1. Introduction
Lung cancer is the leading cause of cancer-related deaths worldwide, with a 5-year survival of approximately 6%.[Citation1] Around 80% of these are of non-small-cell (NSCLC) histological type for which surgical resection or radical chemoradiotherapy offers the best prospect of cure. The vast majority of cases however are diagnosed at an advanced stage, and therapy options are limited. Although, cisplatin-based chemotherapy trials in this group show a clear survival benefit over supportive care alone, the actual increase in median survival is small (4 vs. 5.5 months).[Citation2]
The landscape of treating stage IV disease changed around a decade ago with the discovery of the epidermal growth factor receptor (EGFR) mutations.[Citation3] These were found in lung adenocarcinoma and were associated with a favorable response to EGFR tyrosine kinase inhibitors. Whilst new targeted therapies have been introduced in routine clinical practice, they are only suitable for a low percentage of patients and outside of this outlook has changed little.[Citation3] A better understanding of genetic changes in NSCLC has pushed the development of novel treatments for oncogenic drivers or actionable mutations as identified by preclinical evidence. However, only a small number of patients will benefit.
Novel therapies are needed and we have developed a cell and gene therapy for the treatment of metastatic lung cancer. In this article, we discuss some hurdles we face as we take our product into man in our recently funded phase I/II randomized trial, TACTICAL (Targeted stem cells expressing TRAIL as a therapy for lung cancer).
2. Choosing an anticancer therapy
The majority of patients with stage IV lung cancer will receive a platinum-based chemotherapy, with or without radiotherapy for palliative intent.[Citation3] Chemotherapy is a nontargeted treatment that exhibits significant side effects and toxicity whilst causing damage to healthy organs. The characteristics of an ideal anticancer treatment should exhibit minimal systemic toxicity, and accurately target and kill only transformed cells, in both primary and metastatic sites.
Tumor-necrosis-factor-related apoptosis-inducing ligand (TRAIL) is a protein that causes cellular apoptosis in a pathway independent of p53.[Citation4] It is particularly attractive as a cancer therapy, since it is able to selectively trigger apoptosis in transformed cells, leaving healthy cells unharmed. TRAIL works through the extrinsic apoptosis pathway (see ); however, some cross-talk does occur with the intrinsic system which responds to cellular stress, p53 activation, and DNA damage.[Citation5] Despite the promising results of recombinant TRAIL in vitro, failure in human trials when delivered intravenously have been attributed to its half-life of around 30 min. Investigators have since altered TRAIL to improve its therapeutic performance through ‘tagging’ with another protein to increase its size, or using a PEGylated form with a protracted half-life and efficacy.[Citation5] Monoclonal antibodies (MAbs) to TRAIL receptors have also been investigated, however, the presence of TRAIL decoy receptors and recruitment of immune have again led to disappointing results. Both recombinant TRAIL and MAbs do not cross the blood–brain barrier, further limiting their use in the presence of lung cancer metastases. Therefore, one would ideally deliver TRAIL using a vehicle that homes to and resides at the site of the tumor, delivering a consistent dose of therapy. We and others have successfully used human bone-marrow-derived adult mesenchymal stem cells to deliver TRAIL as an anticancer therapy.[Citation6]
Figure 1. TRAIL signalling activates apoptotic pathways. TRAIL acts on death receptors on the cell surface to activate the extrinsic pathway, while cellular stress and DNA damage causes apoptosis through the intrinsic pathway.
FADD = FAS-activated death domain; BID = BH3 interacting-domain death agonist; BAK = Bcl-2 homologous antagonist; Cyt-C = cytochrome c; Apaf-1 = apoptotic protease-activating factor 1.
