ABSTRACT
Introduction
Understanding the role of efflux pumps in biofilm resistance provides valuable insights for developing effective therapeutic strategies. Drugs designed for targeting efflux pumps in drug design holds promise for combating biofilm-related infections. Nanoparticles offer unparalleled advantages in designing drugs targeting efflux pumps.
Areas covered
This review rigorously examines the existing body of knowledge on the prospective targeting of efflux pumps using metal-based nanoparticles. It includes and analyses the pertinent research findings sourced from the PubMed and SciFinder databases. It covers the experimental studies on efflux inhibition by nanoparticles and provides detailed analyses of their mechanisms of action, elucidating their interactions with the efflux system and their influence on biofilm formation and persistence.
Expert opinion
The potential of nanoparticles to act as potent antibacterial agents through efflux pump inhibition remains tantalizing, although hindered by limited mechanistic understanding. From the burgeoning research landscape nanoparticles emerge as a novel direction for shaping antimicrobial drug design. Notably, beyond their contribution to drug resistance, efflux pumps play a pivotal role in biofilm development. The deliberate disruption of these pumps can effectively reduce biofilm adhesion and maturation. More details however are needed to exploit this potential.
1. Introduction
Biofilm formation is a persistent problem in medicine and the design of antimicrobial drugs and disinfecting agents. According to the most recent estimates, up to 80% of chronic and recurrent infections in humans are caused by biofilms [Citation1], accounting for 3 million infections and 50 000 deaths yearly in the European Union, amounting to annual expenses of $5 billion [Citation2]. Biofilms can develop on literally all medical devices, including catheters, pacemakers and contact lenses, as well as internal and superficial parts of the body, such as gut, urogenital tract, ear canal or mouth. From a physiological viewpoint, biofilm is considered a three-dimensional syntrophic consortium of microbial species (including archaea, bacteria and fungi) as well as a combination of various organisms. Among other traits, microbial swarms have an increased the ability to survive and evade new defense mechanisms. The key features that distinguishing biofilm from its planktonic form are the production of extracellular matrix, mutual interactions and quorum sensing (QS). Consequently, biofilm is morphologically and biochemically distinct from plankton forms of bacteria or fungi. Formation of biofilm requires a major phenotypic shift and changes in regulation patterns of multiple genes [Citation3]. These alterations may substantially increase the energy and metabolic demands for the synthesizing exopolysaccharides, adhesins and other extracellular polymeric substances (EPS) that induce the acquisition of beneficial properties, such as biochemical armor, longevity and cooperative response [Citation4]. This is one of evolutionary strategies that most effectively facilitates colonization in particularly harsh environments, such as hot springs, brine pools or glaciers. One of the most important features of biofilms is their ability to isolate from external biocidal factors while maintaining selective uptake of nutrients. Although biofilm formation is associated mainly with adhesion and strengthening of the intracellular matrix, liberation of planktonic cells is still achieved by this form, ensuring a high virulence potential. Thus, from the medical viewpoint, biofilm occurrence remains a serious common problem in device-derived or nosocomial infections, particularly chronic infections, and is not limited to immunocompromised patients [Citation5]. Polymicrobial biofilms (an assembly of more than one bacterial species or a combination of bacteria and fungi) are responsible for the majority of chronic wound infections [Citation6]. Biofilm formation in chronic wounds is associated with increased resistance to treatment, and even modern approaches consisting of debridement of tissue, balancing moisture and regenerating living tissues, known as TIME, are not fully effective [Citation7]. Biofilms attach firmly to the wound basement and may be invisible at the initial steps, as evident from bioluminescence studies [Citation8]. Finally, the nature of biofilm formation makes it extremely stable and adaptable. The process of generating polymicrobial biofilms is complex and includes priority effects (in the colonization timeline), competition between species and commensal interactions [Citation9]. Interestingly, biofilm formation was initially defined and characterized only 40 years ago, and the underlying process remains largely unexplored. A significant hallmark of biofilm is a thick layer of EPS that protects the infecting microbial population from the immune system and antimicrobials. A fully developed biofilm displays a characteristic structure, including a polymeric coat and water channels responsible for the transport of nutrients and waste. Another feature of fully developed biofilm is quorum sensing achieved by small molecules and peptides, which can be observed in sufficiently dense colonies () [Citation6].
