1. Introduction
The COVID-19 pandemic caused by the SARS-CoV-2 virus is having an unprecedented impact on global health derived from its quick spread, which is attributed to its high stability under environmental conditions, high mobilization of the globalized society, and the capacity to evolve as asymptomatic disease in some individuals. Although several knowledge gaps on this pathogen exist, it is clear that a vaccine is urgently needed to prevent the infection and possibly reinfection in the long term. The race for developing effective and safe SARS-CoV-2 vaccines has begun with several institutions and companies focused in such objective [Citation1]. Although the clinical evaluation of some candidates has been initiated, the pipeline for having an approved vaccine will surpass the first wave of COVID-19; the generated vaccines will be highly valuable to fight SARS-CoV-2 during further outbreaks and probably become tools for the fight of a seasonal pathogen, which will partially depend on its long term genetic evolution that will determine the reinfection risk. The development of MERS-CoV and SARS-CoV-1 vaccines performed over the last years comes up as a key precedent for this field given their genetic similarity and provides vital knowledge for the case of SARS-CoV-2 vaccine development. However, the challenge is still remarkable considering that no human vaccines targeting Coronavirus have been achieved thus far and clinical trials for such MERS-CoV and SARS-CoV-1 are scarce.
Figure 1. Graphic description of the key aspects involved in the development of mucosal anti-SARS-CoV-2 vaccines that might result in enhanced COVID-19 immunity compared to parenteral vaccines, a hypothesis based in the precedents generated by evaluating vaccine candidates against SARS-CoV-1 and MERS-CoV.
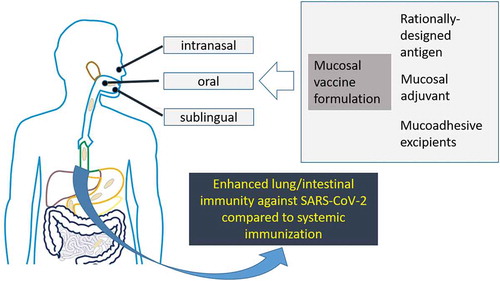
The angiotensin-converting enzyme 2 (ACE2) has been identified as the receptor of SARS-CoV. Since the spike (S) protein has pivotal roles in receptor binding and membrane fusion, this is an attractive antigen for the development of vaccines aimed at inducing antibodies that block virus binding/fusion and ultimately neutralize virus infection [Citation2]. Therefore, numerous SARS-CoV vaccine candidates have been developed having as target the full-length S protein. However, it should be considered that SARS-CoV is an RNA virus and as such it possesses an error-prone genome that is responsible for mutations that eventually allow the pathogen to escape from the host immune response. In fact, experimental SARS-CoV-1 vaccines based on the full-length S protein displayed in distinct nonreplicating viral vectors or in DNA vaccines have not been effective at inducing protective immunity against SARS outbreaks. Moreover, the use of the full-length S protein has arisen safety concerns as antibody responses that enhance the infection are induced [Citation3]. Interestingly, the S1 subunit carries the minimal receptor-binding domain (RBD, a.a. 318–510), which is an attractive target for the induction of neutralizing antibody responses due to its relatively low variability [Citation4]. Among the SARS-CoV-2 experimental vaccines, those based on the RBD as target antigen are in our opinion a better option compared to using the full-length S protein; this strategy not only allows inducing neutralizing antibodies against a well-conserved sequence, but avoids the induction of antibody responses that might enhance infection [Citation5].
Thus far several vaccine candidates against SARS-CoV-2 are under development; they comprise mRNAs coding for the S protein, Nonreplicating adenovirus 5 (Ad5) vector carrying the S gene, nanoparticles carrying antigens from the S protein, DNA vaccines carrying the S gene, and Virus-like particles based on the S protein [Citation1]. It is intriguing that some of the SARS-CoV-2 vaccine candidates under clinical evaluation are based on the full-length S protein; considering the undesired immune responses observed for the case of SARS-CoV-1 vaccines based on it. However, for many candidates details are not public regarding the specific sequences used from the S protein.
