ABSTRACT
Introduction
Immunotherapies are focused on strategies that alter immune responses, using antibodies that binds to receptors on different immune cell subsets and either activate or suppress their functions depending on the immune response being targeted. Hence, the necessity of developing assays that assess the functional and biological effect of a therapeutic on its target. When incorporated into high-parameter flow cytometry panels, receptor occupancy assay can simultaneously evaluate receptor expression and drug occupancy on defined cell subsets, which can provide information related to functional effects, and safety.
Areas covered
This review focuses on the importance of developing, optimizing, and validating a robust Receptor Occupancy Assay (ROA) to improve dose selection, pharmacology monitoring and safety mainly in clinical settings.
Expert opinion
The designing of an ROA can be challenging and can lead to exaggerated pharmacology if not accurately developed, optimized, and validated. However, improvements in our understanding of epitopes, binding, affinities, and pharmacological effects may lead to improved antibody drug targeting and receptor evaluation.
1. Introduction
There are three general types of ROA measurements: the measurements of free receptor, the measurement of total receptor and the measurement of bound receptor ().
Figure 1. Three types of measurements for flow-based ROA. Free Receptor Assay: can be measured using a fluorescent conjugated competitor of the therapeutic or a directly labeled therapeutic to detect unbound. Bound Receptor Assay: can be measured using a specific anti-therapeutic antibody. Total Receptor Assay: can be measured using a non-competing antibody or saturating amount of unlabeled therapeutic.
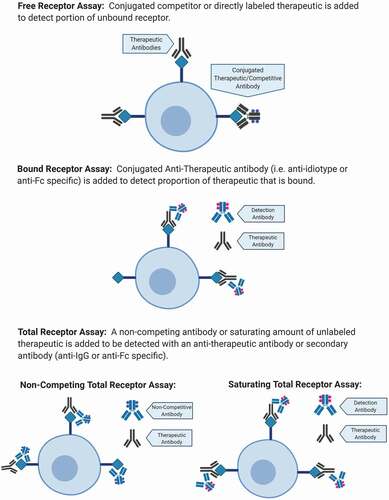
Before diving into the design of a ROA, it is crucial to understand the biology behind the therapeutic and its mechanism of action (MOA). It is also important to establish up front the availability of reagents to decide which of the three method is more suitable for the specific study. The evaluation of a potential therapeutic target usually begins with an assessment of how many receptors are expressed by a given cell subset. For example, if a receptor is abundantly or low expressed, it may be a difficult target to use due to potential off-target effects or the requirement of high/low doses of a therapeutic antibody. The availability of a good detection reagent that binds with specificity, an accurate method of detection of fluorescent background without drug and assay stability are all important.
The free receptors on the cell surface are an indirect determination of the bound receptor by the evaluation of receptors that are not occupied by the biotherapeutic. The detection of free receptors on the surface of the cells is performed using either a fluorescently labeled biotherapeutic or a competitive antibody. A competitive antibody is an antibody that binds to the same or similar epitope as the biotherapeutic agent, meaning that it will bind to the free receptors not occupied by the biotherapeutic. To mimic in vitro, a situation in which a biotherapeutic binds to the receptors on the cell surface, the cells are first stained with an unlabeled therapeutic antibody and then stained with a labeled version of the same antibody, which will only bind to the remaining free receptors. There are a few aspects needed to be considered when developing a free receptor assay: the conjugation chemistry can change the performance of the biotherapeutic affecting the binding site, so detection reagent will no longer binds or will bind to a higher affinity than the drug. It is important to carefully screen each clone in each fluorophore before using them and assign fluorophores to the cell population/s of interest that do not spillover in the labeled biotherapeutic channel. Also, if the affinity of the competitive antibody is lower or equal to the biotherapeutic, it will measure free; but if it is higher it may measure total by displacing some/all of the unlabeled-bound drug [Citation1]. Another consideration is to use molecules that compete with the biotherapeutic for receptor binding and do not have sequence homologies with the biotherapeutic. The latter may cause the presence of interfering factors which could neutralize the detection reagent and cause underestimation of the free receptor. The latter can be observed in some pre-clinical studies where animals develop antibodies to a human therapeutic [Citation2] but also in clinical trials, when the biotherapeutic agent is administered repeatedly [Citation3].
