1. Introduction
1.1. MHC class
Finding and eliminating virus-infected cells is critical for host defense. Cytotoxic T cells have evolved into major players in this host protection mechanism. Major Histocompatibility Complex (MHC) class I plays a central role in the recognition of virus-infected cells by cytotoxic T cells. Viral antigens produced during viral replication are fragmented by proteolytic enzymes, transported to the endoplasmic reticulum by the transporter proteins, and presented on the surface of infected cells after loading onto MHC class I molecules [Citation1]. Once viral antigens loaded on MHC class I are recognized by cytotoxic T cells, the infected cells are killed by the activities of cytotoxic proteins such as perforin and granzymes, leading to the elimination of both the virus and infected cells [Citation1].
1.2. NLRC5
Although it has been known that MHC class I is essential to mount immune responses to infection, the regulatory mechanisms controlling MHC class I expression have remained poorly understood. Recently, we identified the MHC class I transactivator (CITA) or NLRC5 (NLR family CARD domain containing 5). NLRC5 regulates classical MHC class I genes (HLA-A, -B, -C in human), non-classical MHC class I genes (HLA-E, -F), and β2 M, LMP7, and TAP1 that play important roles in MHC-I pathways [Citation2–4]. NLRC5 contains an atypical CARD domain at the N-terminus, a nucleotide-binding oligomerization domain (NBD) in the middle, and leucine-rich repeats(LRRs)at the C-terminus. The NBD contains a Walker A motif, which is required for both the nuclear translocation of NLRC5 and the MHC class I promoter activity induced by NLRC5 [Citation2–4]. NLRC5 regulates the expression of MHC class I genes by forming a complex with multiple transcription factors. Cis elements in the MHC class I promoter for these transcription factors include X1 box bound by RFX (regulatory factor X) complex (RFXAP, RFXB, RFX5), X2 box bound by CREB (cAMP-response-element-binding protein)/ATF1 (activating transcription factor 1), and a Y box bound by NFY (nuclear factor Y) [Citation5]. In addition, studies using promoter mutants of the MHC class I gene revealed that the W/S box (TAACCTG) is also essential for NLRC5-dependent MHC class I gene transactivation. Furthermore, it has become clear that various histone acetyltransferases act in concert with NLRC5 to induce MHC class I gene expression, suggesting that not only transcription factors but also histone modifications may be involved in the induction of MHC expression [Citation5].
2. SARS-CoV-2 inhibits the induction of MHC class I genes
Coronavirus disease-2019 (COVID-19) caused by severe acute respiratory syndrome coronavirus 2 (SARS-CoV-2) is a highly contagious serious respiratory disease that was first identified in Wuhan, China, in December 2019. Since the outbreak was first reported, it has spread in record time, bringing a global health and economic crisis. It was declared a pandemic by the World Health Organization (WHO) in March 2020 [Citation6]. SARS-CoV-2 possesses multiple immune evasion strategies to escape from the surveillance of the host immune system, leading to impaired host antiviral responses. Recent studies have revealed that SARS-CoV-2 suppresses host defense programs by targeting type I and type III IFN signaling pathways [Citation7]. Type I and type III IFNs are secreted from virus-infected cells and induce a cellular state of viral resistance in neighboring cells. In cells infected by SARS-CoV-2, however, the signaling cascade downstream of type I and type I III IFN is inhibited and the host protection mechanism against SARS-CoV-2 is not effectively initiated [Citation8]. This is evident as the activation of kinases involved in type I and type III IFN signaling pathways, as well as the induction of type I IFN stimulated genes, were not observed upon SARS-CoV-2 infection [Citation8]. Additionally, a separate study highlighted the potency of SARS-CoV-2 ORF3b as an interferon antagonist, exhibiting the ability to suppress the type I IFN pathway [Citation9].
Cytotoxic T cells and natural killer (NK) cells play critical roles in eliminating virus-infected cells, and the functional exhaustion of those cells is associated with the disease progression [Citation10]. It has been reported in a recent study that, the number of T cells and cytotoxic T cells was observed to be lower in mild and severe COVID-19 patients compared with those in healthy donors, and remarkably decreased NK cell counts were also shown in patients with severe COVID-19 cases compared with mild cases and healthy donors [Citation11].
