Abstract
A rich variety of processes have modified the glacial landscape of the Scottish Highlands since the last glaciers disappeared 11,500 years ago. Many of these processes can be described as paraglacial (glacially-conditioned): retreat of glacier ice has resulted in exposure of metastable sediment sources (rock slopes, drift-mantled slopes and valley-floor glacigenic deposits) that have been reworked over a range of timescales. Much of the reworked sediment has been deposited in paraglacial sediment stores (talus accumulations, debris cones, alluvial fans, valley fills, river terraces and deltas). The trajectory of paraglacial sediment transfer can be approximated by an exhaustion model. A corollary of this model is that all sediment stores eventually undergo a change from net accumulation to net erosion, reflecting the non-renewable nature of sediment sources. This concept helps to explain why most talus accumulations, debris cones and alluvial fans in the Highlands are now relict, and why many floodplains appear to have experienced an initial period of aggradation followed by later river incision and terrace development. Since deglaciation, rock slopes have experienced paraglacial stress release due to differential deglacial unloading. Many rock slopes have remained stable or experienced gradual adjustment through intermittent rockfall. At over 600 sites, however, large-scale rock-slope failure has occurred, either in the form of catastrophic failure with complete or arrested debris runout, or as major slope deformations. Modification of drift-mantled slopes has been dominated by rainstorm-generated debris flows activity, which have stripped sediment from slopes and redeposited it in debris cones. Radiocarbon dating of buried organic horizons shows that debris flows have occurred intermittently throughout the Holocene. Snow avalanches have played only a localized role in paraglacial sediment transfer. The Holocene alluvial history of the Highlands is poorly understood. Some alluvial fans formed during a few exceptional flood events, and there is evidence that postglacial floodplain aggradation peaked in the late Holocene and was succeeded by floodplain incision and terrace development, possibly reflecting upstream reduction in paraglacial sediment supply. Other processes have operated independently of glacial conditioning. The effects of wind action are dominant in the coastal zone, forming dunefields through aeolian reworking of beach sand, and on plateaux in the form of deflation surfaces and sand sheets. Extensive erosion of plateau-top sands and aeolisols occurred in the period AD 1550–1700, a period of exceptional storminess. Small-scale periglacial processes have also been active on high ground throughout the Holocene, including granular weathering of exposed rock surfaces, the development of small-scale frost-sorted patterned ground on unvegetated terrain, and slow downslope movement of solifluction sheets, solifluction lobes and ploughing boulders.
Introduction
Much of the present form of the Scottish Highlands reflects successive episodes of vigorous glacial erosion superimposed on a landscape of varied lithology that experienced non-uniform uplift during the Neogene and earlier. Glaciers and ice sheets have sculpted the Highlands into a landscape of cliffs, corries, arêtes, troughs and fjords, and their final retreat left the floors of many valleys buried under a cover of till and glacifluvial deposits. However, since the retreat of the last glaciers the mountain landscapes of Scotland have been slowly and subtly adjusting to non-glacial conditions under climates fairly similar to that of the present.
This paper reviews the ways in which the landscapes of the Scottish Highlands have been modified by non-glacial processes during the Holocene Epoch, which extends from the end of the last glacial period about 11 500 years ago to the present, and includes the entire period of known human occupancy in Scotland. Dates are given in calendar years (yr) or millennia (kyr) before present; thus 11.5 kyr = 11 500 years before present. Where appropriate, radiocarbon ages have been converted to calendar years using the Calib 4.4 programme (Stuiver et al., Citation1998). Dating uncertainties are expressed as ± 1σ (standard deviations from the mean) throughout. However, events within the last few centuries are dated in years AD.
The Holocene was initiated when re-establishment of thermohaline circulation in the NE Atlantic Ocean resulted in northwards movement of the oceanic polar front and return of relatively warm surface waters to the latitude of Scotland. This produced rapid climatic warming (Atkinson et al., Citation1987; Brooks & Birks, Citation2000) and disappearance of the glaciers that had formed in the Highlands during the Loch Lomond Stade of ca. 12.9–11.5 kyr. Temperate conditions similar to those of the present were established by 11.0 kyr. Our knowledge of subsequent climatic change in Scotland is derived from various types of proxy evidence, such as pollen assemblages, plant macrofossils and testate amoebae in peat bogs and lake sediments, tree remains in peat and peat humification data (e.g. Dubois & Ferguson, Citation1985; Bridge et al., Citation1990; Huntley & Prentice, Citation1993; Birks, Citation1996; Anderson, Citation1998; Anderson et al., Citation1998; Huntley, Citation1999; Tisdall, Citation2003; Whittington & Edwards, Citation2003; Ballantyne, Citation2004), supplemented by historical records and instrumental data for the past few centuries. There is widespread acceptance that apart from a short-lived cooling event that affected NW Europe at around 8.2 kyr (Klitgaard-Kristensen et al., Citation1998), much of the early Holocene was characterized by relatively warm summers (the ‘climatic optimum’) punctuated by periods of increased wetness. Temperatures during the past 5000 years appear to have oscillated around ‘average’ values by as much as ± 2°C over decades or longer (Briffa & Atkinson, Citation1997), and proxy data from peat bogs indicate at least local shifts in wetness during this period (e.g. Tisdall, Citation2003; Blundell & Barber, Citation2005; Langdon & Barber, Citation2005). During the sixteenth to eighteenth centuries AD Scotland experienced general climatic deterioration during a period often referred to as the ‘Little Ice Age’, even though there is no evidence for recrudescence of mountain glaciers in Scotland at this time. Historical evidence shows that particular years and decades during the Little Ice Age were characterized by cool, wet summers, exceptional storminess, and survival of perennial snowcover on high ground (e.g. Manley, Citation1971; Lamb, Citation1977, Citation1984, 1991; Whittington, Citation1985; Mitchell, Citation1999).
Since Holocene landscape change in the Scottish Highlands was last reviewed in this journal (Ballantyne, Citation1991a), there have been important advances in our understanding this topic, reflecting three developments. The first has been an expansion of interest in post-glacial geomorphic change, manifest in several publications that have radically altered our understanding of the nature of landscape change following deglaciation. The second has been more extensive use of radiocarbon dating techniques to establish the age of Holocene landforms and deposits, supplemented by two powerful new dating tools, optically-stimulated luminescence (OSL) dating and surface exposure dating using cosmogenic isotopes (for an account of these methods see Walker (Citation2005)). The third important change has been the widespread adoption of the concept of paraglacial landscape modification as a paradigm for understanding how the landscapes of the Highlands have altered since deglaciation. The aim of this review is not only to update the earlier paper, but also to demonstrate how many Holocene landscape changes in the Highlands can be related to the paraglacial concept. The review considers in turn the nature of the paraglacial concept, paraglacial adjustment of rock slopes and drift-mantled slopes, Holocene alluvial history, the geomorphic effects of wind action and the effects of Holocene periglacial processes on high ground. Locations mentioned in the text are shown in .
The Paraglacial Concept
Paraglacial geomorphology is the study of the ways in which glaciated landscapes adjust to non-glacial conditions. The term ‘paraglacial’ was introduced by Ryder (Citation1971) to describe “non-glacial processes that are conditioned by glaciation” (Church & Ryder, Citation1972, p. 3059), but its widening usage has prompted its redefinition as a descriptor of “non-glacial Earth-surface processes, sediment accumulations, landforms, landsystems and landscapes that are directly conditioned by glaciation and deglaciation” (Ballantyne, Citation2002a, p. 1938). Retreat of glacier ice often results in exposure of metastable, glacially-conditioned sediment sources that are subsequently released and reworked by various geomorphic processes over a wide range of timescales. Rock masses stressed by the weight of glacier ice experience decompression, opening of stress-release joints and adjustment through rockfalls, rockslides, rock avalanches and rock mass creep. Glacigenic sediments on hillslopes may be reworked by landslides, debris flows, snow avalanches and mountain torrents. Valley-floor glacigenic sediments (till, glacifluvial deposits and glacilacustrine sediments) are eroded, transported and redeposited by rivers, forming alluvial fans and valley fills, or deltas and bottom deposits in lakes and fjords. The paraglacial system may thus be envisaged as an interrupted sediment cascade () in which non-renewable sediment sources (unstable rockwalls, drift-mantled slopes and valley-floor glacigenic deposits) are released or entrained by a range of processes and transported towards terminal sediment sinks. However, source-to-sink transport is often interrupted by sediment redeposition in paraglacial sediment stores such as talus accumulations, debris cones, alluvial fans, valley fills and terraces. Some paraglacial sediment stores continue to accumulate over several millennia, but others experience degradation within a few centuries or millennia of formation (Ballantyne, Citation2002a, Citation2003a, Citation2007a).
Figure 2 Simplified terrestrial paraglacial sediment cascade. Most primary sediment stores have experienced a transition from net accumulation to net erosion during the Holocene. Source: Adapted from Ballantyne (Citation2002a) with permission from Elsevier
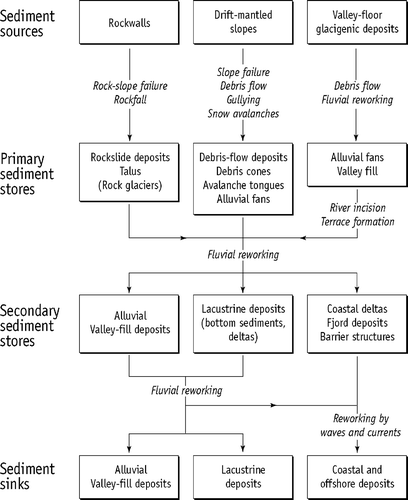
Models of Paraglacial Sediment Yield
Ballantyne (Citation2002b) has argued that for paraglacial systems in which sediment sources are not replenished, the trajectory of sediment reworking through time can be approximated by an exhaustion model in which rate of sediment yield is related to the remaining sediment available for reworking by a negative exponential function:
where t is time elapsed since deglaciation, St is sediment available for reworking at time t, S0 is the sediment available for reworking at t = 0 and λ is the rate of sediment loss by release and/or stabilization (). The value of λ varies over several orders of magnitude. On steep drift-mantled slopes, for example, most sediment reworking may be complete within a few centuries of deglaciation (e.g. Ballantyne & Benn, Citation1994; Curry, Citation1999a, Citation1999b), whereas rock-mass adjustment to glacial unloading may persist for several millennia after deglaciation (e.g. Cruden & Hu, Citation1993; Ballantyne & Stone, Citation2004). The exhaustion model assumes steady-state conditions under which there is no change in process-generating or other boundary conditions. Over millennial timescales this is rarely the case, and paraglacial sediment flux may be influenced by climate change (resulting in changes in catchment hydrology or the magnitude or frequency of extreme rainstorm events), tectonic activity (uplift, tilting or seismic events), base-level change or anthropogenic influences such as deforestation. Such extrinsic perturbations may prolong, rejuvenate or reinitiate paraglacial sediment reworking long after the initial period of paraglacial landscape adjustment is complete (Ballantyne, Citation2002b).
Figure 3 Exhaustion model of paraglacial sediment release, in which rate of sediment release (λ) is related to the proportion of ‘available’ sediment remaining in the sediment source. In this example λ = 0.0005 yr−1 (i.e. 0.05% of remaining ‘available’ sediment is removed each year)
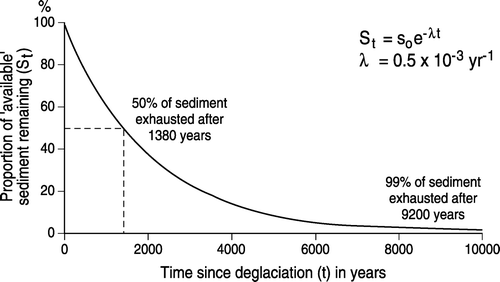
As paraglacial sediment stores such as talus cones, debris cones and alluvial fans accumulate, they are subject to erosional losses. By assuming that rates of erosional loss are ‘sediment-conditioned’ (i.e. related to the volume of sediment in the sediment store), the volume of sediment in storage (S) at time t can be approximated by:
where κ is the rate of sediment loss from the store (Ballantyne, Citation2003a). If S0 is set at 1.00, this simplifies to:
illustrates the change in sediment storage for λ = 1.0 ka−1 and a range of values of κ. An implication of this model is that for all paraglacial sediment stores that experience erosional losses (i.e. κ > 0), an eventual change from net sediment accumulation to net sediment loss is an intrinsic property of paraglacial sediment storage, reflecting the non-renewable nature of paraglacial sediment sources. This concept explains why most Holocene landforms in the Scottish Highlands (talus accumulations, debris cones and alluvial fans) are relict features on which erosion has succeeded deposition as the dominant mode of activity. It also explains why many Holocene floodplains appear to have experienced an initial period of aggradation followed by a later period of river incision and terrace development. However, in many cases other influences (such as exceptional storm events, changes in base level and land-use change) are superimposed on this model of paraglacial development (e.g. Ballantyne, Citation1991b; Lewin et al., Citation2005), complicating Holocene landform evolution.
Figure 4 Change in volume of stored sediment as a fraction of the volume of ‘available’ sediment in a paraglacial sediment source plotted against time elapsed since deglaciation for λ = 1.0 kyr−1 (0.001 yr−1) and a range of values of κ (see text). For κ > 0, storage inevitably reaches a peak then declines. The maximum volume of sediment held in storage and the timing of maximum storage depend on the relative values of λ and κ. Source: Adapted from Ballantyne (Citation2003a)
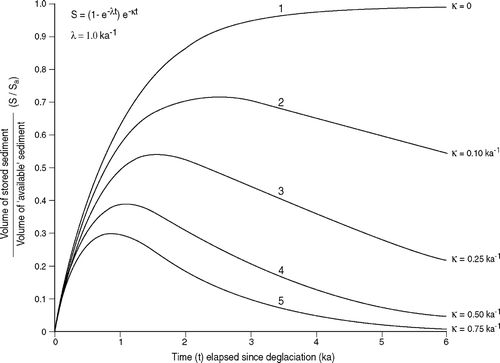
Not all aspects of Holocene geomorphic activity in the Scottish Highlands reflect paraglacial landscape change: periglacial features on high ground and those produced by wind action owe little if anything to glacial conditioning, and some debris cones at the foot of rock gullies may have developed independently of prior glacial influences. However, the dominant Holocene landform assemblages—rock slope failures, talus accumulations, debris cones, alluvial fans, alluvial valley fills, river terraces and deltas—almost invariably reflect modification of glacially-conditioned sediment sources by postglacial processes.