The availability of a non-competing antibody is a straightforward proposition that allows measurement of total receptor on the surface of the cells. The availability of non-competing antibodies allows the detection of all receptors, independent of the presence or absence of the biotherapeutic. A non-competing antibody is a fluorescently labeled antibody that binds to an epitope on the receptor that differs from the one recognized by the biotherapeutic agent. Another way to measure total receptor is pre-incubating the cells with a saturating concentration of the biotherapeutic followed by detection of the biotherapeutic using a fluorescently labeled anti-idiotype antibody. Alternatively, the cells at pre-dose are stained with a saturating amount of labeled biotherapeutic to measure total, and the post-treatment (post-dose) samples are then tested with the labeled biotherapeutic to detect free receptor. The pre-dose total is used for all post-dose time points to calculate Receptor Occupancy (RO).
The third measurement of RO detection is the bound receptor assay. This is a direct determination of RO, the receptor bounded by the biotherapeutic. To detect bound receptors, a fluorescently labeled anti-idiotype is most often used. The biotherapeutic agent that is bound to the target receptor is then detected with the fluorescently labeled anti-idiotype. For this assay, it is crucial to assess the specificity of binding as well as the optimal concentration of the anti-idiotype to be used to determine the background fluorescence. These are the cases in which control cell lines can be useful in establishing the most specific binding of different anti-idiotypes to the biotherapeutic. If the biotherapeutic is a monoclonal antibody (mAb), an anti-human secondary fluorophore-conjugate antibody that binds the biotherapeutic (anti-IgG or anti-Fc) that does not completely inhibit the biotherapeutic-target binding may be used as detection reagent. There are advantages and disadvantages in using a secondary antibody for bound biotherapeutic detection: the advantage is that it can amplify the detection signal specific for low copy number receptors, however, not all the biotherapeutic construct is developed having an FC or an IgG portion and it is easier to get nonspecific binding using a secondary antibody as detection reagent. Measuring the background can be technically difficult, therefore combining the method with a sample pre-incubated with saturating amount of unlabeled biotherapeutic to determine total receptor density can be helpful in determining the real RO.
The method developed can be then combined with PK/PD studies. Combining data from free and total receptor assays over time is a useful method for measuring the kinetics of antibody binding and calculating potential half-lives of these molecules for therapeutic applications.
The biology of the target receptor and its role in delivering the biotherapeutic message to the cells in order to modify a mechanism otherwise altered, is of fundamental importance for design of a ROA. In association to these methods, modulation of ROA can be designed to assess modification in the biologic turnover of the receptor upon binding to the biotherapeutic. These modifications may involve up-regulation, down-regulation, internalization of the target receptor or even release of membrane-bound receptors. Modulation of ROA can be also used to assess drug interference during combo treatment (labeled biotherapeutic in combination with therapeutic B) that can be observed through change in fluorescence of the labeled biotherapeutic (). Receptor modulation assessment can be performed by monitoring changes in total receptor relative to drug treatment dosage and time.
Figure 2. A. Labeled therapeutic spiked into the surrogate sample at saturating concentration. The histogram on the right side shows a saturated signal as indication of the therapeutic binding to all the targets on the cell surface. B. One or more concentration of therapeutic B spiked into the sample surrogate with saturating concentration of labeled therapeutic. The histogram on the right side shows a decrease in signal of the labeled therapeutic as indication of therapeutic B interference of target binding. C. Fluorescent-labeled therapeutic is spiked into the surrogate sample with pHrodo labeled therapeutic. If the target is internalized pHrodo is activated and a green-fluorescent signal will be detected. The fluorescent-labeled therapeutic will bind the target on the surface of the cells.
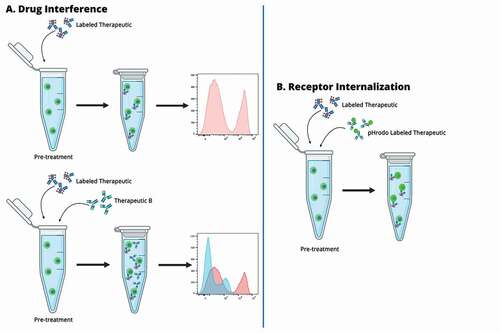
These assays can be used to either assess the frequency of positive cells relative to a known background control (e.g., Fluorescence-Minus-One controls and isotype controls) or to measure the mean or median fluorescent intensity (MFI) of the receptor expression, where the latter approach is more quantitative. It is also possible to calculate the density of the receptor on the cell surface running in parallel MESF beads conjugated with the same fluorophore as the detection reagent ().