Since the MHC class I-dependent immune response plays an essential role in host defense against viral infection, multiple viruses target the MHC class I pathway to evade host immune surveillance. For example, Epstein-Barr virus (EBV) [Citation12], Kaposi’s sarcoma-associated herpesvirus (KSHV) [Citation13], Herpes simplex virus (HSV) [Citation14], and Human cytomegalovirus (HCMV) [Citation15] are reported to suppress the function of multiple key factors involved in the MHC class I pathway. In addition, it has been demonstrated that certain viruses, such as human immunodeficiency virus (HIV)-1, murine CMV (MCMV), and adenovirus possess specific viral factors that inhibit the MHC class I pathway. These viral factors include the negative regulatory factor (Nef) in HIV-1 [Citation16], the MCMV-encoded glycoprotein gp48 [Citation17], and the adenovirus early protein E1A [Citation18]. Recent studies also reported that H5N1 influenza (H5N1-VN1203) and the Middle East respiratory syndrome (MERS)-CoV suppress the expression of MHC class I genes, through modulating epigenetic regulation of the MHC class I gene locus [Citation19].
Multiple studies showed that the MHC class I pathway is also an attractive target of SARS-CoV-2 for immune evasion. The RNA-seq data analysis of nasopharyngeal swabs [Citation20] showed that SARS-CoV-2 infection in the airway epithelium in COVID-19 patients is associated with lower expression of MHC class I compared to that in SARS-CoV-2-negative participants [Citation21] (). Gene expression analysis of primary human bronchial epithelial cells [Citation8] showed that upon innate immunity-triggering by an influenza A virus mutant strain (IAVΔNS1), significant induction of MHC class I and related genes was detected, while the bronchial epithelial cells infected by SARS-CoV-2 were not able to induce MHC class I and related genes [Citation21] (). In addition, suppression of MHC class I and related genes was observed in ex vivo SARS-CoV-2 infected bronchial ciliated cells compared to bystander non-infected cells [Citation21,Citation22]. The impaired induction of MHC class I gene expression was also observed in the in vitro infection studies using various epithelial cell lines including Calu-3 (lung), Caco-2 (colon), and Huh7 (liver) infected with SARS-CoV-2 [Citation21].
Figure 1. SARS-CoV-2 infection inhibits the expression of MHC class I in human epithelial cells. a) Comparison of MHC class I expression levels between the nasal epithelial cells from negative participants and COVID-19 patients. MHC class I is suppressed by SARS-CoV-2 infection. b) Comparison of MHC class I expression in the primary human bronchial epithelial cells (HBEpC) infected by an influenza a virus mutant strain (IAVΔNS1) and SARS-CoV-2. IAVΔNS1 infection leads to the induction of MHC class I, whereas SARS-CoV-2 infection does not induce MHC class I.
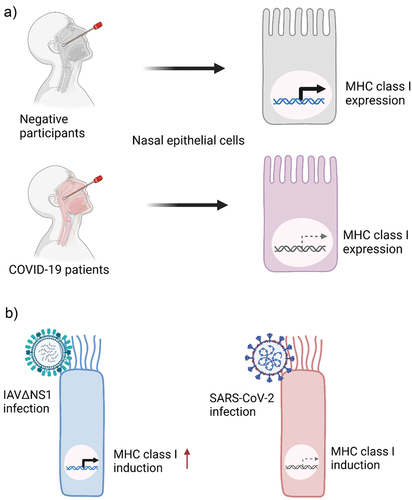
Extensive research on the function of SARS-CoV-2 viral proteins has highlighted the significance of various accessory proteins in immune evasion strategies, particularly in suppressing MHC class I expression. ORF3a has been identified to inhibit the secretory pathway of MHC class I, reducing its proper presentation on the cell surface
[Citation23]. ORF7a interferes with MHC class I assembly by competing with β2 m, a critical component of MHC class I complexes, hindering stable complex formation and promoting the retention of the complex in the endoplasmic reticulum [Citation23]. ORF8 expression in host cells has been shown to cause a decrease in MHC class I molecules, primarily due to an increased lysosomal degradation via autophagy [Citation24]. However, it is important to note that our study did not yield consistent results regarding the ORF8-induced suppression of cell surface MHC class I molecules [Citation21]. Further research is necessary to fully understand the diverse functions associated with ORF8 and its impact on MHC class I expression.