Figure 1. Development of biofilm and its perseverance as a source of new planktonic forms of microorganisms. Maturation of biofilm is associated with development of the extracellular matrix and increasing participation of small-molecule substances as signaling molecules. Increased density in the deeper layers of biofilm results in lower nutrient availability in turn, causing senescence of insider cells (denoted as gray).
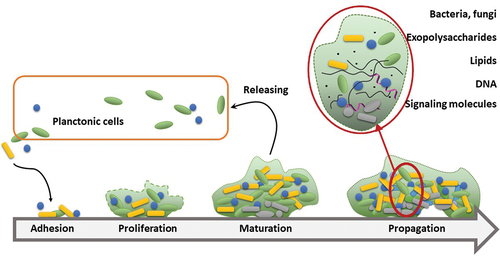
The recent emergence of the novel fungal pathogen species Candida auris has been of significant interest to the scientific and medical community worldwide. C. auris was described for the first time in 2009 in Japan and subsequently rapidly identified in America (2013) and Europe (2016) [Citation10]. This pathogen spreads particularly efficiently in hospitals due to multidrug resistance. It has a strong ability to form biofilm and is reported to be up to hundreds of times more resistant to antifungals than planktonic cells [Citation11]. The fungi can colonize human skin and co-exist with other biofilm-forming microorganisms. Biofilms formed by C. auris considerably alter individual susceptibilities of constituent species, as noted for Staphylococcus aureus in terms of its resistance to vancomycin [Citation12,Citation13]. Moreover, while the biofilm is manageable with typical antiseptics such as chlorhexidine or iodine, its recovery after that is relatively quick, particularly following treatment with hydrogen peroxide.
One possible origin of this highly invasive and multidrug resistant fungal pathogen is wetland species that have adapted to higher temperatures and more saline environments during climate change, as observed for Cryptococcus, Fusarium and Coccidioides spp [Citation10,Citation14]. Regardless of the factual strength of this hypothesis, climate change and globalization continue to impact microorganisms in our environment and microbiomes significantly. Although research in this area is relatively scarce, this effect could lead to substantial changes in the situation in the post-antibiotic era. Crossing the environmental barriers enriches the genomic resource of our microbiome, which may further promote the emergence of resistant organisms. Therefore, a systemic approach is essential to regain effective control over microbial infections, including changes in the management of antibiotic usage and identification of novel drugs, particularly those effective against resistant species and biofilms. In the latter case, the development and implementation of novel strategies are crucial, as the nature of biofilm formation underlies resistance, even if the component microorganisms are susceptible to therapeutic agents at the planktonic stage.
2. Differential efflux in biofilms
An important aspect of biofilm formation and increased antibiotic resistance is associated with efflux pumps (EP). In planktonic forms of bacteria or fungi, these proteins are responsible for the excretion of metabolites and toxic substances, including exogenic antibiotics. However, in biofilm, more complex functions have been determined, including efflux of quorum-sensing molecules and extracellular polymeric substances, indirect regulation of genes in other microorganisms and management of aggregation, which, in turn, can be achieved by increasing or decreasing adhesion [Citation15]. Numerous reports have shown that reducing efflux pump proteins expressions can decrease or prevent biofilm formation in various bacterial strains [Citation16–18]. Notably, the weakening of biofilm can be achieved by indirect effects such as QS disruption [Citation19].
Efflux pumps are divided into five major groups, according to their mechanism of extrusion (). The ATP-binding cassette superfamily (ABC) uses energy from ATP to extrude toxic substances; it is related to multidrug-resistant glycoprotein in eukaryotic cells. Other superfamilies exploit the electrochemical gradient as a driving force. The major facilitator superfamily (MFS), resistance-nodulation-cell division superfamily (RND) and small multidrug superfamily (SMR) use the proton-motive force, while members of the multidrug and toxic compound extrusion superfamily (MATE) are typical sodium- or proton-substrate antiporters. Several reviews comprehensively document the structure, function, and other aspects of efflux pumps in bacteria [Citation20,Citation21].
Figure 2. Efflux pumps are organized into a complex system based on contribution to different processes, substantially extending their role beyond simple facilitation of the extrusion process. Known superfamilies with exemplary proteins have been given.