Table 1. Flow cytometry panel design and RO calculation
Table 2. ROA targets, development stage, and purposes
Table 3. ROA design scheme and considerations
The different methods can be combined over time to measure kinetics of binding of the biotherapeutic, calculating potential half-lives and strategize dosage applications for clinical trials. The readouts of the assay can be reported for all the population of interest in the Immunophenotyping (IPT) panel.
Although the initial assay development steps in which the reagents are identified and the staining protocol optimized can be conducted using cell lines, assessment of sample stability and validation of the final assay must be conducted using the same sample type to be used in the pre-clinical or clinical study.
2. Development and optimization
Prior to design of an ROA, an understanding of the MOA for a biotherapeutic is critical to determine which immune population(s) is the most appropriate for the ROA development. To establish which reagents to use and therefore the type of RO method, an analysis of the level of expression of the target on the immune cell population(s) as well as the frequency and stability of the cell population(s) is necessary to successfully assess and measure RO (). One of the first steps to be performed is to assess the compatibility between the antibodies and fluorochromes in a RO panel. The antibodies and biotherapeutic are first evaluated individually and then in the context of all reagents in the final IPT panel. This assessment is important for a correct setup of the instrument (PMT voltages and compensation) to minimize potential interference or spectral overlap. For Free Receptor or Total Receptor assays that use labeled drug, competitive antibody, or noncompetitive antibody, the reactivity check should initially be performed in the absence of biotherapeutic, to allow for maximum binding of all reagents. For Bound Receptor assays, in which the detection of the therapeutic bound to its target is assessed using an anti-therapeutic secondary antibody, the reactivity check should be performed both in the presence and in the absence of the therapeutic at saturating concentrations, to allow for the detection of maximal signal. To ensure selection of optimal reagent concentration and avoid donor-to-donor variability, it is recommended to perform titration curves using at least 3 donors. All RO antibodies must be used at saturating concentrations to ensure maximum binding to all the available sites and maximize the signal-to-noise ratio. The saturating concentrations may vary according to the level of expression of the target, therefore ROA can be designed, developed, and tested on cell lines expressing different density of the target (low, medium, and high) to assess the specificity of binding of the therapeutic and to find the best anti-idiotype reagent or anti-therapeutic secondary detection reagent to be used in the assay. However, the optimization of the ROA must be performed in samples that resemble the characteristic of the samples to be tested. In both preclinical and clinical evaluation, peripheral whole blood is the preferred and most used specimen type for RO. Whole blood is minimally invasive and contains a wide range of immune cells likely to express the target receptor. It is common that the clinical samples to be tested are human fresh whole blood, therefore the antibodies in the (IPT) will be first titrated in whole blood using appropriate stimulants as needed for the detection of activation markers and will be ultimately tested and optimized in three fresh human whole blood samples, to assess reproducibility in different donors. Although whole blood is a preferred matrix, there are cases in which peripheral blood mononuclear cells (PBMCs) can be useful for the development of RO the assay: – they express more abundantly targets present on different tissues from the blood (i.e. brain); – the expression of stimulation induced markers is more stable and detectable in PBMC than in whole blood, spiking stimulated PBMC in whole blood represent a good approach to standardize a RO method targeting activated immune cells in clinical trial. There are cases in which the target of the therapeutic is not expressed on circulating cells and it is required to sample cells from tissue sites where the receptor is present. The literature reports some successful RO measurement in bone marrow, synovial fluid and colonic tissue matrices [Citation4,Citation5]. Based on our experience in developing RO assays in tissues such brain or bone marrow, this approach presents additional challenges related to more invasive specimen collection procedures as well as post-collection processing. The dissociation of tumor tissues in single cell suspension suitable for flow cytometry assay, can affect the expression and the turnover of the receptor on the surface of the cells. The enzymatic digestion used in tissue processing to degrade tissue structure, may affect the viability and morphology of cells other than the target itself, causing risk that will impact RO measurements. During development of this type of assay is recommended to test at least two different method of enzymatic dissociation, or one in combination with a mechanic-based dissociation, to choose the one that preserve the integrity and the biology of the target. In this instance Patient Derived Xenograft (PDX) models are a valuable tool to test the tumor tissue dissociation methods during development and assess the integrity of the target expressed on single cells. PDX tissues can be use as a test run for RO assays to guarantee success of RO measurement in clinical sample like tissues, before performing the assay for the clinical trial. PDX models might be also used for RO assay validation in the cases in which there is a shortage of availability of clinical samples.