Given the critical role of NLRC5 in the MHC class I activation pathway, it seems reasonable that NLRC5 is an attractive target for immune evasion by cancer and viral pathogens [Citation25–27]. Our data suggested that overexpression of SARS-CoV-2 ORF6 (open reading frame 6) displayed impaired induction of MHC class I expression, through both transcriptional and functional inhibition of NLRC5 [Citation21]. Mechanistically, SARS-CoV-2 ORF6 hampers type II interferon-mediated STAT1 (signal transducer and activator of transcription 1) signaling pathway. Specifically, ORF6 prevents STAT1 transport from the cytoplasm to the nucleus, thereby impeding its ability to activate downstream genes involved in immune responses. One significant consequence of impaired STAT1 nuclear import is the inhibition of NLRC5 transcriptional expression. By suppressing NLRC5 transcription, SARS-CoV-2 ORF6 effectively reduces the production of MHC class I molecules. Moreover, cellular localization studies have revealed that SARS-CoV-2 ORF6 also disrupts the karyopherin-dependent protein importation pathway. This disruption inhibits the translocation of NLRC5 from the cytoplasm to the nucleus, leading to the suppression of transcriptional MHC class I activation and subsequent reduction in the number of cell surface MHC class I molecules. This impedes the ability of infected cells to effectively present viral antigens, thereby limiting the recognition and elimination of infected cells by cytotoxic T cells [Citation21]. ().
Figure 2. SARS-CoV-2 escapes from the human immune system by suppressing the MHC class I pathway. a) Cytotoxic T cells recognize the nonpathogenic virus-infected cells through the interaction between TCR and viral antigen-loaded MHC class I complex (top panel). SARS-CoV-2 infected cells evade cytotoxic T cells by reducing cell surface expression of MHC class I molecules (bottom panel). b) SARS-CoV-2 ORF6 perturbs MHC class I induction by inhibiting STAT1 and NLRC5 nuclear importation. TCR: T-cell receptor, NLRC5: NLR family CARD domain containing 5, STAT1: signal transducer and activator of transcription 1, ORF6: SARS-CoV-2 open reading frame 6 protein.
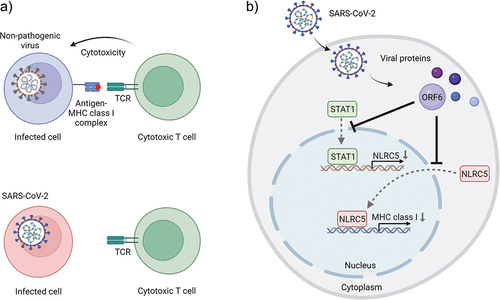
3. New therapeutic targets against SARS-CoV-2?
There is a significant need to identify therapeutic targets for SARS-CoV-2 infection. Multiple groups have identified anti-SARS-CoV-2 drugs targeting SARS-CoV-2 proteases Mpro and PLpro [Citation28–31]. Mpro and PLpro cleave polyproteins of SARS-CoV-2 to produce the non-structural proteins (nsp)1–16, making them promising targets for developing effective treatments against SARS-CoV-2. Other studies revealed that herbal medicine also possesses the ability to target multiple viral proteins, including Mpro [Citation32,Citation33]. By targeting these critical proteins, herbal medicines may be used as a part of supplementary care in addition to anti-viral therapeutic. Further investigations are necessary to explore the exact mechanism of herbal medicine treatment in combating the virus.
RNA interference (RNAi)-based therapeutics utilizing short interfering RNA (siRNA) and short hairpin RNA (shRNA) molecules offer a promising approach for silencing therapeutic targets for anti-SARS-CoV-2 therapy. Considering the severe lung failure caused by SARS-CoV-2 infection, delivering RNAi treatment via inhalation is expected to provide an effective intervention to stop the viral spread. The work by Chang et al (2022) described an inhalable short-interfering RNA, C6G25S, which covers 99.8% of current SARS-CoV-2 variants by targeting SARS-CoV-2 ORF1b. In a mouse model, This intervention was shown to be an effective therapy to reduce viral replication [Citation34]. Four structural proteins involved in viral conformation, spike (S), nucleocapsid (N), membrane (M), and envelope (E) proteins can also be targeted by RNAi in COVID-19 therapy [Citation35–40].
