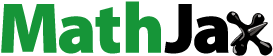
ABSTRACT
Despite its carbon neutrality commitments and the prospect of increasingly stringent climate policy measures, China is continuing to build new coal-fired power plants. In assessing the expected performance of these investments, it is helpful to analyse them through an ‘economic’ framework measuring a broader view of the country-level economic returns on new coal power investments, as a complement to the plant-level financial analysis framework commonly used to assess stranded asset risks. This simplified economic framework, in which inputs and outputs are measured according to the costs and benefits they generate for the national economy, leads to markedly different dynamics than financial analysis alone. This framework can help China to avoid ‘wasting’ scarce public resources by over-investing in new uneconomic power plants through its state-owned enterprises. Applying this approach to a representative new coal plant in China shows that modest shadow carbon pricing (rising from US$15/tCO2 in 2026, to US$30/tCO2 in 2041) eliminates the expected value of the project to China’s economy. Caps on coal-fired electricity generation have less impact on economic returns, but severely undermine financial returns, potentially making such caps a more effective short-term policy tool to dissuade company executives from making new coal investments. Without carbon pricing, only a moratorium on coal-fired power generation in 2030 or earlier prevents new plants from realizing a positive economic return. Comparing these results with an alternative solar/storage investment suggests the renewable option generates higher economic returns than the coal plant under modest shadow carbon pricing and lower electricity storage costs.
Key policy insights
Economic analysis of proposed coal plants complements plant-level financial analysis and better captures governments’ interests in these projects. Both economic and financial analyses are relevant to the decision-making of government shareholders.
State-led coal plant investments in China today is likely to be economically wasteful under modest future climate policy scenarios, particularly in light of declining levelized costs of renewable alternatives.
This framework for combined financial and economic analysis also applies to other countries’ proposed investments in carbon-intensive power generation. The framework is particularly pertinent for countries with state-led coal power investments planned, including India, Indonesia, Pakistan, South Africa, and Vietnam.
Introduction
As of July 2021, 297 GW of new coal power generation capacity was in the planning stages worldwide, with another 185 GW under construction (Global Energy Monitor et al., Citation2021). Large-scale net capacity additions of unabated coal-fired power is incompatible with the emissions reductions needed to achieve the temperature goals enshrined in the Paris Agreement, given that plants built in the 2020s would normally operate well into the 2050s (International Energy Agency, Citation2020a, Citation2021). Of the currently planned new capacity, India accounts for 21 GW, Vietnam 19 GW, Indonesia 18 GW, Turkey 12 GW, and Bangladesh 10 GW. Other countries planning coal power additions in excess of 4 GW include Laos, Mongolia, Zimbabwe, and Pakistan (Global Energy Monitor et al., Citation2021). In many of these countries, new coal investments are likely to be carried out by power companies wholly – or majority-owned by the state rather than private investors, with the public sector thereby providing a significant portion of investment capital for coal expansion.
It is China, however, that dominates planned coal power at 163 GW; 56% of the global total. In September 2020, China announced its aim to peak carbon dioxide (CO2) emissions ‘before 2030’ and become carbon neutral by 2060 (Normile, Citation2020). The International Energy Agency (Citation2020b, p. 396) estimates that coal power contributes half of China’s energy sector CO2 emissions, at 4.9GTCO2 in 2019. Energy transition pathways consistent with Paris Agreement targets see China’s coal-fired electricity generation falling 25% by 2030, and 60% by 2040, relative to 2018 (International Energy Agency, Citation2019). J. He et al. (Citation2020) suggest Paris compliance will require China to eliminate unabated coal power generation by 2050.
Electricity demand in China is expected to increase by 60–75% over 2018 levels by 2040, whether or not a Paris-compliant development pathway is followed (International Energy Agency, Citation2019). Electricity’s share of the primary energy mix is likely to rise from 45% to 75% by 2040 (He, Citation2020). A range of power technologies are available for meeting this demand (G. He et al., Citation2020), but despite China’s long-term emissions reduction targets, coal capacity is continuing to expand. Commissioning of new coal capacity in 2020 totalled 38.4 GW, and approvals for new plants reached 36.9 GW, exceeding the 28.5 GW approved in 2017–19 combined (Shearer & Myllyvirta, Citation2021).Footnote1
Newly built coal plants will join a coal fleet already seeing low utilization rates (China Electric Power Statistics and Data Center, Citation2020). Additionally, competition from renewable alternatives is increasing. Levelized cost of electricity estimates suggest that solar and wind power generation are already competitive across most of China’s provinces (Gray & Sundaresan, Citation2020a). Further, G. He et al. (Citation2020) find that under optimistic cost projections for renewables, 62% of China’s electricity could come from non-fossil sources by 2030 at 11% lower cost than business-as-usual, and power sector emissions can be reduced by 50% at 6% lower cost.
Stranded coal assets: current approaches
This paper seeks to fill an important gap in the existing literature on stranded asset risks for thermal coal power. Existing studies largely define stranded assets one of two ways. ‘Financial’ analysis defines them as expected or realized financial losses for corporate owners (Spencer et al., Citation2017), consistent with accepted definitions in the wider literature (Caldecott et al., Citation2013) and global or regional stranded asset calculation methodologies (Mercure et al., Citation2018). ‘Technical’ analysis identifies stranded capacity (GW) or electricity generation (TWh), using a sectoral carbon budget or policy setting as a starting point and identifying stranded assets by the extent to which the early retirement or under-utilization of power generation infrastructure occurs under various policy pathways or carbon budget constraints (Caldecott et al., Citation2017; Caldecott et al., Citation2018; Johnson et al., Citation2015; Pfeiffer et al., Citation2018).
These approaches are helpful guides, but do not consider what stranded asset risks imply for governments, which are often the ultimate arbiters of whether or not new coal power projects should proceed. This is particularly true in China, as well as those countries where the government is the majority or sole shareholder in most power companies and associated electricity generation projects. From a government planner’s perspective, expectations of financial losses and early retirement do not necessarily imply that building a new coal plant is unjustified on an economic basis. Indeed, governments subsidize financially risky projects precisely because they consider them to be economically worthwhile. Could this be the case for coal? To the best of the authors’ knowledge, project-level evaluation of expected economic returns on coal power investments at the country level (as opposed to financial returns at corporate level) is not addressed in the literature on stranded assets. Applications of an approach based on economic returns are largely confined to ex-ante project assessments by development finance institutions, who have routinely employed economic metrics to evaluate investments’ value to a country, for coal and other individual projects (Asian Development Bank, Citation2013; Meier, Citation2017a; World Bank, Citation2011), based on a methodology elaborated at the World Bank by Squire and Van der Tak (Citation1975).