3. RO in pre-clinical and clinical settings
RO assay can be useful and add important information in and to each stage of development of a therapeutic agent. In early phases, RO assays are valuable for species selection, compound screening, to demonstrate target binding and engagement in disease models and to correlate target engagement to efficacy in vivo (). RO assay can also be used in a pre-clinical setting to determine the minimal effective dose to be used in human subject in early Phase I clinical studies [Citation6–14]. A good example is represented by the translational PK/PD modeling analysis of MCLA-128, a mAb bispecific for HER2 and HER3, to predict safety and first clinical dose in humans. The simulated PK/PD model was created aligning with an allometric scale data obtained from PK studies in cynomolgus monkey and tumor growth data in mice [Citation15]. The importance of the RO was also demonstrated from the results of the dose escalation cohorts and the first effective concentration (EC) for Isatuximab (IgG1 anti-Kappa anti-CD38 mAb that binds to a specific epitope on CD38), in which only patients who attained RO ≥ 70% achieved a clinical response. An additional dose (20 mg/kg) was also used in this study as the initial dose established with RO (10 mg/kg) didn’t reach plateau in all the patients. Overall, the higher dose showed preferable pharmacokinetics (PK) parameters, including RO [Citation16]. Differently from Isatuximab, Hu5F9-G4 (5F9), a humanized IgG4 antibody that targets CD47 to enable phagocytosis, showed a linear PK and increases in drug exposure were proportional in solid tumors and lymphoma patients. Different doses were used, however, according to the target population: the low maintenance doses recommended for saturation of the target on red blood cells, didn’t reach saturation on circulating white blood cells for which RO was reached at higher dosage [Citation17].
ROA developed in preclinical setting in animal models require to be further optimized in early phases of clinical trials to confirm that the developed RO assay still performs as expected in humans. This is because species differences in receptor density levels and species-specific ligands may cause differences in the read-out of the assay, that if not considered, may lead to wrong result interpretation and potentially wrong dosing strategy in later phases of clinical trials. For this reason, is extremely important to demonstrate that and ROA developed in preclinical models still performs as expected in humans.
To generate a repeatable, reproducible, and high-quality assay staining conditions as temperature, time, reagent titration, instrument acquisition parameters must be fully optimized. It is extremely important to standardize the sample by having a global method Standard Operating Procedure (SOP) for assay, proficiency testing, global Quality Control (QC) monitoring, identical reagents, and cross-lab correlation. Also, especially if the ROA includes quantitative measurements of antigen expression the instrument setup must be optimized and regulated (global equipment SOP, inter-instrument standardization, global calibration beads) to enable a reproducible sample acquisition process that on its own requires global acquisition templates and checklist and identical acquisition software. Finally, data analysis requires global analysis templates, training and proficiency testing, secondary review of all data and identical analysis software. As the trial often is multi-center and longitudinal, all these steps are mandatory to guarantee success in the reproducibility and repeatability of the assays and to be able to correlate the data among patients and regions [Citation33–35]. Because of the shipment in different clinical sites, the selection of the appropriate tube of sample collection and anticoagulant is fundamental for the maintenance of the expression and stability of the target agent and of the bound drug and requires an anticipated knowledge of the time in which the specimen will be collected for testing [Citation36]. If stability of the target is not suitable in standard blood collection tubes (e.g., sodium heparin, EDTA, ACD), blood collection tubes incorporating stabilizing reagents (e.g., Cyto-ChexTM Streck, TransfixTM Cytomark) can be evaluated [Citation37]. These reagents have been reported to maintain epitopes of circulating lymphocytes in the blood for up to 14 days [Citation38]. Stabilization formulations that can be used to mitigate the shipping and handling risk associated with multi-site clinical trials, but they may impact extracellular receptor detection and thus also the RO assessment. For this reason, is important to assess sample stability at this stage of development. Assessing sample stability is crucial if samples analysis does not occur after few hours from samples collection. The assessment of the stability of cell population, target, and each single marker of the IPT panel is essential to guarantee the success of the ROA. The sample stability will be tested in unstimulated and stimulated samples, to evaluate the expression of markers that are not present in naïve cell populations. Sample stability can be assessed holding at least three different donors freshly collected at the temperature required for the assay for a defined number of time points, established based on the sample type, on the cell population expressing the target and on the shipment condition-in case of international shipments time points for sample stability assessment can reach up to 4 days [Citation39].