Paraglacial Adjustment of Rock Slopes
Glaciation and deglaciation condition rock slope stability in two ways. Glacial erosion, particularly along troughs, tends to steepen and lengthen rock slopes. Rock-slope steepening increases self-weight shear stresses within the rock mass, and deepening of troughs by glacial erosion has a similar effect by increasing the rock-slope height. These effects may generate tensile conditions under the lower slope, promoting rock mass weakening (Augustinus, Citation1995). The second way in which glaciation affects rock slopes is through differential loading under the weight of glacier ice. Part of the resulting ice-load deformation is stored within the rock mass as strain energy. Decompression of rock masses during deglaciation results in release of strain energy, resulting in major changes in the orientation of the principal stress field and development of a zone of tension within the slope. Relaxation of tensile stresses during and after ice downwastage causes ‘rebound’ or stress-release within the rock. This results in propagation of the internal joint (fracture) network, with associated loss of cohesion along joint planes and reduction of internal locking stresses.
The response of glacially-steepened rock to deglaciation is conditioned by bedrock lithology and structure, and in particular by joint density and the orientation and inclination of joints and other planes of weakness relative to that of the valley-side slope. In the Highlands, three types of paraglacial response are evident: catastrophic rock-slope failure (slides, topples and rock avalanches, with complete or arrested runout of debris); rock-mass deformations, where the rock slope has experienced limited displacement, without debris runout; and slow recovery of equilibrium through numerous rockfalls, resulting in progressive accumulation of talus against the lower slope. However, in many areas rock slopes have remained stable, and have been largely unaffected by paraglacial adjustment; this is true even of some steep cliffed slopes, particularly where these are developed in massive lithologies, or rocks with gently-dipping beds.
Over 600 sites of rock-slope failure (RSF) have been recorded in the Highlands and Islands of Scotland (Ballantyne, Citation1986a; Jarman, Citation2007a), and others remain to be identified. Jarman (Citation2006) has documented 140 rock-slope failures exceeding 0.25 km2 in area on the Scottish Mainland, and classified these into five types (). Three of these (‘cataclasmic’, ‘sub-cataclasmic’ and ‘arrested’) represent catastrophic failure, with different degrees of debris runout. The remaining two types represent rock-mass deformation. However, many Scottish RSFs exhibit characteristics of more than one category of failure, and definitive classification of individual RSFs is often difficult. Overall, arrested translational slides are probably most common, but the majority of large (> 0.25 km2) RSFs are slope deformations.
Figure 5 Classification of large rock-slope failures in the Scottish Highlands. All categories depicted represent idealized end members of a continuum of failure types. Source: Adapted from Jarman (Citation2006), with permission from Elsevier
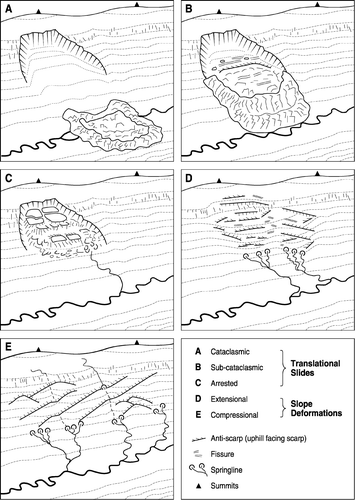
Catastrophic Failures
Catastrophic failures are characterized by distinct headscarps and flank scarps, a distinctive failure plane and translocation of failed rock downslope in the form of a coherent mass, large blocks of intact rock, an apron of runout debris or, rarely, large debris-flow lobes ( –). Semi-intact arrested translational slides probably represent the majority of catastrophic failures (Jarman, Citation2006): good examples include the Tullich Hill RSF in the SW Highlands, the An Sornach RSF in upper Glen Affric and a slide in upper Glen Roy that over-ran lake shorelines of Loch Lomond Stadial age (Sissons & Cornish, Citation1982; ). The most spectacular catastrophic failure in Scotland is the Beinn Alligin rock avalanche. Approximately 4000 years ago, about 9 Mt of sandstone bedrock south of the summit of Beinn Alligin (Torridon) failed by sliding down a 42° failure plane bounded by upslope-converging fault scarps. The exceptional energy of this failure allowed the rock debris to surge up the opposite side of the corrie and along the corrie floor for 1.25 km (Ballantyne, Citation2003b; Ballantyne & Stone, Citation2004; ). Another impressive rock avalanche occurred near the mouth of Coire Gabhail near Glencoe, where about 0.6 Mt of rhyolitic lavas failed about 1800 years ago and accumulated as a massive talus cone that dammed the upper glen (Ballantyne, Citation2007d; ). At a handful of sites, sliding failure has resulted in viscous flow of rock debris to form thick debris tongues bounded by levées. Examples of this type of runout occur on Ben Gulabin, near Spittal of Glenshee in the SE Grampians, and in Gleann na Guiserein, Knoydart (Ballantyne, Citation1992, Citation2007e; ).
Figure 6 Arrested translational rockslide in Glen Roy (Lochaber). The slide has disrupted and over-run shorelines formed at the margins of a lake that was dammed by glacier ice during the Loch Lomond Stade
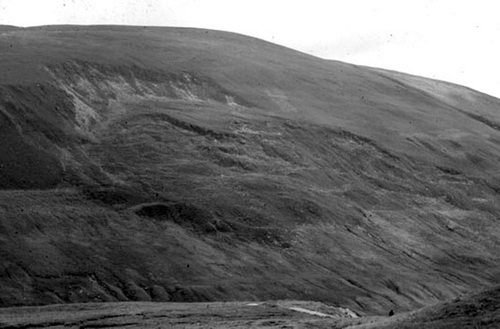
Figure 7 The Beinn Alligin rock avalanche, Torridon. Roughly nine million tonnes of rock failed along a 42° failure plane bounded by converging fault scarps and travelled 1.25 km down the floor of a corrie. Cosmogenic isotope dating of runout boulders places the date of failure at 4.0 ± 0.3 kyr, approximately 7500 years after deglaciation
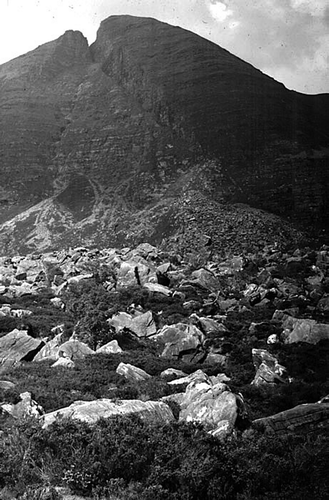
Figure 8 The Coire Gabhail rock avalanche, Glen Coe. Failure of rhyolitic lavas about 1800 years ago resulted in the formation of a huge talus cone
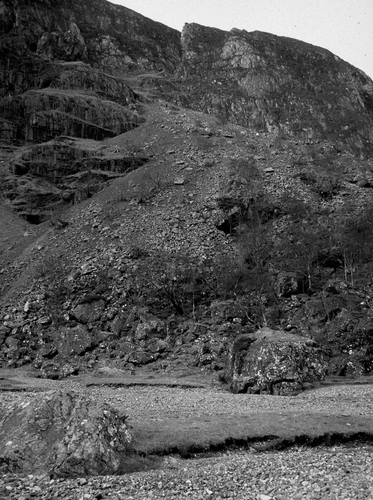
Figure 9 The Carn Ban rock-slope failure near Spittal of Glenshee. Initial sliding failure along bedding planes generated two massive debris flow lobes on either side of a bedrock protrusion
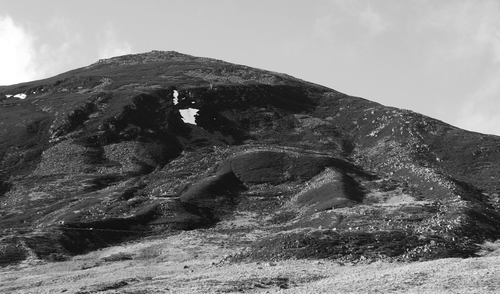
A distinctive group of RSFs occurs on Skye and Mull where Palaeocene basaltic lavas overlie relatively weak sedimentary rocks of Mesozoic age. Where this succession is exposed in steep cliffs, failure of the sedimentary rocks under the weight of the lavas has produced lateral movements of lava blocks. The archetype of this type of terrain is the Trotternish escarpment in northern Skye. East of the escarpment is the most extensive area of landside terrain in Britain, occupying ∼40 km2 and subdivided into two zones: an outer zone of subdued, ice-moulded landslide blocks that was over-ridden by the last ice sheet, and an inner zone of tabular landslide blocks and pinnacles that represent rock-slope failure since deglaciation (). Although failure of the scarp has traditionally been attributed to rotational sliding within the sedimentary rocks (e.g. Anderson & Dunham, Citation1966; Ballantyne, Citation1991b) the configuration of detached blocks suggests planar sliding or gliding of lavas over deforming shale (Ballantyne, Citation2007f). Near the southern end of the escarpment the entire SE face The Storr (719 m) collapsed about 6500 years ago to form a great hollow (Coire Faoin) occupied by a labyrinth of lava blocks, narrow defiles and shattered pinnacles (Ballantyne et al., Citation1998).
Figure 10 The Trotternish escarpment, northern Skye. The area of landslide east of the scarp (shaded) is the largest area of landslide terrain in Britain, but only the inner zone of angular landslide blocks represents failure since deglaciation, which in this area occurred about 17 000 years ago. Cosmogenic isotope dating of landslide pinnacles indicates that The Storr landslide occurred about 6500 years ago
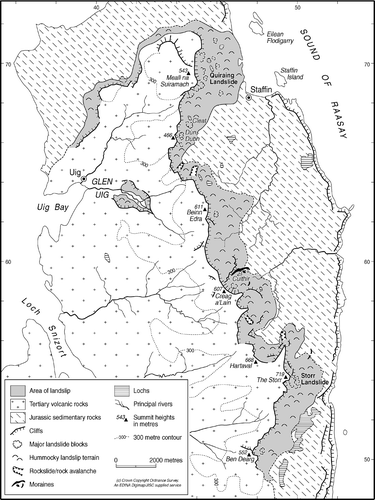
Slope Deformations
Unlike catastrophic failures that have involved significant downslope movement of landslide debris, slope deformations involve limited downslope displacement of largely intact rock slopes; lateral margins are indistinct, and headscarps are not always present. Slope deformations can be huge, involving entire mountainsides. The largest, on Beinn Fhada (Ben Attow) above Gleann Lichd in Kintail, covers approximately 3 km2 (Jarman & Ballantyne, Citation2002; ); that on Ben Our near Loch Earn is approximately 2.9 km2 in area (Jarman, Citation2007b). Jarman (Citation2006) has suggested that 89 RSFs > 0.25 km2 on the Scottish mainland can be classified as slope deformations. He suggested that these may be subdivided into extensional and compressional types. The former are characterized by distinctive headscarps, upper slopes supporting ridge-top trenches and crevasse-like fissures aligned along-slope, and bulging slopes crossed by uphill-facing rock scarps (antiscarps). Compressional deformations tend to lack a headscarp, and exhibit limited overall deformation characterized by upthrust antiscarps. However, the distinction between the two types is not always clear, and individual slope deformations may involve both extensional and compressional zones, with extension evident on the upper slope and compression farther downslope.
Figure 11 The Beinn Fhada slope deformation in Kintail, which covers an area of about 3 km2 and supports slope bulges and antiscarps up to 800 m long and 10 m high. Source: Photograph by D. Jarman
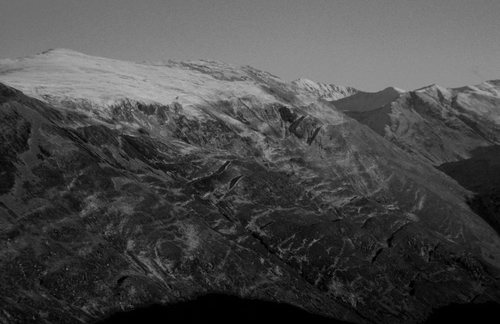
Much remains speculative about the nature of slope deformation in the Scottish Highlands. It is not established, for example, whether downslope displacement occurred in a single rapid event, in a series of intermittent shifts or gradually as a result of rock mass creep. The nature of failure is also unclear: this may have occurred over a continuous shear surface, or two or more shear planes separated by zones of fractured rock, or over a deep-seated zone of crushed rock. The nature of antiscarp development is also contested. These impressive rock scarps, commonly up to 5 m high and sometimes extending hundreds of metres across the slope, could represent outwards rotation of blocks within the deforming slope, or possibly reverse faulting induced by differential stress release due to unequal ice-loading of summits and valley floors (Stewart et al., Citation2000; Jarman & Ballantyne, Citation2002; Jarman, Citation2006).
Distribution and Timing of Rock-Slope Failure
On the mainland, the great majority of RSFs are associated with Moine and Dalradian metasediments, particularly in areas of steep relief, but the distribution of RSFs on such terrain is uneven, with distinct clusters in some areas such as Kintail, Lochaber and the Arrochar region (Ballantyne, Citation1986a; Jarman, Citation2006; ). Proposed explanations for these clusters in terms of former glacier limits and palaeoseismicity (e.g. Fenton, Citation1992; Ballantyne, Citation1997a) appear inadequate to explain this distribution. Jarman (Citation2006, Citation2007a) has noted an association with steep-sided glacial breaches, but the relationship is imperfect: some breaches lack RSFs and many RSFs are not associated with breaches. A more general explanation is that RSFs tend to be associated with narrow, steep-sided troughs (including glacial breaches) that were occupied by fast-moving, warm-based ice at some stage during the last ice-sheet glaciation, and therefore experienced marked valley-wall steepening and valley-floor deepening, conditions conducive to paraglacial rock-slope failure.
