federal Republic of Ethiopia. Retrieved December 30, 2021, from https://www4.unfccc.int/sites/ndcstaging/PublishedDocuments/Ethiopia%20First/Ethiopia%27s%20updated%20NDC%20JULY%202021%20Submission_.pdf
- Haider, L. J., Hentati-Sundberg, J., Giusti, M., Goodness, J., Hamann, M., Masterson, V. A., Meacham, M., Merrie, A., Ospina, D., Schill, C., & Sinare, H. (2018). The undisciplinary journey: Early-career perspectives in sustainability science. Sustainability Science, 13(1), 191–204. https://doi.org/10.1007/s11625-017-0445-1
- Hameso, S. (2018). Farmers and policy-makers’ perceptions of climate change in Ethiopia. Climate and Development, 10(4), 347–359. https://doi.org/10.1080/17565529.2017.1291408
- Hare, M. (2011). Forms of participatory modelling and its potential for widespread adoption in the water sector. Environmental Policy and Governance, 21(6), 386–402. https://doi.org/10.1002/eet.590
- Harris, R. (2014). Learning strategy 3: Summarizing. Version date: February 27, 2014. Retrieved June 30, 2021, from https://www.virtualsalt.com/learn3.html
- Harvey, C. A., Rakotobe, Z. L., Rao, N. S., Dave, R., Razafimahatratra, H., Rabarijohn, R. H., Rajaofara, H., & MacKinnon, J. L. (2014). Extreme vulnerability of smallholder farmers to agri-cultural risks and climate change in Madagascar. Philosophical Transactions of the Royal Society B, 369(1639), 20130089. https://doi.org/10.1098/rstb.2013.0089
- IPCC. (2021). Climate change 2021: The physical science basis. Contribution of working group I to the sixth assessment report of the intergovernmental panel on climate change [Masson-Delmotte, V., P. Zhai, A. Pirani, S.L. Connors, C. Péan, S. Berger, N. Caud, Y. Chen, L. Goldfarb, M.I. Gomis, M. Huang, K. Leitzell, E. Lonnoy, J.B.R. Matthews, T.K. Maycock, T. Waterfield, O. Yelekçi, R. Yu, and B. Zhou (eds.)]. Cambridge University Press. In Press.
- Krueger, K., Page, T., Hubacek, K., Smith, L., & Hiscock, K. (2012). The role of expert opinion in environmental modelling. Environmental Modelling & Software, 36, 4–18. https://doi.org/10.1016/j.envsoft.2012.01.011
- Lorenzoni, I., Nicholson-Cole, S., & Whitmarsh, L. (2007). Barriers perceived to engaging with climate change among the UK public and their policy implications. Global Environmental Change, 17(3–4), 445–459. https://doi.org/10.1016/j.gloenvcha.2007.01.004
- MacSween, K., & Feliciano, D. (2018). Comparison of online greenhouse gas accounting tools for agriculture. CCAFS Info Note. Wageningen, The Netherlands: CGIAR Research Program for Climate Change, Agriculture and Food Security (CCAFS).
- Marenya, P. P., & Barrett, C. B. (2007). Household-level determinants of adoption of improved natural resources management practices among smallholder farmers in western Kenya. Food Policy, 32(4), 515–536. https://doi.org/10.1016/j.foodpol.2006.10.002
- Mihiretu, A., Okoyo, E. N., & Lemma, T. (2021). Causes, indicators and impacts of climate change: Understanding the public discourse in Goat based agro-pastoral livelihood zone, Ethiopia. Heliyon, 7(3), e06529. https://doi.org/10.1016/j.heliyon.2021.e06529
- Moran, D., MacLeod, M., Wall, E., Eory, V., McVittie, A., Barnes, A., Rees, B., Pajot, G., Matthews, R., Smith, P., & Moxey, A. (2011). Developing carbon budgets for UK agriculture, land-use, land-use change and forestry out to 2022. Climate Change, 105(3-4), 529–553. https://doi.org/10.1007/s10584-010-9898-2
- Moser, S. C., & Dilling, L. (2007). Creating a climate for change: Communicating climate change and facilitating social change. Cambridge University Press.
- Mutyasira, V., Hoag, D., Pendell, L. D., & Manning, D. (2018). Is sustainable intensification possible? Evidence from Ethiopia. Sustainability, 10(11). https://doi.org/10.3390/su10114174
- Niang, I., Ruppel, O. C., Abdrabo, M. A., Essel, A., Lennard, C., Padgham, J., Urquhart, P. (2014). Africa. In V. R. Barros, C. B. Field, D. J. Dokken, M. D. Mastrandrea, K. J. Mach, T. E. Bilir, M. Chatterjee, K. L. Ebi, Y. O. Estrada, R. C. Genova, B. Girma, E. S. Kissel, A. N. Levy, S. MacCracken, P. R. Mastrandrea, L. L. White, (Eds.), Climate change 2014: Impacts, adaptation, and vulnerability. Part B: Regional Aspects. Contribution of Working Group II to the Fifth Assessment Report of the Intergovernmental Panel on Climate Change (pp. 1199–1265). Cambridge, New York: Cambridge University Press.