2. Implications of the immunization route
One key aspect that comes up in this topic has to do with the vaccination route and mucosal immunity. Although some aspects of specific SARS-CoV-2 vaccine candidates are kept confidential; it is clear that the majority of the formulations are parenterally administered, which is not surprising as it is the conventional approach that better fits the current vaccines production systems; resulting in a product that is relatively easy to formulate under an accurate dosage with the availability of well-characterized adjuvants. In fact, the majority of vaccines approved for human use are mainly administered parenterally; leading to the induction of immune responses that cover, to some extent, the protection of mucosal surfaces. Moreover, the recent knowledge on the homing of parenterally-primed lymphocytes to the lung or the well-known mechanisms regarding homing to the gut are leading to the selection of adjuvants and antigens to favor such mechanisms. Therefore these approaches promise to ultimately optimize parenteral vaccines for the induction of strong, protective IgA responses at the mucosal compartments [Citation6]. Among the trends to achieve this objective are the use of CAF01 and retinoic acid as adjuvants [Citation7]. Hence, antigens and/or adjuvants that induce the required homing molecules (integrins and chemokine receptors) on APCs and lymphocytes are a promising focus to induce mucosal immune responses. Nonetheless, this goal is certainly limited by the fact that the mechanisms that mediate T cell lung homing are not well understood yet. In this regard, nasal immunization is still considered by many authors as the ideal and more straightforward approach to induce potent immune responses against respiratory infections [Citation8]. Nonetheless, only few SARS-CoV-2 vaccine candidates are administered by mucosal routes, which include the following: a vaccine developed by Janssen (Belgium) consisting of a Nonreplicating adenovirus 26 (Ad26) vector carrying undisclosed genetic material of SARS-CoV-2, which is administered intranasally; a candidate produced by Vaxart (US) comprising an oral vaccine formulation (pill) based on different SARS-CoV-2 antigens; the intranasal CoroFlu vaccine under development by the University of Wisconsin–Madison, FluGen and Bharat Biotech, and the candidate designed by Altimmune (US), which corresponds to an intranasal vaccine [Citation9,Citation10].
Should vaccine developers focus on a higher degree in mucosal immunity? The results from the evaluation of several vaccine candidates against SARS-CoV-1 and MERS-CoV (both cononaviruses closely related to SARS-CoV-2) are a pivotal knowledge to determine the most effective path in this vaccine developmental race [Citation11]. summarizes a series of experimental SARS-CoV-1/MERS-CoV vaccines in which mucosal immunization led to attractive correlates of protection (even higher than those achieved by parenteral vaccines). Strategies directed toward RBD plus Poly(I:C) are promising due to the capacity to induce mucosal IgA responses in lung washes via the intranasal route. Replication defective rADV encoding the S protein administered via i.n. or s.l. routes showed to be an effective strategy; inducing cellular and humoral immune responses in the lung. The s.l. route offers the advantage that, unlike intranasal vaccination, the rADV vector is not directed to the central nervous system via the olfactory bulb, which could compromise the vaccine safety [Citation12]. I.n. immunization with SARS-CoV-1 VLPs proved to be an effective strategy to induce neutralizing IgA antibodies in serum and diverse secretions. DNA vaccines encoding the S protein have also been tested i.n.; leading to mucosal and cellular responses in the lung [Citation13]. The spike protein expressed in modified Vaccinia Ankara vector (MVA-S) and tested via i.n. and p.o. routes was able to induce higher antibody responses than the i.m. route [Citation14]. A recombinant adeno-associated virus (rAAV) expressing the receptor binding-domain of the SARS-CoV-1 S protein (RBD-rAAV) was assessed in i.n. immunization schemes; inducing humoral and cellular immune responses and higher protection against a virus challenge when compared to the i.m. route [Citation15]. I.n. immunization with Protollin formulated S protein also induced higher lung IgA and Th1 responses in comparison to the intramuscularly immunized mice [Citation16]. Overall, these studies suggest that even if mucosal immunization against Coronavirus does not provide sterilizing immunity, the ability to induce anti-SARS-CoV-2 IgA responses in the respiratory tract may prevent virus dissemination to the lung and prevent respiratory distress.
Table 1. Key outcomes derived from the preclinical evaluation of MERS-CoV and SARS-CoV-1 mucosal vaccine candidates.