Figure 12 Distribution of rock-slope failures exceeding 0.25 km2 in area on the Scottish mainland, showing the clustering of large RSFs in some areas such as Kintail, Lochaber and Arrochar. Source: Adapted from Jarman (Citation2006), with permission from Elsevier
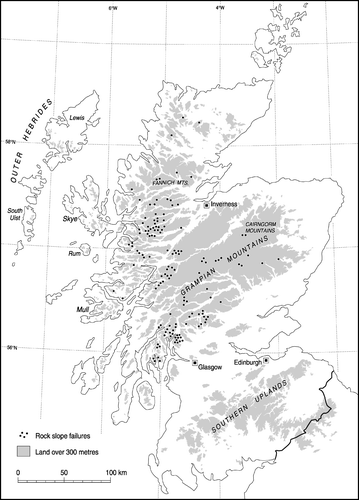
Most RSFs in the Scottish Highlands occur within the limits of Loch Lomond Stadial glaciation, and are therefore demonstrably of Holocene age. The exhaustion model of paraglacial landscape modification predicts that the frequency of rock-slope failure should be greatest immediately after deglaciation and decline in frequency thereafter (Cruden & Hu, Citation1993; Ballantyne, Citation2002a). However, reliable dating evidence for the timing of RSFs in the Scottish Highlands is very limited. Granite RSFs that have been dated using cosmogenic 10Be failed during the Lateglacial period prior to 11.5 kyr (Ballantyne et al., Citation2008) and a small number of RSFs exhibit evidence of failure during the Loch Lomond Stade, notably a quartzite RSF on Maol Cheann-Dearg (Torridon) where the runout debris was apparently redistributed by glacier ice. However, other RSFs dated using cosmogenic isotopes occurred during the mid or late Holocene, notably the Storr landslide on Skye (6.5 ± 0.5 kyr; Ballantyne et al., Citation1998), the Beinn Alligin rock avalanche (4.0 ± 0.3 kyr; Ballantyne & Stone, Citation2004) and the Coire Gabhail rock avalanche (ca. 1.8 kyr; Ballantyne, Citation2007d). However, no data have yet been published on the ages of more ‘typical’ RSFs on Moine or Dalradian metasediments, so it is premature to conjecture the temporal pattern of Holocene RSF activity.
Rockfalls and Talus Accumulation
The most common form of paraglacial rock-slope adjustment in many areas does not involve large-scale rock-slope failure, but gradual modification of steep rockwalls by intermittent rockfalls, sometimes accompanied by overall decline in rock-slope gradient. Rockfall debris has gradually accumulated as talus at the slope foot; talus sheets have formed where rockfall delivery has been fairly uniform across the slope, and talus cones have developed where rockfall has been focused by gullies in the parent cliff. Both types of accumulation comprise an upper rectilinear slope resting at a gradient of 34–38° and a basal concavity.
Although occasional rockfalls still occur (Ballantyne & Eckford, Citation1984), almost all talus accumulations in the Highlands are relict landforms that support extensive vegetation cover and exhibit evidence for erosion by stream incision, shallow landslips and particularly debris flows, implying that erosion has replaced accumulation as the dominant mode of geomorphic activity (Ballantyne & Harris, Citation1994, pp. 219–226; ). Several authors have also noted that talus accumulations located outside the limits of Loch Lomond Stadial glaciation tend to be markedly more mature than those developed during the Holocene inside these glacial limits (Ballantyne & Eckford, Citation1984; Kotarba, Citation1984). This contrast suggests that talus accumulation was more rapid during the Late Devensian Lateglacial (prior to 11.5 kyr) than during the Holocene. Although this contrast almost certainly represents more effective frost wedging of rockwalls under severe periglacial conditions during the Lateglacial, it probably also reflects the initial instability of glacially-steepened rockwalls following downwastage of the last ice sheet (Ballantyne & Kirkbride, Citation1987; Hinchliffe & Ballantyne, Citation1999).
Figure 13 Relict talus accumulations at the foot of the Trotternish escarpment, northern Skye. Radiocarbon dating of buried soils exposed in gullies incised into the talus has allowed reconstruction of the history of talus accumulation and reworking by debris flows at this site. Most talus accumulation occurred in the Lateglacial and early Holocene
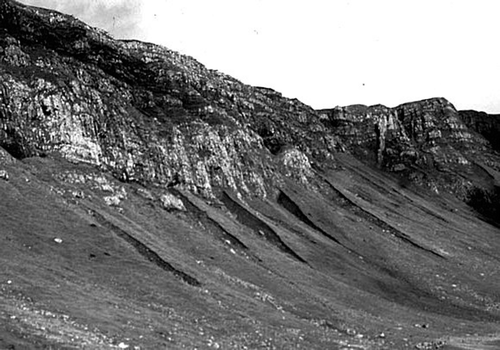
On the Trotternish escarpment in northern Skye, gullies incised deep into vegetated talus sheets () reveal the stratigraphy of relict talus deposits, and have allowed the history of talus accumulation to be reconstructed (Hinchliffe et al., Citation1998; Hinchliffe & Ballantyne, Citation1999). Calculations based on the volume of talus at the foot of a basalt cliff indicate that 4.3–7.8 m of cliff retreat has occurred since deglaciation at ca. 17.0 kyr, and the stratigraphy of the talus suggests that about 80% of rockwall retreat occurred in the interval between ice-sheet downwastage and the beginning of the Holocene (17.0–11.5 kyr). During the Holocene, talus accumulation was succeeded by net erosion and reworking of talus sediments by debris flows and slopewash (Hinchliffe, Citation1999). Interestingly, some 27–30% of the sediment in relict talus accumulations comprises sand and silt particles derived from granular weathering of the cliff face (Salt & Ballantyne, Citation1997; Hinchliffe et al., Citation1998). Hinchliffe & Ballantyne (Citation1999) calculated that late Holocene cliff retreat rates at the Trotternish escarpment were less than 0.1 m kyr−1, most of which is probably attributable to granular weathering rather than rockfall.
Synthesis
Since deglaciation, rock slopes in the Highlands have experienced paraglacial stress release in response to differential deglacial unloading. The nature of rock-slope response has depended on a complex of factors, of which lithology, structure, glacial history and degree of glacial modification are probably the most important. Many steep rock slopes have remained essentially stable, or experienced gradual adjustment through intermittent rockfall, most of which occurred during the Lateglacial and/or early Holocene. Most talus accumulations produced by intermittent rockfall are now relict and eroded. However, at over 600 locations large-scale slope failure has occurred. In many cases, this took the form of large-scale slope deformation, with limited downslope displacement of rock masses. Elsewhere, catastrophic failure resulted in arrested translational slides or rock avalanches or rockslides associated with runout of coarse bouldery debris. The causes and timing of these events are still poorly understood, although the persistence of dated catastrophic failures into the late Holocene implies that the possibility of future high-magnitude RSFs cannot be excluded.
Paraglacial Modification of Drift-mantled Slopes
Debris-flow Activity
The retreat of glacier ice, particularly at the end of the Loch Lomond Stade (12.9–11.5 kyr) left many slopes in the Highlands blanketed in a thick mantle of glacigenic sediment, often composed of stacked lateral moraines (e.g. Ballantyne, Citation2002c, Citation2007b; Benn & Ballantyne, Citation2005). In many areas, particularly on slopes <25°, such drift cover has remained largely intact except where it has been dissected by tributary streams. In others, it has been extensively reworked by paraglacial processes, particularly by debris flows, so that little remains of the original drift cover.
A debris flow is the rapid downslope flow of poorly-sorted debris mixed with water. The term is also used to describe the resulting landform assemblage, which consists of a steep-sided feeder gully upslope, a narrow track (commonly a few metres wide) defined on either side by bouldery levées, and a terminal lobe or lobes (). Two types are widespread on steep mountain slopes: hillslope debris flows, which occur on open hillslopes, and valley-confined debris flows that originate in bedrock gullies and are channeled for part of their length along gully floors. Deposition by successive debris flows has in places resulted in the formation of debris cones at the slope foot. Superb examples line the flanks of many glacial troughs, such as Glen Etive, Glen Coe, and Gleann Einich (). Low-viscosity flows with high water content may also spread laterally, covering wider areas of slope than high-viscosity flows confined by levées. Debris flow is presently the dominant agent of mass movement in many parts of the Highlands, although individual flows are usually small. A survey by Innes (Citation1985) suggested that 90% of recent flows carried <60 m3 (∼18 t) of sediment, although some low-viscosity valley-confined flows have transported > 1000 m3 (∼300 t) of debris (Common, Citation1954; Brazier & Ballantyne, Citation1989).
Figure 14 A hillslope debris flow in the Lairig Ghru, Cairngorm Mountains, photographed soon after a heavy rainstorm in 1978 released 71 debris flows into the valley
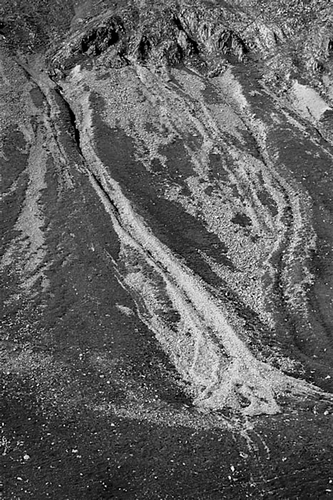
Figure 15 Debris flows and debris cones on the flanks of glacial troughs: Gleann Einich, Cairngorms (left) and Glen Docherty near Kinlochewe (right). Source: Adapted from a map in Curry (Citation1999a) and reproduced with the author's permission
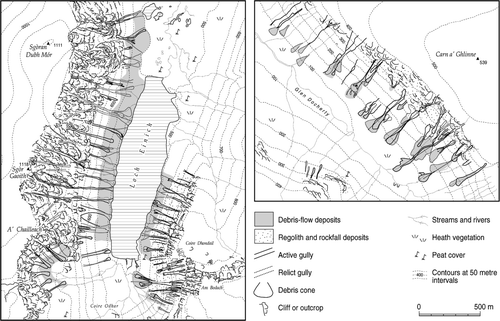
Debris flows on open hillslopes are usually initiated by shallow (0.3–3.0 m thick) translational landslides, often over bedrock; the transition from sliding to flow reflects loss of internal frictional strength, with consequent liquifaction and remoulding of the mobile sediment (Jenkins et al., Citation1988). Within gullies, however, flood torrents may be transformed into debris flows by the addition of sediment from gully walls and floors (Bovis & Dagg, Citation1992) or failure of debris dams (van Steijn et al., Citation1988). In all cases, debris flows are initiated when a build-up of porewater pressures in sediments reduces shearing resistance, leading to failure and flow. All documented instances of recent debris flows in Scotland have occurred during prolonged rainstorms of exceptional intensity (Common, Citation1954; Baird & Lewis, Citation1957; Jenkins et al., Citation1988; Luckman, Citation1992). At sites that are prone to recurrent debris-flow activity, such as the Lairig Ghru or Drumochter Pass, the present recurrence interval between debris flow events is of the order of 10–50 years (Ballantyne, Citation2002d). An exceptional rainstorm in 1978 produced 71 individual flows in the Lairig Ghru (Innes, Citation1983a, Luckman, Citation1992; ), but since then no debris flows have occurred in this valley.
Hillslope debris flows are generated on sediment-mantled slopes of 30–46° (Innes, Citation1983b), although valley-confined flows may be initiated on gully floors with slightly gentler gradients. Hillslope flows tend to be most common on slopes mantled by sediment with a cohesionless sandy matrix, and are therefore particularly widespread on sandstone and granite mountainsides (Innes, Citation1983b, Citation1986). Hillslope flows occur on talus (Hinchliffe et al., Citation1998; Hinchliffe, Citation1999) and regolith derived from weathered bedrock (Innes, Citation1986; Reid & Thomas, Citation2006), but are most widespread on glacigenic deposits mantling valley-side slopes (Baird & Lewis, Citation1957; Brazier et al., Citation1988; Brazier & Ballantyne, Citation1989, Ballantyne & Benn, Citation1996; Curry Citation2000a, Citation2000b; ). The characteristic landforms produced by drift reworking are deep drift-cut gullies feeding coalescing debris cones with surface gradients averaging 10–25°.
Timing of Drift-Slope Reworking
Research in the Jostedalen area of Norway has shown that debris flows may cause extensive reworking of valley-side drift deposits (nested lateral moraines) within a few decades of recent deglaciation (Ballantyne & Benn, Citation1994, Citation1996; Ballantyne, Citation1995; Curry Citation1999b), creating a paraglacial landsystem of deep drift gullies feeding coalescing debris cones. In some areas of Scotland, stratigraphic evidence suggests that extensive reworking of hillslope drift by debris flows similarly occurred during or immediately after deglaciation (Benn, Citation1992; Ballantyne & Benn, Citation1996; Bennett, Citation1999). However, at many sites there is evidence that remobilization of drift by debris flows has also occurred intermittently throughout the Holocene. Such evidence takes the form of buried soil or peat horizons within debris-flow deposits on lower slopes, particularly within debris cones. The development of a soil or peat layer implies a prolonged period of slope stability between flow events. Radiocarbon dates obtained for the top of a buried soil or peat layer yield a maximum age for the overlying debris-flow deposit, whereas dates obtained for the base of a buried soil or peat layer provide a minimum age for the underlying debris flow deposit. At some locations, stacked debris flow deposits are separated by two or more dated soil or peat horizons (), allowing a fairly detailed history of Holocene debris-flow activity to be reconstructed (Brazier & Ballantyne, Citation1989; Hinchliffe, Citation1999; Curry, Citation2000a, Citation2000b; Reid & Thomas, Citation2006).
Figure 16 Section cut in debris-flow deposits, Trotternish, northern Skye. The dark bands are buried organic soils, and the intervening and overlying beds are debris-flow deposits that buried the soils. Radiocarbon dating of the top and base of individual soil horizons allows the timing of debris flows to be reconstructed
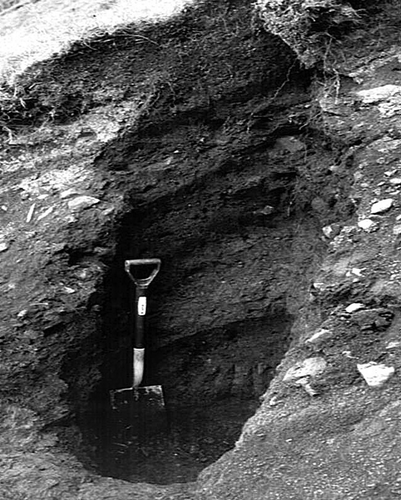
The dating evidence from nine sites in the Scottish Highlands is summarized in . The data show that intermittent debris-flow activity has reworked glacial drift, talus deposits and weathered bedrock over at least the last 7000 years. The absence of older buried soil or peat layers is probably a sampling artifact, as most sites have been sampled to depths of only 1–3 m. As large debris cones reach depths of 10 m or more, an implication is that rapid debris cone accumulation occurred in the early Holocene prior to ∼7.0 cal kyr BP, consistent with the paraglacial exhaustion model and with research on rapid paraglacial reworking of drift-mantled slopes in Norway (Ballantyne & Benn, Citation1994, Citation1996; Curry, Citation1999b).