4. RO assay validation
Differently from pre-clinical assays, ROA used for clinical trials follow a fit-for-purpose validation specific to flow cytometry methods [Citation40–42]. Validation is an iterative approach where a series of activities built upon one another provide comprehensive evidence of the integrity of the data generated using the developed RO method. For exploratory clinical trials where there is no need to establish the maximum tolerated dose (MTD), a so-called mini validation is performed to determine sample stability, and precision assay. A more robust validation method is required for clinical trial in later phases and require following specific guidelines for validation of flow cytometry developed assays [Citation43]. These guidelines have stricter requirements for intra- and inter-assay precision and take in account long term, post-fixation, and post-collection samples stability.
Unlikely most of the flow cytometry assay, in an ROA surrogate samples containing varying levels of the biotherapeutic spiked into matrix samples are created for assay development, optimization, and validation. The samples are incubated with different and multiple concentrations of the biotherapeutic at specific time point and temperature needed to ensure a stable and equilibrated reaction. It is important the samples used during validation mimic the in vivo situation, where the biotherapeutic has achieved binding equilibrium with the target. If the clinical samples are whole blood or PBMCs, each step of validation will be run accordingly using three different naïve whole blood samples or naïve PBMCs samples. In the event, the clinical samples are solid tumors, ideal would be to run all the validation transcripts in tumor tissue, however, the tumor size may affect the ability to run all test. Three fresh solid tumor samples will be used to test sample stability, if possible, otherwise replaced by three fresh PDX solid tumor samples to closely resemble conditions observed into the clinic and naïve PBMC donors will be used to test fixed stability and precision. The intra-assay precision measures the closeness in results among the same sample performed by a single analyst on a single occasion. It should include a minimum of three samples and tested in triplicate. The % Coefficient of Variance (CV) considered adequate to pass the assessment changes according to the rarity of the target population and/or the heterogeneity and expression of the target (up to 25%/35%) [Citation43,Citation44]. The inter-assay precision assesses the variability between analyst on multiple days and on multiple instruments. It also should include a minimum of three samples, tested in triplicate in more analytical runs (). It is important to have an established ROA before assessing and validating sample stability [Citation36; Citation43] because the stability of each component of the ROA as well as the result (% RO) must be assessed and validated. Surrogate samples must be generated to assess sample stability trying to mimic conditions during shipment and will be processed at different time points post collection using different concentrations of the biotherapeutic to run the ROA. Fixed stability will be also determined using three donors, at storage temperature of the clinical samples and at different time points post-fixation. If the clinical samples are solid tumor biopsies, a quality control sample testing can be tested during validation where a surrogate sample will be thawed and incubated with two different concentrations of the biotherapeutic and without the biotherapeutic, in duplicate ().
Table 4. Validation parameters, samples, and acceptance criteria
5. Expert opinion
Drug-receptor interactions through ROA are critically important throughout the development life cycle. They improve species selection by quickly assessing receptor binding. They improve dose selection by measuring available free receptors that could potentially increase drug efficacy with a higher dose. They improve understanding of drug safety since biological toxicity of biotherapeutics, such as antibodies is usually due to exaggerated pharmacology [Citation45]. This is especially important for immunology targets, where it is important to understand the activation status of immune cells following drug treatment. When incorporated into high-parameter flow cytometry panels, one can simultaneously evaluate receptor expression and drug occupancy on increasingly more defined cell subsets, which can provide information related to functional effects, and safety.
Several biological factors must be evaluated during assay development to ensure reliable results for a receptor occupancy assay. Depending on the pharmacology, receptors may be modulated following binding to either ligand of drug. This may include multimerization, intracellular signaling, translocation into lipid rafts, change in conformational shape, as well as internalization. The half-life of the receptor may be affected by ligand or drug binding. Receptor expression may differ between cell types. Some receptors can be cleaved, others form multimers. Some antibody-based drugs are bi-specific, binding to two different targets, and all antibody drugs contain Fc regions which could bind to Fc receptors unless designed to prevent. Therefore, it is important to understand which binding is occurring to evaluate their effects on the ROA.