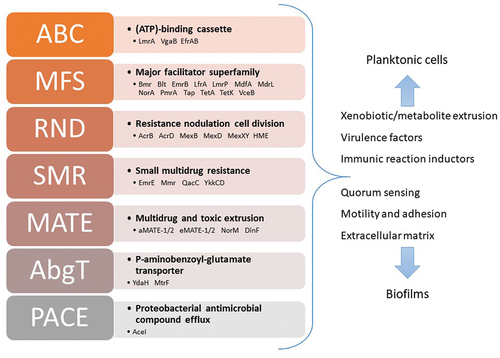
Given these considerations, several issues need to be addressed. Efflux pumps are typically considered xenobiotic- and metabolite-extruding membrane proteins responsible for drug resistance. Although potentially toxic substances can be generated during metabolism and absorbed from the environment, this hypothesis is not entirely convincing. Firm selective pressure has been developed with habitats packed with antibiotics over the last 50 years. However the scope and time of this pressure are limited, causing it to lose its significance from an evolutionary standpoint, even for microorganisms. The efflux pumps evolved long before the antibiotic era. Meanwhile, antibiotic-producing microorganisms colonize similar areas and are a potential source of pressure for selecting drug resistance features. Indeed, drug resistance mechanisms are common in bacterial species, besides the efflux process, include alteration of the drug target, enzymatic degradation of the drug and decrease in membrane permeability [Citation22]. Drug- and multi-drug resistant bacteria naturally occurring in non-anthropogenic ecosystems do not always exploit efflux pumps as a mechanism for survival [Citation23]. In this light, evolutionary stress factors cannot explain the abundance of extruding proteins. Notably, the number of EPs is constant for various species, i.e. within 3–5 concerning 100 kilobases of the bacterial genome [Citation24,Citation25]. A convincing proposal has been presented by Ryan et al. [Citation24] based on the premise that the number of proteins actually involved in drug efflux is substantially lower than the total number of efflux systems in the bacterial genome. Therefore, this phenomenon requires further exploration, which should progressively change our current interpretation of the role and functionality of efflux proteins. Interaction with the environment is one of the more feasible factors that may promote efflux systems in bacteria. The bacterial species that contain more significant numbers of genes encoding EPs often colonize natural environments, such as plants or soil. The extrusion of siderophores [Citation26], toxic alkaloids, and even symbiotic interactions are achieved by efflux systems in bacteria [Citation27,Citation28]. The efflux system’s key role is the extrusion of heavy metals abundant in numerous environments colonized by bacteria. Membrane proteins expel a broad range of substrates, including Cd2+, Co2+, Cu2+, Hg2+, Ni2+, Pb2+, Sb3+ and Zn2+ [Citation29,Citation30]. The metal resistance system also includes cytoplasmic metallochaperones transferring the substrate to membrane proteins and enzymes that convert ions such as AsO43- to less toxic AsO33- [Citation31,Citation32]. Direct transport of metal ions through EPs has been demonstrated, particularly for proteins belonging to the RND family. For example, Long et al. [Citation33] reported the crystal structure of the CusA pump responsible for the extrusion of Cu+ and Ag+. This is the first example of a heavy metal efflux (HME) subfamily belonging to the RND family of proteins.
HME proteins can bind to metal ions from the cytoplasm and periplasm. The periplasmic cleft is closed without ions and opens in the presence of Cu(I) and Ag(I). The initial steps of extrusion are potentially activated when metal ions enter the three-methionine (M672-M573-M623) binding site directly through the periplasmic cleft (as denoted in ) or via the methionine pairs (M410-M501, M486-M403, M391-M1009) below in the transmembrane region [Citation34]. The final extrusion of ions occurs through the periplasmic funnel located above the M271-M755 pair.
Figure 3. Schematic representation of the metal-transporting CusA protein. Methionine triads and pairs responsible for transport of ions are circled in red. The amino acids forming the conserved region of internal cleft are also presented.