Figure 17 Calibrated radiocarbon ages relating to debris-flow units overlying or underlying buried soils or peat layers at nine sites, showing possible periods of enhanced activity. The horizontal bars encompass the 95% confidence limits for each calibrated age
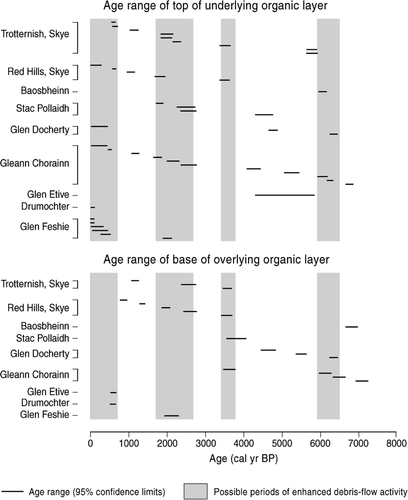
The radiocarbon dates obtained on organic soil and peat horizons intercalated with debris-flow deposits () nonetheless demonstrate renewed or delayed reworking of sediment-mantled slopes long after the cessation of initial paraglacial activity. The causes of such renewed sediment reworking are uncertain. No direct link has been established with anthropogenic activity, but it is possible that burning or deforestation locally increased the vulnerability of sediment-mantled slopes to failure and erosion by debris flows (Hinchliffe, Citation1999). Progressive pedogenesis may also have reduced the shearing resistance of steep drift-mantled slopes, rendering them more vulnerable to failure during extreme rainstorms (Brooks et al., Citation1995). Exceptional rainstorm events, possibly related to long-term cycles of climate change, represent the most likely trigger of debris flow activity following the initial phase of paraglacial hillslope readjustment in the early Holocene (Brazier & Ballantyne, Citation1989; Hinchliffe, Citation1999; Curry, Citation2000a, Citation2000b; Reid & Thomas, Citation2006). Although the available data () show that debris flow activity has occurred throughout much of the past 7000 years, clustering of radiocarbon ages suggests that periods of enhanced debris-flow activity may have occurred within the past 700 years, 1700–2700 years ago and (more tentatively) 3400–3800 and 5900–6400 years ago.
Considerable debate has centred on the proposition that reworking of hillslope sediments by debris flows has been enhanced during the past two or three centuries. This view was proposed by Innes (Citation1983a) on the basis of lichenometric dating of boulders on flow lobes. Innes' lichenometric data seemed to show that debris-flow activity had been negligible prior to about AD 1700, and increased radically thereafter. However, lichenometric dating of flow lobes suffers from a critical drawback, in that recent debris flows often bury older flow deposits, introducing a bias towards younger flow ages. This was illustrated by Luckman (Citation1992), who showed that flows generated by an extreme rainstorm event in the Lairig Ghru in 1978 obliterated a previous generation of flow lobes deposited in 1956 (). However, geomorphological evidence also supports recent enhancement of debris-flow activity in some areas. Ballantyne (Citation2002d) argued that if sites that have experienced recent widespread hillslope flow activity such as the Lairig Ghru, Glen Docherty and Gleann Einich () had experienced major debris-flow events throughout the Holocene, hillslope sediment cover would have been extensively removed within a few centuries or millennia (cf. Ballantyne & Benn, Citation1994; Curry, Citation1999b). Sediment sources on such slopes, however, are often largely intact but incised by fresh, drift-cut gullies that acted as sources for recent flows. The causes of possible recent enhancement of debris-flow activity, however, remain uncertain (Ballantyne, Citation1991c). Innes (Citation1983a) suggested that burning of heather to improve grazing may have increased the susceptibility of slopes to failure and consequent debris-flow activity by destroying bryophytes and thus increasing the likelihood of soil saturation during extreme rainstorms. However, Brazier & Ballantyne (Citation1989) argued that renewed debris-flow activity at a site in Glen Feshie within the past three centuries may have been triggered by one or more exceptional rainstorm events during the Little Ice Age of the sixteenth to nineteenth centuries AD, lowering the threshold for subsequent activity by stripping vegetation cover from gully sides.
Figure 18 Recent debris flows in the Lairig Ghru, Cairngorm Mountains, mapped from aerial photographs taken in 1961 and 1980 and showing extensive burial of an earlier (1956) generation of debris flow deposits by later (1978) flows. Source: Adapted from Luckman (Citation1992)
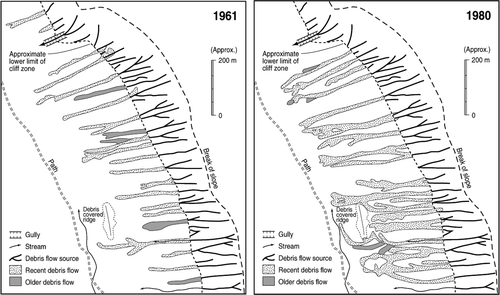
Whatever the cause of enhanced debris flow activity in the past few centuries, recent and anticipated climate changes seem likely to reduce the recurrence interval of major flow events. Annual precipitation totals for Scotland exhibit a general (although oscillatory) increase over the past century, with unprecedented high rainfall totals and annual river discharges in the last 25 years (Smith & Bennett, Citation1994; Smith, Citation1995; Barnett et al., Citation2006). A recent increase in flood frequency (Black, Citation1995, Citation1996; Black & Burns, Citation2002) also suggests that extreme rainstorm events are now more common than formerly, favouring more frequent debris-flow events than in the past.
Other Processes
Although debris flows represent the dominant agent responsible for paraglacial reworking of valley-side drift deposits in most areas, other processes have also modified the drift cover. Small translational slides have affected many drift-covered slopes, leaving cuspate failure scars, now degraded and vegetation-covered. Tributary streams have dissected lateral moraines on valley sides, and redeposited sediment as small paraglacial fans on valley floors. Snow avalanches were probably responsible for reworking valley-side drift during retreat of the last glaciers, but geomorphological evidence for recent avalanche activity is confined to only a handful of locations, and usually confined to small-scale effects such as uprooting of turf, localised erosion of talus and deposition of perched boulders downslope (Ward, Citation1985; Ward et al., Citation1985). Active avalanche landforms appear to be confined to a high corrie on Ben Nevis (Ballantyne, Citation1989) and high ground in the Cairngorms. The best examples occur in the Lairig Ghru, where avalanche boulder tongues extend across the valley floor and some distance up the opposite slope, damming the Pools of Dee at the highest point of the glen (Luckman, Citation1992; ).
Holocene Alluvial History
Since deglaciation, most of the sediment carried by Highland rivers has consisted of reworked glacigenic deposits; only a minor component of their load has been supplied by bedrock erosion or mass movement. The alluvial landforms and deposits of the Highlands are therefore essentially of paraglacial origin (). They fall into four categories: floodplain deposits or alluvial valley fill, produced by aggradation of fluvial sediments on valley floors; river terraces, produced by floodplain incision; alluvial fans, formed where tributary streams entering a trunk valley have deposited a low gradient fan (); and deltas, where rivers have deposited bedload on entering a loch or the sea.
Figure 20 Relict Holocene alluvial fan in Glen Elchaig, Kintail. The parent stream has incised the upper fan, and most of the fan is now vegetation-covered and inactive
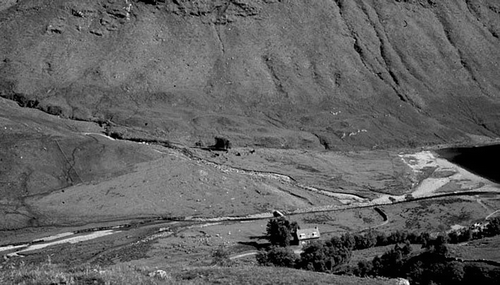
Highland rivers exhibit a great variety of channel patterns, including braided and meandering alluvial channels, bedrock-constricted reaches, mountain torrents and steep headwater streams (Werritty & Hoey, Citation2003). Most trunk valleys are occupied by wandering gravel-bed rivers (), characterized by a meandering planform with localised braiding and a tendency for channel migration through lateral erosion of banks or avulsion (channel switching during floods). Such rivers occupy alluvial basins separated by bedrock reaches that dictate local base level. Considerable research has been carried out on changes in alluvial channel patterns within the past few centuries or decades, and how such changes relate to climate, land-use change, river management and flood events (Werritty & Ferguson, Citation1980; Ferguson & Werritty, Citation1983; Werritty, Citation1984; Maizels, Citation1988; McEwen & Werritty, Citation1988; McEwen, Citation1989, Citation1994; Ferguson & Ashworth, Citation1990; Winterbottom, Citation2000; Werritty & Leys, Citation2001). However, recent reviews highlight the dearth of information regarding the longer-term Holocene evolution of alluvial landforms (McEwen, Citation1997; Werritty & McEwen, Citation1997). Indeed, the paucity of information on Holocene alluvial history represents a major gap in our understanding of the Highland landscape: few research papers deal with the long-term evolution of river terraces, valley fills or alluvial fans, and none with the deltas that have prograded into lochs and fjords since deglaciation.
Figure 21 The River Cononish, Strath Fillan, a wandering gravel-bed river typical of many in the Highlands, with a meandering planform exhibiting localised braiding
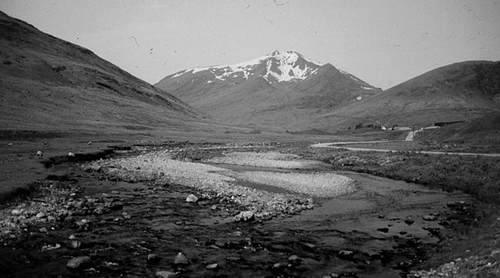
Alluvial Valley Fills and River Terraces
Alluvial valley fills represent aggradation of fluvial sediments on valley floors, forming low-gradient floodplains that occupy major valleys such as those drained by the Spey, Garry, Oykel, Carron and Etive and their major tributaries. In many Highland valleys the situation is complicated by thick fills of glacifluvial deposits laid down by powerful meltwater streams during glacier retreat. During the Lateglacial and early Holocene, major rivers cut down into these deposits, producing spectacular flights of glacifluvial terraces (Maizels & Aitken, Citation1991). However, there is also evidence that later in the Holocene net aggradation of valley fills occurred, possibly representing paraglacial redeposition of glacigenic deposits entrained farther upstream. This evidence takes the form of a prominent Holocene river terrace, up to about 3 m above the active floodplain, which occurs in many valleys, particularly in the Eastern Grampians. This terrace, the Main Holocene Terrace, implies that a period of Holocene floodplain aggradation was succeeded by renewed incision (). The Main Holocene Terrace was first identified in Glen Feshie by Robertson-Rintoul (Citation1986), who obtained a radiocarbon date on charcoal fragments buried by terrace gravels that demonstrates that Holocene floodplain aggradation culminated after ca. 3.9 kyr, and was succeeded by floodplain incision in the course of which three lower terraces were formed. Radiocarbon dates obtained on organic layers within a terrace buried by alluvial fan deposits in upper Gleann Lichd (Kintail) also indicate floodplain aggradation in the Late Holocene, after ca. 4.5 kyr (Reid et al., Citation2003). The Main Holocene Terrace in the Edendon Valley near Drumochter Pass has been more closely dated by Ballantyne & Whittington (Citation1999), who obtained three radiocarbon dates from organic deposits immediately below and immediately above the terrace surface. These dates demonstrated that Holocene floodplain aggradation in this valley culminated after 2.7 kyr but before 2.4 kyr, after which the river began to incise its floodplain. The cause of this change in behaviour is uncertain. Ballantyne & Whittington (Citation1999) found no evidence for deforestation in the pollen record at this site, and suggested that floodplain incision may reflect the onset of cooler and wetter conditions by ca. 2.6 kyr (cf. Briffa & Atkinson, Citation1997). An alternative possibility is that the onset of incision reflects the intrinsic change from net aggradation to net erosion that is characteristic of all paraglacial sediment stores (Ballantyne, Citation2003a; ).
Figure 22 Section through the Main Holocene Terrace of the River Findhorn in upper Strathdearn. The spade is resting on the edge of the active river channel. The stratigraphy of the terrace at this site comprises 1.1 m of coarse bar deposits overlain by 0.3 m of sandy overbank deposits and 0.9 m of late Holocene peat. The terrace implies that the Holocene floodplain at this site was once 1.4 m higher than now, and has subsequently been incised by the river
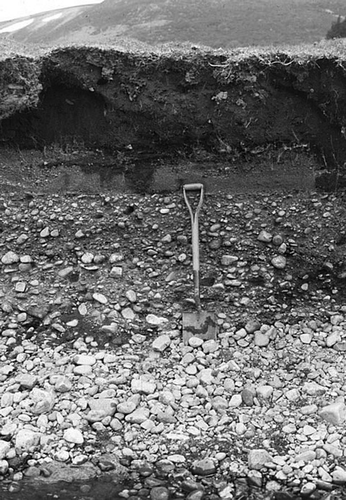
Alluvial Fans
Numerous alluvial fans occur where steep tributary valleys join glacial troughs, and rather more extensive fans are located are the confluence of major rivers, such as that at the mouth of the Feshie where it joins the Spey (McEwen, Citation1997). Most valley-side fans are vegetated, relict landforms, deeply incised by their parent streams. It seems likely that many are paraglacial fans that accumulated through reworking of glacigenic sediment by tributary streams soon after deglaciation. Several paraglacial fans in Glen Roy (Lochaber), for example, appear to have been formed in the interval between ice-sheet retreat and the damming of lakes in the glen by glacier ice during the Loch Lomond Stade (Peacock, Citation1986).
Other alluvial fans, however, developed much later. A small fan in the Edendon Valley near Drumochter Pass () has been shown, through radiocarbon dating of buried peat beds, to have formed through fluvial reworking of moraines during just three brief depositional events at around 2.2, 1.9 and 0.8 kyr (Ballantyne & Whittington, Citation1999). During each of these events, morainic deposits were stripped by flood torrents from the walls of a feeder gully, ultimately building a fan with a volume of ∼6750 m3. The three depositional events were apparently triggered by exceptional rainstorms, implying that the entire fan accumulated within a few hours within an overall timescale of slightly more than 2000 years. The Edendon fan represents a classic example of delayed paraglacial sedimentation (Ballantyne, Citation2002b), and its history suggests that other small relict fans in the Highlands may similarly have formed by episodic reworking of glacigenic sediments during a few exceptional flood events.
