ABSTRACT
Introduction
In this review, recent developments and applications with cell-penetrating peptides (CPP) are discussed. CPPs are widely used tools for the delivery of various macromolecular therapeutics, such as proteins and nucleic acids.
Areas covered
The current review focuses on recent important advances and reports that demonstrate high clinical and translational potential. Most important clinical developments have occurred with the CPP-drug conjugate approaches that target various protein–protein interactions, and these have been highlighted subsequently. Most of the applications are targeting cancer, but recently, noteworthy advances have taken place in the field of antisense oligonucleotides and muscular dystrophies, lung targeting, and trans-BBB targeting.
Expert opinion
Successful applications and clinical development with the drug conjugate approaches are discussed. On the other hand, the reasons of why the nanoparticle approaches are not as far in development are analyzed.
1. Introduction
Cell-penetrating peptides (CPP) are usually 5–30 amino acid long, amphipathic or cationic motifs that have the ability to cross the cell membrane. The CPP field began when a protein-transduction domain of the trans-activator of transcription protein was described by a group studying the human immunodeficiency virus in the 1980s [Citation1]. Since then the number of CPPs has been rapidly increasing. CPPs have been extensively studied due to their ability to transport cargo molecules into the cells ()). Various types of cargo have been delivered with CPPs, including nucleic acids, nanoparticles, peptides, proteins, and small drug molecules [Citation2], placing CPPs to the focal point of drug delivery system research ()). The aim of the current review is to discuss the most recent updates regarding the development of CPP drug delivery systems, focusing on works that have the highest application potential, i.e., having impact from the perspective of either clinical or biotechnological use. Throughout the current text, the term “drug delivery system“ (DDS) includes both association of CPP with bioactive cargo and a bioactive cargo that has CPP properties. Concomitantly, some aspects of CPP research will be out of scope for the current review and we refer the reader to other overviews that discuss various types and the design of CPPs [Citation2,Citation3,Citation4], characterize CPP uptake mechanisms [Citation5,Citation6], and discuss aspects of utilizing the CPP drug delivery systems for the gene therapeutic applications [Citation7].
Figure 1. (a). The general idea of the using cell-penetrating peptides (CPP) for the drug delivery purposes. CPP offer a method for achieving delivery of macromolecular therapeutic cargo into the cell. For more information about cellular uptake mechanisms of CPP, please refer to the reviews in [Citation5,Citation6]. The most important intracellular targets are cytoplasm (please refer to the section “Targeting protein-protein interactions”) and cell nucleus [Citation7]. In order to achieve targeting of specific tissues (such as the liver), additional components should be integrated to the drug delivery system, as discussed in the current review. (b). There are various approaches for associating the carrier and the cargo. The main options are covalent attachment of the CPP and the therapeutic molecule via conjugation, or simply by fusing the (shorter) sequence of the cargo peptide with that of the CPP and obtaining a single peptide. Additionally, CPPs have been incorporated into proteins as protein domains, such as in case of developing nanobodies. Finally, CPPs are frequently used as a component of nanoformulations and nanoparticles either by noncovalent complexation or by covalently attaching the CPP at the surface of the nanosystem
![Figure 1. (a). The general idea of the using cell-penetrating peptides (CPP) for the drug delivery purposes. CPP offer a method for achieving delivery of macromolecular therapeutic cargo into the cell. For more information about cellular uptake mechanisms of CPP, please refer to the reviews in [Citation5,Citation6]. The most important intracellular targets are cytoplasm (please refer to the section “Targeting protein-protein interactions”) and cell nucleus [Citation7]. In order to achieve targeting of specific tissues (such as the liver), additional components should be integrated to the drug delivery system, as discussed in the current review. (b). There are various approaches for associating the carrier and the cargo. The main options are covalent attachment of the CPP and the therapeutic molecule via conjugation, or simply by fusing the (shorter) sequence of the cargo peptide with that of the CPP and obtaining a single peptide. Additionally, CPPs have been incorporated into proteins as protein domains, such as in case of developing nanobodies. Finally, CPPs are frequently used as a component of nanoformulations and nanoparticles either by noncovalent complexation or by covalently attaching the CPP at the surface of the nanosystem](/cms/asset/595350ff-d016-48d8-b17e-e257f9cfe3fe/iebt_a_1823368_f0001_oc.jpg)
2. CPP applications in cancer targeting
Due to their cell penetration ability and the possibility to internalize larger cargo molecules, CPPs have been used as a transporter component, giving therapeutic biomolecules access into the cells.
Without a doubt, cancer is the most frequently pursued clinical direction where new drug delivery systems (DDS) are tested. A representative successful example is development of a stapled α-helical CPP, codenamed ALRN-6924 [Citation8]. The bioactivity of ALRN-6924 arises from simultaneous inhibition of the oncogenes MDMX and MDM2, which has been shown to activate p53 protein in normally dividing cells. As a therapeutic strategy, this can be used to temporarily halt cell cycle activity and protect non-tumor cells from the side effects of chemotherapy. The peptide has advanced to several phase I and II clinical studies as a myelopreservation agent in patients with p53-mutant cancers [Citation8] (). Whereas the above example does not need specific tissue targeting, in the majority of the cases, there is a need to direct the therapeutic only to specific subpopulation of the cells. Since the CPPs mostly lack inherent tissue targeting, a combination with targeting ligands or various passive targeting methods is required.
Table 1. Highlighted examples of the DDS developments
2.1. CPPs with active cancer-targeting elements
Neundorf’s group recently demonstrated that CPP and targeting ligands follow a “kiss and run” model in cellular uptake process, in which the cell-selective uptake is achieved during a relatively short period of time when specific affinity interaction occurs. This short window (of opportunity) can be used with the help of a CPP that can then facilitate the uptake of the whole DDS. Although the CPP–ligand conjugate approach has been utilized by many researchers, Neundorf’s group demonstrated the process by using αvβ3 integrin-targeting ligand as an example [Citation11].