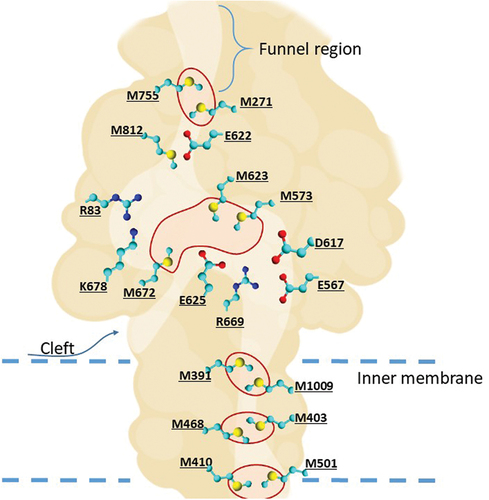
Further research in this field is essential to elucidate the interactions between metal homeostasis and extrusion mechanisms and uncover more examples of the HME subfamily. This robust system controlling toxic ions is responsible for bacterial extremophiles’ success in colonizing environments that can be extremely harmful to other organisms [Citation35]. This is also an exceptionally promising target for metal-based antibacterial agents. Among these, metallic or metal-based nanoparticles are particularly promising regarding their ability to facilitate the prolonged release of metal ions and interact with cellular proteins.
The extraordinary ability of biofilms to survive in both physically and chemically harsh environments is tightly associated with so-called persister cells (). These comprise only a tiny fraction of the bacterial population and are characterized by very slow metabolism and proliferation [Citation36]. The composition of the biofilm matrix may explain their existence. In deeper layers of dense biofilm, the limited availability of nutrients results in lowered metabolism of cells. Another essential factor of this regulation is acquiring quorum sensing ability [Citation37]. Moreover, the efflux system of these cells is considerably more robust than that of their more typical neighbors. Based on their low proliferation and decreased genetic expression characteristics, these cells should be considered relatively tolerant to antibiotics. However, more sophisticated roles of EPs have been recently uncovered, at least in E. coli. Overexpression of AcrAB-TolC protein is associated with lower expression of the gene repair system [Citation38]. Therefore, bacteria escaping drug action via an elevation of efflux pumps expression acquire mutation capability. Another hypothesis is that the combination of horizontal gene transfer and the ability to persist in the presence of antibiotics constitutes an essential new strategy for biofilm survival [Citation39]. Thus, the efflux systems incorporating extruding proteins expressed in adjacent cells combined with metabolic and genetic interplay in response to environmental stress are the main strengths of biofilm. Notably, the same factors are at least partially responsible for passive defense systems of biofilm, specifically, density and permeability. Recently, Van Acker and Coenye described the influence of EPs on the adaptation of biofilm to environmental changes [Citation40]. The density is closely related to biofilm susceptibility to biocides and other auxiliary agents that can be utilized to eradicate infection. Consequently, combinations of antibiotics with EP inhibitors may be less effective for biofilms than planktonic forms, regardless of their synergistic interactions [Citation41]. Improved results can be achieved with compounds able to increase membrane permeability, such as polymyxin B, according to Ferrer-Espada et al [Citation42]. This observation has some application potential evident when considering the relative toxicity of Eps inhibitors and polymyxin B to humans. Meanwhile, as shown by Kvist et al. [Citation43], blocking the efflux system has a detrimental effect on biofilm formation ability.
The complexity and adaptability of biofilm necessitate special requirements for treatment strategies and drug design. Impaired penetration affects the activities of not only typical antibiotics but also adjuvants and disinfection agents. Coincidentally, prolonged contact with biofilm may be detrimental regarding drug effects due to enzymatic or oxidative decomposition. Therefore, nanoparticulate drugs may have considerable therapeutic value because of their improved stability and penetrability.