Apart from targeting functional proteins involved in viral assembly and replication, recent studies on SARS-CoV-2 immune evasion have unveiled a promising target for inhibiting SARS-CoV-2. ORF6, the SARS-CoV-2 accessory protein, is a potent virulence factor that antagonizes the host antiviral responses [Citation41]. SARS-CoV-2 ORF6 has been reported to suppress interferon-stimulated gene (ISG) expression by inducing nuclear retention of innate immune signaling-related transcription factors through interfering docking of the cargo KPNA1-KPNB1 complexes at the nuclear pore [Citation42,Citation43]. Additionally, SARS-CoV-2 ORF6 was shown to inhibit the nuclear export of newly synthesized mRNAs by binding to the nuclear pore complex (NPC) protein components Nup98 and Rae1, resulting in the suppression of the expression of multiple genes in SARS-CoV-2 infected cells [Citation44,Citation45]. Moreover, our study showed that SARS-CoV-2 ORF6 disables the induction of MHC class I by perturbing NLRC5 at both transcriptional and functional levels [Citation21].
Based on these findings, it is anticipated that silencing ORF6 can lead to the restoration of both the IFN pathway and MHC class I pathway, thereby reversing the suppression of the host immune system that occurs during SARS-CoV-2 infection.
In addition to RNAi therapy, an FDA-approved drug selinexor is another effective treatment for COVID-19. Selinexor targets XPO1 and blocks the Nup98-Rae1 complex-dependent nuclear export pathway [Citation46]. In both in vitro and in vivo systems, it has been reported that selinexor dose-dependently attenuates ORF6-mediated pathogenic effects, such as lethality and tissue-specific phenotypes observed in Drosophila [Citation47,Citation48] ().
Table 1. Therapeutic targets for COVID-19.
As an alternative approach to target ORF6, cell-penetrating peptides (CPPs), typically consisting of short peptides (fewer than 30 amino acids) with membrane penetrating functions [Citation49], could also be a potential strategy for COVID-19 therapy. Considering the virulent function of SARS-CoV-2 ORF6 by targeting the nuclear pore complex, further investigation on the binding region of ORF6 and nuclear pore complex may provide more detailed information for designing ORF6 decoys that can be carried by CPPs.
In summary, various studies have pointed out that SARS-CoV-2 ORF6 represents a significant virulence factor that promotes SARS-CoV-2 immune evasion. The inhibitory mechanism of NLRC5-MHC class I axis by ORF6 would be considered a key potential molecular target for developing next-generation therapeutics against COVID-19.
4. Expert opinion
The COVID-19 pandemic caused by SARS-CoV-2 has had a profound impact on global health and societies worldwide. The identification of the suppression of the MHC class I pathway by SARS-CoV-2 expands our understanding of COVID-19. This knowledge offers promising therapeutic targets, particularly ORF6, that can be explored for the development of treatments aimed at restoring immune recognition and enhancing the elimination of virus-infected cells.
While some of the potential treatments mentioned above may not be utilized in clinical practice due to side effects or limited effectiveness, they provide valuable insights and ideas for exploring additional therapeutic targets. These technologies can inspire further research and development, potentially leading to the discovery of more efficient treatments. For example, combining ORF6-targeting siRNAs with effective siRNAs that target viral replication and assembly proteins as a cocktail therapy presents a potential therapeutic strategy against COVID-19. This combination approach holds the potential to simultaneously inhibit viral replication and restore the host’s immune functions, offering a multi-pronged approach against COVID-19.
Moreover, SARS-CoV-2 is known for its ability to mutate rapidly, giving rise to new variants with different characteristics. The effectiveness of targeting ORF6 or any specific viral protein as a therapeutic strategy may be influenced by the emergence of new variants that possess altered immune evasion mechanisms. Ongoing surveillance and monitoring of viral evolution will be crucial to assess the long-term viability of targeting specific viral proteins.