In line with the design of active targeting mechanisms, efficient binding ligands are needed. One of the important methods that helps discover such new molecules is phage display. Teesalu’s group has recently reported an application with their previously discovered LinTT1 peptide that specifically targets cell surface p32, a cancer marker protein. The authors functionalized iron oxide nanoworms with the homing peptide and a proapoptotic peptide KLAKLAK and demonstrated efficient targeting across a panel of five glioblastoma models in mice. Importantly, increased survival time was achieved [Citation12]. Glioblastoma belongs amongst the most challenging malignancies and is most difficult to treat in humans and any progress in this front deserves special attention.
Another approach is to use antibodies as anti-cancer agents in combination with CPPs. Gaston et al fused a CPP to full-length IgG to serve as a therapeutic antibody transporter. The authors used an antibody specific for carcinoembryonic antigen in combination with several different CPPs and showed that a specific set of CPPs can facilitate penetration of antibody into cancer cells [Citation13].
ANG1005 is a novel non-targeted therapeutic candidate for the treatment of metastatic breast cancer with spread in the CNS. A recent phase 2 clinical study showed that ANG1005 exerted symptom improvement and prolonged survival in adult patients with recurrent brain metastases from breast cancer [Citation14]. ANG1005 is a peptide–drug conjugate that consists of 3 paclitaxel molecules covalently linked to Angiopep-2, can cross the BBB and reach its target in the CNS where paclitaxel is released to exhibit its anti-tumor activity.
2.2. Cancer environment responsive strategies
One frequently used passive targeting technique utilizes pH-sensitive methods that are responsive to acidic tumor microenvironment. For example, pH-sensitive drug delivery was used by Han et al, who integrated a pH-responsive peptide. In their design, a low pH insertion peptide (pHLIP) was conjugated to the surface of magnetic nanoparticles, in combination with a chemotherapeutic drug gemcitabine. pHLIP peptide gains CPP activity in acidic (tumor) microenvironment, due to formation of α-helix that facilitates transmembrane positioning and cellular internalization. Effective tumor growth inhibition was achieved in both subcutaneous and orthotopic tumor mouse models [Citation15].
Juang et al introduced a multifunctional DDS that relied both on passive pH-responsive targeting and on active binding to extracellular cancer targets. As an anticancer agent, the authors designed pH-sensitive and peptide-modified liposomes to encapsulate microRNA miR-200, which can suppress epithelial-mesenchymal transition and inhibit cancer metastasis. Since it has been shown that miR-200 by itself is not sufficient as a cancer therapeutic, the authors used combinational therapy with liposomal irinotecan, a chemotherapeutic drug for colorectal cancer. Both the nanoparticles and liposomes were modified with (i) a ligand targeting cancer neovasculature undergoing angiogenesis, (ii) a CPP for particle internalization, (iii) a mitochondria targeting peptide, which promotes specific disruption of mitochondrial membranes in cancer cells and should lead to apoptosis [Citation16]. Finally, the nanoparticles were modified with passive targeting peptide – a pH-sensitive PEG–lipid, which could utilize the low pH of cancer microenvironment. All these components were designed to grant cancer targeting, cancer cell death, and a possibility to overcome multidrug resistance. The authors observed significant tumor growth inhibition and enhanced anti-cancer efficacy in CT26 colon tumor-bearing mice.
Ihsanullah et al utilized similar pH-activatable system, but elegantly combined this into a multifunctional drug delivery system. The authors added a hypoxia responsive element and combined the delivery of hypoxia-activatable prodrug tirapazamine and photosensitizer chlorine e6. The strategy behind their idea was to use a photosensitizer with external laser treatment, which would produce reactive oxygen ROS in affected tumor cells, which in turn would create a hypoxic environment in distal tumor cells. The nanoparticles were coated with a CPP with dimethylmaleic anhydride protection groups on the lysines, sensitive to lower pH environment. The polymeric core composition included hypoxia-responsive Azo-benzene bond that disintegrates in hypoxic environment and releases the chemotherapeutic, the latter is again hypoxia-sensitive. Therefore, the DDS strategy includes a serial activation and targeting steps: passive accumulation into the tumor tissue, pH-activation of the CPP, photodynamic stimulus and release of the chemotherapeutic, as well as induction of hypoxia, and finally, hypoxia-activated drug effect. The authors convincingly demonstrated the efficacy of the DDS in a mouse xenograft model [Citation17].
Another extensively studied (cancer) targeting strategy involves the use of extracellular enzyme-triggered activation. Matrix metalloproteinase (MMP) based targeting or activation has been used for the delivery of various anticancer agents or bioactive molecules (). MMPs are a family of endopeptidases capable of degrading all components of the extracellular matrix, therefore playing an integral role in tumor invasion and metastasis. Since MMPs are generally overexpressed in cancer, the idea of combining the abilities of CPPs with MMP targeting has been established as a cancer-targeting mechanism: polycationic CPP motif is complemented with anionic counterpart that results in a charge-neutral (inactive) conjugate. The activation occurs through proteolytic cleavage by MMP in the tumor tissue, liberating the CPP component [Citation18]. This strategy – termed aCPP (activatable CPP) – has been used for the tumor-targeted delivery of nucleic acid by using gene therapy [Citation19] or delivery of chemotherapeutics [Citation20].
Figure 2. Targeting strategies for the development of DDS. (a) Passive targeting with environmental activation of the DDS. The environmental stimulus can be tissue-specific difference in enzyme levels, pH, temperature, ROS levels etc. (b) Covalent conjugation of the affinity ligand, CPP functionality and a cargo biomolecule. The targeting ligand can be an antibody (fragment), homing peptide, receptor ligand etc
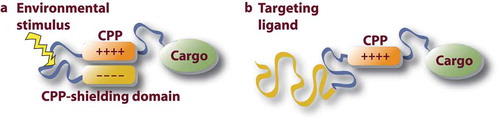
The ever-lasting dilemma of achieving either excellent tissue targeting without cellular uptake, or a CPP activity without disease targeting has been elegantly raised and tackled by Hingorani et al. As a starting point of their report, the authors showed that an antibody–drug conjugate exhibited excellent tumor targeting, but no bioactivity, whereas a CPP–drug conjugate, albeit resulting in drug bioactivity, did so without any tissue specificity. As a solution, the authors used the aCPP conjugate with a radiosensitizing drug and demonstrated targeting of the DDS to the tumor tissue, tumor-specific activation of the construct, and antitumor effect in a mouse xenograft model [Citation21].