3. Nanoparticles as efflux pump inhibitors
Unlike small-molecule antimicrobial drugs, the biological activity of nanoparticles depends on their preparation, composition and internal chemical structure [Citation44]. Consequently, the design of antimicrobial nanoparticle is more complex and complicates intellectual property issues, but also allows fine-tuning for their application. In a typical situation, the preparation involves several factors such as reducing agents, temperature, solvents and other parameters that can significantly change the NPs’ physicochemical properties, leading to loss of desirable activity. Moreover, NPs can be coated and modified to enhance their permeability or targeting ability, further increasing their application potential. Based on their nature, NPs are classified as organic, inorganic or organic/inorganic composites. The NP type often determines the production process since organic NPs are typically built up from scratch as polymers, liposomes and micelles. At the same time, inorganic particles are mainly metallic and can be obtained in chemical processes from solutions or ground down to the desirable dimensions, which may additionally affect the activity and potential applications. Organic liposome encapsulation is typically exploited to alter the pharmacokinetic parameters of drugs [Citation45]. In contrast, metallic NPs are generally synthesized to provide biological activity without further modifications, as comprehensively shown for silver nanoparticles [Citation46]. In general, metallic nanoparticles have been studied in significantly more detail, particularly in the antimicrobial agents field. Metallic oxides are materials of choice for various NPs, including zinc, copper, and iron, among others. These nanoparticles can be used in combination therapies with antibiotics as organic molecules that easily attach to the metal oxide surface [Citation47]. Another significant aspect of metal and metal oxide NPs is photothermal and photodynamic activities that facilitate further extension of their applications, particularly in the antimicrobial field. Although the activities of NPs against various pathogenic bacteria and fungi have been broadly investigated, their application in the field of biofilms requires further exploration.
Metal oxide nanoparticles have been more comprehensively explored than other materials. Their synthetic availability and modification possibilities and knowledge of their physicochemical properties are particularly advantageous for projects focused on drug design. Zinc oxide is a known semiconductor used for ferromagnetic induction and thus has utility in material science. On the other hand, it can be doped with transition metals to confer photothermal and photocatalytic properties useful in antimicrobial [Citation48] and anticancer [Citation49] applications. In a study by Iqbal et al. [Citation50], an inhibitory effect of cobalt-doped ZnO nanoparticles on EPs was reported. NPs were synthesized from a homogenous mixture of Co and Zn nitrates, and coated with various concentrations of thiolated chitosan. Although coating of ZnO did not affect photothermal activity, substantially improved activity against MRSA was achieved. However, antimicrobial tests were not designed for biofilms and efflux inhibition was determined via the ethidium bromide (EtBr) accumulation assay. These findings were validated in another study focusing on Zn-NPs functionalized with glutamic acid and thiosemicarbazide (TSC) [Citation51]. In this work, nanoparticles were obtained in the presence of glutamic acid and conjugated with TSC. Testing of the resulting Zn-NPs against a panel of multidrug resistant S. aureus isolates, and a reference strain revealed average to good antibacterial activity. Moreover, functionalization with TSC induced a substantial increase in activity. In particular, synergistic interactions between Zn-NPs and TSC confirmed a membrane-oriented mechanism of action of NPs and showed desirable activity profiles against biofilm. As suggested by the authors, chelation of essential metal ions in the bacterial membrane with TSC could increase NP permeability. Thus, a combination of promising antibacterial effects of functionalized NPs may facilitate their utility in anti-biofilm applications. The potent inhibitory activity against efflux pumps confirmed in EtBr tests and observed effects on efflux-related gene expression reinforce this hypothesis and highlight the necessity for more comprehensive research. A number of reports on the anti-biofilm activity of Zn-NPs have been published. A detailed study by Lee and coworkers showed that ZnO-NPs, even at non-bactericidal concentrations, exert an anti-biofilm effect by interfering with several mechanisms, including the efflux system [Citation52]. Their studies were designed with P. aeruginosa as a target, which is known for its tendency to form biofilms with increased resistance to antibiotic and antiseptic agents [Citation53]. The robust efflux system and less permeable membrane of this microbe render it more resistant to heavy metal ions. When subjected to sub-lethal doses of ZnO-NPs, P. aeruginosa responded with elevated synthesis of EP proteins but simultaneously produced fewer QS molecules, such as pyocyanin, pyochelin and Pseudomonas quinolone signals (PQS). Pyocyanin is a red-blue phenazine derivative responsible for biofilm development and protection from other bacteria. Due to its ability to generate ROS and cross the membrane, pyocyanin has been characterized as a primary toxic metabolite of P. aeruginosa and a virulent factor. Therefore, inhibition of its secretion is particularly interesting from the drug development perspective.