Declaration of interests
The authors have no relevant affiliations or financial involvement with any organization or entity with a financial interest in or financial conflict with the subject matter or materials discussed in the manuscript. This includes employment, consultancies, honoraria, stock ownership or options, expert testimony, grants or patents received or pending, or royalties.
Reviewer disclosures
Peer reviewers on this manuscript have no relevant financial or other relationships to disclose.
Acknowledgments
The authors thank C. Matsukawa and K. Ogawa for secretarial assistance and all members of the Department of Immunology for their support.
Additional information
Funding
References
- Natarajan K, Li H, Mariuzza RA, et al. MHC class I molecules, structure and function. Rev Immunogenet. 1999;1(1):32–46.
- Kuenzel S, Till A, Winkler M, et al. The nucleotide-binding oligomerization domain-like receptor NLRC5 is involved in IFN-dependent antiviral immune responses. J Immunol. 2010 Feb 15;184(4):1990–2000.
- Meissner TB, Li A, Kobayashi KS. NLRC5: a newly discovered MHC class I transactivator (CITA). Microbes Infect. 2012 Jun;14(6):477–484. doi: 10.1016/j.micinf.2011.12.007
- Meissner TB, Li A, Liu YJ, et al. The nucleotide-binding domain of NLRC5 is critical for nuclear import and transactivation activity. Biochem Biophys Res Commun. 2012 Feb 24;418(4):786–791.
- Meissner TB, Liu YJ, Lee KH, et al. NLRC5 cooperates with the RFX transcription factor complex to induce MHC class I gene expression. J Immunol. 2012 May 15;188(10):4951–4958.
- Bedford J, Enria D, Giesecke J, et al. COVID-19: towards controlling of a pandemic. Lancet. 2020;395(10229):1015–1018. doi: 10.1016/S0140-6736(20)30673-5
- Park A, Iwasaki A. Type I and type III interferons – induction, signaling, evasion, and application to combat COVID-19. Cell Host Microbe. 2020 Jun 10;27(6):870–878.
- Blanco-Melo D, Nilsson-Payant BE, Liu WC, et al. Imbalanced host response to SARS-CoV-2 drives development of COVID-19. Cell. 2020 May 28;181(5):1036–1045 e9.
- Konno Y, Kimura I, Uriu K, et al. SARS-CoV-2 ORF3b is a potent interferon antagonist whose activity is increased by a naturally occurring elongation variant. Cell Rep. 2020;32(12):108185. doi: 10.1016/j.celrep.2020.108185
- Zhang C, Wang XM, Li SR, et al. NKG2A is a NK cell exhaustion checkpoint for HCV persistence. Nat Commun. 2019 Apr 3;10(1):1507.
- Zheng M, Gao Y, Wang G, et al. Functional exhaustion of antiviral lymphocytes in COVID-19 patients. Cell Mol Immunol. 2020 May;17(5):533–535. doi: 10.1038/s41423-020-0402-2
- Levitskaya J, Coram M, Levitsky V, et al. Inhibition of antigen processing by the internal repeat region of the Epstein–Barr virus nuclear antigen-1. Nature. 1995 Jun 22;375(6533):685–688.
- Sorel O, Chen T, Myster F, et al. Macavirus latency-associated protein evades immune detection through regulation of protein synthesis in cis depending upon its glycin/glutamate-rich domain. PLOS Pathogens. 2017 Oct;13(10):e1006691. doi: 10.1371/journal.ppat.1006691
- Hill A, Jugovic P, York I, et al. Herpes simplex virus turns off the TAP to evade host immunity. Nature. 1995 Jun 1;375(6530):411–415.
- Ahn K, Gruhler A, Galocha B, et al. The ER-Luminal domain of the HCMV glycoprotein US6 inhibits peptide translocation by TAP. Immunity. 1997;6(5):613–621. doi: 10.1016/S1074-7613(00)80349-0
- Schwartz O, Marechal V, Le Gall S, et al. Endocytosis of major histocompatibility complex class I molecules is induced by the HIV–1 Nef protein. Nat Med. 1996 Mar;2(3):338–342. doi: 10.1038/nm0396-338
- Reusch U, Muranyi W, Lucin P, et al. A cytomegalovirus glycoprotein re-routes MHC class I complexes to lysosomes for degradation. EMBO J. 1999 Feb 15;18(4):1081–1091.