2.3. Targeting protein-protein interactions
Recently, Rebollo and her group developed a peptide mimicking an important interaction in cancer growth: an interaction between caspase-9 and serine/threonine phosphatase PP2A. Combining a CPP with the interacting peptide resulted in a therapeutic peptide construct that was able to penetrate cells, inhibit the interaction of PP2A and caspase-9, and induce apoptosis in cell cultures [Citation22]. Importantly, the peptide was effective in reducing tumor growth in various animal models, including lung and breast cancer. Rebollo’s group has recently used a similar approach, where they blocked the interaction of PP2A with SET protein, an often over-expressed oncoprotein that is involved in the initiation and progression of cancer cells. The motif of PP2A binding to SET was identified and fused to a CPP to generate a chimeric protein that would block PP2A and SET interactions. Again, this peptide demonstrated anti-tumor activity in chronic lymphocytic leukemia xenograft model and breast cancer xenograft model [Citation23].
Protein PCNA (proliferating cell nuclear antigen) interacts with numerous cellular proteins via a motif called APIM. APIM motif is present in proteins involved in DNA repair. Its importance in cancers is that the DNA damage tolerance mechanisms are frequently de-regulated in solid tumors. Otterlei’s group has discovered that using PCNA interaction mimicry offers a potential cancer therapeutic strategy. The group developed a PCNA interaction mimicry and cell-penetrating peptide, called APIM peptide. The APIM peptide effectively inhibited PCNA interactions with its binding partners and resulted in increased hypersensitivity of cancer cells to chemotherapies in animal models [Citation24]. The peptide is currently being tested in a phase I study as a single agent intravenous therapy with advanced solid tumors. Recently, the group combined an APIM containing CPP with an EGFR/HER2/VEGFR inhibitor for enhanced combinatorial effect and confirmed its effects on tumor growth inhibition in mice [Citation25]. Finally, in a recent report, Otterlei’s group has extended their PCNA-interacting peptide platform to prokaryotes, as the bacterial homolog, β-clamp, shares the critical sequence with the eukaryotic PCNA. Hence, the authors showed that their CPP-modified peptide inhibited bacterial DNA replication and bacterial growth in a mouse skin infection model. This is an interesting find that has implications beyond cancer research and is important from the perspective of antimicrobial resistance [Citation26].
Jaraíz-Rodríguez et al fused a region of a gap junction protein Connexin43 (Cx43) to a CPP. It is known that Cx43 interacts with c-Src, a proto-oncogene that is overexpressed in gliomas. By using a CPP for translocating the chimeric CPP-Cx43 protein into the cells and interacting with c-Src, the authors succeeded in reducing the growth, invasion, and progression of malignant gliomas in gliomal stem cells, including primary gliomal stem cells from the patients and freshly removed surgical specimens [Citation27].
In an interesting approach, Zhang et al aimed to target a transcription factor FOXM1 which controls DNA replication and mitosis in cells. Because inhibition of FOXM1 terminates the cell proliferation and FOXM1 is capable of inhibiting the transcriptional activity of itself, the authors aimed to develop a chimeric protein–peptide anti-cancer drug with a CPP to gain entry of the recombinant protein into cancer cells. Accordingly, they fused the R9 CPP with a domain from FOXM1 and recombinantly expressed such chimeric construct in a bacterial expression system. In the end, their protein–CPP anti-cancer drug inhibited the tumorigenic ability of cancer cells and tumor growth in nude mouse xenograft models [Citation28].
According to recent reports, another intracellular protein targeting strategy deserves attention. Dai et al reported a strategy to direct protein-of-interest to proteolytic degradation pathway. The authors proposed a chimeric polypeptide or protein that consists of (i) a CPP motif, (ii) proteasome-targeting motif, and (iii) target protein binding motif (). The authors demonstrated the method with estrogen receptor-positive breast cancers and thus, in their example, the target protein to be degraded was estrogen receptor targeting ligand. As a result, the authors achieved efficient cytotoxic effects on estrogen receptor-positive cancer cells, as well as cancer growth inhibition in a mouse model [Citation29].
Figure 3. A strategy for specifically knocking down a protein of interest (POI). A chimeric protein is designed that includes a target protein binding motif (POI binding motif), a proteasome-targeting motif and a CPP functionality. The CPP facilitates the uptake of the chimeric construct, whereupon it binds to the POI. Finally, the proteasome-targeting motif is responsible for the degradation of the POI
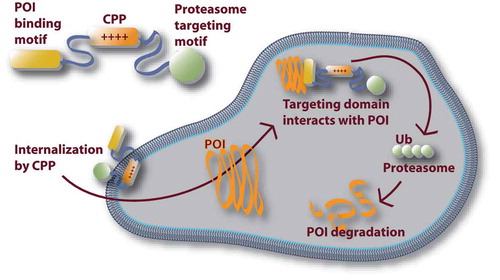
3. CPP applications in non-tumor diseases
3.1. Muscular dystrophies
A separate line of development has resulted in a promising approach of a CPP–antisense oligonucleotide (ASO) conjugates and their utilization in splicing correction and antisense-based approaches to treatment of muscular dystrophies, such as Duchenne (DMD) and myotonic dystrophy DM1. The muscular dystrophy group contains 9 different types of genetic disorders, including DMD and DM1. These diseases could theoretically be cured (or partially restored) by application of ASO, targeting genetic mutations or splicing sites. Since the affected tissue of the dystrophies is skeletal muscle, ubiquitous systemic delivery to skeletal muscle has to be achieved. A tandem contribution between the groups of Michael Gait and Matthew Wood has resulted in development of a delivery platform Pip6a for systemic administration and muscle delivery of ASO. This DDS has been applied for the treatment of DMD [Citation30], spinal muscular atrophy (SMA) [Citation31], and most recently, myotonic dystrophy DM1 [Citation32]. Their delivery platform is based on an arginine-rich CPP Pip6a and its covalent conjugate with the nucleic acid cargo.