The literature on coal asset stranding in China that does exist clearly underlines the scale of its potential financial exposure to coal power asset stranding, both in absolute terms and as a share of the global total – as much as 45% of an estimated US$927 billion global power sector asset stranding by 2050, albeit under an accelerated renewables scenario (Saygin et al., Citation2019). In other studies, coal power stranding is estimated at US$15–53 billion (CNY 100–340 billion) by 2030 under high – and low-carbon pathways, respectively (H. Zhang et al., Citation2020). Analyses of China’s coal fleet consistently find that both current and proposed power plants face stranded asset risks under most climate policy scenarios that are amplified by weak underlying profitability and market conditions (Cui et al., Citation2021, Citation2020; Gray & Sundaresan, Citation2020b; Spencer et al., Citation2017; Yuan, Guo, Zhang, Zhou, et al., Citation2019; Yuan & Zhang, Citation2017). Ongoing reforms to liberalize power markets are likely to further depress coal plants’ net revenues and impair commercial operation by exposing them to greater competition (Lin et al., Citation2019). The finding, based on financial analysis, in Mo et al. (Citation2021) is therefore unsurprising: a modest US$7.5 (50 CNY) per ton carbon dioxide price (close to China’s national ETS price at the time of writing) reduces coal plant lifetimes by 5.4 years on average, with five provinces seeing retirements 12–17 years earlier than planned.
What the literature on coal stranding in China does not address is whether continuing to build coal plants is justified on an country-level economic basis. This is more representative of the government’s perspective in its role as shareholder of state-owned companies and requires measuring projects’ contributions to economic growth (i.e. the benefits of electricity production to the national economy after accounting for economic costs), rather than just the net private benefits to the plant’s corporate owner (i.e. return on equity after interest and taxes). This article complements standard financial analysis with country-level economic analysis to assess under what conditions building a new plant today constitutes a productive – or wasteful – use of resources by the government of China, both on a standalone basis and relative to other options for meeting electricity demand.
Coal power investment and government shareholders
This country-level ‘economic’ framing is important for several reasons. In China, key actors across the coal power value chain are state-owned enterprises (SOEs) – including owners of coal mines and power plants (Global Energy Monitor & CREA, Citation2020), banks financing plant construction (Herve-Mignucci et al., Citation2015) and grid operators (Mayer et al., Citation2017). Potential financial losses from coal plant stranding represent direct liabilities for individual SOEs, and ultimately contingent liabilities for China’s government in its capacity as shareholder (depending on how the stranding of each coal asset affects the government’s overall portfolio). Additionally, the prevalence of SOEs in China’s coal sector – an estimated 94% of coal capacity in 2015 was state-owned (Herve-Mignucci et al., Citation2015) – means that the financial arrangements determining stranding risk for a given coal power asset (i.e. the proportion of the original investment that is not recovered under a given scenario) are negotiated between companies with a common government shareholder, making the corresponding contracts essentially transfers between subsidiaries of the state, and raising the possibility that government ownership distorts the pricing and value of these contracts. In China, therefore, financial-only stranded asset analysis is an incomplete, and potentially misleading, representation of the value of the asset to the majority government shareholder that the SOE serves.
In this paper, we are not, however, concerned with assessing the financial risks to SOEs or the contingent liabilities these represent for the government. Rather, we propose a framework for assessing the risk of new coal power investments being economically wasteful from a government’s perspective. Of course, as social planners, governments are in principle concerned with the economic rationale for all coal investments, whether or not they are state-owned. Indeed, in China, electric power – predominantly from coal – has been ‘one of the main driving forces’ advancing China’s social and economic development (Zhang et al., Citation2017). However, governments that are both social planners and shareholders have both financial and economic interests in projects. In these cases, complementing existing financial analyses of planned coal power investments with economic analysis is particularly relevant. Governments investing through their SOEs need to consider not only whether new coal power plants will be profitable, but whether they contribute to wider economic development or produce economic returns below the social discount rate. In the latter case, they are unable to generate sufficient benefits to recover their economic costs, thereby constituting a waste of national resources relative to alternative uses: what can be termed an ‘economically “stranded” asset’.
It is in this context of ubiquitous state presence in the coal sector and the centrality of coal to China’s economic development that this paper situates its contribution to the literature on stranded assets and coal power. Given the dual roles of China’s government as both the beneficial owner of power plants and manager of the economy, its investments in electricity generation, and the stranded asset risk associated with them, should be analyzed both in terms of their financial return to plant owners as well as their potential contribution to the country’s economic growth. Here, we explicitly represent the critically important governmental perspective missing from much existing work on China’s coal transition and stranded asset risk, and examine how it responds to changes in policy and technology. This framing also applies, with country-specific qualifications, to other economies planning coal power expansion in which coal SOEs feature heavily, notably India, Indonesia, Pakistan, South Africa, and Vietnam.
Economic analysis of coal capacity additions
Coal plants generate economic costs and benefits for the wider economy, notably from (i) generation and consumption of electricity generated by businesses, households and other users, and (ii) economic effects on sectors linked to plant construction and operations.Footnote2 As a consequence, the scope and valuation of costs and benefits from economic and financial standpoints differs substantially (see Methods section). Comparing the financial and economic benefits from new coal projects under different climate policy scenarios for coal can help identify economic – as distinct from financial – rationales and risks for coal power investment in China, and determine where corporate and government interests may diverge.
In this paper, we consider how a new coal power investment in China looks when assessing the costs and benefits to the national economy from the perspective of a government shareholder concerned with the investment’s contribution to national economic growth. This approach looks beyond plant-level financial returns to understand the rationale for new coal power investments from the government’s point of view. It improves our understanding of whether and how new coal plants might be perceived by the Chinese government to generate country-level economic returns, and the conditions under which new plants risk becoming ‘stranded’ on an economic basis, representing a waste of public sector investments better allocated to other power sector investment options. We do this under several plausible climate policy scenarios. We then compare the results with those for a power generation investment in a hypothetical ‘renewable with storage’ alternative and discuss the implications for coal power investment policy in China.
Approaching the coal investment question from this perspective is not intended to promote coal as a sensible investment option, but rather to recognize the relevance of economic returns to power investments in China and rebase the ‘stranded asset’ conversation around a more comprehensive representation of governments’ incentives.
Methods
Framework for economic analysis
We explore the rationale for coal power investments from two analytical foci. First, the standard ‘financial’ approach, measuring the net present value to equity holders (FNPV) of nominal free cash flows produced by the coal project over its lifetime (not including benefits to other businesses owned by the same company, e.g. in coal mining and transport), using a private discount rate based on the company’s weighted average cost of capital (1). Second, the country-level economic returns to China, i.e. the real-terms net present value of net economic benefits (ENPV) within China’s borders, using a social discount rate (2). See Supplementary Material (SM) for further detail.