3. Expert opinion
The above described panorama indicates with no doubt that mucosal immunity should be prominently considered in the path of developing effective SARS-CoV-2 vaccines; especially when considering that the ocular, airway, and mouth mucosae are the main port of entry for this pathogen. In fact, the main cause of death due to COVID-19 is pneumonia, therefore achieving robust immunity in the lung would better prevent/control the SARS-CoV-2 infection. Moreover, the induction of humoral responses able to achieve viral clearance in the gastrointestinal tract acquires a key relevance considering that SARS-CoV-2 infection extends to such compartment and potential fecal-oral transmission can last even after viral clearance in the respiratory tract [Citation27]. It is clear that the study of mucosal vaccine candidates should be encouraged (). In fact, it is widely known that the intranasal route is better alternative than parenteral routes when seeking to achieve immunity; particularly at the lung mucosa [Citation28]. The major challenges that have often hampered the development of mucosal vaccines include the requirement of higher antigen doses, the need of effective and safe mucosal adjuvants, and the difficulties to elicit significant immune responses as the default response is tolerance; especially toward soluble or subunit antigens. The mucosal surfaces have evolved in sophisticated mechanisms to maintain homeostasis as they are continually exposed to a wide variety of antigens, nonetheless the majority of them do not represent major danger. In healthy conditions the lung mucosal immune system is able to distinguish between environmental innocuous inhaled antigens versus potential pathogens and mount efficient and rapid immune responses against invading pathogens, nevertheless strong inflammatory responses are avoided to maintain the mucosal barrier homeostasis and prevent tissue damage; phenomena that are finely regulated by a network of tissue-resident cells [Citation29].
There is a myriad of alternatives to achieve a proper bioavailability and immunogenicity for mucosal vaccines; namely using refined vaccine formulations containing rationally-designed antigens, mucoadhesive excipients, and specific adjuvants [Citation30]. The design of antigens optimized for mucosal immunogenicity is one of the key aspects to ensure vaccine efficacy. The use of well-known mucosal carriers with adjuvant activity such as the B subunit of the heat labile enterotoxin from E. coli is advisable [Citation31]. Targeting M cell through the use of specific ligands, e.g. the Co-1 peptide, is also advisable [Citation32]. Another possible innovation consists in developing vaccines based on low-cost oral delivery vehicles (e.g. plant cells, yeast, and Gram-positive bacteria), which could render low-cost mucosal vaccines with the added benefit of showing improved immunogenicity with respect to soluble antigens [Citation33]. Moreover, the design of VLPs with improved mucosal immunogenicity can be also achieved by different approaches. For instance, decorating VLPs with variant specific surface proteins from Giardia lamblia has allowed to effectively induce, upon oral administration, immunoprotection against influenza and cancer in murine models [Citation34].
The cases of the nasal influenza vaccine licensed for human use and the development of dried formulations to be administered by noninvasive routes illustrate the potential of mucosal vaccines to surpass the difficulties during their developmental path [Citation35]. Moreover, other mucosal routes are acquiring special interest in vaccinology. For instance vaccines against pathogens causing respiratory pathologies, namely influenza and tuberculosis, have been administered by the s.l. route leading to promising results where the induced humoral responses compared, even surpassing, to those induced by parenteral immunization [Citation36,Citation37]. Oral immunization is also a highly attractive approach in terms of administration simplicity; avoiding sterile devices, the need for trained personnel, and pain during administration. Moreover oral immunization has the capacity to result in robust immune responses at the respiratory tract mucosa [Citation38]. The assessment of the immunoprotective potential of SARS-CoV-2 vaccines consisting of immunization protocols based on priming and boosting by distinct routes (including mucosal routes) will also generate important insights for the field.
In conclusion, considering mucosal immunity in the race of developing efficient vaccines against SARS-CoV-2 will lead to significant advances that will help, with no doubt, to cope with the SARS-CoV-2 emerging pathogen.
Declaration of interest
The authors have no other relevant affiliations or financial involvement with any organization or entity with a financial interest in or financial conflict with the subject matter or materials discussed in the manuscript apart from those disclosed.