Recently, Pip6a was assessed as a treatment strategy for myotonic dystrophy DM1. Pip6a–ASO conjugate efficiently reversed DM1 molecular defects and demonstrated physiological benefit in a mouse model [Citation32]. The efficacy was demonstrated after systemic administration, where the clinical effects were observed at lower cumulative doses than with alternative ASO treatments. It is encouraging that substantial levels of ASO were also detected in the heart and diaphragm tissues, as the mouse model does not allow assessment of the functional effects in these tissues, but these are critically affected in DM1 disease. Furthermore, the authors also tested the efficacy in human myogenic DM1 cells and similarly to the mouse model, the molecular indications of DM1 correction were achieved [Citation32]. The question that should be asked now is – how efficient is Pip6a–ASO tissue penetration in humans?
Wood and Gait’s approach of using molecular conjugate instead of a nanoparticle formulation may provide mobility advantage over the bulkier and not-so-strictly defined nanosystems. Indeed, simple conjugates may provide critical access through the most challenging tissues, such as muscle, and barriers, such as the BBB. Accordingly, the same groups recently identified an ApoE derivative peptide and showed that its conjugate with splice-switching oligonucleotide resulted in significant exon inclusion in the CNS, with positive therapeutic effects in a mouse model of SMA [Citation31]. Obviously, this work is exciting not only in the context of SMA, but represents a potential platform for the therapeutic delivery of oligonucleotides through BBB, into the CNS.
3.2. Trans-BBB delivery and targeting CNS
Subsequently, recent examples are briefly described that achieved successful trans-BBB delivery of therapeutic macromolecules. Henning-Knechtel et al were interested in trans-BBB delivery of a peptide and designed a fusion between amyloid beta–derived peptide and a CPP and tested its cell-penetrating and anti-aggregation potential in silico, in vitro and in cell culture models. The authors observed that their peptide inhibited amyloid beta fibrillation in a cell culture model, giving hope that such an approach can be used for the treatment of Alzheimer’s disease [Citation33].
One of the common neurodegenerative disorders is Parkinson disease (PD), accompanied by α-synuclein aggregation in neurons. In a similar manner as described above, Qu et al aimed delivery of a bulkier cargo and designed a chimeric peptide – in a recombinant expression system – composed of a CPP, an α-synuclein-binding motif, and a proteasome-targeting motif, with the aim of targeting α-synuclein to degradative pathways (). The authors showed induced degradation of α-synuclein through proteasome pathway and achieved beneficial functional effects: rescue of the mitochondrial dysfunction in a cell model and in primary neurons [Citation34]. It is noteworthy that this strategy of affinity-binding and concomitant initiation of cellular degradation of the target protein is similar to the one described above [Citation29] and may prove to be an efficient biomodulation strategy.
Maybe one of the most taunting drug delivery challenges is trans-BBB targeting of nanoparticulate systems for the delivery of nucleic acid polymers. Nevertheless, there are many neurological disorders that could potentially benefit from gene therapy. As a recent example, a series of works from the same group have explored and assessed the utility of functionalizing liposomes with a CPP and a targeting ligand. These works were accomplished by using DSPE- and PEG-modified liposomal components that offer a well-studied method of embedding a functionality onto the surface of the liposomes and shielding the surface with a biocompatible polymer. First, Arora et al assessed a gene therapeutic approach to treat Alzheimer’s disease (AD) by induction of BDNF gene expression in brain. Liposome nanoparticles were constructed for the targeted delivery of plasmid encoding BDNF gene to the brain. The targeting was achieved by double surface-modification of liposomes with (i) mannose that should interact with glucose transporter, and (ii) a CPP penetratin. The functionalized liposomes showed somewhat higher transfection of (i) primary astrocytes and neurons, (ii) exhibited transport across the BBB cell culture model, and finally, (iii) offered BDNF transfection in brain tissue after single i.v. administration into mice [Citation35]. The authors demonstrated 1.7x increase of BDNF levels from native baseline in brain after single administration. Whether this is sufficient for achieving a functional effect and clinical benefit, remains to be assessed in the future studies. The same group has conducted another study and developed similar plasmid transfection liposomes as described above, dual-functionalized with transferrin (Tf) ligand and a CPP penetration. This time, the plasmid was encoding NGF. Again, similar in vitro BBB delivery efficacy and primary neuron transfection was demonstrated. In order to assess the therapeutic potential, the CPP- and Tf-modified liposomes were administered to APP/PS1 mice longitudinally over 4 weeks. As a result, the authors observed decreased levels of Aβ peptide compared to the untreated mice, showing a potential for the AD disease [Citation36].
Dos Santos et al encapsulated pDNA into an RVG- and transferrin-modified liposomes. RVG is a cell and tissue penetrating peptide derived from rabies virus glycoprotein. It has shown to specifically target neuronal cells expressing nicotinic acetylcholine receptors. Transferrin, on the other hand should offer a means of achieving translocation through the BBB. Indeed, the authors showed delivery of the cargo to the brain [Citation37].