Figure 23 The Edendon alluvial fan in the central Grampians. The front of the fan has been exposed through lateral migration of the trunk stream, revealing three buried peat horizons. The lowest peat marks the top of the Main Holocene Terrace. Radiocarbon dating of the buried peats has shown that the entire fan was deposited during just three flood events between 2.3 kyr and 0.8 kyr
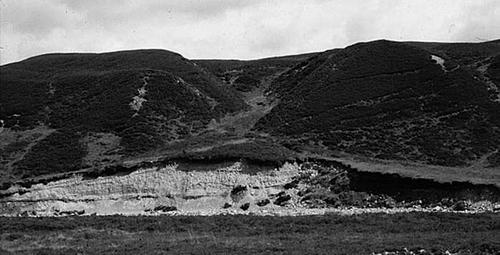
Few other fans in the Highlands have been securely dated. Several radiocarbon dates have been obtained from organic deposits associated with two ‘alluvial fans’ in upper Gleann Lichd (Kintail) by Reid et al. (Citation2003). The smaller of these probably represents a debris cone formed by successive debris flows after ca. 7.4 kyr. The larger occupies an area of about 125 000 m2 and appears to comprise alluvial sediments. Although the upper parts of this fan are undated, its toe prograded over a floodplain terrace after ca. 1.2 kyr, indicating continued deposition during the late Holocene. In Glen Etive, fluvial erosion of an early Holocene debris cone resulted in redeposition of alluvial sediment about 550 years ago (Brazier et al., Citation1988). The pollen record at this site and the presence of abundant charcoal fragments suggest that this event followed burning of woodland and clearance for agriculture, providing an example of geomorphic instability associated with human activity.
Synthesis
The evidence provided by radiocarbon dates associated with alluvial deposits in river terraces and fans at a handful of sites provides no more than tantalizing insights into the Holocene alluvial history of the Highlands. Whether this history is dominated by a paraglacial cycle of floodplain and fan aggradation and incision, as suggested here, remains in the realms of hypothesis. Some researchers (e.g. Macklin & Lewin, Citation2003; Lewin et al., Citation2005) have attempted to interpret the pattern of Holocene alluviation in Britain in terms of centennial-scale climate change, associated episodes of increased flood frequency and land-use change. It is possible that in the Scottish Highlands such influences are superimposed on a cycle of paraglacial aggradation and incision, but this remains to be determined through a more focused dating strategy than that hitherto adopted.
Geomorphic Effects of Wind Action
A notable feature of the climate of upland Scotland is the strength and persistence of the wind. Regionally, this reflects the location of Scotland in the path of depressions tracking along the North Atlantic Polar Front zone, particularly in winter. In upland areas, it also reflects concentration and acceleration of airflow as it passes over mountain barriers. At sea level, gusts of 100 km h−1 are not uncommon; at the summit of Cairngorm (1245 m) the average windspeed is about 47 km h−1 and 24-hour average windspeeds as high as 146 km h−1 have been recorded, with exceptional gusts exceeding 250 km h−1. The geomorphological influence of the wind is evident mainly on coasts, where sandy beaches furnish an abundant supply of cohesionless unvegetated sand, and on high plateaux, where stripping of vegetation cover exposes the underlying soil cover to wind scour.
Wind Action on Coasts
On the exposed coasts of the western Highlands, Holocene wind action is most evident in the accumulation of sand dunes inland from beaches (Ritchie, Citation1972; Robertson-Rintoul & Ritchie, Citation1990). Magnificent examples of bayhead dunefields occur on the Atlantic coast of the Outer Hebrides at Uig on Lewis and Luskentyre in south Harris (), and along parts of the machair of windblown calcareous shell sand that girts the west coast of South Uist (Hansom & Angus, Citation2005). Initial accumulation of many west coast dunefields appears to be related to slowing sea-level rise in the early Holocene. This enabled sediment transfer from nearshore shelves and consequent accumulation of beach sediments (Ritchie et al., Citation2001); beach sands were then reworked by westerly winds into dunes, often more than 10 m thick, that stabilized under vegetation cover. However, reduction of nearshore sediment supply has subsequently resulted in net erosion of many dune systems. Some have been eroded by shoreface erosion, and others have experienced the development of erosional blowouts, locally resulting in the formation of secondary dunes farther inland. Peat horizons and thick organic palaeosols exposed in eroded dune faces represent periods of stability, and overlying and underlying dune sands represent periods of dune-sand accumulation. Radiocarbon dating of buried peat and palaeosol units indicates that the timing of periods of sand accumulation and reworking varies from location to location, even between closely-spaced sites (Ritchie & Whittington, Citation1994; Gilbertson et al., Citation1999; Ritchie et al., Citation2001; Wilson, Citation2002). Some episodes of dune reworking appear to coincide with periods of cooler, stormier climate; in particular, there is evidence of dune destabilization and widespread sand blow during the Little Ice Age of the seventeenth and eighteenth centuries AD (Ritchie, Citation1979; Lamb, Citation1991; Wilson, Citation2002).
Figure 24 Coastal dunes at Luskentyre on the west coast of Harris, Outer Hebrides. At this site, removal of protective vegetation cover has resulted in renewed wind erosion of previously-stable dunes. Source: Reproduced with permission from Crawford (Citation2008)
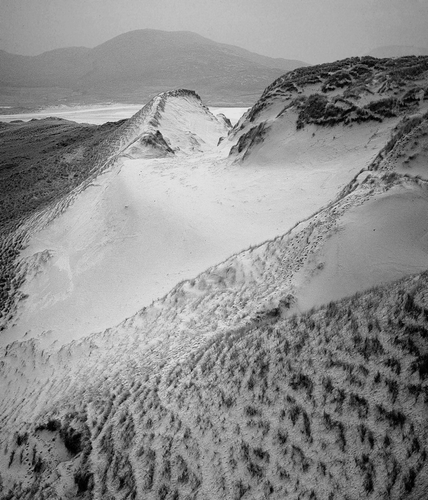
The erosional influence of winds on some beaches is also evident in the form of wind-abraded boulders and rock outcrops. Abrasion by windblown beach sand has modified exposed rock and boulder surfaces, forming polished facets and fluted, grooved and polished surfaces. Beautiful examples are illustrated by Wilson et al. (Citation2002) from beaches in Wester Ross and elsewhere in northern Scotland (). Wind abrasion has probably occurred throughout the Holocene, and orientation of abraded surfaces suggests a wind regime similar to that of today.
Wind Action on Mountains
On high plateaux, the effects of wind action are widespread. At exposed sites, wind-driven sand and ice particles have abraded upstanding boulders on some lithologies, producing distinctive facetted, fluted, pitted and polished surfaces (Christiansen, Citation2004). The interaction of wind and vegetation cover is evident on many plateaux in the development of aeolian microforms, notably deflation scars, wind stripes (straight or wavy lines of vegetation alternating with bare ground) and wind crescents, arcuate clumps of vegetation that slowly migrate across bare surfaces (Bayfield, Citation1984; Ballantyne & Harris, Citation1994, pp. 260–261). On upper slopes, a combination of strong winds, selective vegetation growth and downslope creep of soil has produced flights of turf-banked terraces, step-like microforms with vegetated risers up to a metre high and broader unvegetated treads (Ballantyne & Harris, Citation1994, pp. 261–267).
The most striking products of wind erosion on high plateaux are deflation surfaces, broad expanses of frost-weathered regolith where removal of vegetation cover has exposed the underlying soil to wind scour. Strong winds have winnowed away particles up to about 5 mm in diameter, leaving a sterile surface armoured by boulders and carpeted with lag gravels. Outstanding examples occur on the granite plateau of the Cairngorms and on the Torridon Sandstone mountains of NW Scotland (), but deflation surfaces occur on almost all lithologies and are developed as low as 400 m in the Northern Isles. In some locations, remnant vegetated ‘islands’ of windblown sand deposits or aeolisols stand proud of the adjacent wind-eroded terrain, demonstrating that sand or soil cover was formerly much more extensive, for example on the hills of Orkney and Shetland and the northern plateau of An Teallach in Wester Ross (e.g. Kelletat, Citation1970; Ball & Goodier, Citation1974; Goodier & Ball, Citation1975; Birse, Citation1980; Ballantyne & Morrocco, Citation2006).
Figure 26 Deflation surface at 800 m on the northern plateau of An Teallach, Wester Ross, showing the lag deposit of fine gravel amidst larger boulders. The light-coloured boulders are Cambrian quartzite erratics
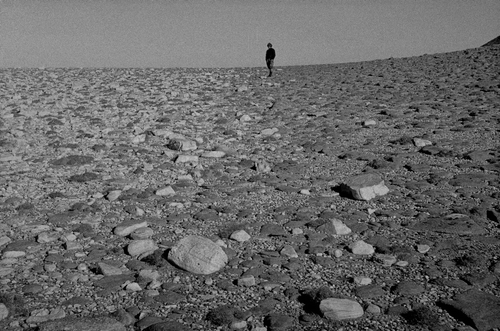
Complementing such evidence of widespread wind erosion, some mountains also support extensive aeolian deposits in the form of sand sheets, vegetation-covered expanses of windblown sediments, usually dominated by sand, that have accumulated on or at the margins of plateaux (). The thickest sand sheets often occur on lee slopes bordering exposed plateaux, and represent redeposition of windblown sediments derived from deflation surfaces upwind. Sand sheets achieve their greatest extent and thickness (up to 4 m) on Torridon Sandstone plateaux in the NW Highlands, but also occur on the Devonian sandstones of Ward Hill in Orkney, granite on Shetland and in the Cairngorms, quartzite in NW Scotland, basalts on Mull and Skye, ultrabasic rocks on Rum, and schists in the NW Highlands and Grampians.
Figure 27 Eroded vegetation-covered sand sheets at the margin of the northern plateau of An Teallach, Wester Ross. The sand at the plateau margin reaches depths of up to 4 m
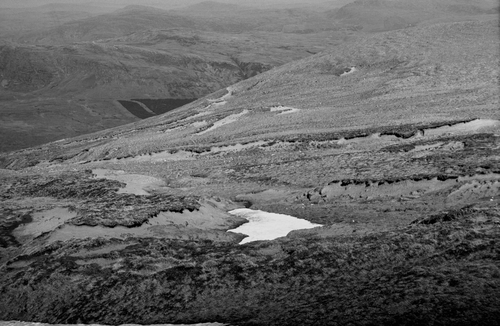
Several studies suggest that widespread stripping of vegetated covers of sand and soil from deflation surfaces is a fairly recent phenomenon. Ball & Goodier (Citation1974), for example, observed that buried soil horizons within remnant sand deposits on Ronas Hill in Shetland indicate a history of alternating accumulation and stability, with no evidence for periods of extensive erosion prior to that operating at present. On Arkle in Sutherland, accumulation of fresh aeolian deposits on lee slopes reflects recent stripping of vegetation cover and erosion of soil from exposed sites upwind (Pye & Paine, Citation1983). The stratigraphy of aeolian deposits on An Teallach (Wester Ross) also provides evidence for fairly recent widespread erosion on plateau surfaces (Ballantyne & Whittington, Citation1987; Ballantyne & Morrocco, Citation2006). On lee slopes on this mountain, sand accumulation began in the early Holocene, slowly building up to depths of up to 2.2 m at average rates of up to 18 mm per century. The subsequent deposition of an upper unit of fresh unweathered sand up to 2.3 m thick on top of the older weathered deposits on lee slopes indicates catastrophic erosion and concomitant redeposition of plateau-surface sands and aeolisols. Optically-stimulated luminescence (OSL) dating of the boundary between the lower weathered sand unit and the upper unit of fresh sand on An Teallach demonstrates that widespread erosion of stable vegetated sand deposits on the plateau commenced within the interval AD 1550–1700 (Morrocco et al., Citation2007). Though the trigger of widespread erosion of plateau deposits cannot be conclusively established, it is notable that this interval coincides with a period of marked climatic deterioration characterized by prolonged snow-lie on high ground and an increase in the frequency and ferocity of cyclonic storms (Lamb, Citation1977, Citation1979; Mitchell, Citation1999). It is not clear whether increased wind stress alone was responsible for stripping the protective vegetation mat from the plateau, opening blowouts and triggering catastrophic erosion of the plateau-surface sand deposits and soils; physiological stress induced in plant communities by prolonged or even perennial snowcover may also have contributed. Interestingly, OSL dating of lee-slope accumulations of windblown sediments on the Red Cuillin on Skye and Fionn Bheinn near Achnasheen showed that widespread wind erosion of plateau soils commenced at these sites at roughly the same time, implying that this was a widespread phenomenon in NW Scotland. At a site in the Central Grampians, however, deflation and redeposition of plateau-surface soils apparently did not commence until about AD 1900 (Morrocco et al., Citation2007).
Probably the most fascinating upland sand sheet is that on The Storr (719 m), the highest point of the Trotternish escarpment in northern Skye. A sheet of predominantly sandy, vegetation-covered aeolian sediment up to 2.9 m thick covers the summit plateau of The Storr, immediately above a steep cliff of basalt lavas exposed by a major rockslide that occurred at 6.5 ± 0.5 kyr (Ballantyne et al., Citation1998). Radiocarbon dating of soils buried beneath and within the sand sheet suggests that the onset of sand accumulation coincided with exposure of the cliff, and both the thickness of the deposit and mean grain size diminish with distance from the edge of the plateau. From this evidence, Ballantyne (Citation1998a) inferred that the plateau-top aeolian deposits on The Storr represent particles released from the cliff by weathering and blown upwards on to the plateau, where they were anchored by vegetation growth. Plateau-top aeolian sediments on some other Scottish mountains probably accumulated in a similar way.
Holocene Periglacial Processes on High Ground
Throughout the Holocene, the high plateaux and summits of the Highlands have experienced maritime periglacial conditions characterized by shallow seasonal ground freezing, extreme wetness, seasonal snowcover and very strong winds. Present mean annual temperatures exceed 0°C even on the highest summits and winter air temperatures below −10°C are infrequent. Permafrost is absent, although seasonal ground freezing may reach depths of about 0.5 m in shaded, snow-free sites (Ballantyne & Harris, Citation1994). Precipitation gradients are steep (Ballantyne, Citation1983), so that above 600 m all but the most easterly summits experience a mean annual precipitation > 2000 mm, with some westerly summits receiving > 4000 mm. During much of the twentieth century average snow-lie (>50% cover) exceeded 100 days per year at 600 m and 150–180 days at 900 m (Manley, Citation1971), though in recent years snow-lie has been less persistent (Watson et al., Citation2007). During the Little Ice Age, however, perennial snowbeds existed on some plateaux (Mitchell, Citation1999). Most periglacial features on Scottish mountains are inactive forms that developed under the much more severe climate of the Loch Lomond Stade (Ballantyne, Citation1984), but several frost action processes are active at present, and probably operated throughout the Holocene (Ballantyne, Citation1987, Citation1991a, Citation1997b; Ballantyne & Harris, Citation1994, pp. 292–297).