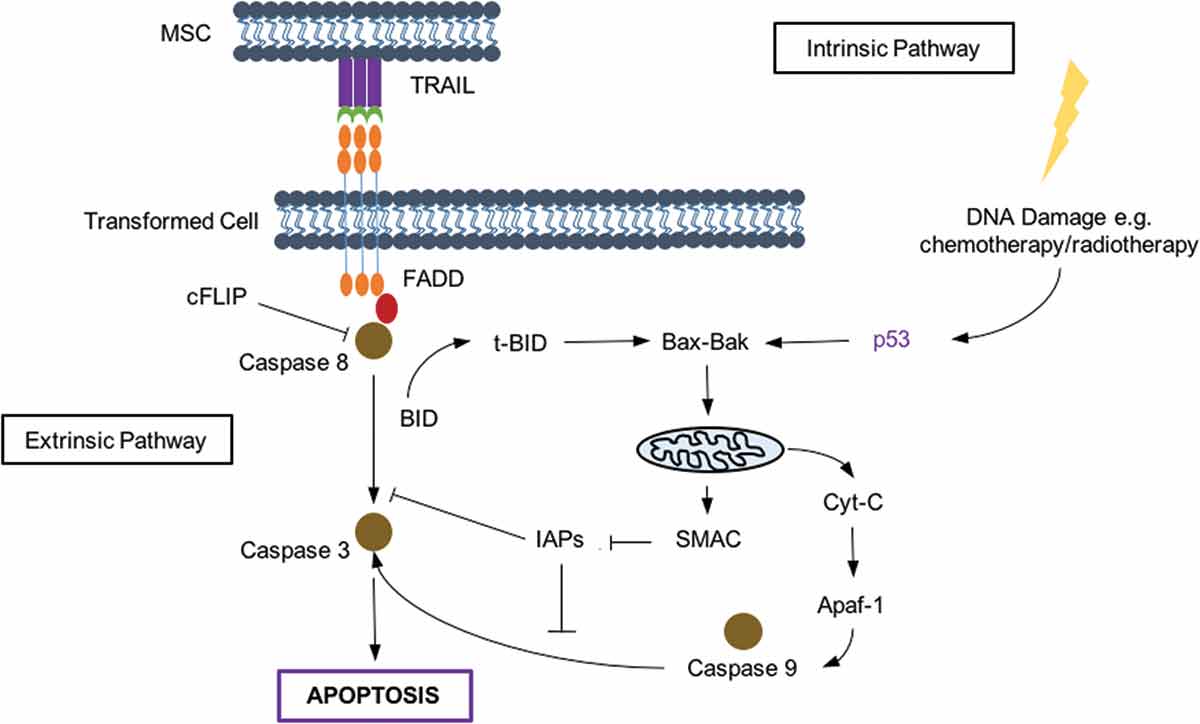
3. Mesenchymal stem cells: a vehicle to deliver anticancer therapies
Stem cells are undifferentiated and have capacity for unlimited self-renewal, and multi- or pluripotent differentiation. Mesenchymal stromal stem cells (MSCs) are one of the best characterized adult stem cells and have emerged as attractive candidates for cell therapies. They are present in virtually all tissues and can be derived from umbilical cord blood, adipose tissue, or most commonly, bone marrow.[Citation7] MSCs have a direct immunosuppressive effect, which has been exploited in clinical studies over the last decade to treat graft-versus-host disease and other immunologically mediated conditions including acute and chronic pulmonary disease.[Citation4] These studies have demonstrated that MSCs are safe, and low immunogenicity has obviated the need for human leukocyte antigen matching of allogeneic cells.[Citation4]
MSCs have multiple characteristics that make them attractive as a cell therapy for cancer (see ). The key feature being their ability to home to and engraft into tumors. Hence, they have potential to be used as a ‘Trojan Horse’ to deliver anticancer therapies. MSCs express chemokine receptors and cell adhesion molecules that aid in migration and homing to target tissues. Similar to leucocytes, MSCs may undergo both passive and active homing, and chemotaxis is thought to be largely dependent on CXCR4, which binds to CXCL12, which is known to be overexpressed at tumor sites. However, in lung cancer we have demonstrated overexpression of macrophage inhibitory factor, which also binds CXCR4 maybe a key modulator of MSC tumor tropism.[Citation8]
Table 1. Mesenchymal stem cell properties that make them ideal candidates for a cell therapy for cancer.
MSCs have been modified and used as therapeutic vectors in several diseases.[Citation10] In cancer, they have been successfully transduced with interferon-γ, interferon-β, thymidine kinase, or CX3CL1 expressing vectors [Citation4] resulting in effective inhibition of tumor growth in preclinical studies. One of the first trials in man will use MSCs genetically modified with herpes simplex virus thymidine kinase (HSV-tk) that activates pro-drug ganciclovir.[Citation11] This is a single-arm phase I/II study that will assess safety and efficacy of this treatment for advanced gastrointestinal cancers.
Our group has produced and used MSCs that have been stably transfected with vectors containing a gene construct of TRAIL.[Citation6] We have shown that this genetically modified cell therapy leads to either complete elimination or reduction of tumor growth when delivered intravenously in models of lung metastasis and mesothelioma. Despite the preclinical promise of gene and cell therapy, there are a number of challenges to overcome before a product is ready for clinical use, from gene transfer, to culture expansion of a clinical grade product, and tracking the cells once delivered.