Khare et al. have reported similar findings regarding gold NPs coated with chitosan and embelin [Citation54]. Embelin, a p-benzoquinone derivative naturally present in Embelia ribes berries, possesses broad biological activities, including anticancer, antioxidative and antiparasitic properties [Citation55]. NPs were obtained by stepwise coating Au-NPs with chitosan and crosslinking the layer with embelin. The resulting molecules were tested against strains of multidrug resistant P. aeruginosa and E. coli in relation to the activities of ciprofloxacin and embelin. The fractional inhibitory concentration method was utilized to evaluate drug-drug interactions between ciprofloxacin and embelin as well as NPs. In both cases, a synergistic interaction was observed, with a slightly stronger effect against P. aeruginosa. This finding suggests that embelin itself can inhibit the efflux pump, and restore the susceptibility to antibacterial quinolones. This hypothesis was confirmed via ethidium bromide accumulation and molecular docking with multiprotein systems involved in multidrug resistance in the bacterial strains investigated. Interestingly, embelin has robust photocatalytic potency and can be successfully exploited as a sensitizer in photodynamic therapy (PDT) [Citation56]. Although this feature was not investigated in the above study, it may provide an interesting direction for future research. Gold NPs coated with an additional layer designed to provide supplementary activity have been described in the literature. However, to date, only a few examples of nanoparticle targeting or testing against efflux pumps are documented, and further research on this interesting field is warranted. For example, the essential oil of Nigella sativa has been used as a bioactive coat in Au-NPs to confer active anti-biofilm and anticancer activity [Citation57]. The exceptionally broad biological activities of N. sativa extracts or their components included anti-MDR and efflux inhibition effects, which should be explored further [Citation58,Citation59]. A particularly interesting aspect of the current review is the work of Khosravi et al. [Citation60] on Au-NPs obtained by reduction with extracts from Anthemis atropatana. The resulting NPs were examined against a panel of clinical Klebsiella pneumoniae isolates, including biofilm-forming and MDR strains. Overexpression of efflux proteins was additionally confirmed in the study. Importantly, although extracts from Anthemis plants are known for their antibacterial activity against various microorganisms, an anti-biofilm effect could be observed with essential oils, which was generally weaker than that against the planktonic form [Citation61]. The available information does not cover K. pneumoniae, no efflux pump inhibitory activity for this pathogen has been reported. NPs obtained in this study also showed antibacterial activity against biofilm and MDR strains. The mechanism of action appeared to be associated with the formation of ROS, although inhibition of efflux was additionally confirmed in EtBr tests. The anti-biofilm activity of Au-NPs, including an anti-slime effect and inhibition of exopolysaccharide formation at sub-MIC concentrations, was further validated. Notably, this work focused on uncoated metallic NPs. However, the production process allowed for the residual presence of active ingredients from A. atropatana, which was confirmed with FT-IR analysis. The NPs generated were washed and dried before use in biological tests. A similar method of synthesis was employed for Ag-NPs with antibacterial activity. However, no biofilm formation or efflux pump inhibition was examined in this experimental schedule [Citation62]. Medlar (Mespilus germanica) extract has been used for synthesize Ag-NPs that are active against the biofilm of K. pneumoniae [Citation63]. The QS pathway was examined by quantifying the expression of luxS and mrkA genes involved in this vital communication system. These genes, particularly luxS, are also responsible for the expression of efflux pump proteins that play a crucial role [Citation64]. This hypothesis was further supported by data published by Behdad et al. [Citation65] on Acinetobacter baumannii, a gram-negative bacterium that naturally exists in soil but causes opportunistic infections in humans. This bacterium is also known for its rapidly emerging resistance due to overexpression of efflux proteins. Ag-NPs were obtained by reduction with ethanolic extract, and antibacterial tests, including EtBr, confirmed their activity in efflux pump inhibition. Moreover, an analysis of a series of genes responsible for efflux-related resistance was performed to clarify the mechanisms of action further.
Although antimicrobial activities of NPs constructed with other metals have been frequently reported, surprisingly little is known about their impact on biofilm-oriented targets, particularly the efflux system. Only one report on TiO2-based NPs is available in the literature [Citation66]. Fortunately, this work is unusually comprehensive, including the detailed examination of effects against a broad panel of P. aeruginosa isolates and the discussion of the plausible mechanisms of action. In this study, TiO2-NPs were first synthesized via the sol-gel method and tested against a panel of bacteria, including drug-resistant strains. Interestingly bulky TiO2 exerted little or no activity against those strains. Subsequently, anti-biofilm activity as well as synergistic interactions with antibiotics, were examined. The results clearly revealed a negative impact of TiO2-NPs on the efflux system in bacteria. PCR experiments showed TP-induced downregulation of efflux- and QS-related genes.