- Ogle, S. M., Breidt, F. J., & Paustian, K. (2005). Agricultural management impacts on soil organic carbon storage under moist and dry climatic conditions of temperate and tropical regions. Biogeochemistry, 72(1), 87–121. https://doi.org/10.1007/s10533-004-0360-2
- Olson, K., Ebelhar, S., & Lang, J. (2014). Long-term effects of cover crops on crop yields, soil organic carbon stocks and sequestration. Open Journal of Soil Science, 4((08|8)), 284–292. https://doi.org/10.4236/ojss.2014.48030
- Paul, C. J., & Weinthal, E. (2019). The development of Ethiopia's climate resilient green economy 2011–2014: Implications for rural adaptation. Climate and Development, 11(3), 193–202. https://doi.org/10.1080/17565529.2018.1442802
- Peters, G. P., Andrew, R. M., Canadell, J. G., Fuss, S., Jackson, B. R., Korsbakken, J. I., Le Quéré, C., & Nakicenovic, N. (2017). Key indicators to track current progress and future ambition of the Paris agreement. Nature Climate Change, 7(2), 118. https://doi.org/10.1038/nclimate3202
- Redda. (2015). Coping with persistent drought in Awash, Ethiopia knowledge network. Retrieved June 30, 2021, from https://cdkn.org/2015/06/news-ethiopia-is-first-least-developed-country-to-submit-its-indc/?loclang=en_gb
- Smith, P., Martino, D., Cai, Z., Gwary, D., Janzen, H., Kumar, P., McCarl, B., Ogle, S., O’Mara, F., Rice, C., Scholes, B., Sirotenko, O., Howden, M., McAllister, T., Pan, G., Romanenkov, V., Schneider, U., & Towprayoon, S. (2007). Policy and technological constraints to implementation of greenhouse gas mitigation options in agriculture. Agriculture, Ecosystems & Environment, 118(1-4), 6–28. https://doi.org/10.1016/j.agee.2006.06.006
- Smith, P., Powlson, D., Glendining, M., & Smith, J. (1997). Potential for carbon sequestration in European soils: Preliminary estimates for five scenarios using results from long-term experiments. Global Change Biology, 3(1), 67–79. https://doi.org/10.1046/j.1365-2486.1997.00055.x
- Stagnari, F., Galieni, A., Speca, S., Cafiero, G., & Pisante, M. (2014). Effects of straw mulch on growth and yield of durum wheat during transition to conservation agriculture in Mediterranean environment. Field Crops Research, 137, 51–63. https://doi.org/10.1016/j.fcr.2014.07.008
- Tarfasa, S., Balana, B. B., Tefera, T., Woldeamanuel, T., Moges, A., Dinato, M., & Black, H. (2017). Modeling smallholder farmers’ preferences for soil management measures: A case study from South Ethiopia. Ecological Economics, 145, 410–419. https://doi.org/10.1016/j.ecolecon.2017.11.027
- Teklewold, H., Mekonnen, A., & Kohlin, G. (2018). Climate change adaptation: A study of multiple climate-smart practices in the Nile basin of Ethiopia. Climate and Development, 11(2), 180–192. https://doi.org/10.1080/17565529.2018.1442801
- Tongwane, M., Mdlambuzia, T., Moeletsia, M., Tsubo, M., Mliswa, V., & Grootbooma, L. (2016). Greenhouse gas emissions from different crop production and management practices in South Africa. Environmental Development, 19, 23–35. https://doi.org/10.1016/j.envdev.2016.06.004
- UN. (2019). Report of the secretary-general on SDG progress 2019 special edition. Retrieved June 30, 2021, from https://sustainabledevelopment.un.org/content/documents/24978Report_of_the_SG_on_SDG_Progress_2019.pdf
- UNFCCC. (2021). The Paris agreement. Retrieved June 30, 2021, from https://unfccc.int/process-and-meetings/the-paris-agreement/the-paris-agreement
- USAID. (2016). Climate change risk profile in Ethiopia: Country fact sheet. Retrieved June 30, 2021, from https://www.climatelinks.org/sites/default/files/asset/document/2016%20CRM%20Factsheet%20-%20Ethiopia_use%20this.pdf
- van Kessel, C., Venterea, R., Six, J., Adviento-Borbe, M. A., Linquist, B., & van Groenigen, K. J. (2013). Climate, duration, and N placement determine N2O emissions in reduced tillage systems: A meta-analysis. Global Change Biology, 19(1), 33–44. https://doi.org/10.1111/j.1365-2486.2012.02779.x
- Wang, X., Ren, Y., Zhang, S., Chen, Y., & Wang, N. (2017). Applications of organic manure increased maize (Zea mays L.) yield and water productivity in a semi-arid region. Agricultural Water Management, 187, 88–98. https://doi.org/10.1016/j.agwat.2017.03.017
- Whittaker, C., McManus, M. C., & Smith, P. (2013). A comparison of carbon accounting tools for arable crops in the United Kingdom. Environmental Modelling and Software, 46, 228–239. https://doi.org/10.1016/j.envsoft.2013.03.015
- World Meteorological Organization. (2020). Retrieved November 17, 2021 from https://library.wmo.int/doc_num.php?explnum_id=10421
- Wreford, A., Ignaciuk, A., & Gruère, G. (2017). Overcoming barriers to the adoption of climate-friendly practices in agriculture. OECD Food, Agriculture and Fisheries Papers, No. 101, OECD Publishing, Paris. Retrieved June 30, 2021, from https://www.oecd-ilibrary.org/agriculture-and-food/overcoming-barriers-to-the-adoption-of-climate-friendly-practices-in-agriculture_97767de8-en
- Zerihun, A., Wakiaga, J., & Kibret, H. (2016). Ethiopia: African economic outlook. AfDP, OECD, UNDP, pp. 1–14. Retrieved 30, 2021, from https://www.afdb.org/en/countries/east-africa/ethiopia/ethiopia-economic-outlook