Reagent variables for ROA include detection antibody (i.e., anti-idiotypes) affinities, which could displace bound drug if too high, or fail to bind if too low. Steric hindrance could be affected. Fluorophore quenching could occur in larger, combined panels, reducing assay sensitivity. Receptor structure and epitope binding are important to ensure competitive and noncompetitive binding to allow for detection of free and total receptor, respectively.
Assay validation helps to ensure a consistent, reproducible assay, with defined stability, precision, and sensitivity, despite the assay variables defined above. This is important for interpretation of results. It is important to understand variability of the assay in different donors. Normalization of machine is required to derive consistent results from the same machine over time, from different instruments, and different locations. Often the validation may be performed using samples from healthy donors, but it is prudent to demonstrate that diseased samples behave similarly. Future improvements to ROA are likely to include improved reagent availability including drug detection, instrument sensitivity, consistency, and reproducibility. Development of the ROA is crucial to ensure a safe assay for clinical testing. With implementation of components within the upcoming H62 flow cytometry validation guidelines, ROA will become more defined, reproducible, and consistent. In addition, improvements in our understanding of epitopes, binding, affinities, and pharmacological effects may lead to improved antibody drug targeting and receptor evaluation. With the advent of nucleic acid-tagged antibody technologies and the ever-expanding numbers of fluorophores, combined with instrumentation to distinguish the signals generated, will surely improve our understanding of the receptor biology. Finally, evaluation of other, non-antibody, biologics, which require target binding to initiate pharmacological effect, are also likely to benefit from ROA.
Article highlights
Understanding drug-receptor interaction through ROA became extremely important for pre-clinical and clinical studies.
High dimensional flow cytometry allows for the identification of specific cell subsets within heterogeneous populations and the detection of poorly expressed targets.
There are three type of ROA and understanding the biology behind the therapeutic and the availability of reagents will determine which type of assay will be designed.
ROA improve species selection by assessing receptor binding; dose selection by measuring available free receptors.
ROA improves drug safety since biological toxicity of antibody drugs is usually due to exaggerated pharmacology.
This box summarizes key points contained in the article.
Declaration of interest
Each of the authors on this manuscript is an employee of Champions Oncology. The authors have no other relevant affiliations or financial involvement with any organization or entity with a financial interest in or financial conflict with the subject matter or materials discussed in the manuscript apart from those disclosed.
Reviewer disclosures
Peer reviewers on this manuscript have no relevant financial relationships or otherwise to disclose.
Acknowledgments
This review would not have been possible without the support of Champions Oncology. We thank our colleague Amy Wesa, Head of Research and Development department, and her team for providing insights and expertise in developing and performing ROA.
Additional information
Funding
References
- Stewart JJ, Green CL, Jones N, et al. Role of receptor occupancy assays by flow cytometry in drug development. Cytometry B Clin Cytom. 2016;90(2):110–116.
- Liang M, Schwickart M, Schneider AK, et al. Receptor occupancy assessment by flow cytometry as a pharmacodynamic biomarker in biopharmaceutical development. Cytometry B Clin Cytom. 2016;90(2):117–127.
- Schwickart M, Chavez C, Henderson S, et al. Evaluation of assay interference and interpretation of CXCR4 receptor occupancy results in a preclinical study with MEDI3185, a fully human antibody to CXCR4. Cytometry B Clin Cytom. 2016;90(2):209–219.
- Choy EH, Pitzalis C, Cauli A, et al. Percentage of anti-CD4 monoclonal antibody-coated lymphocytes in the rheumatoid joint is associated with clinical improvement. Implications for the development of immunotherapeutic dosing regimens. Arthritis Rheum. 1996;39(1):52–56.
- Vermeire S, O’Byrne S, Keir M, et al. Etrolizumab as induction therapy for ulcerative colitis: a randomised, controlled, phase 2 trial. Lancet. 2014;384(9940):309–318.
- Freeman DJ, McDorman K, Ogbagabriel S, et al. Tumor penetration and epidermal growth factor receptor saturation by panitumumab correlate with antitumor activity in a preclinical model of human cancer. Mol Cancer. 2012;11(1):47.
- Gupta S, Indelicato SR, Jethwa V, et al. Recommendations for the design, optimization, and qualification of cell-based assays used for the detection of neutralizing antibody responses elicited to biological therapeutics. J Immunol Methods. 2007;321(1–2):1–18.