(1)
(1)
(2)
(2)
The economic and financial analyses share common physical and operational attributes, but the valuation and scope of costs and benefits considered differ substantially. One significant, but often overlooked, distinction between the two approaches is that the financial analysis is based on nominal contracted costs and revenues, whereas economic analysis assesses real-terms costs and benefits to the economy (Belli et al., Citation1998; Meier, Citation2017a, Citation2017b). In the financial analysis, for instance, construction costs reflect the face value of the engineering, procurement and construction (EPC) contract. In the economic analysis, these are adjusted to exclude domestic import duties and taxes on capital and labour (which are effectively transfers between different economic actors within China), reflecting the cost to the economy of fulfilling the contract. Similar adjustments apply to operating costs and financing costs. Environmental costs are included in the financial analysis only to the extent they are priced into the market; in the economic analysis, they are valued on the basis of the government’s estimate of the national economic costs of local and global pollution, both at the plant and upstream/downstream. Interest payments between Chinese entities are not included in economic analysis as these constitute domestic transfers. In the financial analysis, the benefits of generation correspond to revenues from the sale of electricity by the power plant operator. In economic analysis, the benefits of generation are assessed further down the value chain, at the point of actual use by consumers, based on their willingness-to-pay (WTP) for the electricity after deducting the cost of transmission and distribution (‘T&D’) incurred in delivering the power.
Comprehensive economic analysis should account for a broad array of economic and environmental effects linked to the plant’s construction and operation both upstream and downstream.
Local pollution costs have come under increased scrutiny in China in recent years.Footnote3 In addition to environmental impacts from the combustion of coal (see ‘Key model parameters’ below), there are additional costs ‘beyond the plant’, such as the environmental and health impacts of coal mining, processing, and transportation upstream. Several scholars have suggested that the combined environmental costs of coal power generation (both within and beyond coal plants), are sufficiently large to outweigh all other economic benefits, even without considering available alternatives (Fei, Citation2014; Selim et al., Citation2020; Xiang & Kuang, Citation2020). These broader effects are therefore clearly important, but also highly context-specific, especially when applied to a specific plant (depending on the coal mines supplying it, population density in surrounding area, etc.). Consequently, while we do not include environmental damages beyond the plant in this simplified analysis, findings from other literature placing a high cost on these damages are important to bear in mind when interpreting our results.
Additional employment, income and investment stimulated by the economic activity associated with plant construction and coal production, also generates economic benefits. Conversely, where a coal plant becomes ‘economically stranded’ as part of a sharp policy – and/or technology-induced transition away from coal, the wider social consequences of future job and tax revenue losses, and any relocation, retraining and opportunity costs associated with finding alternative employment, are also relevant to government, as are the implications for equity and fairness among those negatively affected by the transition. While not measured in this exercise, they may be of particular short – and medium-term salience for China’s government amid the economic fallout from the COVID-19 pandemic (Benoit & Tu, Citation2020; Tu, Citation2020).
Decision-making criteria
To be justified financially/economically, a project (in this case, a coal plant) should deliver a financial/economic rate of return (FIRR/EIRR) exceeding the private/social discount rate, respectively, reflecting the investor/government’s assessment of the relative benefits of future versus present financial value/ economic growth (Meier, Citation2017b, p. 63). The second criterion for justification is whether alternative investments would generate higher financial/economic returns than the proposed coal plant project.
Financial and economic approaches estimate the coal project’s value with respect to each corresponding stakeholder: the corporate owner and the Chinese government. In the example modelled here and as discussed above, the central government is both the indirect owner and the referent party to the economic analysis. Subnational levels of government may also be involved in the coal project, financially or through ownership of provincially owned SOEs. In these cases, economic analysis should be conducted from the perspective of both national and provincial governments, with the cost–benefit analysis undertaken based on the appropriate subnational boundaries in the latter case. Future work comparing economic returns at these different levels of government would be a useful expansion of this paper, given the well-documented and historically rooted differences and tensions between central and provincial government interests (Chung, Citation1995; Li, Citation1998; Zeng, Citation2016), particularly with respect to environmental policy (Li et al., Citation2019). Footnote4
Key model parameters
In our ‘Base Case’, with plant parameters and costs reflecting the Chinese context, we model a one gigawatt-rated (GW) ultra-supercritical coal plant with a 48% starting load factor, consistent with average national coal utilization rates and well below the plant’s technical maximum.Footnote5 Debt is provided by Chinese banks, meaning that interest rates and debt-equity ratios – which do affect the financial return on equity – have no impact on country-level economic results. The financial discount rate is 6%, reflecting weighted average cost of capital to the corporate owner. The social discount rate is 8%, consistent with reported practice in China for state-led infrastructure projects (World Bank, Citation2019, p. 18,50). lists other key financial and economic parameters (see SM Table A3, for expanded detail and sources).
Table 1. Key data points for pro forma coal plant model (see SM Table A3 for expanded table and sources).
Under the national carbon emissions trading system (ETS) launched in July 2021, China allows free allocation of permits for plants operating within emissions thresholds designed to penalize older, less efficient plants rather than high-efficiency, ultra-supercritical capacity additions. The ‘Base Case’ scenario, therefore, does not include a carbon price, either financial (in the form of a price or quantity-based market instrumentFootnote6) or economic (such as a shadow price used for government decision-making). The Base Case includes local pollution costs based on Environmental Pollution Tax (EPT) charges for sulfur dioxide (SO2), nitrogen oxides (NOx), and particulate matter (PM2.5) emissions from coal plants.Footnote7 The financial analysis uses the median tax across all provinces. The administratively-set upper limit (applied only by Beijing, Tianjin, and Hebei) for pollution taxes is used for the economic analysis, as a conservative proxy for the lower bound on the true social cost of local pollution.Footnote8 Significantly higher figures are found in the literature (Biao et al., Citation2020; Fei, Citation2014), and presents the results of using a higher social cost closer to the estimates in the broader literature. WTP for electricity is, by definition, at least as high as the electricity retail price that consumers actually pay. We assume that on average, electricity consumers will generate an economic return on electricity consumption equal to China’s annual GDP growth rate and are willing to pay a corresponding premium of 6% over retail prices.
Table 2. Selected sensitivities.
Alternatives to coal power
Available alternatives to additional coal power investments include dispatchable non-coal generation technologies, transmission infrastructure investments, increased interprovincial electricity trading, demand-side management and efficiency measures.
We assume a non-fungible need for additional generation capacity, partly reflecting a bias towards capacity additions over greater inter-provincial electricity trading as an economic stimulus strategy (Hove, Citation2020). The illustrative alternative modelled here is a utility-scale solar photovoltaic (PV) plant with sufficient storage to provide dispatchable power comparable to the coal plant.Footnote9 Illustrative technical parameters for the solar/storage model are listed in SM, Table A1. Most other parameters (e.g. financing arrangements) are the same as those for coal.
Like coal, solar/storage investments generate external economic impacts beyond the plant itself. Liu et al. (Citation2019) find that renewable energy development in China delivers greater external economic benefits than coal even without accounting for environmental costs, while Garrett-Peltier (Citation2017) finds that renewable energy capital investment consistently generates more jobs than coal, and contingent valuation studies (Guo et al., Citation2014; Xie & Zhao, Citation2018; L. Zhang & Wu, Citation2012) find mixed evidence of greater WTP for renewable electricity in China. The negative environmental impacts upstream of the solar/storage alternative are likely much smaller (albeit non-zero) than those associated with coal plants. To maintain comparability, we have once again, as for the coal analysis, not quantified these effects, but the net benefits of beyond-plant effects for solar are likely to be significantly greater than for coal.