Bedrock Weathering
A striking contrast in the degree of bedrock weathering inside and outside the upper limits of Loch Lomond Stadial glaciers (Ballantyne, Citation1984, Citation1998b) indicates that widespread frost wedging of bedrock (macrogelifraction) has been largely inoperative since final deglaciation. Some researchers (e.g. Godard, Citation1959, Citation1965; Hills, Citation1969) have suggested that frost splitting may operate on certainly lithologies under present conditions, but there is limited evidence for this. Plateau frost debris or mountain-top detritus often supports a cover of moss, vegetation or peat that betrays its relict status. Granular disaggregation and flaking affect exposed boulders and bedrock under present conditions, but their effects are discriminatory. In terms of rounding of exposed surfaces and abundance of recent granular detritus, sandstones, granites and granulites appear most susceptible to superficial frost weathering or microgelifraction (Ballantyne, Citation1998b; ). Fine-grained igneous or metamorphic rocks such as basalt or quartzite are characteristically angular or subangular, suggesting that they have been relatively immune to granular disaggregation. Fresh surfaces on rocks with marked bedding, foliation or cleavage indicate recent small-scale flaking. However, on all lithologies there is a remarkable contrast in rounding between exposed and buried clast surfaces. This demonstrates that the effects of granular disaggregation are limited to exposed surfaces, so that the process is constrained by the cover of soil, peat and vegetation that mantles most high ground. The widespread presence of aeolian deposits and aeolisols (soils derived from windblown sediment) on high plateaux suggests that granular disaggregation of bedrock and exposed clasts has persisted throughout the Holocene.
Frost-Sorted Patterned Ground
Frost-sorted patterned ground is terrain that exhibits surface patterns (circles, nets, polygons and stripes) defined by the alternation of fine and coarse debris. Such patterns develop on unvegetated soil through differential mass displacement of fine soil and clasts during freezing and/or thawing of the ground (Ballantyne, Citation2007c). High plateaux mantled by bouldery regolith often support large (>1 m diameter) sorted nets, circles and stripes produced by deep seasonal ground freezing, but such forms are usually vegetated and clearly inactive, and have been interpreted as the product of severe periglacial conditions during the Loch Lomond Stade or earlier (Ballantyne, Citation1984). Active sorted patterns rarely exceed 0.7 m in width, and sorting is confined to the uppermost 0.2 m of soil (e.g. Simpson, Citation1932; Kelletat, Citation1970; Ryder & McCann, Citation1971; Ballantyne, Citation1987, Citation1997b; ). They are confined to unvegetated terrain characterized by small clasts (<15 cm long) embedded in fine-grained frost-susceptible soil that develops needle-ice during nocturnal freezing, or subsurface ice lenses during longer periods of ground freezing. Active patterned ground is therefore limited to bare soil underlain by rocks that have weathered to produce small clasts and silt-rich soil, such as basalts and some schists and quartzites; it is rare on granite and absent on sandstones where the soil matrix is too coarse. Little research has been carried out on ground patterning in the Highlands, but well-developed sorted stripes at 640 m on Tinto Hill in southern Scotland have been shown to re-form through needle-ice action over one to three winters (Ballantyne, Citation2001a). Remarkably, climate seems to impose little constraint on altitude of occurrence: sorted stripes occur on till bluffs at 230 m on Skye, and sorted nets have been successfully ‘grown’ in unvegetated felsite regolith near sea level over a single winter (Ballantyne, Citation1996).
Solifluction
The term ‘solifluction’ describes the gradual downslope movement of soil as a result of seasonal freezing and thawing. Solifluction is restricted to frost-susceptible soils in which pore spaces are sufficiently small to allow ice lenses to form during freezing. Two processes are involved: frost creep, the ratchet-like downslope movement of soil due to expansion during freezing and contraction during thaw, and gelifluction, the localised failure and flow of supersaturated soil due to build-up of porewater pressures during thaw. Solifluction is presently the dominant mechanism of mass movement on many Scottish mountains above about 550–600 m. Its effects are widespread, particularly on vegetated soils derived from schists. The most widespread solifluction landforms are smooth sheets of seasonally-mobile soil that terminate downslope at a steep step or riser 0.2–1.0 m high on upper slopes, sometimes giving a ‘staircase’ appearance to higher slopes (). As gradient increases downslope, the plan form of risers becomes crenulate or lobate in response to across-slope variations in rates of soil movement, forming lobate solifluction sheets. On some slopes, lobate sheets over ride one another, isolating individual solifluction lobes from their neighbours.
Figure 30 Active solifluction sheets in the North-West Highlands. The steep fronts (risers) are characteristic of present activity
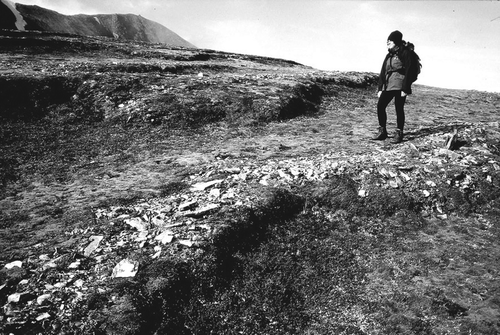
Although many solifluction sheets and lobes are relics of formerly more severe ground freezing, active Holocene solifluction landforms are widespread above 550 m (Kelletat, Citation1970). Active forms differ from their relict counterparts in that risers are generally lower (<1 m high) and steeper (> 45°), and sometimes bulge downslope (). Moreover, solifluction forms that have been active during the Holocene often overlie organic soil horizons or peat remnants that have been buried under advancing sheets and lobes. Radiocarbon dating of organic matter buried by the downslope movement of small solifluction lobes demonstrates intermittent movement over at least the last 5500 years (Sugden, Citation1971; Mottershead, Citation1978). Recent movement of a lobe in the Fannich Mountains is indicated by five radiocarbon ages of 0.9–0.5 kyr yr obtained from a soil horizon buried by lobe advance. As these dates are statistically indistinguishable from the radiocarbon age of the soil horizon itself, they imply rapid, recent frontal advance of the lobe over three metres at a rate of at least 22 mm yr−1 (Ballantyne, Citation1986b). In the Drumochter Hills, Chattopadhyay (Citation1982) measured 50 mm of surface displacement over three winters, declining to zero at 20 cm depth.
Closely associated with active solifluction sheets and lobes are ploughing boulders, which have moved downslope faster than the surrounding soil, pushing up a ridge downslope and leaving a vegetated furrow in the soil upslope (). Most ploughing boulders occur on vegetated upper slopes with gradients of 8–34° and are aligned downslope due to rotation during movement. Activity is indicated by a deep niche a few centimetres wide that extends to the base of the boulder at its upslope end. Average measured rates of recent boulder movement range from 1.5–7.0 mm yr−1 on the Drumochter Hills and 0.3–8.7 mm yr−1 on Lochnagar to 3.6–30.3 mm yr−1 on the Fannich Mountains (Ballantyne, Citation2001b). Downslope movement of boulders at rates faster than that of the surrounding soil appears to result from differences in thermal diffusivity. These promote ice lens formation under boulders during freezing and high porewater pressures under boulders during thaw, allowing boulders to slide downslope on a bed of liquefied soil (Ballantyne, Citation2001b).
Conclusions
Since this topic was last reviewed (Ballantyne, Citation1991a), there have been some remarkable advances in our understanding of how the landscape of the Scottish Highlands has changed during the Holocene Epoch. The usefulness of paraglacial landscape modification as a model for change, first identified in the earlier review, has become widely accepted in studies of rock-slope instability and reworking of drift-mantled slopes, but remains to be established for fluvial systems. Moreover, the paraglacial model provides a general explanation for the fact that most Holocene sediment stores (talus accumulations, debris cones, alluvial fans and alluvial valley fills) are now relict landforms on which net erosion has succeeded net accumulation. Greatly improved dating control has shown that almost all forms of geomorphic activity identified above have persisted or occurred intermittently throughout much or all of the Holocene, and emphasized the probable role of extreme climatic events in triggering episodes of enhanced erosion or sediment accumulation.
Despite these advances, there are still major gaps in our understanding of Holocene geomorphic activity in the Scottish Highlands. The nature, causes and timing of major rock-slope failures (particularly slope deformations) are still incompletely understood, and our understanding of Holocene alluvial history prior to about AD 1800 is based on a handful of widely-separated dated sites of uncertain representativeness. Moreover, much research on Holocene landscape evolution in Scotland has tended to be essentially inductive and based on serendipitious discovery of datable exposures in talus accumulations, debris cones, alluvial fans, river terraces and aeolian deposits. In many cases, no coherent, consistent and verifiable regional chronology has emerged, despite the ingenuity of some researchers in attempting to correlate dates from one site with those from others or with climatic shifts of uncertain timing or validity. If we are to further our understanding of the causes and chronology of Holocene environmental change we must adopt a hypothesis-driven approach. The paraglacial model offers one such general hypothesis; the notion that many landform-generating events are due to exceptional rainstorms of random occurrence offers another; recent accelerated activity due to human interference represents a third. None of these are mutually exclusive, and all are potentially testable. Despite all the recent accomplishments documented above, much yet has to be learned about landscape change in Scotland after the disappearance of glacier ice.
Acknowledgements
The author is grateful to numerous friends, colleagues, students and research collaborators for their stimulating insights and collaboration in the field during 30 years of research on Holocene landscape evolution in Scotland. Particular thanks are due to five former PhD students, Professor Doug Benn, Dr Vanessa Brazier, Dr Alastair Curry, Dr Simon Hinchliffe and Dr Stefan Morrocco. Grateful thanks are due to the editor, Dr Jim Hansom, for encouragement to undertake this review, to Graeme Sandeman for drafting the figures and maps, and to Professor Bob Crawford, David Jarman and Dr Peter Wilson for providing photographs. The support of NERC and the Carnegie Trust for the Universities of Scotland are also acknowledged for funding research on Holocene landscape evolution over many years, the NERC radiocarbon dating facility (East Kilbride) for funding and provision of radiocarbon analyses, Dr John Stone (University of Washington) for analyses of cosmogenic isotopes, Dr Ruth Robinson (St Andrews) for overseeing OSL analyses of windblown sand deposits and Professor Graeme Whittington (St Andrews) for undertaking pollen analyses of peat deposits and buried soils on my behalf. Thanks are also due to Professor John Gordon and Dr David Evans for painstaking and constructive reviews.
References
- Anderson , D. E. 1998 . A reconstruction of Holocene climate changes from peat bogs in north-west Scotland . Boreas , 27 : 208 – 224 .
- Anderson , D. E. , Binney , H. A. and Smith , M. A. 1998 . Evidence for abrupt climatic change in northern Scotland between 3500 and 3900 calendar years BP . The Holocene , 8 : 97 – 103 .
- Anderson , F. W. and Dunham , K. W. 1966 . The Geology of Northern Skye , Edinburgh : HMSO . Memoir of the Geological Survey
- Atkinson , T. C. , Briffa , K. R. and Coope , G. R. 1987 . Seasonal temperatures in Britain during the past 22,000 years reconstructed using beetle remains . Nature , 325 : 587 – 592 .
- Augustinus , P. C. 1995 . Glacial valley cross-profile development: the influence of in situ rock stress and rock mass strength, with examples from the Southern Alps, New Zealand . Geomorphology , 14 : 87 – 97 .
- Baird , P. D. and Lewis , W. V. 1957 . The Cairngorm floods: summer solifluction and distributary formation . Scottish Geographical Magazine , 73 : 91 – 100 .
- Ball , D. F. and Goodier , R. 1974 . “ Ronas Hill, Shetland: a preliminary account of its ground pattern features resulting from the action of frost and wind ” . In The Natural Environment of Shetland , Edited by: Goodier , R. 89 – 106 . Edinburgh : Nature Conservancy Council .
- Ballantyne , C. K. 1983 . Precipitation gradients in Wester Ross . Weather , 38 : 379 – 387 .
- Ballantyne , C. K. 1984 . The Late Devensian periglaciation of upland Britain . Quaternary Science Reviews , 3 : 311 – 343 .
- Ballantyne , C. K. 1986a . Landslides and slope failures in Scotland: a review . Scottish Geographical Magazine , 102 : 134 – 150 .
- Ballantyne , C. K. 1986b . Late Flandrian solifluction on the Fannich Mountains, Ross-shire . Scottish Journal of Geology , 22 : 395 – 406 .
- Ballantyne , C. K. 1987 . “ The present-day periglaciation of upland Britain ” . In Periglacial Landforms and Processes in Britain and Ireland , Edited by: Boardman , J. 113 – 126 . Cambridge : Cambridge University Press .
- Ballantyne , C. K. 1989 . Avalanche impact landforms on Ben Nevis, Scotland . Scottish Geographical Magazine , 105 : 38 – 42 .
- Ballantyne , C. K. 1991a . Holocene geomorphic activity in the Scottish Highlands . Scottish Geographical Magazine , 107 : 84 – 98 .
- Ballantyne , C. K. 1991b . The landslides of Trotternish, Isle of Skye . Scottish Geographical Magazine , 107 : 130 – 135 .
- Ballantyne , C. K. 1991c . Late Holocene erosion in upland Britain: climatic deterioration or human influence . The Holocene , 1 : 81 – 85 .
- Ballantyne , C. K. 1992 . Rock-slope failure and debris flow, Gleann na Guiserein, Knoydart . Scottish Journal of Geology , 28 : 77 – 80 .
- Ballantyne , C. K. 1995 . Paraglacial debris cone formation on recently-deglaciated terrain . The Holocene , 5 : 25 – 33 .
- Ballantyne , C. K. 1996 . Formation of miniature sorted patterns by shallow ground freezing: a field experiment . Permafrost and Periglacial Processes , 7 : 409 – 424 .