- Latek R, Fleener C, Lamian V, et al. Assessment of belatacept-mediated costimulation blockade through evaluation of CD80/86-receptor saturation. Transplantation. 2009;87(6):926–933.
- Lowe PJ, Hijazi Y, Luttringer O, et al. On the anticipation of the human dose in first-in-man trials from preclinical and prior clinical information in early drug development. Xenobiotica. 2007;37(10–11):1331–1354.
- Ma A, Dun H, Song L, et al. Pharmacokinetics and pharmacodynamics of ASKP1240, a fully human anti-CD40 antibody, in normal and renal transplanted Cynomolgus monkeys. Transplantation. 2014;97(4):397–404.
- Mao C-P, Brovarney MR, Dabbagh K, et al. Subcutaneous versus intravenous administration of rituximab: pharmacokinetics, CD20 target coverage and B-cell depletion in cynomolgus monkeys. PLoS One. 2013;8(11):e80533.
- Woska JR Jr., Last-Barney K, Rothlein R, et al. Small molecule LFA-1 antagonists compete with an anti-LFA-1 monoclonal antibody for binding to the CD11a I domain: development of a flow-cytometry-based receptor occupancy assay. J Immunol Methods. 2003;277(1–2):101–115.
- Wyant T, Lackey A, Green M. Validation of a flow cytometry based chemokine internalization assay for use in evaluating the pharmacodynamic response to a receptor antagonist. J Transl Med. 2008;6(1):76.
- Sakhdari A, Mujib S, Vali B, et al. Tim-3 negatively regulates cytotoxicity in exhausted CD8+ T cells in HIV infection. PLoS One. 2012;7(7):e40146.
- de Vries Schultink AHM, Doornbos RP, Bakker ABH, et al. Translational PK-PD modeling analysis of MCLA-128, a HER2/HER3 bispecific monoclonal antibody, to predict clinical efficacious exposure and dose. Invest New Drugs. 2018;36(6):1006–1015.
- Martin T, Strickland S, Glenn M, et al. Phase I trial of isatuximab monotherapy in the treatment of refractory multiple myeloma. Blood Cancer J. 2019;9(4):41.
- Sikic BI, Lakhani N, Patnaik A, et al. First-in-human, first-in-class phase I trial of the anti-CD47 antibody Hu5F9-G4 in patients with advanced cancers. J Clin Oncol. 2019;37(12):946–953.
- Suchard SJ, Davis PM, Kansal S, et al. A monovalent anti-human CD28 domain antibody antagonist: preclinical efficacy and safety. J Immunol. 2013;191(9):4599–4610.
- Brahmer JR, Drake CG, Wollner I, et al. Phase I study of single-agent anti-programmed death-1 (MDX-1106) in refractory solid tumors: safety, clinical activity, pharmacodynamics, and immunologic correlates. J Clin Oncol. 2010;28(19):3167–3175.
- Advani A, Coiffier B, Czuczman MS, et al. Safety, pharmacokinetics, and preliminary clinical activity of inotuzumab ozogamicin, a novel immunoconjugate for the treatment of B-cell non-Hodgkin’s lymphoma: results of a phase I study. J Clin Oncol. 2010;28(12):2085–2093.
- Waibler Z, Sender LY, Merten C, et al. Signaling signatures and functional properties of anti-human CD28 superagonistic antibodies. PLoS One. 2008;3(3):e1708.
- Zheng Y, Scheerens H, Davis JC, et al. Translational pharmacokinetics and pharmacodynamics of an FcRn-variant anti-CD4 monoclonal antibody from preclinical model to phase I study. Clin Pharmacol Ther. 2011;89(2):283–290.
- Bensinger W, Maziarz RT, Jagannath S, et al. A phase 1 study of lucatumumab, a fully human anti-CD40 antagonist monoclonal antibody administered intravenously to patients with relapsed or refractory multiple myeloma. Br J Haematol. 2012;159(1):58–66.
- Byrd JC, Kipps TJ, Flinn IW, et al. Phase I study of the anti-CD40 humanized monoclonal antibody lucatumumab (HCD122) in relapsed chronic lymphocytic leukemia. Leuk Lymphoma. 2012;53(11):2136–2142.
- Goldwater R, Keirns J, Blahunka P, et al. A phase 1, randomized ascending single-dose study of antagonist anti-human CD40 ASKP1240 in healthy subjects. Am J Transplant. 2013;13(4):1040–1046.