- Chatterjee-Kishore M, van Den Akker F, Stark GR. Adenovirus E1A down-regulates LMP2 transcription by interfering with the binding of stat1 to IRF1. J Biol Chem. 2000 Jul 7;275(27):20406–20411.
- Menachery VD, Schafer A, Burnum-Johnson KE, et al. MERS-CoV and H5N1 influenza virus antagonize antigen presentation by altering the epigenetic landscape. Proc Natl Acad Sci, USA. 2018 Jan 30;115(5):E1012–E1021.
- Lieberman NAP, Peddu V, Xie H, et al. In vivo antiviral host transcriptional response to SARS-CoV-2 by viral load, sex, and age. PLoS Biol. 2020 Sep;18(9):e3000849. doi: 10.1371/journal.pbio.3000849
- Yoo JS, Sasaki M, Cho SX, et al. SARS-CoV-2 inhibits induction of the MHC class I pathway by targeting the STAT1-IRF1-NLRC5 axis. Nat Commun. 2021 Nov 15;12(1):6602.
- Ravindra NG, Alfajaro MM, Gasque V, et al. Single-cell longitudinal analysis of SARS-CoV-2 infection in human airway epithelium identifies target cells, alterations in gene expression, and cell state changes. PLoS Biol. 2021 Mar;19(3):e3001143. doi: 10.1371/journal.pbio.3001143
- Arshad N, Laurent-Rolle M, Ahmed WS, et al. SARS-CoV-2 accessory proteins ORF7a and ORF3a use distinct mechanisms to downregulate MHC-I surface expression. bioRxiv: The Preprint Server For Biology. 2022 May 17. doi: 10.1101/2022.05.17.492198
- Zhang Y, Chen Y, Li Y, et al. The ORF8 protein of SARS-CoV-2 mediates immune evasion through down-regulating MHC-Ι. Proc Natl Acad Sci, USA. 2021 Jun 8;118(23): doi: 10.1073/pnas.2024202118
- Yoshihama S, Roszik J, Downs I, et al. NLRC5/MHC class I transactivator is a target for immune evasion in cancer. Proc Natl Acad Sci, USA. 2016 May 24;113(21):5999–6004.
- Chelbi ST, Guarda G. NLRC5, a promising new entry in tumor immunology. J Immunother Cancer. 2016;4(1):39. doi: 10.1186/s40425-016-0143-z
- Yoshihama S, Vijayan S, Sidiq T, et al. NLRC5/CITA: a key Player in cancer immune surveillance. Trends Cancer. 2017 Jan;3(1):28–38. doi: 10.1016/j.trecan.2016.12.003
- Narayanan A, Narwal M, Majowicz SA, et al. Identification of SARS-CoV-2 inhibitors targeting Mpro and PLpro using in-cell-protease assay. Commun Biol. 2022 Feb 25;5(1):169.
- Liu H, Iketani S, Zask A, et al. Development of optimized drug-like small molecule inhibitors of the SARS-CoV-2 3CL protease for treatment of COVID-19. Nat Commun. 2022 Apr 7;13(1):1891.
- Al-Karmalawy AA, El-Gamil DS, El-Shesheny R, et al. Design and statistical optimisation of emulsomal nanoparticles for improved anti-SARS-CoV-2 activity of N-(5-nitrothiazol-2-yl)-carboxamido candidates: in vitro and in silico studies. J Enzyme Inhib Med Chem. 2023 Dec;38(1):2202357. doi: 10.1080/14756366.2023.2202357
- Jan JT, Cheng TR, Juang YP, et al. Identification of existing pharmaceuticals and herbal medicines as inhibitors of SARS-CoV-2 infection. Proc Natl Acad Sci, USA. 2021 Feb 2;118(5): doi: 10.1073/pnas.2021579118
- Fuzimoto AD, Isidoro C. The antiviral and coronavirus-host protein pathways inhibiting properties of herbs and natural compounds - additional weapons in the fight against the COVID-19 pandemic? J Tradit Complement Med. 2020 Jul;10(4):405–419. doi: 10.1016/j.jtcme.2020.05.003
- Gao LQ, Xu J, Chen SD. In silico screening of potential Chinese herbal medicine against COVID-19 by targeting SARS-CoV-2 3CLpro and Angiotensin converting enzyme II using molecular docking. Chin J Integr Med. 2020 Jul;26(7):527–532. doi: 10.1007/s11655-020-3476-x
- Chang YC, Yang CF, Chen YF, et al. A siRNA targets and inhibits a broad range of SARS-CoV-2 infections including Delta variant. EMBO Mol Med. 2022 Apr 7;14(4):e15298.