- Ballantyne , C. K. 1997a . Holocene rock slope failures in the Scottish Highlands . Paläoklimaforschung , 19 : 197 – 205 .
- Ballantyne , C. K. 1997b . “ The periglacial geomorphology of Scotland ” . In Reflections on the Ice Age In Scotland , Edited by: Gordon , J. E. 166 – 178 . Glasgow : Scottish Natural Heritage .
- Ballantyne , C. K. 1998a . Aeolian deposits on a Scottish mountain summit: characteristics, provenance, history and significance . Earth Surface Processes and Landforms , 23 : 625 – 641 .
- Ballantyne , C. K. 1998b . Age and significance of mountain-top detritus . Permafrost and Periglacial Processes , 9 : 327 – 345 .
- Ballantyne , C. K. 2001a . The sorted stone stripes of Tinto Hill . Scottish Geographical Journal , 117 : 313 – 324 .
- Ballantyne , C. K. 2001b . Measurement and theory of ploughing boulder movement . Permafrost and Periglacial Processes , 12 : 267 – 288 .
- Ballantyne , C. K. 2002a . Paraglacial geomorphology . Quaternary Science Reviews , 21 : 1935 – 2017 .
- Ballantyne , C. K. 2002b . A general model of paraglacial landscape response . The Holocene , 12 : 371 – 376 .
- Ballantyne , C. K. 2002c . The Loch Lomond Readvance on the Isle of Mull, Scotland: glacier reconstruction and palaeoclimatic implications . Journal of Quaternary Science , 17 : 759 – 771 .
- Ballantyne , C. K. 2002d . Debris flow activity in the Scottish Highlands: temporal trends and wider implications for dating . Studia Geomorphologica Carpatho-Balcanica , 36 : 7 – 27 .
- Ballantyne , C. K. 2003a . Paraglacial landform succession and sediment storage in deglaciated mountain valleys: theory and approaches to calibration . Zeitschrift für Geomorphologie, Supplementband , 132 : 1 – 18 .
- Ballantyne , C. K. 2003b . A Scottish Sturzstrom: the Beinn Alligin rock avalanche, Wester Ross . Scottish Geographical Journal , 119 : 159 – 167 .
- Ballantyne , C. K. 2004 . “ After the ice: paraglacial and postglacial evolution of the physical environment of Scotland, 20,000 to 5000 BP ” . In Mesolithic Scotland: The Early Holocene Prehistory of Scotland and its European Context , Edited by: Saville , A. Edinburgh : Society of Antiquaries of Scotland, Monograph Series .
- Ballantyne , C. K. 2007a . “ Paraglacial geomorphology ” . In Encyclopedia of Quaternary Science , Edited by: Elias , S. 2170 – 2182 . Rotterdam : Elsevier .
- Ballantyne , C. K. 2007b . Loch Lomond Stadial glaciers in North Harris, NW Scotland: glacier reconstruction and palaeoclimatic implications . Quaternary Science Reviews , 26 : 3134 – 3149 .
- Ballantyne , C. K. 2007c . “ Patterned ground ” . In Encyclopedia of Quaternary Science , Edited by: Elias , S. 2182 – 2191 . Rotterdam : Elsevier .
- Ballantyne , C. K. 2007d . “ Coire Gabhail, Highland ” . In Mass Movements in Great Britain , Edited by: Cooper , R. G. 132 – 136 . Peterborough : Joint Nature Conservation Committee . Geological Conservation Review Series No. 33
- Ballantyne , C. K. 2007e . “ Carn Dubh, Ben Gulabin, Perthshire ” . In Mass Movements in Great Britain , Edited by: Cooper , R. G. 107 – 111 . Peterborough : Joint Nature Conservation Committee . Geological Conservation Review Series No. 33
- Ballantyne , C. K. 2007f . “ Trotternish Escarpment, Isle of Skye, Highland ” . In Mass Movements in Great Britain , Edited by: Cooper , R. G. 196 – 204 . Peterborough : Joint Nature Conservation Committee . Geological Conservation Review Series No. 33
- Ballantyne , C. K. and Benn , D. I. 1994 . Paraglacial slope adjustment and resedimentation in response to recent glacier retreat, Fåbergst⊘lsbreen, Norway . Arctic and Alpine Research , 26 : 255 – 269 .
- Ballantyne , C. K. and Benn , D. I. 1996 . “ Paraglacial slope adjustment during recent deglaciation and its implications for slope evolution in formerly glaciated environments ” . In Advances in Hillslope Processes , Edited by: Brooks , S. and Anderson , M. G. 1173 – 1195 . Chichester : Wiley .
- Ballantyne , C. K. and Eckford , J. D. 1984 . Characteristics and evolution of two relict talus slopes in Scotland . Scottish Geographical Magazine , 100 : 20 – 33 .
- Ballantyne , C. K. and Harris , C. 1994 . The Periglaciation of Great Britain , Cambridge : Cambridge University Press .
- Ballantyne , C. K. and Kirkbride , M. P. 1987 . Rockfall activity in upland Britain during the Loch Lomond Stadial . Geographical Journal , 153 : 86 – 92 .
- Ballantyne , C. K. and Morrocco , S. M. 2006 . The windblown sands of An Teallach . Scottish Geographical Journal , 122 : 149 – 159 .
- Ballantyne , C. K. and Stone , J. O. 2004 . The Beinn Alligin rock avalanche, NW Scotland: cosmogenic 10Be dating, interpretation and significance . The Holocene , 14 : 461 – 466 .
- Ballantyne , C. K. and Whittington , G. 1987 . Niveo-aeolian sand deposits on An Teallach, Wester Ross, Scotland . Transactions of the Royal Society of Edinburgh: Earth Science , 78 : 51 – 63 .
- Ballantyne , C. K. and Whittington , G. W. 1999 . Late Holocene alluvial fan formation and floodplain incision, Central Grampian Highlands, Scotland . Journal of Quaternary Science , 14 : 651 – 671 .
- Ballantyne , C. K. , Stone , J. O. and Fifield , L. K. 1998 . Cosmogenic Cl-36 dating of postglacial landsliding at the Storr, Isle of Skye, Scotland . The Holocene , 8 : 347 – 351 .
- Ballantyne , C. K. , Schnabel , C. and Xu , S. 2008 . “ Exposure dating and reinterpretation of coarse debris accumulations (‘rock glaciers’) in the Cairngorm Mountains, Scotland ” . In Journal of Quaternary Science , Vol. 23 , (in press) .
- Barnett , C. , Hossell , J. , Perry , M. , Proctor , C. and Hughes , G. 2006 . A Handbook of Climate Trends across Scotland SNIFFER project CC03, Scotland and Northern Ireland Forum for Environmental Research, 62 pp. Available at http://www.sniffer.org.uk/climatehandbook/index.html
- Bayfield , N. G. 1984 . The dynamics of heather (Calluna vulgaris) stripes in the Cairngorm Mountains, Scotland . Journal of Ecology , 72 : 515 – 527 .
- Benn , D. I. 1992 . The genesis and significance of ‘hummocky moraine’: evidence from the Isle of Skye, Scotland . Quaternary Science Reviews , 11 : 781 – 779 .
- Benn , D. I. and Ballantyne , C. K. 2005 . Palaeoclimatic inferences from reconstructed Loch Lomond Stadial glaciers . Journal of Quaternary Science , 20 : 577 – 592 .
- Bennett , M. R. 1999 . Paraglacial and periglacial slope adjustment of a degraded lateral moraine in Glen Torridon . Scottish Journal of Geology , 35 : 79 – 83 .
- Birks , H. J. B. 1996 . “ Great Britain—Scotland ” . In Palaeoecological Events During the Last 15,000 Years: Regional Syntheses of Palaeoecological Studies of Lakes and Mires in Europe , Edited by: Berglund , B. E. , Birks , H. J. B. , Ralska-Jasiewiczowa , M. and Wright , H. E. 95 – 143 . Chichester : Wiley .
- Birse , E. L. 1980 . Suggested amendments to the world soil classification to accommodate Scottish aeolian and mountain soils . Journal of Soil Science , 31 : 117 – 124 .
- Black , A. R. 1995 . Flood seasonality and physical controls in flood risk estimation . Scottish Geographical Magazine , 111 : 187 – 190 .
- Black , A. R. 1996 . Major flooding and increased flood frequency in Scotland since 1988 . Physics and Chemistry of the Earth , 20 : 463 – 468 .
- Black , A. R. and Burns , J. C. 2002 . Re-assessing the flood risk in Scotland . The Science of the Total Environment , 294 : 169 – 184 .
- Blundell , A. and Barber , K. E. 2005 . A 2800-year palaeoclimatic record from Tore Hill Moss, Strathspey, Scotland: the need for a multi-proxy approach to peat-based climatic reconstructions . Quaternary Science Reviews , 24 : 1261 – 1277 .
- Bovis , M. J. and Dagg , B. R. 1992 . Debris flow triggering by impulsive loading: mechanical modeling and case studies . Canadian Geotechnical Journal , 29 : 345 – 352 .
- Brazier , V. and Ballantyne , C. K. 1989 . Late Holocene debris cone evolution in Glen Feshie, western Cairngorm Mountains, Scotland . Transactions of the Royal Society of Edinburgh: Earth Sciences , 80 : 17 – 24 .
- Brazier , V. , Whittington , G. W. and Ballantyne , C. K. 1988 . Holocene debris cone evolution in Glen Etive, western Grampian Highlands, Scotland . Earth Surface Processes and Landforms , 13 : 525 – 531 .
- Bridge , M. C. , Haggart , B. A. and Lowe , J. J. 1990 . The history and palaeoclimatic significance of subfossil remains of Pinus silvestris in blanket peats from Scotland . Journal of Ecology , 78 : 77 – 99 .
- Briffa , K. R. and Atkinson , T. C. 1997 . “ Reconstructing Late-glacial and Holocene climates ” . In Climates of the British Isles: Present, Past and Future , Edited by: Hulme , M. and Barrow , E. 84 – 111 . London : Routledge .
- Brooks , S. J. and Birks , H. J. B. 2000 . Chironomid-inferred Late-glacial air temperatures at Whitrig Bog, southeast Scotland . Journal of Quaternary Science , 15 : 759 – 764 .
- Brooks , S. M. , Anderson , M. G. and Collinson , A. J. C. 1995 . Modelling the role of climate, vegetation and pedogenesis in shallow translational hillslope failure . Earth Surface Processes and Landforms , 20 : 231 – 242 .
- Chattopadhyay , G. P. 1982 . “ Periglacial geomorphology of part of the Grampian Highlands of Scotland ” . Unpublished PhD Thesis, University of Edinburgh
- Christiansen , H. H. 2004 . Windpolished boulders and bedrock in the Scottish Highlands: evidence and implications of Late Devensian wind activity . Boreas , 33 : 82 – 94 .
- Church , M. and Ryder , J. M. 1972 . Paraglacial sedimentation: a consideration of fluvial processes conditioned by glaciation . Bulletin of the Geological Society of America , 83 : 3059 – 3067 .
- Common , R. 1954 . A report on the Lochaber, Appin and Benderloch floods, May 1953 . Scottish Geographical Magazine , 70 : 6 – 20 .
- Crawford , R. M. M. 2008 . Plants at the Margin , Cambridge : Cambridge University Press .
- Cruden , D. M. and Hu , X. Q. 1993 . Exhaustion and steady-state models for predicting landslide hazards in the Canadian Rocky Mountains . Geomorphology , 8 : 279 – 285 .
- Curry , A. M. 1999a . “ Paraglacial modification of drift-mantled hillslopes ” . Unpublished PhD thesis, University of St Andrews
- Curry , A. M. 1999b . Paraglacial modification of slope form . Earth Surface Processes and Landforms , 24 : 1213 – 1228 .
- Curry , A. M. 2000a . Holocene reworking of drift-mantled hillslopes in the Scottish Highlands . Journal of Quaternary Science , 15 : 529 – 541 .
- Curry , A. M. 2000b . Holocene reworking of drift-mantled hillslopes in Glen Docherty, Northwest Highlands, Scotland . The Holocene , 10 : 509 – 518 .
- Dubois , A. D. and Ferguson , D. K. 1985 . The climatic history of pine in the Cairngorms based on radiocarbon dates and stable isotope analysis, with an account of the events leading up to its colonization . Review of Palaeobotany and Palynology , 46 : 55 – 80 .
- Fenton , C. H. 1992 . Neotectonics in NW Scotland: a Field Guide , Glasgow : University of Glasgow .
- Ferguson , R. I. and Ashworth , P. 1990 . Slope-induced changes in channel character along a gravel-bed stream: the Allt Dubhaig, Scotland . Earth Surface Processes and Landforms , 16 : 65 – 82 .
- Ferguson , R. I. and Werritty , A. 1983 . “ Bar development and channel changes in the gravelly River Feshie ” . In Modern and Ancient Fluvial Systems Edited by: Collinson , J. D. and Lewin , J. 181 – 193 . International Association of Sedimentologists, Special Publication 6
- Gilbertson , D. D. , Schwenninger , J.-L. , Kemp , R. A. and Rhodes , E. J. 1999 . Sand drift and soil formation along an exposed north Atlantic coastline: 14,000 years of diverse geomorphological, climatic and human impacts . Journal of Archaeological Science , 26 : 439 – 469 .
- Godard , A. 1959 . Contemporary periglacial phenomena in western Scotland . Scottish Geographical Magazine , 75 : 55
- Godard , A. 1965 . Recherches de Géomorphologie en Écosse du Nord-Ouest , Paris : Masson .
- Goodier , R. and Ball , D. F. 1975 . “ Ward Hill, Orkney: patterned ground features and their origins ” . In The Natural Environment of Orkney , Edited by: Goodier , R. 47 – 56 . Edinburgh : Nature Conservancy Council .
- Hansom , J. D. and Angus , S. 2005 . Machair nan Eilean Siar (Machair of the Western Isles) . Scottish Geographical Journal , 121 : 401 – 412 .
- Hills , R. C. 1969 . Comparative weathering of granite and quartzite in a periglacial environment . Geografiska Annaler , 51A : 46 – 47 .
- Hinchliffe , S. 1999 . Timing and significance of talus slope reworking, Trotternish, Isle of Skye, Scotland . The Holocene , 9 : 483 – 494 .
- Hinchliffe , S. and Ballantyne , C. K. 1999 . Talus accumulation and rockwall retreat, Trotternish, Isle of Skye, Scotland . Scottish Geographical Journal , 115 : 53 – 70 .