4. Combined cell & gene therapy for lung cancer: from bench to bedside
4.1. Choice of viral vector and gene transfer
Viral vectors gain entry, survive, and transfer their genetic material into the host genome. The lentivirus is able to deliver large amounts of genetic information and infect both dividing and quiescent cells. They induce stable, long-term gene expression in the host, whilst avoiding insertion into oncogene promoters. The primary concern with viral vectors is the potential to create a replication-competent virus. Third-generation viruses for clinical use remove the virulent components and bundle it with a 4-plasmid system that contains information on the viral envelope, packaging, and the gene of interest. The packaging sequence is removed from all components other than the vector so they produce progeny only during virus manufacture.
4.2. Mesenchymal stem cells: manufacture & release criteria
MSCs constitute 0.002% of the bone marrow total stromal cell population.[Citation12] Their classification is specified in the position paper by Dominici et al. for the International Society for Cellular Therapy (ISCT).[Citation13] Although, MSCs are readily expandable up to 50 population doublings in 10 weeks, it has been suggested that in the clinic population doubling is kept below 20 for safety and efficacy.[Citation14] One challenge therefore faced is developing a stem cell therapeutic product of scale to cope with clinical demand. A single donor bone-marrow derived MSCs expanded for 20 population doublings in the laboratory will generate approximately 1 × 108–1 × 109 cells. Since, most clinical trials using stem cells deliver MSCs doses of up to 5 × 106 kg−1, each patient can require as many as 350 million cells per dose. Taking into account the number of patients in a trial, an excess of 100 billion cells will be needed. In order to cope with manufacturing demands of this scale, it is understandable that commercial suppliers look to the establishment of allogeneic pooled donor, cryopreserved cell banks that can simply be thawed when required.
Since massive expansion of MSCs is required for cell therapy trials, conventional culture methods are not practical and closed-system bioreactors to culture cells on a large-scale are available. However, reducing biological divergence is key, with close regulation of cell density, population doubling times, frequency of media changes, and harvesting of adherent cells before downstream processing and storage. Cryopreservation will be crucial to the storage of MSCs to deliver cell therapy for lung cancer on a large-scale. Whilst freeze–thawing leaves homing and adhesion molecules largely unharmed, we have shown there can be significant cell loss (around 20%) and disruption of MSC immune-modulatory properties (article in press). Therefore, protocols including that of storage time, freezing media composition, use of cryoprotectants and which cooling devices are used, all need to be optimized.
Translating the product to the bedside requires strict compliance with regulations set out by the European Medicine Agency. This requires development of release criteria for a product before it is taken to the bedside. With there being a clear consensus on cell surface markers to identify MSCs and regulation of GMP facilities, the tripartite component of these criteria – identification, viability, and sterility of MSCs pose little controversy.[Citation15] However, some of the other challenges are summarized in . The efficacy of MSCs should be measured, using assays predictive of potency for its intended clinical application. The ISCT recently published their perspective on immune functional assays for MSCs as potency criteria.[Citation15] Whilst there is limitation in defining the attributes of MSCs used in immune disorders, cells with a transferred gene often have a much clearer read-out of potency.
Table 2. Cell therapy for lung cancer: addressing the challenges of clinical manufacture.
4.3. Tracking MSCs in man
Optimal strategies for identifying the fate of MSCs following systemic infusion remain unclear. Although use of optical imaging to measure fluorescent and bioluminescent reporters has been successful in small animals, there is limitation with its depth of penetration when it comes to humans.[Citation9] Magnetic resonance imaging used to detect stem cells loaded with super-paramagnetic compounds is under investigation. However, positron emission tomography is likely to be at the forefront of cell imaging with development of new contrast agents. Investigators have combined HSV-tk with radioisotopes to image at high resolution.[Citation9] However, direct labeling of stem cells using novel radioisotopes may prove useful in tracking the fate of MSCs over longer periods of time, determining dosing schedules and in identifying any ‘off-target’ affects.
5. Expert opinion
Individuals with lung cancer most commonly present with advanced disease with little therapeutic option. Successful preclinical studies using genetically modified stem cells to treat cancer have paved the way for novel treatment in man. TRAIL selectively causes death in transformed cells and the use of MSCs overcomes previous problems of recombinant TRAIL half-life by delivering the treatment direct to tumor sites. We have shown the delivery of ‘full-length’ TRAIL using MSCs rather than soluble TRAIL is superior and may overcome some cancer cell resistance seen with the recombinant form. Further improvements in efficacy are seen when combined with chemotherapy, which may be as a result of TRAIL induced interaction with the intrinsic death pathway or downregulation of inhibitors of apoptosis. Either way, TRAIL appears potently synergistic with traditional lung cancer therapies.
Translating this novel treatment into a full-scale GMP-approved process for use in man is a Herculean challenge. MSC culture and modification need careful attention and upscaling this process to safely deliver sufficient treatment doses whilst ensuring cell characteristics have been preserved is paramount. We are overcoming the novel problems of large-scale cell transduction as well as expansion of a cell and gene therapy product. This knowledge will hopefully be useful for the rapidly expanding field of cell and gene therapies. Regardless of the challenges faced, modifying MSCs to deliver TRAIL may offer tremendous promise to thousands who have little treatment option.