Policy scenarios
Increasingly stringent CO2 reduction policies in China are inevitable if 2060 targets are to be achieved. These policies could take various forms, including progressive tightening of emissions trading markets, direct carbon taxation, obligatory shadow carbon pricing for new investments, preferential tariffs for renewables, absolute coal power generation caps, or moratoriums on the operation of coal plants failing to meet certain criteria. Electricity dispatch reforms may also expose coal power to greater competition from renewables, leading to fewer operating hours. Direct regulatory measures to close inefficient plants, or compel them to meet emissions standards, have been progressively deployed since 2007 (Tang et al., Citation2019; Yuan, Guo, Zhang, Chen, et al., Citation2019).
describes two sets of possible policy scenarios affecting the economic analysis of coal: carbon pricing (implemented explicitly through a trading system or taxation, or implicitly through regulatory measures) and electricity production caps (regulated or informal). These scenarios have been structured along China’s Five-Year Plan (FYP) schedule, such that changes are implemented in each FYP’s first year. The scenarios do not attempt to estimate the full economic cost of carbon emissions (the ‘social cost of carbon’) from coal power generation, but rather reflect a more conservative position for the Chinese government in setting a cost for emissions from coal generation.
Table 3. Summary of climate policy scenarios.
In the carbon pricing scenarios, a shadow carbon price (SCP) is implemented by the government. The Modest Ambition SCP scenario (MA-SCP) starts at US15$/tCO2 in 2026 (the 15th FYP’s first year), increasing by US$5 every five years to reach US$30/tCO2 in 2041. In the Higher Ambition SCP scenario (HA-SCP), the value increases by US$15 every five years to reach US$60/tCO2 in 2041. These prices are well below the range currently applied by the World Bank (Citation2017) for project evaluation, which is explored below.Footnote10
In the production cap scenarios, load factor restrictions (LR) are imposed on the plant, consistent with projected changes in the share of coal in China’s total primary energy supplyFootnote11 under the ‘Stated Policies’ (SP-LR) (falling from 43% in 2026 to 18% by 2041) and ‘Below 2C’ (B2C-LR) scenarios (from 41% to 13%), respectively, set out by the Energy Research Institute and China National Renewable Energy Centre (Citation2019, p. 207). We also model the effect of moratoriums on the coal plant coming into effect in 2030 and 2035.
Results
The EIRR in the Base Case with no carbon pricing or production limits is 19.1%, with an ENPV of US$472 m. By comparison, the FIRR (return on equity) is just 8.4%. This divergence in results is driven largely by the difference between financial and economic values for capital and fuel costs, and the different valuation points for benefits (i.e. privately contracted electricity price versus WTP of ultimate consumers). This is unsurprising: governments make investments through SOEs precisely because they generate economic benefits in addition to any financial return the plant owner – often also the government – receives. Investors require 21 years to see a positive financial return (leaving their capital highly vulnerable to climate policies implemented before 2040), but the project requires just seven years to break even economically, meaning the project could deliver net economic value even if it was forced to close before 2030, under a Base Case scenario that does not incorporate any shadow carbon pricing. This difference in the rate of recovery of the initial investment offers an explanation for continued coal plant construction by China’s SOEs, even when the long-term financial case appears tenuous.
The plant’s economic value is sensitive to changes in WTP for electricity, the plant’s utilization rate, and the social costs of local pollution. Its financial performance is, by comparison, relatively sensitive to wholesale electricity tariffs, higher coal prices, and lower load factors. summarizes the effects of these and other sensitivities on returns and net present value.
Carbon pricing scenarios
Shadow carbon pricing reduces economic returns by adding a cost to each kWh of electricity generated. Under the (lower) MA-SCP scenario, the plant’s EIRR drops to 5.1% and the plant creates an economic loss of US$50 m, never recovering its initial investment in economic terms (). This suggests that if China’s government expects to introduce the equivalent of even a modest SCP in subsequent FYPs, an apparently appealing coal plant investment becomes economically undesirable and should not, in principle, proceed to commissioning. The (higher) HA-SCP scenario magnifies the economic loss to US$355 m (see SM for full details). Using the much higher shadow price ranges applied by the World Bank dramatically aggravates the economic costs induced by the plant, to between US$1 billion and US$2.7 billion.
The plant begins to make an economic operating loss (accounting for fixed and variable costs) at an SCP of US$28/tCO2 or more, a level reached in 2041 in the MA-SCP scenario, and 2031 in the HA-SCP scenario (see ). Assuming the plant is already operating, it would be economically desirable to permanently cease operation at these inflection points in each respective scenario to prevent further economic losses in each scenario, cutting the plant’s intended lifetime short by 22 and 12 years, respectively.
Shadow carbon pricing only affects plant-level financial returns if it is passed through to the generator as an actual charge (under an ETS, as a carbon tax, through a technology standard, or other measures). The carbon price required to result in a financial operating loss is lower, at US$18/tCO2 (2020 US$); China’s average ETS price in July 2021 was just under $8/tCO2. Thus, in the modelled case, even if the government’s SCP exceeds US$28/tCO2, unless it is translated into a financial charge exceeding US$18/tCO2, there remains a financial incentive to operate the plant even as it destroys economic value, placing the government at odds with SOE-level financial incentives.
Production cap scenarios
Constraining production levels also affects country-level economic returns, but by less than an SCP (see ). The decline in plant utilization rate under the SP-LR scenario reduces ENPV from US$472 m to US$229 m, and the B2C-LR scenario results in US$161 m. Even a moratorium on generation in 2035, consistent with coal phase-out policies in several EU states,Footnote12 still produces a positive economic return of $179 m. Absent any shadow carbon pricing, the plant only fails to generate a positive economic return when forced to close in 2030 or earlier. Since each marginal kWh still delivers the same economic benefit under LR scenarios, being unaffected by a shadow carbon cost, the threat of load factor restrictions may not be an effective tools for dissuading government investment in new coal power generation relative to carbon pricing.
Production caps have a much more severe effect on financial returns, generating losses (i.e. stranded asset values) of US$124 m and US$176 m in SP-LR and B2C-LR scenarios, respectively. The difference between economic and financial returns is so large because the marginal economic benefit of each kWh produced (the difference between WTP and economic cost of producing and delivering electricity) in the absence of shadow carbon pricing significantly exceeds the marginal financial benefit (the difference between electricity tariff revenue and financial cost, including interest) to the plant owners. This is why the Base Case economic breakeven point required to justify the investment is reached after eight years of operation, compared to 20 for financial breakeven.
By reducing the volume of electricity sales, which does not affect the marginal benefit of production, the LR scenarios have a greater impact on financial than economic results and severely limit the project’s ability to recover its initial financial investment. The same dynamics apply for an absolute moratorium on production. A moratorium starting in 2030 would generate a financial loss of US$113 m, and a moratorium starting in 2035, a US$72 m loss.