Khafagy et al recently used combined intranasal administration of a simple mixture of a CPP and leptin, a hormone that acts through receptors in hypothalamus and regulates satiety and body weight. The authors’ aim was to facilitate brain uptake of leptin and as a result, decrease hunger signals, as a strategy to reduce body weight. Indeed, such co-administration succeeded in reducing the weight of the laboratory rats [Citation38]. Another similar approach was proposed by Samaridou et al. These authors described an approach for the delivery of miRNA from the “nose-to-the-brain”, which is basically an intranasal administration, followed by enhanced passage through the olfactory epithelium – with the help of a CPP – as a potential therapeutic approach to AD. The benefits of avoiding systemic administration are also briefly discussed in other parts of the current review. Their DDS was based on noncovalent complexation of nucleic acid. The components were fatty acid-modified octaarginine CPP and miR-132, a miRNA that has been shown to exert beneficial physiological effects in AD preclinical models. The authors quantified miRNA levels in brain and concluded that their delivery system provides trans-BBB delivery. Additionally, molecular confirmation for the miRNA activity was provided, as the expression of two predicted mRNA targets in different brain areas were affected by their treatment [Citation39]. Future reports should show if such approach allows also functional effects from the modified genes, as well as clinical effects and will show how much potential it holds for the neurological disorders.
3.3. Lung-targeting DDS
Respiratory diseases represent an important group of disorders from the perspective of drug delivery. Currently available medical intervention for the diseases such as cystic fibrosis (CF) and asthma are mainly only symptomatic alleviation, but not causative treatment. Hence, gene therapeutic interventions represent especially huge potential, if the problem of delivery of macromolecular drugs would be solved. The delivery problem consists of 2 components: efficient transport of the therapeutic and tissue targeting [Citation40].
In addition to pulmonary diseases, lung cancer is an important topic under lung-targeting DDS. Ishiguro et al showed that nontargeted TAT CPP, in combination with calcium chloride can be used for gene delivery into lung and lung cancer. Interestingly, the authors explored and compared the efficacies of both local and systemic administration of plasmid encoding pro-apoptotic gene. In their approach, the authors achieved cancer growth inhibition with both regimes in a mouse xenograft model [Citation41]. Qiu et al reported a lung cancer-specific CPP and showed its efficacy – elevated survival in orthotopic lung tumor-bearing mice – with loaded siRNA against polo-like kinase 1 [Citation42] after single i.v. injection.
Local route offers a great way of avoiding the systemic administration, mainly because of two aspects: it allows the use of nontargeted DDS and the toxicity concerns are minimized, potentially allowing the use of higher doses, achieving more potent clinical effects. Indeed, in the above example, Ishiguro et al observed more efficient tumor growth inhibition with the intrathecal (i.e. local) administration of their nanoformulation [Citation41].
Kim et al have previously designed an amphiphilic α-helical leucine- and lysine-rich CPP that may have specific advantage in overcoming the mucoepithelial barrier, an important characteristic in the context of local delivery and respiratory system. The authors used intranasal delivery of resveratrol–CPP conjugate and demonstrated drug transport into the nasal epithelium and functional effects related to treatment of chronic rhinosinusitis in a mouse model [Citation43].
Osman et al have developed a PEGylated CPP nanoplatform for non-covalent complexation of pDNA and showed its efficacy in lung transfection in a mouse model. Their nucleic acid carrier consisted of a CPP and glycosaminoglycan-binding peptide. In order to improve in vivo characteristics and mucus penetration, tunable PEGylation strategy was utilized, where peptides with and without PEG were mixed, exactly as described in another report previously [Citation44]. This allows easy and flexible optimization of PEGylation density, offering a combination of optimal colloid stability in mucus model environment and reporter gene transfer activity in vivo by intratracheal administration of aerosol. Although this report only modeled the gene delivery efficacy and mucus penetration ability through reporter systems, this approach may be usable as a lung gene therapy in the future, and has a potential for the treatment of CF [Citation45].
Kurrikoff et al have taken this approach further and have achieved gene therapeutic modulation beyond a reporter model. In their report, the efficacies of two types of nucleic acid – pDNA and siRNA – were assessed with two different pulmonary disorders: acute pulmonary inflammation and chronic asthma [Citation46]. Instead of using local lung administration of the therapeutic, Kurrikoff used systemic administration of a nanoformulation that was based on lung-targeting CPPs [Citation47]. Their DDS consisted of a noncovalent complexes of CPPs PF14 or NF55 (exerting lung preference [Citation47]) and nucleic acid. Two gene expression modulation strategies were assessed in parallel: the RNAi against pro-inflammatory cytokine TNFα by either siRNA or pDNA encoding shRNA. The authors achieved successful therapeutic effect in a mouse model, with significant reduction in pulmonary pathology. Interestingly, these effects were reported with both of the RNAi approaches and in both acute pulmonary inflammation and asthma, demonstrating a promising approach for the future gene therapy.
3.4. Other diseases
Schiroli et al aimed to develop a DDS for local gene therapeutic manipulation of cornea with the aim of achieving noninvasive gene therapy through RNAi. As is typically needed for the delivery of nucleic acid cargo, CPP and noncovalent complexes with an siRNA was used. The authors took an original CPP POD [Citation48] and duplicated a peptide modification strategy that was demonstrated before [Citation49]. First, they added an N-terminal alkyl chain to induce the formation of micelles in aqueous environment. Secondly, chloroquine analog was covalently added as an agent to disrupt the endosomes and enhance cytoplasmic delivery (). Their DDS was assessed in a mouse model and achieved a topical knockdown of a reporter gene expression in the corneal epithelium [Citation50]. Unfortunately, the authors did not include the original peptides as controls in their study, as it would have been interesting to assess if their modifications indeed resulted in increased efficiency.