Unlike zinc or gold, copper and iron ions can change their oxidation state because of a low energetic barrier. Therefore in biological environments they may provide interesting redox profiles. This effect is more potent in complexes and may be exploited to design novel antibacterial and anticancer drugs [Citation67,Citation68]. This feature may also have a significant advantage in anti-biofilm nanoparticles, as all metallic NPs generally generate ROS through releasing ions or as a cellular response to toxic agents. In this regard, copper is particularly interesting, as its ions exert a strong antibacterial effect and are preferentially excreted by efflux pumps, lowering the overall efficacy of the discharge system. Therefore, redox active metals with multitargeted mechanisms of action serve as desirable anti-biofilm agents. Christena et al. put forward a similar theory in a study testing Cu-NPs against P. aeruginosa and S. aureus [Citation69]. Although activity against biofilms of these bacteria was relatively moderate exceeding 100 μM, sublethal doses (0.5 or 0.25×MIC) were effectively inhibited efflux pumps. Interestingly, this activity was exerted only partially by Cu ions and partially by NPs, as confirmed with EDTA experiments. Moreover, in the presence of Cu-NPs, the bacterial membrane was permeabilized, especially in the case of S. aureus. Activities such as synergistic effects with antibacterial agents (4-fold in the case of ciprofloxacin) and disruption of the efflux system further paved the way for overcoming drug resistance of MRSA using those nanoparticles. These results were in good agreement with a former study on E. coli that confirmed the importance of NP decomposition and liberation of Cu ions [Citation70]. Similarly, the generation of ROS and degradation of proteins and lipids, as well as a dissipative effect on the cell membrane, were suggested in this report. Importantly, these proposals are based on (at least partial) liberation and solubilization of Cu-NPs.
Iron-based NPs have been broadly evaluated for their biological activities, including anti-biofilm properties. These nanoparticles are particularly valuable for research due to simple preparation, replicative properties and various potential applications due to magnetic, thermal and Fe ion release effects. However, their impact on efflux pumps has not been clarified to date. Although the mechanisms and effects described so far suggest that the efflux system is one of the targets of Fe-NPs, this hypothesis requires proof through further comprehensive investigations. For example, the ability of superparamagnetic Fe-NPs [Citation71] and iron oxyhydroxides [Citation72] to enhance antibiotic activity and disrupt biofilm structure has been reported. The thermal approach has also been described for iron oxide NPs coated with poly(ethylene glycol) acrylate [Citation73]. In this work, the combination with gemcitabine improved therapeutic response without inducing hyperthermal activation, while subsequent experiments on cellular mechanisms supported the hypothesis of disruption of the efflux system.
Interesting results have been published by Khalid et al. [Citation74] on iron oxide NPs coated with rhamnolipid surfactant. Rhamnolipids are a class of biosurfactants produced by P. aeruginosa involved in various mechanisms affecting virulence, including the efflux system [Citation75,Citation76]. In this study, iron oxide and silver NPs were synthesized, coated with rhamnolipid, and tested for activity against P. aeruginosa and S. aureus biofilms. In addition to the expected activities, both NPs reduced adhesiveness and formation of the EPS matrix, suggesting at least an indirect influence on the efflux system.
The general mechanism of action of metallic NPs is associated with ion release. The same is responsible for the toxicity and eco-toxicity of NPs based on metals or their oxides and salts, regardless of the toxicity of metals in the bulk form. This phenomenon has also been detected in noble metals, such as Ag, Au and Pt [Citation77]. The effect has been studied in silver to a greater extent than in other metals, probably due to the historical use of silver as an anti-bacterial agent. Analysis of the kinetics of the antibacterial activity of Ag-NPs supports these observations, as ion release plays a crucial role. The ROS-generating phase needs a greater load of ions than the basic antimicrobial level, as described for drug-resistant Burkholderia pseudomallei [Citation78]. The stable coating on NPs may hamper or prevent ion release, although special care should be taken to analyze the fate of nanoparticles in vivo, as some coatings may not be stable enough in acidic or enzymatically active compartments such as lysosomes or endosomes. This instability may affect activity, as ions of several transition metals are crucial for bacterial virulence. Similar effects may be observed in NPs with metals that exert only a toxic effect on microorganisms, such as silver. Mishra et al. reported polysaccharide-capped Ag-NPs that were more active than simple uncovered NPs [Citation79]. However, ion release was not indirectly determined the NPs appeared stable. Consequently the expression of efflux pumps did not affect their activity. It should be noted here that the experiments focused on measuring protein expression (ArcA/B and TolC), and therefore, the potential inhibitory effects on these pumps remain unclear.