The renewable alternative
The solar/storage alternative yields an 8.7% EIRR, and an ENPV of US$54 m, both substantially lower than coal in the Base Case. However, while the SCP and LR scenarios depress economic returns for coal generation, they have no effect on the renewable alternative, improving its relative appeal. Even low levels of shadow carbon pricing (i.e. the MA-SCP scenario) can make solar/storage economically preferable to coal. The renewable alternative delivers an ENPV that is US$105 m larger than coal under the MA-SCP scenario (an advantage that increases to US$409 m under the HA-SCP scenario). In contrast, coal remains more economically attractive than the solar/storage alternative under the two LR scenarios.Footnote13
While the renewable alternative does generate a positive ENPV, it requires 25 years to break even economically due to the high initial cost of storage infrastructure. However, a 15% decline in the capital costs of storage, consistent with the NREL mid-case battery storage cost projection from 2020 to 2023 (Cole & Frazier, Citation2019), raises the EIRR to 10.9% and the ENPV to US$328 m (US$378 m greater than coal in the MA-SCP case), bringing breakeven forward to 14 years. A 30% decline (anticipated by NREL in 2027) produces a 13.8% EIRR and US$602 m ENPV. This is US$130 m greater than that of coal in the (no-climate-policy) Base Case. It achieves breakeven within ten years. These suggest dispatchable solar is likely to be competitive with coal as an economic investment before the late 2020s on current cost trends.
As noted above, the true relative benefits of the renewable alternative are likely understated in these results since the negative upstream environmental and health impacts associated with coal supply are not factored in. If these factors were included, assuming the economic stimulus benefits of either option are similar, the renewable alternative would likely be more competitive, and sooner, on economic terms.
Notwithstanding the growing competitiveness of unsubsidized solar generation both in China (Energy Monitor Worldwide, Citation2020; Yang et al., Citation2020) and elsewhere (Naschert, Citation2020), the dispatchable solar/storage alternative still faces financial challenges. The financial return is below 1%, weighed down by the cost of storage. Achieving a return on equity exceeding that for the coal Base Case would require raising the tariff received by solar/storage from its US$47/MWh baseline to US$62/MWh.Footnote14
Discussion
Climate policy tightening in China (through carbon pricing or otherwise) is increasingly likely well within the operating lifetimes of plants built today. Other scholars have shown that this, along with poor market conditions for coal power, will result in significant volumes of financially stranded coal assets. However, as continued coal plant construction in China suggests, weak or negative profitability alone may not be sufficient to dissuade new coal power investment, particularly where the government is the ultimate plant owner, more likely to be concerned with coal’s contribution to economic growth as well as its financial viability. Distinguishing between financial and economic perspectives can help uncover differences between the rationales for coal plant construction at corporate and governmental level and identify the policy or technological changes required to counter the potential economic case for coal.
Economic analysis of the representative new coal plant modelled here suggests that modest shadow carbon pricing – well below the social cost of carbon recently proposed by the administration of U.S. President Biden (Chemnick, Citation2021) or the range required to limit global warning to under 2C derived by Stiglitz et al. (Citation2017) – negates any expected positive economic returns. Compared to a US$472 m present value in the Base Case, the MA-SCP scenario generates an economic loss of US$50 m in present terms, while the HA-SCP scenario generates a loss of US$355 m.
If China’s government recognizes the negative economic impact of carbon emissions through a (implied or real) shadow carbon price, but does not fully translate it into a financial expense for plant owners, there is a potential misalignment of incentives for China’s government in its dual roles as shareholder and social planner. The coal plant ceases generating marginal economic benefits for SCPs in excess of US$28/tCO2, but only makes operating financial losses if the SCP manifests as a cost of US$18/tCO2 or more. Unless the Chinese government, as part of its carbon neutrality goal, establishes credible expectations for an ETS or carbon tax exceeding this value, it may find itself at odds with its own SOEs, where the financial incentive to continue building and operating coal plants remains.
Production caps have a more limited impact on economic returns, since they do not affect the marginal economic benefits of production. This means that even under the B2C-LR Scenario, the plant breaks even economically by mid-2031 and requires a permanent moratorium in 2030 or earlier to be prevented from producing a positive economic return (with no shadow carbon pricing). However, the production cap scenarios impair financial returns much more significantly and prevent the plant from recovering its initial investment at all, potentially discouraging company executives from making the investment in the first place. Where meaningful carbon taxes are not a short-term policy option, therefore, production caps may be effective in dissuading company executives from building new coal plants.
Overall, this analysis shows that the degree to which the pro forma coal plant becomes ‘stranded’ from an economic perspective depends both on the stringency and type of policy adopted. Our modelling suggests that a shadow price is a more effective tool than production caps to disincentivize government support for a new coal investment because of its stronger negative impact on economic returns, but is only effective at influencing financial decision-making to the extent it imposes costs on the company (in the form of a carbon tax, ETS, or equivalent instrument).
Comparison with an illustrative solar/storage alternative further weakens the economic case for coal under climate policy tightening scenarios. The solar/storage plant produces greater economic returns than the coal plant in both SCP scenarios (by US$105 m and US$409 m in MA-SCP and HA-SCP, respectively). A 15% decline in storage capital costs (expected by 2023, when currently planned coal capacity will remain under construction) widens its economic advantage to US$378 m (MA-SCP) and US$683 m (HA-SCP) over coal, while also outperforming coal in each LR and moratorium scenario.
With 30% lower storage costs, expected before the late 2020s, the solar/storage alternative delivers an ENPV of US$602 m, over US$100 m greater than the coal Base Case, with no shadow carbon pricing or load factor restrictions in place. This suggests that in circumstances where solar/storage is an option, there may be no economic case for new coal from the late 2020s irrespective of climate policy tightening. The combination of some shadow pricing (or, to a lesser extent, some production constraints on coal) and cost declines for solar/storage technologies suggests that investing in coal today is likely to prove a poor use of scarce economic resources.
Expected financial returns from solar/storage remain lower than those for coal in the short – and medium-term due to the capital intensity of storage and correspondingly high financing costs. Aligning government incentives with investors (even where state-owned) will require measures to address this, potentially including direct subsidies for storage, preferential lending criteria to reduce debt costs, and higher electricity tariffs. The latter has precedent in Chinese electricity markets, in which the regulatory framework already differentiates tariffs by generation source (Lee, Citation2019). As the cost of storage falls and climate policies tighten, the potential net economic benefits of solar/storage investment over coal could justify the expansion of subsidies to enhance financial returns for solar/storage and encourage investment by SOEs and private firms alike.
Conclusions
This paper has applied a framework for joint financial and economic analysis of prospective government investments. It represents a more complete approximation of a governmental perspective on coal power investment through state-owned enterprises than the financial-only analyses predominant in the existing literature. The results suggest that at least some of the new coal plants planned in China may never generate positive economic returns, especially if the government elects to implement even relatively modest market-based or control policies in pursuit of carbon neutrality and other environmental and societal goals.