Figure 4. The structures of the original peptide PF6 (a) and the modified version of the POD peptide (b). The siRNA delivery strategy consists of (i) a CPP with basic amino acid residues, (ii) an N-terminal fatty acid chain that facilitates formation of micelles in water and (iii) an endosomolytic residue, trifluoromethylquinoline that has been covalently attached via lysine branching
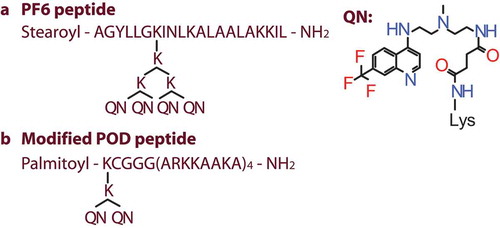
An interesting study was presented by Zhang et al, who recently developed a peptide that inhibits viral infection by looking at the human papillomavirus L2 capsid protein interactions with the retromer complex during the infection process. The authors assessed if a peptide consisting of the retromer-binding motif together with the CPP motif present in the L2 inhibit the viral entry process by acting as a retromer and L2 interacting peptide. TAT CPP motif was also assessed. Interestingly, the interacting peptide containing retromer-binding motif sequesters retromer from viral entry and inhibits the viral escape from the endosomes, inhibiting infection, the latter was also demonstrated in a mouse infection model [Citation51]. This shows that intracellular virus trafficking is a vulnerability that can be harnessed in therapeutic purposes and retromer binding is a potential antiviral target for the HPV.
4. Expert opinion
The above status update, with an emphasis on CPP research illustrates recent trends in the drug delivery field. The selection of the referenced research reports is by no means exhaustive, as there are numerous interesting article publications that have not been covered here. The idea behind the current selection was to discuss the achievements that have the most translational potential, i.e. applications that most directly relate to either clinical or biotechnological applications within the past couple of years.
Unsurprisingly, most of the DDS developments look at the cancer as a clinical target. Cancer as a pathology has direct and known genetic causative links that are available for the DDS development. Combined with the abundance of cell culture models and relatively easy models in animals, this is frequently the first choice to assess the efficacy of a new DDS. Nevertheless, the number of reports that target non-cancer pathologies is clearly on the rise. As can be observed from the current overview, there are interesting developments in antisense conjugation applications with respect to muscle dystrophies or lung targeting, among others. Hopefully, we will see the first clinical trials in these fields, especially with the ASO conjugates.
When reflecting the reported literature of the field, it is frequently sensible to divide the DDS development into two broad categories: the drug conjugates and the nanoparticles. Although these approaches have the same aim – to facilitate the transport of a therapeutic molecule into a diseased tissue – the association of the drug and the carrier is inherently different and apparently, the nanoparticle formation as a more complex system still has a long way to go. As a result, the more straightforward “conjugate approach” (as opposed to the nanoparticle development) is much closer to the clinical development, reflected by the increasing number of drug candidates that are entering clinical trials (). Nevertheless, the nanotechnology side also offers optimism: the number of preclinical studies with interdisciplinary drug vector design that can be described as “smart,” “programmable,” or “artificial virus” is ever increasing and as a result, the published reports show ever improved efficacies, enhanced targeting and good biocompatibility.
Regarding the shortcomings in clinical development with the CPPs, similar reviews in the past have repeatedly drawn attention and raised critics about some inadequacies of study designs or interpretation of results [Citation7,Citation40,Citation52,Citation53] as the reason for slow progress in the field. As a positive side, this situation has been improving, which is reflected by the large number of well-designed studies referenced above. Within the above-mentioned shortcomings, a number of specific points have been raised that should be overcome and it seems that improvement has indeed occurred: the preclinical study design has been improving. There are more studies that include relevant in vivo models. The quality of in vivo models, in turn, has been improving: these tend to be better controlled and designed where frequently the actual bioactivity of the drug and not only the accumulation data is presented; the bioactivity arises from physiological measurements and not only from the reporter models. The technical level of the works has been improving and this has been beneficial from the pharmaceutical development point of view ().These aspects help design more relevant DDS.
As a conclusion, the current review summarizes recent applications with the development of peptide-based drug delivery systems. Numerous examples of new drug candidates have been listed that have high potential to reach in clinical use in the future. While most of these examples predictably aim cancer intervention, there are also a number of new directions from the other domains.
Article highlights
The analysis of the drug delivery system development within the recent years demonstrates increased number of works with high clinical potential.
Most applications target cancer, but there are increasing number of other pathologies that are being developed.
Recent interesting examples with the ASO delivery are potential for the treatment of muscular dystrophies.
Several DDS with drug conjugates have reached to the level of clinical assessment and this represents the most potential CPP application area.
A number of promising CPP nanoformulations have been reported, but this subfield is more complex and needs more time to mature enough to reach the clinical stage.This box summarizes key points contained in the article.
Reviewer disclosures
Peer reviewers on this manuscript have no relevant financial relationships or otherwise to disclose.
Declaration of interest
The authors have no other relevant affiliations or financial involvement with any organization or entity with a financial interest in or financial conflict with the subject matter or materials discussed in the manuscript apart from those disclosed.
Abbreviations
aCPP - activatable CPP
AD - Alzheimer’s disease
ASO - antisense oligonucleotide
BBB – blood-brain barrier
BDNF - Brain-derived neurotrophic factor
CF - cystic fibrosis
CNS – central nervous system
CPP – cell penetrating peptide
Cx43 –gap junction protein Connexin43
DDS – drug delivery system
DM1 – myotonic dystrophy type 1
DMD - Duchenne muscular dystrophy
DSPE - 1,2-Distearoyl-sn-glycero-3-phosphorylethanolamine
ELP - elastin-like polypeptide
FOXM1 – transcription factor forkhead box M1
miRNA - microRNA
MMP – matrix metalloproteinase
NGF – nerve growth factor
NP – nanoparticle
PCNA - proliferating cell nuclear antigen
PD - Parkinson disease
pDNA – plasmid DNA
PEG - polyethylene glycol
pHLIP - low pH insertion peptide
POD - peptide for ocular delivery
PP2A – serine/threonine phosphatase
PPI – protein-protein interaction
PROTAC - proteolysis targeting chimera
RNAi – RNA interference
RVG - rabies viral glycoprotein
siRNA – small interfering RNA
SMA - spinal muscular atrophy
Tf - transferrin
Additional information
Funding
References
- Frankel AD, Pabo CO. Cellular uptake of the tat protein from human immunodeficiency virus. Cell. 1988 Dec;55(6):1189–1193.
- Langel Ü. CPP, cell-penetrating peptides. 1 ed. Singapore: Springer; 2019.