Reviewer disclosures
Peer reviewers on this manuscript have no relevant financial relationships or otherwise to disclose.
Additional information
Funding
References
- Chen WH, Strych U, Hotez PJ, et al. The SARS-CoV-2 vaccine pipeline: an overview. Curr Trop Med Rep. 2020;3:1–4.
- Wan Y, Shang J, Graham R, et al. Receptor recognition by the novel coronavirus from wuhan: an analysis based on decade-long structural studies of SARS coronavirus. J Virol. 2020;94(7):e00127–20.
- Yang ZY, Werner HC, Kong WP, et al. Evasion of antibody neutralization in emerging severe acute respiratory syndrome coronaviruses. Proc Natl Acad Sci USA. 2005;102:797–801.
- He Y, Li J, Li W, et al. Cross-neutralization of human and palm civet severe acute respiratory syndrome coronaviruses by antibodies targeting the receptor-binding domain of spike protein. J Immunol. 2006;176(10):6085–6092.
- Du L, He Y, Zhou Y, et al. The spike protein of SARS-CoV–a target for vaccine and therapeutic development. Nat Rev Microbiol. 2009;7(3):226–236.
- Christensen D, Mortensen R, Rosenkrands I, et al. Vaccine-induced Th17 cell are established as resident memory cells in the lung and promote local IgA responses. Mucosal Immunol. 2017;10(1):260–270.
- Riccomi A, Piccaro G, Christensen D, et al. Parenteral vaccination with a tuberculosis subunit vaccine in presence of retinoic acid provides early but transient protection to M. Tuberculosis infection. Front Immunol. 2019;10:934.
- Allie SR, Bradley JE, Mudunuru U, et al. The establishment of resident memory B cells in the lung requires local antigen encounter. Nat Immunol. 2019;20(1):97–108.
- COVID-19 Vaccine Frontrunners. [cited 2020 Apr 11]. Available from https://www.the-scientist.com/news-opinion/covid-19-vaccine-frontrunners-67382
- Bharat Biotech. [cited 2020 Apr 11]. Available from https://www.bharatbiotech.com/
- Wang N, Shang J, Jiang S, et al. Subunit vaccines against emerging pathogenic human coronaviruses. Front Microbiol. 2020;11:298.
- Shim BS, Stadler K, Nguyen HH, et al., Sublingual immunization with recombinant adenovirus encoding SARS-CoV spike protein induces systemic and mucosal immunity without redirection of the virus to the brain. Virol J. 9(1): 215. 2012.
- Shim BS, Park SM, Quan JS, et al., Intranasal immunization with plasmid DNA encoding spike protein of SARS-coronavirus/polyethylenimine nanoparticles elicits antigen-specific humoral and cellular immune responses. BMC Immunol. 11(1): 65. 2010.
- Huang X, Lu B, Yu W, et al., A novel replication-competent vaccinia vector MVTT is superior to MVA for inducing high levels of neutralizing antibody via mucosal vaccination. PLoS One. 4(1): e4180. 2009.
- Du L, Zhao G, Lin Y, et al., Intranasal vaccination of recombinant adeno-associated virus encoding receptor-binding domain of severe acute respiratory syndrome coronavirus (SARS-CoV) spike protein induces strong mucosal immune responses and provides long-term protection against SARS-CoV infection. J Immunol. 180(2): 948–956. 2008.
- Hu MC, Jones T, Kenney RT, et al. Intranasal Protollin-formulated recombinant SARS S-protein elicits respiratory and serum neutralizing antibodies and protection in mice. Vaccine. 2007;25(35):6334–6340.
- Zhao J, Zhao J, Mangalam AK, et al. Airway memory CD4+ T cells mediate protective immunity against emerging respiratory coronaviruses. Immunity. 2016;44(6):1379–1391.
- Li E, Chi H, Huang P, et al. A novel bacterium-like particle vaccine displaying the MERS-CoV receptor-binding domain induces specific mucosal and systemic immune responses in mice. Viruses. 2019;11(9):799.
- Munster VJ, Wells D, Lambe T, et al. Protective efficacy of a novel simian adenovirus vaccine against lethal MERS-CoV challenge in a transgenic human DPP4 mouse model. NPJ Vaccines. 2017;2(1):1–4.