Modelling of a pro forma coal plant representative of coal capacity additions in China suggests that modest shadow carbon pricing, rising linearly from US$15 to 30/tCO2 from 2026 through 2041, is sufficient to prevent the project from ever generating a positive economic return. A more ambitious shadow carbon pricing trajectory, increasing to US$60/tCO2 in 2041, causes the plant to generate an economic loss of US$355 m. Production caps reduce economic returns to a lesser extent but cause the plant to generate negative financial returns, perhaps introducing a stronger deterrent to new plant construction for company-level executives than for the government as shareholder. Under any of the scenarios presented, investing in a coal plant today on an economic basis risks wasting productive resources better used elsewhere, especially given the decreasing costs of low-carbon alternatives immune to the effects of policies targeting carbon emissions, here represented by a solar/storage dispatchable generation plant. China’s government and, by extension, its SOEs as instruments of economic policy, should therefore carefully consider the economic viability of new, long-lived coal investments today in light of future climate policies and trends in competing renewable technologies.
This analysis also points to several possible directions for future research. First, plant-level economic performances under a more detailed range of climate policy scenarios. Second, a broader and deeper assessment of the relative economic costs and benefits of coal power (e.g. loss of employment, upstream environmental impact, investment multiplier effects), and of alternative power sector investment options for China. Third, application of this framework to other generation technologies (including natural gas and the deployment of carbon capture and storage) and alternative means of meeting demand without additional generation (such as energy efficiency, demand response and additional transmission capacity). Fourth, the use of this framework to assess the economic returns for all actual planned coal plants in China, scaling up from the single pro-forma plant analyzed here, to determine the aggregate potential for economically stranded coal assets if current coal expansion plans go ahead.
Moreover, while this analysis is tailored to China given its dominant contribution to coal capacity additions, over-investing in coal assets is an issue of global concern, particularly to countries with relatively new fleets and significant expansion plans. Understanding the convergences and divergences between financial and economic returns is particularly pertinent where there is a major state presence in the power sector, such as India, Indonesia, Mexico, Pakistan, South Africa and Vietnam. Despite coal project cancellations in Bangladesh, the Philippines, Vietnam, Indonesia, and Pakistan in 2020, an estimated 503 GW of capacity remains under development globally, 344 GW outside China (Global Energy Monitor et al., Citation2021). The application of an economic framework can complement existing (financial) stranded asset analysis by assessing the economic rationale for proposed coal power projects, thereby providing governments and their SOEs with insights into prospective economic returns at the country level, as distinct from the corporate financial risks.
Finally, both within China and other countries featuring semi-autonomous subnational governments investing in coal, the principles behind this analysis can be applied to evaluate economic and financial returns at different levels of government (e.g. for provincial jurisdictions such as Inner Mongolia, Shaanxi, Shanxi, and Xinjiang), whereby sub-national boundaries are used as the pertinent unit to which economic costs and benefits accrue. This can provide insight into the political economy of provincial governments’ coal investments, particularly where the economic case for coal is significantly stronger in specific provinces than at the national level, and inform the national government of misaligned perspectives on coal investment with subnational authorities.
Supplemental Material
Download MS Word (38.3 KB)Acknowledgements
For their insights and helpful comments on earlier drafts, the authors wish to thank Peter Meier, Arnaud Hubert, David Sandalow, Thomas Hale, and several anonymous reviewers. The views expressed here are those of the authors alone.
Notes
1 An additional 38.4 GW was commissioned and 31.5 GW resumed construction. Major coal-producing provinces Inner Mongolia and Shaanxi accounted for over half of newly permitted capacity.
2 Economic returns are of interest to governments whether or not they are shareholders in the company implementing the project, but tools available to government for influencing investment and operational decisions of state-owned enterprises are greater and more diverse than those it can typically apply to private sector companies (Benoit, Citation2019).
3 Mitigating air, water and ground pollution is a major policy priority in China, reflected in the rapid installation of pollution control measures across the coal fleet, closure or relocation of poorly performing plants and tax reforms (see Chang et al. (Citation2016) and Mining Technology (Citation2017)).
4 In practice, their interests can, and often do, diverge, leading to a more complex decision-making dynamic.
5 China operates an ‘equal shares’ system such that coal power is contractually dispatched for a fixed number of hours per year. In combination with limited inter-provincial electricity trading, this means the average load factor of China’s coal plants is just 48% (Gray & Sundaresan, Citation2020b), far below their technical capacity. Well-planned transmission expansions and dispatch liberalization could pre-empt any financial or economic need for new generation capacity. This would not necessarily be consistent with power sector decarbonization trajectories, particularly if the plants in which load factors increase are less efficient than the ultra-supercritical example here.
6 Pilot carbon emissions trading systems (ETSs) have been operational in several provinces in China and a national scheme was launched in February 2021, but prices are low and volatile. It is not yet clear how caps and permit allocation will work, and the extent to which emitters will need to trade in permits.
7 Local air pollution taxes range from 1.2-12 CNY/kg for regulated pollutants, depending on the province. The Base Case assumes 1.2 CNY/kg, the level set by most of the less population-dense provinces building new coal capacity.
8 Estimates of social costs associated with coal production and combustion suggest that local pollution taxes only partially internalize these pollution costs. See Fei (Citation2014) and Selim et al. (Citation2020).
9 Dispatchable renewable electricity costs are highly sensitive to the choice of generation and storage technologies. Here we set out an illustrative solar/storage alternative, but there may be configurations that ultimately are more cost-effective, especially when considering China’s system-decarbonisation needs (G. He et al., Citation2020).
10 The High-Level Commission on Carbon Prices concluded that a range of US$40-80 per ton of CO2e in 2020, rising to US$50-100 by 2030, is consistent with achieving the 2C Paris target, provided supportive policies are in place. The World Bank recommends these ranges for project economic analysis, with prices extrapolated from 2030 to 2050 at the same growth rate (2.25% per year).
11 These are probably conservative estimates of coal power’s declining share in electricity generation because electricity is likely to be decarbonized more rapidly than others in which coal also features heavily, notably heavy industry and space heating.
12 This is comparable with coal phase-out plans in numerous countries (e.g., Germany’s intended phase-out by 2038, U.S. President Biden’s campaign pledge to make power generation carbon-free by 2035) and is less constraining that the United Kingdom’s announced ban on unabated coal power by 2025.
13 Different generation options are often compared using a “levelized cost of electricity” (LCOE), which is calculated using financial costs. Because, in the Chinese context, these costs result in part from regulated and other government-influenced pricing (e.g., regulated coal prices, or EPC contract negotiated between state-owned power and construction companies), it might be more revealing and useful to calculate levelized cost using the methodology and values for the country-level economic analysis presented here, including shadow carbon pricing and other pertinent externalities – namely, the “levelized economic cost of electricity” (LECOE). The LECOE may better assist the government in identifying and advancing new generation choices with lower economic costs and thereby support the government’s goals of keeping electricity tariffs low.
14 The average tariff in China for solar PV (including rooftop) in 2018 was US$124/MWh. The average for gas generation was US$84.5/MWh (Lee, Citation2019). The $62/MWh required for solar/storage equity returns to exceed coal is roughly 20% higher than average benchmark feed-in tariffs for coal at approximately US$52/MWh (Guoneng Hutong Inner Mongolia Network Technology Co Ltd, Citation2019).
References
- Asian Development Bank. (2013). Qinghai delignha concentrated solar thermal power project - economic analysis.