- Kardani K, Milani A,H, Shabani S, et al. Cell penetrating peptides: the potent multi-cargo intracellular carriers. Expert Opin Drug Deliv. 2019 Nov;16(11):1227–1258.
- Peraro L, Kritzer JA. Emerging methods and design principles for cell-penetrant peptides. Angew Chem Int Ed Engl. 2018 Sep;57(37):11868–11881.
- Uusna J, Langel K, Langel Ü. Toxicity, immunogenicity, uptake, and kinetics methods for CPPs. Methods Mol Biol. 2015;1324:133–148.
- Pei D, Buyanova M. Overcoming endosomal entrapment in drug delivery. Bioconjug Chem. 2019 Feb;30(2):273–283.
- Kurrikoff K, Gestin M, Langel Ü. Recent in vivo advances in cell-penetrating peptide-assisted drug delivery. Expert Opin Drug Deliv. 2016 Mar;13(3):373–387.
- Meric-Bernstam F, Saleh MN, Infante JR, et al. Phase I trial of a novel stapled peptide ALRN-6924 disrupting MDMX- and MDM2-mediated inhibition of WT p53 in patients with solid tumors and lymphomas. J clin oncol. 2017;35(15_suppl):2505.
- Bennett G, Lutz R, Park P, et al. Abstract 1167: development of BT1718, a novel bicycle drug conjugate for the treatment of lung cancer. Cancer Res. 2017;77(13 Supplement):1167.
- Lulla RR, Goldman S, Yamada T, et al. Phase I trial of p28 (NSC745104), a non-HDM2-mediated peptide inhibitor of p53 ubiquitination in pediatric patients with recurrent or progressive central nervous system tumors: a pediatric brain tumor consortium study. Neuro Oncol. 2016 Sep;18(9):1319–1325.
- Feni L, Parente S, Robert C, et al. Kiss and run: promoting effective and targeted cellular uptake of a drug delivery vehicle composed of an integrin-targeting diketopiperazine peptidomimetic and a cell-penetrating peptide. Bioconjug Chem. 2019 Jul;30(7):2011–2022.
- Säälik P, Lingasamy P, Toome K, et al. Peptide-guided nanoparticles for glioblastoma targeting. J Control Release. 2019 Aug;308:109–118.
- Gaston J, Maestrali N, Lalle G, et al. Intracellular delivery of therapeutic antibodies into specific cells using antibody-peptide fusions. Sci Rep. 2019 Dec;9(1):18688.
- Kumthekar P, Tang SC, Brenner AJ, et al. ANG1005, a brain-penetrating peptide-drug conjugate, shows activity in patients with breast cancer with leptomeningeal carcinomatosis and recurrent brain metastases. Clin Cancer Res. 2020 Jan;26:2789–2799.
- Han H, Hou Y, Chen X, et al. Metformin-induced stromal depletion to enhance the penetration of gemcitabine-loaded magnetic nanoparticles for pancreatic cancer targeted therapy. J Am Chem Soc. 2020 Mar;142(10):4944–4954.
- Juang V, Chang CH, Wang CS, et al. pH-responsive PEG-shedding and targeting peptide-modified nanoparticles for dual-delivery of irinotecan and microRNA to enhance tumor-specific therapy. Small. 2019 Dec;15(49):e1903296.
- Ihsanullah KM, Kumar BN, Zhao Y, et al. Stepwise-activatable hypoxia triggered nanocarrier-based photodynamic therapy for effective synergistic bioreductive chemotherapy. Biomaterials. 2020 Jul;245:119982.
- Jiang T, Olson ES, Nguyen QT, et al. Tumor imaging by means of proteolytic activation of cell-penetrating peptides. Proc Natl Acad Sci U S A. 2004 Dec;101(51):17867–17872.
- Künnapuu K, Veiman K-L, Porosk L, et al. Tumor gene therapy by systemic delivery of plasmid DNA with cell penetrating peptides. FASEB BioAdv. 2018;1(2):105–114.
- Guo F, Fu Q, Zhou K, et al. Matrix metalloprotein-triggered, cell penetrating peptide-modified star-shaped nanoparticles for tumor targeting and cancer therapy. J Nanobiotechnology. 2020 Mar;18(1):48.
- Hingorani DV, Crisp JL, Doan MK, et al. Redirecting extracellular proteases to molecularly guide radiosensitizing drugs to tumors. Biomaterials. 2020 Jul;248:120032.
- Arrouss I, Nemati F, Roncal F, et al. Specific targeting of caspase-9/PP2A interaction as potential new anti-cancer therapy. PLoS One. 2013;8(4):e60816.
- Andrini L, Marin GH, Inda AM, et al. Anti-tumoral effect of a cell penetrating and interfering peptide targeting PP2A/SET interaction. Folia Med (Plovdiv). 2020 Mar;62(1):31–36.
- Sogaard CK, Blindheim A, Rost LM, et al. “Two hits - one stone”; increased efficacy of cisplatin-based therapies by targeting PCNA’s role in both DNA repair and cellular signaling. Oncotarget. 2018 Aug 21;9(65):32448–32465.
- Søgaard CK, Nepal A, Petrovic V, et al. Targeting the non-canonical roles of PCNA modifies and increases the response to targeted anti-cancer therapy. Oncotarget. 2019 Dec;10(68):7185–7197.
- Nedal A, Ræder SB, Dalhus B, et al. Peptides containing the PCNA interacting motif APIM bind to the β-clamp and inhibit bacterial growth and mutagenesis. Nucleic Acids Res. 2020 Jun;48(10):5540–5554.
- Jaraíz-Rodríguez M, Talaverón R, García-Vicente L, et al. Connexin43 peptide, TAT-Cx43266-283, selectively targets glioma cells, impairs malignant growth, and enhances survival in mouse models in vivo. Neuro Oncol. 2020 Apr;22(4):493–504.