- Hinchliffe , S. , Ballantyne , C. K. and Walden , J. 1998 . The structure and sedimentology of relict talus, Trotternish, northern Skye, Scotland . Earth Surface Processes and Landforms , 23 : 545 – 560 .
- Huntley , B. 1999 . “ Climatic change and reconstruction ” . In Holocene Environments of Prehistoric Britain (Quaternary Proceedings, 7) , Edited by: Edwards , K. J. and Sadler , J. P. 513 – 520 . Chichester : Wiley .
- Huntley , B. and Prentice , I. C. 1993 . “ Holocene vegetation and climates in Europe ” . In Global Climates since the Last Glacial Maximum , Edited by: Wright , H. E. , Kutzbach , J. E. , Webb , T. , Ruddiman , W. F. , Street-Perrott , F. A. and Bartlein , P. J. 136 – 168 . Minneapolis : University of Minneapolis Press .
- Innes , J. L. 1983a . Lichenometric dating of debris-flow deposits in the Scottish Highlands . Earth Surface Processes and Landforms , 8 : 579 – 588 .
- Innes , J. L. 1983b . Debris flows . Progress in Physical Geography , 7 : 469 – 501 .
- Innes , J. L. 1985 . Magnitude-frequency relations of debris flows in northwest Europe . Geografiska Annaler , 67A : 23 – 32 .
- Innes , J. L. 1986 . Textural properties of regoliths on vegetated steep slopes in upland regions . Transactions of the Royal Society of Edinburgh: Earth Sciences , 77 : 241 – 250 .
- Jarman , D. 2006 . Large rock slope failures in the Highlands of Scotland: characterisation, causes and spatial distribution . Engineering Geology , 83 : 161 – 182 .
- Jarman , D. 2007a . “ Introduction to the mass movements in the older mountain areas of Great Britain ” . In Mass Movements in Great Britain , Edited by: Cooper , R. G. 33 – 56 . Peterborough : Joint Nature Conservation Committee . Geological Conservation Review Series No. 33
- Jarman , D. 2007b . “ Glen Ample, Stirling ” . In Mass Movements in Great Britain , Edited by: Cooper , R. G. 82 – 91 . Peterborough : Joint Nature Conservation Committee . Geological Conservation Review Series No. 33
- Jarman , D. and Ballantyne , C. K. 2002 . Beinn Fhada, Kintail: an example of large-scale paraglacial rock slope deformation . Scottish Geographical Journal , 118 : 59 – 68 .
- Jenkins , A. , Ashworth , P. J. , Ferguson , R. I. , Grieve , I. C. , Rowling , P. and Stott , T. A. 1988 . Slope failures in the Ochil Hills, Scotland, November 1984 . Earth Surface Processes and Landforms , 13 : 69 – 76 .
- Kelletat , D. 1970 . Rezente Periglazialerscheinungen im Schottischen Hochland . Göttinger Geographische Abhandlungen , 51 : 67 – 140 .
- Klitgaard-Kristensen , D. , Sejrup , H. P. , Haflidason , H. , Johnsen , S. and Spurk , M. 1998 . A regional 8200 cal yr BP cooling event in northwest Europe, induced by the final stages of the Laurentide ice-sheet deglaciation . Journal of Quaternary Science , 13 : 165 – 169 .
- Kotarba , A. 1984 . Slope features in areas of high relief in maritime climate (with the Island of Rhum as example) . Studia Geomorphologica Carpatho-Balcanica , 17 : 77 – 88 .
- Lamb , H. H. 1977 . Climate: Past, Present and Future. Volume 2: Climatic History and the Future , London : Methuen .
- Lamb , H. H. 1979 . Climatic variation and changes in the wind and ocean circulation: the Little Ice Age in the Northeast Atlantic . Quaternary Research , 11 : 1 – 20 .
- Lamb , H. H. 1984 . “ Some studies of the Little Ice Age of recent centuries and its great storms ” . In Climatic Changes on a Yearly to Millennial Basis , Edited by: Morner , N. A. and Karlén , W. 291 – 309 . Dordrecht : Reidel .
- Lamb , H. H. 1991 . Historic Storms of the North Sea, British Isles and Northwest Europe , Cambridge : Cambridge University Press .
- Langdon , D. G. and Barber , K. E. 2005 . The climate of Scotland over the last 5000 years inferred from multiproxy peatland records: inter-site correlations and regional variability . Journal of Quaternary Science , 20 : 549 – 566 .
- Lewin , J. , Macklin , M. G. and Johnstone , E. 2005 . Interpreting alluvial archives: sedimentological factors in the British Holocene alluvial record . Quaternary Science Reviews , 24 : 1873 – 1889 .
- Luckman , B. H. 1992 . Debris flows and snow avalanche landforms in the Lairig Ghru, Cairngorm Mountains, Scotland . Geografiska Annaler , 74A : 109 – 121 .
- Macklin , M. G. and Lewin , J. 2003 . River sediments, great floods and centennial-scale Holocene climate change . Journal of Quaternary Science , 18 : 101 – 105 .
- Maizels , J. K. 1988 . “ Sediment size and channel changes in braided and meandering gravel bed streams, upper Deeside, Scotland ” . In International Conference on River Regime , Edited by: White , W. R. 215 – 230 . Chichester : Wiley .
- Maizels , J. K. and Aitken , J. 1991 . “ Palaeohydrological change during deglaciation in upland Britain: a case study from northeast Scotland ” . In Temperate Palaeohydrology: Fluvial Processes in the Temperate Zone During the Last 15,000 years , Edited by: Starkel , L. , Gregory , K. J. and Thornes , J. B. 105 – 145 . Chichester : Wiley .
- Manley , G. 1971 . Scotland's semi-permanent snows . Weather , 26 : 458 – 471 .
- McEwen , L. J. 1989 . “ River channel changes in response to flooding in the upper River Dee catchment, Aberdeenshire, over the last 200 years ” . In Floods: Hydrological, Sedimentological and Geomorphological Implications , Edited by: Beven , K. and Carling , P. 219 – 238 . Chichester : Wiley .
- McEwen , L. J. 1994 . Channel pattern adjustment and streampower variations on the middle River Coe, Western Grampian Highlands . Catena , 21 : 357 – 374 .
- McEwen , L. J. 1997 . “ Geomorphological change and fluvial landscape evolution during the Holocene ” . In Reflections on the Ice Age in Scotland , Edited by: Gordon , J. E. 116 – 129 . Glasgow : Scottish Association of Geography Teachers and Scottish Natural Heritage .
- McEwen , L. J. and Werritty , A. 1988 . The hydrology and long-term geomorphic significance of a flash flood in the Cairngorm Mountains, Scotland . Catena , 21 : 357 – 374 .
- Mitchell , I. 1999 . Scotland's Mountains before the Mountaineers , Edinburgh : Luath Press .
- Morrocco , S. M. , Ballantyne , C. K. , Spencer , J. Q. and Robinson , R. A. J. 2007 . Age and significance of aeolian sediment reworking on high plateaux in the Scottish Highlands . The Holocene , 17 : 349 – 360 .
- Mottershead , D. N. 1978 . High-altitude solifluction and postglacial vegetation, Arkle, Sutherland . Transactions of the Royal Society of Edinburgh , 43 : 17 – 24 .
- Peacock , J. D. 1986 . Alluvial fans and an outwash fan in upper Glen Roy, Lochaber . Scottish Journal of Geology , 22 : 347 – 366 .
- Pye , K. and Paine , A. D. M. 1983 . Nature and source of aeolian deposits near the summit of Ben Arkle, Northwest Scotland . Geologie en Mijnbouw , 68 : 13 – 18 .
- Reid , E. and Thomas , M. F. 2006 . A chronostratigraphy of mid- and late-Holocene slope evolution: Creagan a'Chaorainn, Northern Highlands, Scotland . The Holocene , 16 : 429 – 444 .
- Reid , E. , Thomas , M. F. and Tipping , R. M. 2003 . “ Holocene alluvial fan and fluvial activity in upper Gleann Lichd, Kintail ” . In The Quaternary of Glen Affric and Kintail: Field Guide , Edited by: Tipping , R. M. 57 – 164 . London : Quaternary Research Association .
- Ritchie , W. 1972 . The evolution of coastal sand dunes . Scottish Geographical Magazine , 88 : 19 – 35 .
- Ritchie , W. 1979 . Machair development and chronology in the Uists and adjacent islands . Proceedings of the Royal Society of Edinburgh , 77B : 107 – 122 .
- Ritchie , W. and Whittington , G. 1994 . Non-synchronous aeolian sand movements in the Uists: the evidence of the intertidal organic and sand deposits at Cladach Mór, North Uist . Scottish Geographical Magazine , 110 : 40 – 46 .
- Ritchie , W. , Whittington , G. and Edwards , K. J. 2001 . Holocene changes in the physiography and vegetation of the Atlantic littoral of the Uists, Outer Hebrides, Scotland . Transactions of the Royal Society of Edinburgh , 92 : 121 – 136 .
- Robertson-Rintoul , M. and Ritchie , W. 1990 . The geomorphology of coastal sand dunes in Scotland: a review . Catena Supplement , 18 : 41 – 49 .
- Robertson-Rintoul , M. S. E. 1986 . A quantitative soil-stratigraphic approach to the correlation and dating of post-glacial river terraces in Glen Feshie, Western Cairngorms . Earth surface Processes and Landforms , 11 : 605 – 617 .
- Ryder , J. M. 1971 . The stratigraphy and morphology of some paraglacial alluvial fans in south-central British Columbia . Canadian Journal of Earth Sciences , 8 : 279 – 298 .
- Ryder , R. H. and McCann , S. B. 1971 . Periglacial phenomena in the Isle of Rhum in the Inner Hebrides . Scottish Journal of Geology , 7 : 293 – 303 .
- Salt , K. E. and Ballantyne , C. K. 1997 . The structure and sedimentology of relict talus, Knockan, Assynt . Scottish Geographical Magazine , 113 : 82 – 89 .
- Simpson , J. B. 1932 . Stone polygons on Scottish mountains . Scottish Geographical Magazine , 48 : 37
- Sissons , J. B. and Cornish , R. 1982 . Differential isostatic uplift of crustal blocks at Glen Roy, Scotland . Quaternary Research , 18 : 268 – 288 .
- Smith , K. 1995 . Precipitation over Scotland, 1757–1992: some aspects of temporal variability . International Journal of Climatology , 15 : 543 – 556 .
- Smith , K. and Bennett , A. M. 1994 . Recently-increased river discharge in Scotland: effects on flow hydrology and some implications for water management . Applied Geography , 14 : 123 – 133 .
- Stewart , I. S. , Saber , S. and Rose , J. 2000 . Glacio-seismotectonics: ice sheets, crustal deformation and palaeoseismicity . Quaternary Science Reviews , 19 : 1367 – 1389 .
- Stuiver , M. , Reimer , P. J. and Braziunas , T. F. 1998 . High precision radiocarbon age calibration for terrestrial and marine samples . Radiocarbon , 40 : 1127 – 1151 .
- Sugden , D. E. 1971 . The significance of periglacial activity on some Scottish mountains . Geographical Journal , 137 : 388 – 392 .
- Tisdall , E. 2003 . “ West Glen Affric: peat-stratigraphic data on Holocene climate change—humification patterns and the identification of a long-term temperature record ” . In The Quaternary of Glen Affric and Kintail: Field Guide , Edited by: Tipping , R. M. 55 – 62 . London : Quaternary Research Association .
- Van Steijn , H. , de Ruig , J. and Hoozemans , F. 1988 . Morphological and mechanical aspects of debris flow in parts of the French Alps . Zeitschrift für Geomorphologie , 32 : 143 – 161 .
- Walker , M. J. C. 2005 . Quaternary Dating Methods , Chichester : Wiley .
- Ward , R. G. W. 1985 . Geomorphological evidence of avalanche activity in Scotland . Geografiska Annaler , 67A : 247 – 256 .
- Ward , R. G. W. , Langmuir , E. D. G. and Beatty , B. 1985 . Snow profiles and avalanche activity in the Cairngorm Mountains, Scotland . Journal of Glaciology , 31 : 18 – 27 .
- Watson , A. , Duncan , D. and Pottie , J. 2007 . No Scottish snow survives until winter 2006/07 . Weather , 62 : 71 – 73 .
- Werritty , A. 1984 . “ Stream response to flash floods in Scotland ” . In Catchment Experiments in Fluvial Geomorphology , Edited by: Burt , T. P. and Walling , D. E. 537 – 560 . Norwich : Geobooks .
- Werritty , A. and Ferguson , R. I. 1980 . “ Pattern changes on a Scottish braided river over 1, 30 and 200 years ” . In Timescales in Geomorphology , Edited by: Cullingford , R. A. , Davidson , D. A. and Lewin , J. 53 – 68 . Chichester : Wiley .
- Werritty , A. and Hoey , T. 2003 . Geomorphological Changes and Trends in Scotland: River Channels and Processes , Edinburgh : Scottish Natural Heritage Commissioned Report F00AC107B .
- Werritty , A. and Leys , K. 2001 . The sensitivity of Scottish rivers and upland valley floors to recent environmental change . Catena , 42 : 251 – 273 .
- Werritty , A. and McEwen , L. J. 1997 . “ The fluvial geomorphology of Scotland ” . In Fluvial Geomorphology of Great Britain , Edited by: Gregory , K. J. 21 – 32 . London : Chapman & Hall . Geological Conservation Review Series No. 13
- Whittington , G. 1985 . The Little Ice Age and Scotland's weather . Scottish Geographical Magazine , 101 : 174 – 178 .
- Whittington , G. and Edwards , K. J. 2003 . “ Climate change ” . In Scotland After the Ice Age: Environment, Archaeology and History, 8000 BC–AD 1000 , Edited by: Edwards , K. J. and Ralston , I. B. M. 11 – 22 . Edinburgh : Edinburgh University Press .
- Wilson , P. 2002 . Holocene coastal dune development on the South Erradale peninsula, Wester Ross, Scotland . Scottish Journal of Geology , 38 : 5 – 13 .
- Wilson , P. , Christiansen , H. H. and Ross , S. M. 2002 . The geomorphological context and significance of wind-abraded gravels, boulders and outcrops from the coast of Scotland . Scottish Geographical Journal , 118 : 41 – 57 .
- Winterbottom , S. J. 2000 . Medium and short-term channel planform changes on the Rivers Tay and Tummel, Scotland . Geomorphology , 34 : 195 – 208 .