- Belli, P., Anderson, J., Barnum, H., Dixon, J., & Tan, J.-P. (1998). Handbook on economic analysis of investment operations. World Bank.
- Benoit, P. (2019). Engaging state-owned enterprises in climate action. https://energypolicy.columbia.edu/research/report/engaging-state-owned-enterprises-climate-action
- Benoit, P., & Tu, K. J. (2020). Is China still a developing country, and why it matters for energy and climate. C. University.
- Biao, H., Hongjia, D., Ping, J., & Jingan, Z. (2020). Analysis of the applicable rate of environmental tax through different tax rate scenarios in China. Sustainability (Basel, Switzerland), 12(4233), 4233. https://doi.org/10.3390/su12104233
- Caldecott, B., Dericks, G., Tulloch, D. J., Liao, X., Kruitwagen, L., Bouveret, G., & Mitchell, J. (2017). Stranded assets and thermal coal in China: An analysis of environment-related risk exposure: University of Oxford, Smith School of the Environment, Sustainable Finance … .
- Caldecott, B., Howarth, N., & McSharry, P. (2013). Stranded assets in agriculture: Protecting value from environment-related risks. https://www.smithschool.ox.ac.uk/publications/reports/stranded-assets-agriculture-report-final.pdf
- Caldecott, B., McCarten, M., & Triantafyllidis, C. (2018). Carbon lock-in curves and Southeast Asia: Implications for the Paris agreement. https://www.smithschool.ox.ac.uk/research/sustainable-finance/publications/Carbon-Lock-in-Curves-and-Southeast-Asia.pdf
- Chang, S., Zhuo, J., Meng, S., Qin, S., & Yao, Q. (2016). Clean coal technologies in China: Current status and future perspectives. Engineering (Beijing, China), 2(4), 447–459. https://doi.org/10.1016/J.ENG.2016.04.015
- Chemnick, J. (2021). Cost of carbon pollution pegged at $51 a Ton. Scientific American.
- China Electric Power Statistics and Data Center. (2020). Overview of the operation of the power industry from January to October 2020. https://www.cec.org.cn/detail/index.html?3-290358
- Chung, J. H. (1995). Studies of central–provincial relations in the People's Republic of China: A mid-term appraisal. The China Quarterly, 142, 487–508. https://doi.org/10.1017/S0305741000035025
- Cole, W., & Frazier, W. (2019). Cost projections for utility-scale battery storage. Golden, CO https://www.nrel.gov/docs/fy19osti/73222.pdf
- Cui, R., Hultman, N., Cui, D., McJeon, H., Yu, S., Edwards, M., Sen, A., Song, K., Bowman, C., Clarke, L., Kang, J., Lou, J., Yang, F., Yuan, J., Zhang, W., & Zhu, M. (2021). A plant-by-plant strategy for high-ambition coal power phaseout in China. Nature Communications, 12(1), 1–10. https://doi.org/10.1038/s41467-021-21786-0
- Cui, R., Hultman, N., Jiang, K., McJeon, H., Yu, S., Cui, D., … Zhang, W. (2020). A high ambition coal phaseout in China: Feasible strategies through a comprehensive plant-by-plant assessment. Center for Global Sustainability.
- Energy Monitor Worldwide. (2020). Chinese fishery hosting 260 MW of unsubsidized solar. Energy Monitor Worldwide.
- Energy Research Institute, & China National Renewable Energy Centre. (2019). China renewable energy outlook 2019.
- Fei, T. (2014). The true cost of coal. http://coalcap.nrdc.cn/pdfviewer/web/?15083101061229121311.pdf
- Garrett-Peltier, H. (2017). Green versus brown: Comparing the employment impacts of energy efficiency, renewable energy, and fossil fuels using an input-output model. Economic Modelling, 61, 439–447. https://doi.org/10.1016/j.econmod.2016.11.012
- Global Energy Monitor, & CREA. (2020). A new coal boom in China: Country accelerates new coal plant permitting and proposals. Briefing Series.
- Global Energy Monitor, Sierra Club, CREA, Climate Risk Horizons, GreenID, & Ekosfer. (2021). Boom and bust 2021: Tracking the global coal plant pipeline. Boom and Bust. https://globalenergymonitor.org/wp-content/uploads/2021/04/BoomAndBust_2021_final.pdf
- Gray, M., & Sundaresan, S. (2020a). How to waste over half a trillion dollars: The economic implications of deflationary renewable energy for coal power investments. C. T. Initiative.
- Gray, M., & Sundaresan, S. (2020b). Political decisions, economic realities: The underlying operating cashflows of coal power during COVID-19.
- Guo, X., Liu, H., Mao, X., Jin, J., Chen, D., & Cheng, S. (2014). Willingness to pay for renewable electricity: A contingent valuation study in Beijing, China. Energy Policy, 68, 340–347. https://doi.org/10.1016/j.enpol.2013.11.032
- Guoneng Hutong Inner Mongolia Network Technology Co Ltd. (2019). National and provincial electricity coal price index 2019. From Guoneng Hutong Inner Mongolia Network Technology Co Ltd http://www.imcec.cn/zgdm_2019
- He, G, Lin, J, Sifuentes, F, Liu, X, Abhyankar, N, & Phadke, A. (2020). Rapid cost decrease of renewables and storage accelerates the decarbonization of China’s power system. Nature Communications, 11(1), 1–9. https://doi.org/10.1038/s41467-019-13993-7
- He, J. (2020). China's long-term low-carbon development strategy and pathway. Climate Change Global Lectures.
- He, J., Li, Z., Zhang, X., Wang, H., Dong, W., Chang, S., Ou, X., Guo, S., Tian, Z., Gu, A., Teng, F., Yang, X., Chen, S., Yao, M., Yuan, Z., Zhou, L., & Zhao, X. (2020). Comprehensive report on China's long-term Low-carbon development strategies and pathways. Chinese Journal of Population, Resources and Environment, 18(4), 263–295. https://doi.org/10.1016/j.cjpre.2021.04.004
- Herve-Mignucci, M., Wang, X., Nelson, D., & Varadarajan, U. (2015). Slowing the growth of coal power in China: The Role of Finance in State-Owned Enterprises.
- Hove, A. (2020). Trends and contradictions in China's renewable energy policy.
- International Energy Agency. (2019). World energy outlook 2019. International Energy Agency.
- International Energy Agency. (2020a). Energy technology perspectives 2020. Energy technology perspectives.
- International Energy Agency. (2020b). World energy outlook 2020. International Energy Agency.
- International Energy Agency. (2021). Net zero by 2050: A roadmap for the global energy sector. International Energy Agency.
- Johnson, N., Krey, V., McCollum, D. L., Rao, S., Riahi, K., & Rogelj, J. (2015). Stranded on a low-carbon planet: Implications of climate policy for the phase-out of coal-based power plants. Technological Forecasting and Social Change, 90, 89–102. https://doi.org/10.1016/j.techfore.2014.02.028
- Lee, H. M. (2019). China's electricity price from gas drops, but still over 30% higher than coal. NEA. S&P Global Platts.