Declaration of interest
S Janes is a Wellcome Trust Senior Fellow in Clinical Science (WT091730MA) supported by the Roy Castle Lung Cancer Foundation, Rosetrees Trust, the Welton Trust, the Garfield Weston Trust and UCLH Charitable Foundation. The cell therapy for lung cancer trial is supported by MRC DPFS scheme MR/M015831/1. UCLH/UCL received a proportion of funding from the Department of Health’s NIHR Biomedical Research Centre’s funding scheme, the UCL ECMC, and the CRUK Lung Cancer Centre of Excellence. E Sage is a clinical lecturer funded from cell therapy for lung cancer trial, supported by MRC DPFS scheme. The authors have no other relevant affiliations or financial involvement with any organization or entity with a financial interest in or financial conflict with the subject matter or materials discussed in the manuscript. This includes employment, consultancies, honoraria, stock ownership or options, expert testimony, grants or patents received or pending, or royalties.
References
- Jemal A, Bray F, Center MM, et al. Global cancer statistics. CA Cancer J Clin. 2011;61(2):69–90. doi:10.3322/caac.20107.
- Non-small Cell Lung Cancer Collaborative. Chemotherapy in non-small cell lung cancer: a meta-analysis using updated data on individual patients from 52 randomised clinical trials. Non-small cell lung cancer collaborative group. BMJ. 1995;311:899–909.
- Johnson DH, Schiller JH, Bunn PA Jr. Recent clinical advances in lung cancer management. J Clin Oncol. 2014;32(10):973–982. doi:10.1200/JCO.2013.54.6911.
- Loebinger MR, Janes SM. Stem cells as vectors for antitumour therapy. Thorax. 2010;65(4):362–369. doi:10.1136/thx.2009.128025.
- Stuckey DW, Shah K. TRAIL on trial: preclinical advances in cancer therapy. Trends Mol Med. 2013;19(11):685–694. doi:10.1016/j.molmed.2013.08.007
- Loebinger MR, Eddaoudi A, Davies D, et al. Mesenchymal stem cell delivery of TRAIL can eliminate metastatic cancer. Cancer Res. 2014;69(10):4134–4142. doi:10.1158/0008-5472.CAN-08-4698.
- Hoogduijn MJ, Popp F, Verbeek R, et al. The immunomodulatory properties of mesenchymal stem cells and their use for immunotherapy. Int Immunopharmacol. 2010;10(12):1496–1500. doi:10.1016/j.intimp.2010.06.022.
- Lourenco S, Teixeira VH, Kalber T, et al. Macrophage migration inhibitory factor-CXCR4 is the dominant chemotactic axis in human mesenchymal stem cell recruitment to tumors. J Immunol. 2015;194(7):3463–3474. doi:10.4049/jimmunol.1402705.
- Stuckey DW, Shah K. Stem cell-based therapies for cancer treatment: separating hope from hype. Nat Rev Cancer. 2014;14(10):683–691. doi:10.1038/nrc3798
- Heathman TRJ, Nienow AW, McCall MJ, et al. The translation of cell-based therapies: clinical landscape and manufacturing challenges. Regen Med. 2015;10(1):49–64. doi:10.2217/rme.14.73.
- Niess H, Von Einem JC, Thomas MN, et al. Treatment of advanced gastrointestinal tumors with genetically modified autologous mesenchymal stromal cells (TREAT-ME1): study protocol of a phase I/II clinical trial. BMC Cancer. 2015;15(1):1–13. doi:10.1186/s12885-015-1584-3.
- Jung S, Panchalingam KM, Wuerth RD, et al. Large-scale production of human mesenchymal stem cells for clinical applications. Biotechnol Appl Biochem. 2012;59(2):106–120. doi:10.1002/bab.1006.
- Dominici M, Le Blanc K, Mueller I, et al. Minimal criteria for defining multipotent mesenchymal stromal cells. Int Soc Cell Ther Position Statement. Cytotherapy. 2006;8(4):315–317.
- Prockop DJ, Brenner M, Fibbe WE, et al. Defining the risks of mesenchymal stromal cell therapy. Cytotherapy. 2010;12(5):576–578. doi:10.3109/14653249.2010.507330.
- Galipeau J, Krampera M, Barrett J, et al. International society for cellular therapy perspective on immune functional assays for mesenchymal stromal cells as potency release criterion for advanced phase clinical trials. Cytotherapy. 2015;18(2):151–159. doi:10.1016/j.jcyt.2015.11.008.