- Phan T. Genetic diversity and evolution of SARS-CoV-2. Infection, genetics and evolution: journal of molecular epidemiology and evolutionary genetics in infectious diseases. Infect Genet Evol. 2020 Jul;81:104260. doi: 10.1016/j.meegid.2020.104260
- Pillay TS. Gene of the month: the 2019-nCov/SARS-CoV-2 novel coronavirus spike protein. J Clin Pathol. 2020 Jul;73(7):366–369. doi: 10.1136/jclinpath-2020-206658
- Walls AC, Park YJ, Tortorici MA, et al. Structure, function, and antigenicity of the SARS-CoV-2 spike glycoprotein. Cell. 2020 Apr 16;181(2):281–292 e6.
- Wu C, Liu Y, Yang Y, et al. Analysis of therapeutic targets for SARS-CoV-2 and discovery of potential drugs by computational methods. Acta Pharm Sin B. 2020 May;10(5):766–788. doi: 10.1016/j.apsb.2020.02.008
- Medeiros IG, Khayat AS, Stransky B, et al. A small interfering RNA (siRNA) database for SARS-CoV-2. Sci Rep. 2021 Apr 23;11(1):8849.
- Saadat K. RNAi-mediated siRNA sequences to combat the COVID-19 pandemic with the inhibition of SARS-CoV2. Gene Rep. 2022 Mar;26:101512. doi: 10.1016/j.genrep.2022.101512
- Li JY, Liao CH, Wang Q, et al. The ORF6, ORF8 and nucleocapsid proteins of SARS-CoV-2 inhibit type I interferon signaling pathway. Virus res. 2020 Sep;286:198074.
- Lei X, Dong X, Ma R, et al. Activation and evasion of type I interferon responses by SARS-CoV-2. Nat Commun. 2020 Jul 30;11(1):3810.
- Xia H, Cao Z, Xie X, et al. Evasion of type I interferon by SARS-CoV-2. Cell Rep. 2020 Oct 6;33(1):108234.
- Miorin L, Kehrer T, Sanchez-Aparicio MT, et al. SARS-CoV-2 Orf6 hijacks Nup98 to block STAT nuclear import and antagonize interferon signaling. Proc Natl Acad Sci, USA. 2020 Nov 10;117(45):28344–28354.
- Addetia A, Lieberman NAP, Phung Q, et al. SARS-CoV-2 ORF6 disrupts bidirectional nucleocytoplasmic transport through interactions with Rae1 and Nup98. MBio. 2021 Apr 13;12(2): doi: 10.1128/mBio.00065-21
- Gordon DE, Jang GM, Bouhaddou M, et al. A SARS-CoV-2 protein interaction map reveals targets for drug repurposing. Nature. 2020 Jul;583(7816):459–468. doi: 10.1038/s41586-020-2286-9
- van de Leemput J, Han Z. Understanding individual SARS-CoV-2 proteins for targeted drug development against COVID-19. Mol Cell Biol. 2021 Aug 24;41(9):e0018521.
- Zhu JY, Lee JG, van de Leemput J, et al. Functional analysis of SARS-CoV-2 proteins in Drosophila identifies Orf6-induced pathogenic effects with selinexor as an effective treatment. Cell Biosci. 2021 Mar 25;11(1):59.
- Tripathi PP, Arami H, Banga I, et al. Cell penetrating peptides in preclinical and clinical cancer diagnosis and therapy. Oncotarget. 2018 Dec 14;9(98):37252–37267.