- Li, L. C. (1998). Loci and scope of state power: Central-provincial tensions in China. Asian Economic and Political Issues, 4, 117–134.
- Li, X., Yang, X., Wei, Q., & Zhang, B. (2019). Authoritarian environmentalism and environmental policy implementation in China. Resources, Conservation and Recycling, 145, 86–93. https://doi.org/10.1016/j.resconrec.2019.02.011
- Lin, J., Kahrl, F., Yuan, J., Liu, X., & Zhang, W. (2019). Challenges and strategies for electricity market transition in China. Energy Policy, 133. https://doi.org/10.1016/j.enpol.2019.110899
- Liu, J., Li, J., & Yao, X. (2019). The economic effects of the development of the renewable energy industry in China. Energies (Basel), 12(9), 1808. https://doi.org/10.3390/en12091808
- Mayer, B., Rajavuori, M., & Fang, M. M. (2017). The contribution of state-owned enterprises to climate change mitigation in China. Climate Law, 7(2-3), 97–124. https://doi.org/10.1163/18786561-00702002
- Meier, P. (2017a). Power sector investment projects: Guidelines for economic analysis. World Bank.
- Meier, P. (2017b). Power sector investment projects: Guidelines for economic analysis: Technical notes. World Bank.
- Mercure, J. F., Pollitt, H., Viñuales, J. E., Edwards, N. R., Holden, P. B., Chewpreecha, U., Salas, P., Sognnaes, I., Lam, A., & Knobloch, F. (2018). Macroeconomic impact of stranded fossil fuel assets. Nature Climate Change, 8(7), 588–593. https://doi.org/10.1038/s41558-018-0182-1
- Mining Technology. (2017). Clean break: How China’s new environmental protection tax law could affect mining. Mining Technology.
- Mo, J., Zhang, W., Tu, Q., Yuan, J., Duan, H., Fan, Y., Pan, J., & Meng, Z. (2021). The role of national carbon pricing in phasing out China's coal power. iScience, 24(6), 102655. https://doi.org/10.1016/j.isci.2021.102655
- Naschert, C. (2020). European power outlook: Falling costs to drive more unsubsidized solar activity. S&P Global.
- Normile, D. (2020). China's bold climate pledge earns praise-but is it feasible? Science (New York, N.Y.), 370(6512), 17–18. https://doi.org/10.1126/science.370.6512.17
- Pfeiffer, A., Hepburn, C., Vogt-Schilb, A., & Caldecott, B. (2018). Committed emissions from existing and planned power plants and asset stranding required to meet the Paris agreement. Environmental Research Letters, 13(5). https://doi.org/10.1088/1748-9326/aabc5f
- Saygin, D., Rigter, J., Caldecott, B., Wagner, N., & Gielen, D. (2019). Power sector asset stranding effects of climate policies. Energy Sources, Part B: Economics, Planning, and Policy, 14(4), 99–124. https://doi.org/10.1080/15567249.2019.1618421
- Selim, K., Ichisugi, Y., Abeynayaka, A., & Itsubo, N. (2020). External-cost estimation of electricity generation in G20 countries: Case study using a global life-cycle impact-assessment method. Sustainability, 12(5), 2002. https://doi.org/10.3390/su12052002
- Shearer, C., & Myllyvirta, L. (2021). China dominates 2020 coal plant development. Global Energy Monitor Briefings.
- Spencer, T., Berghmans, N., & Sartor, O. (2017). Coal transitions in China's power sector: A plant-level assessment of stranded assets and retirement pathways. S. Po.
- Squire, L., & Van der Tak, H. G. (1975). Economic analysis of projects. World Bank Publications.
- Stiglitz, J. E., Stern, N., Duan, M., Edenhofer, O., Giraud, G., Heal, G. M., … Pangestu, M. (2017). Report of the high-level commission on carbon prices.
- Tang, L., Qu, J., Mi, Z., Bo, X., Chang, X., Anadon, L. D., Wang, S., Xue, X., Li, S., Wang, X., & Zhao, X. (2019). Substantial emission reductions from Chinese power plants after the introduction of ultra-low emissions standards. Nature Energy, 4(11), 929–938. https://doi.org/10.1038/s41560-019-0468-1
- Tu, K. J. (2020). COVID-19 pandemic's impacts on China's energy sector: A preliminary analysis.
- World Bank. (2011). Project appraisal document on a proposed loan in the amount of US$330 million to the Socialist Republic of Vietnam for the Trung Son Hydropower Project. (57910-VN). World Bank.
- World Bank. (2017). Shadow price of carbon in economic analysis: Guidance note. The World Bank Group.
- World Bank. (2019). Project appraisal document: China renewable energy and battery storage promotion project. The World Bank Group.
- Xiang, H., & Kuang, Y. (2020). Who benefits from China’s coal subsidy policies? A computable partial equilibrium analysis. Resource and Energy Economics, 59. https://doi.org/10.1016/j.reseneeco.2019.101124
- Xie, B.-C., & Zhao, W. (2018). Willingness to pay for green electricity in Tianjin, China: Based on the contingent valuation method. Energy Policy, 114, 98–107. https://doi.org/10.1016/j.enpol.2017.11.067
- Yang, Y., Campana, P. E., & Yan, J. (2020). Potential of unsubsidized distributed solar PV to replace coal-fired power plants, and profits classification in Chinese cities. Renewable and Sustainable Energy Reviews, 131. https://doi.org/10.1016/j.rser.2020.109967
- Yuan, J., Guo, X., Zhang, W., Chen, S., Ai, Y., & Zhao, C. (2019). Deregulation of power generation planning and elimination of coal power subsidy in China. Utilities Policy, 57, 1–15. https://doi.org/10.1016/j.jup.2019.01.007
- Yuan, J., Guo, X., Zhang, W., Zhou, J., & Qin, C. (2019). Stranded coal power assets in China: A case study of jilin province. Emerging Markets Finance and Trade, 55(12), 2673–2688. https://doi.org/10.1080/1540496X.2018.1541134
- Yuan, J., & Zhang, W. (2017). Power generation liberalisation, coal subsidies and stranded assets.
- Zeng, Q. (2016). Control, discretion and bargaining: The politics of provincial leader rotation in China. Chinese Political Science Review, 1(4), 623–644. https://doi.org/10.1007/s41111-016-0045-8
- Zhang, C., Zhou, K., Yang, S., & Shao, Z. (2017). On electricity consumption and economic growth in China. Renewable and Sustainable Energy Reviews, 76, 353–368. https://doi.org/10.1016/j.rser.2017.03.071
- Zhang, H., Zhang, X., & Yuan, J. (2020). Transition of China's power sector consistent with Paris Agreement into 2050: Pathways and challenges. Renewable and Sustainable Energy Reviews, 132. https://doi.org/10.1016/j.rser.2020.110102
- Zhang, L., & Wu, Y. (2012). Market segmentation and willingness to pay for green electricity among urban residents in China: The case of Jiangsu province. Energy Policy, 51, 514–523. https://doi.org/10.1016/j.enpol.2012.08.053