- Tolcher AW, Sarantopoulos J, Patnaik A, et al. Phase I, pharmacokinetic, and pharmacodynamic study of AMG 479, a fully human monoclonal antibody to insulin-like growth factor receptor 1. J Clin Oncol. 2009;27(34):5800–5807.
- Kern B, Li W, Bono C, et al. Receptor occupancy and blocking of STAT5 signaling by an anti-IL-7 receptor α antibody in cynomolgus monkeys. Cytometry B Clin Cytom. 2016;90(2):191–198.
- Fu J, Wang F, Dong L-H, et al. Receptor occupancy measurement of anti-PD-1 antibody drugs in support of clinical trials. Bioanalysis. 2019;11(14):1347–1358.
- Vey N, Bourhis J-H, Boissel N, et al. A phase 1 trial of the anti-inhibitory KIR mAb IPH2101 for AML in complete remission. Blood. 2012;120(22):4317–4323.
- Junker F, Gulati P, Wessels U, et al. A human receptor occupancy assay to measure anti-PD-1 binding in patients with prior anti-PD-1. Cytometry A. 2021. DOI:https://doi.org/10.1002/cyto.a.24334.
- Ribrag V, Dupuis J, Tilly H, et al. A dose-escalation study of SAR3419, an anti-CD19 antibody maytansinoid conjugate, administered by intravenous infusion once weekly in patients with relapsed/refractory B-cell non-Hodgkin lymphoma. Clin Cancer Res. 2014;20(1):213–220.
- Lapusan S, Vidriales MB, Thomas X, et al. Phase I studies of AVE9633, an anti-CD33 antibody-maytansinoid conjugate, in adult patients with relapsed/refractory acute myeloid leukemia. Invest New Drugs. 2012;30(3):1121–1131.
- Green CL, Brown L, Stewart JJ, et al. Recommendations for the validation of flow cytometric testing during drug development: i instrumentation. J Immunol Methods. 2011;363(2):104–119.
- Purvis N, Stelzer G. Multi-platform, multi-site instrumentation and reagent standardization. Cytometry. 1998;33(2):156–165.
- Perfetto SP, Ambrozak D, Nguyen R, et al. Quality assurance for polychromatic flow cytometry using a suite of calibration beads. Nat Protoc. 2012;7(12):2067–2079.
- Brown L, Green CL, Jones N, et al. Recommendations for the evaluation of specimen stability for flow cytometric testing during drug development. J Immunol Methods. 2015;418:1–8.
- Canonico B, Betti M, Luchetti F, et al. Flow cytometric profiles, biomolecular and morphological aspects of transfixed leukocytes and red cells. Cytometry B Clin Cytom. 2010;78(4):267–278.
- Davis C, Wu X, Li W, et al. Stability of immunophenotypic markers in fixed peripheral blood for extended analysis using flow cytometry. J Immunol Methods. 2011;363(2):158–165.
- Hilt E, Sun YS, McCloskey TW, et al. Best practices for optimization and validation of flow cytometry-based receptor occupancy assays. Cytometry B Clin Cytom. 2020;100:63–71.
- Cunliffe J, Derbyshire N, Keeler S, et al. An approach to the validation of flow cytometry methods. Pharm Res. 2009;26(12):2551–2557.
- O’Hara DM, Xu Y, Liang Z, et al. Recommendations for the validation of flow cytometric testing during drug development: II assays. J Immunol Methods. 2011;363(2):120–134.
- Wood B, Jevremovic D, Béné MC, et al. Validation of cell-based fluorescence assays: practice guidelines from the ICSH and ICCS - part V - assay performance criteria. Cytometry B Clin Cytom. 2013;84(5):315–323.
- Litwin V, Oldaker T, Afonina E, et al. Validation of assays performed by flow cytometry. (1st ed. Wayne, PA. CLSI: CLSI document H62. Clinical Laboratory Standards Institute; 2021.
- Green CL, Stewart JJ, Högerkorp C-M, et al. Recommendations for the development and validation of flow cytometry-based receptor occupancy assays. Cytometry B Clin Cytom. 2016;90(2):141–149.
- Agency EM. ICH guideline S6 (R1) – preclinical safety evaluation of biotechnology-derived pharmaceuticals Science Medicine Health. 2011 JUN;22.