- Haagmans BL, van den Brand JM, Raj VS, et al. An orthopoxvirus-based vaccine reduces virus excretion after MERS-CoV infection in dromedary camels. Science. 2016;351(6268):77–81.
- Ma C, Li Y, Wang L, et al. Intranasal vaccination with recombinant receptor-binding domain of MERS-CoV spike protein induces much stronger local mucosal immune responses than subcutaneous immunization: implication for designing novel mucosal MERS vaccines. Vaccine. 2014;32(18):2100–2108.
- Raghuwanshi D, Mishra V, Das D, et al. Dendritic cell targeted chitosan nanoparticles for nasal DNA immunization against SARS CoV nucleocapsid protein. Mol Pharm. 2012;9(4):946–956.
- Gai WW, Zhang Y, Zhou DH, et al. PIKA provides an adjuvant effect to induce strong mucosal and systemic humoral immunity against SARS-CoV. Virol Sin. 2011;26(2):81–94.
- Lu B, Huang Y, Huang L, et al. Effect of mucosal and systemic immunization with virus‐like particles of severe acute respiratory syndrome coronavirus in mice. Immunology. 2010;130(2):254–261.
- Schulze K, Staib C, Schätzl HM, et al. A prime-boost vaccination protocol optimizes immune responses against the nucleocapsid protein of the SARS coronavirus. Vaccine. 2008;26(51):6678–6684.
- Luo F, Feng Y, Liu M, et al. Type IVB pilus operon promoter controlling expression of the severe acute respiratory syndrome-associated coronavirus nucleocapsid gene in Salmonella enterica Serovar Typhi elicits full immune response by intranasal vaccination. Clin Vaccine Immunol. 2007;14(8):990–997.
- Xiao F, Tang M, Zheng X, et al. Evidence for gastrointestinal infection of SARS-CoV-2. Gastroenterology. 2020;pii:S0016-5085(20)30282–1.
- Marasini N, Kaminskas LM. Subunit-based mucosal vaccine deliveryg systems for pulmonary delivery-Are they feasible? Drug Dev Ind Pharm. 2019;45(6):882–894.
- Holt PG, Strickland DH, Wikström ME, et al. Regulation of immunological homeostasis in the respiratory tract. Nat Rev Immunol. 2008;8(2):142–152.
- Hellfritzsch M, Scherließ R. Mucosal vaccination via the respiratory tract. Pharmaceutics. 2019;11(8):375.
- Ma Y. Recent advances in nontoxic Escherichia coli heat-labile toxin and its derivative adjuvants. Expert Rev Vaccines. 2016;15(11):1361–1371.
- Kim SH, Seo KW, Kim J, et al. The M cell-targeting ligand promotes antigen delivery and induces antigen-specific immune responses in mucosal vaccination. J Immunol. 2010;185(10):5787–5795.
- Rosales-Mendoza S, Angulo C, Meza B. Food-grade organisms as vaccine biofactories and oral delivery vehicles. Trends Biotechnol. 2016;34(2):124–136.
- Serradell MC, Rupil LL, Martino RA, et al. Efficient oral vaccination by bioengineering virus-like particles with protozoan surface proteins. Nat Commun. 2019;10(1):361.
- Tomar J, Born PA, Frijlink HW, et al., Dry influenza vaccines: towards a stable, effective and convenient alternative to conventional parenteral influenza vaccination. Expert Rev Vaccines. 15(11): 431–1447. 2016.
- Eickhoff CS, Blazevic A, Killoran EA, et al. Induction of mycobacterial protective immunity by sublingual BCG vaccination. Vaccine. 2019;37(36):5364–5370.
- Gallorini S, Taccone M, Bonci A, et al. Sublingual immunization with a subunit influenza vaccine elicits comparable systemic immune response as intramuscular immunization, but also induces local IgA and TH17 responses. Vaccine. 2014;32(20):2382–2388.
- Samuelson DR, Welsh DA, Shellito JE. Regulation of lung immunity and host defense by the intestinal microbiota. Front Microbiol. 2015;6:1085.