- Zhang Z, Bu H, Yu J, et al. The cell-penetrating FOXM1 N-terminus (M1-138) demonstrates potent inhibitory effects on cancer cells by targeting FOXM1 and FOXM1-interacting factor SMAD3. Theranostics. 2019;9(10):2882–2896.
- Dai Y, Yue N, Gong J, et al. Development of cell-permeable peptide-based PROTACs targeting estrogen receptor α. Eur J Med Chem. 2020 Feb;187:111967.
- Gait MJ, Arzumanov AA, McClorey G, et al. Cell-penetrating peptide conjugates of steric blocking oligonucleotides as therapeutics for neuromuscular diseases from a historical perspective to current prospects of treatment. Nucleic Acid Ther. 2019 Feb;29(1):1–12.
- Shabanpoor F, Hammond SM, Abendroth F, et al. Identification of a peptide for systemic brain delivery of a morpholino oligonucleotide in mouse models of spinal muscular atrophy. Nucleic Acid Ther. 2017 Jun;27(3):130–143.
- Klein AF, Varela MA, Arandel L, et al. Peptide-conjugated oligonucleotides evoke long-lasting myotonic dystrophy correction in patient-derived cells and mice. J Clin Invest. 2019 Nov;129(11):4739–4744.
- Henning-Knechtel A, Kumar S, Wallin C, et al. Designed cell-penetrating peptide inhibitors of amyloid-beta aggregation and cytotoxicity. Cell Rep Phys Sci. 2020 Feb 26;1(2):100014.
- Qu J, Ren X, Xue F, et al. Specific knockdown of α-synuclein by peptide-directed proteasome degradation rescued its associated neurotoxicity. Cell Chem Biol. 2020 Apr;27:763.
- Arora S, Sharma D, Singh J. GLUT-1: an effective target to deliver brain-derived neurotrophic factor gene across the blood brain barrier. ACS Chem Neurosci. 2020 May;11:1620–1633.
- Rodrigues BDS, Kanekiyo T, Singh J. Nerve growth factor gene delivery across the blood-brain barrier to reduce beta amyloid accumulation in AD mice. Mol Pharm. 2020 Apr;17:2054–2063.
- Dos Santos Rodrigues B, Arora S, Kanekiyo T, et al. Efficient neuronal targeting and transfection using RVG and transferrin-conjugated liposomes. Brain Res. 2020 May;1734:146738.
- Khafagy ES, Kamei N, Fujiwara Y, et al. Systemic and brain delivery of leptin via intranasal coadministration with cell-penetrating peptides and its therapeutic potential for obesity. J Control Release. 2020 Mar;319:397–406.
- Samaridou E, Walgrave H, Salta E, et al. Nose-to-brain delivery of enveloped RNA - cell permeating peptide nanocomplexes for the treatment of neurodegenerative diseases. Biomaterials. 2020 Feb;230:119657.
- Kurrikoff K, Langel Ü. Recent CPP-based applications in medicine. Expert Opin Drug Deliv. 2019 Nov;16(11):1183–1191.
- Ishiguro S, Alhakamy NA, Uppalapati D, et al. Combined local pulmonary and systemic delivery of AT2R gene by modified TAT peptide nanoparticles attenuates both murine and human lung carcinoma xenografts in mice. J Pharm Sci. 2017 Jan;106(1):385–394.
- Qiu M, Ouyang J, Wei Y, et al. Selective cell penetrating peptide-functionalized envelope-type chimeric lipopepsomes boost systemic RNAi therapy for lung tumors. Adv Healthc Mater. 2019 Jun;24:e1900500.
- Kim Y, Hwang S, Khalmuratova R, et al. α-Helical cell-penetrating peptide-mediated nasal delivery of resveratrol for inhibition of epithelial-to-mesenchymal transition. J Control Release. 2020 Jan;317:181–194.
- Veiman K-L, Künnapuu K, Lehto T, et al. PEG shielded MMP sensitive CPPs for efficient and tumor specific gene delivery in vivo. J Control Release. 2015 Jul 10;209:238–247.
- Osman G, Rodriguez J, Chan SY, et al. PEGylated enhanced cell penetrating peptide nanoparticles for lung gene therapy. J Control Release. 2018 Sep;285:35–45.
- Kurrikoff K, Freimann K, Veiman KL, et al. Effective lung-targeted RNAi in mice with peptide-based delivery of nucleic acid. Sci Rep. 2019 Dec 27;9(1):19926.
- Kurrikoff K, Veiman KL, Künnapuu K, et al. Effective in vivo gene delivery with reduced toxicity, achieved by charge and fatty acid -modified cell penetrating peptide. Sci Rep. 2017 Dec;7(1):17056.
- Johnson LN, Cashman SM, Read SP, et al. Cell penetrating peptide POD mediates delivery of recombinant proteins to retina, cornea and skin. Vision Res. 2010 Mar;50(7):686–697.
- Andaloussi SEL, Lehto T, Mäger I, et al. Design of a peptide-based vector, PepFect6, for efficient delivery of siRNA in cell culture and systemically in vivo. Nucleic Acids Res. 2011 MAY;39(9):3972–3987.
- Schiroli D, Gómara MJ, Maurizi E, et al. Effective in vivo topical delivery of siRNA and gene silencing in intact corneal epithelium using a modified cell-penetrating peptide. Mol Ther Nucleic Acids. 2019 Sep;17:891–906.
- Zhang P, Moreno R, Lambert PF, et al. Cell-penetrating peptide inhibits retromer-mediated human papillomavirus trafficking during virus entry. Proc Natl Acad Sci U S A. 2020 Mar;117(11):6121–6128.
- Deprey K, Becker L, Kritzer J, et al. Trapped! A critical evaluation of methods for measuring total cellular uptake versus cytosolic localization. Bioconjug Chem. 2019 Apr;30(4):1006–1027.
- Lehto T, Kurrikoff K, Langel Ü. Cell-penetrating peptides for the delivery of nucleic acids. Expert Opin Drug Deliv. 2012 Jul;9(7):823–836.