4. General remarks on the mechanism and perspectives
Depending on their biological roles, metals are divided into two main groups, biologically relevant or micronutrients (such as Fe, Zn, Mn, Cu) and toxic/heavy metals. However, all metal ions in excess exert toxicity via multiple mechanisms, including generating reactive oxygen species and membrane and protein damage () [Citation80,Citation81]. Excessive supply of metal ions can impair cell proliferation and induce the defense strategy [Citation82]. Bacteria have evolved mechanisms for tight regulation of ions from the first group. The irrelevant metal ions are expelled and their metabolism relies solely on this process. Regardless of the metal group, safe levels of all ions are systematically maintained, a process in which efflux pumps play a key role. Several sound reviews have documented the impact of elevated levels of metals on bacterial virulence and survival in the host-pathogen setting [Citation83,Citation84].
Figure 4. Multitargeted activities of nanoparticles. Liberated metal ions interact with proteins, membrane or DNA (1). Their excess is extruded by efflux pumps (2). Redox active metals generate ROS responsible for damage of membrane and DNA (3) and potentially interact with quorum-sensing molecules (4) or membranes (5). Similar mechanisms are observed in biofilm matrix, whereby liberated ROS attack QS molecules and the polysaccharide matrix, increasing the permeability of the biofilm.
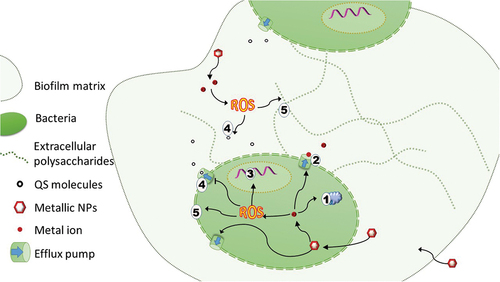
This complexity provides clues on the therapeutic significance of metal acquisition and extrusion systems in bacteria. Animal tissues have evolved a strategy of iron depletion to control the invasiveness of pathogenic microbes. Therefore, the evolutionary force for an effective iron uptake system in pathogenic bacteria is as strong as the efflux system for this element. In contrast, elevated levels of other metals, predominantly copper, are used as an active force in nutritional immunity [Citation85]. This strategy is utilized by phagocytes and plays a key role in innate immunity [Citation86,Citation87]. Interestingly, several similarities exist between bacterial and eukaryotic cells regarding metal homeostasis and excretion. Ions of heavy metals, such as cadmium or mercury, can also serve as substrates for P-glycoprotein or multidrug resistance proteins in human cells [Citation88]. However, from an evolutionary perspective, the activity of P-glycoprotein in extruding Cd ions is controversial. Earlier reports suggest that metalorganic compounds act through the efflux system to reverse drug resistance in cancer cells [Citation89]. Moreover, natural resistance-associated macrophage proteins (Nramps) are responsible for extruding metal ions from the phagosomal lumen [Citation90]. These findings highlight the importance of proper management of metal concentration in all biological systems, underlying growth and antagonism between organisms.
5. Conclusions
The biological roles of efflux pumps extend beyond extrusion processes, encompassing interactions with the environment, output for information and strong evolutionary potential for resistance to antibiotics. Unfortunately, no EP inhibitors have been approved for treatment of bacterial infections to date. In the case of biofilms, the problem of combating the efflux system is an even more significant challenge. Metal-based nanoparticulate agents appear especially promising based on their high activity, relatively low toxicity to host organisms and usage for environmental purposes. Progressive decomposition with the release of metal ions ensures longer activity and biodegradation in waste or the environment. A range of valuable metals and compositions pave the way for more rational design based on the mechanisms of action and pharmacokinetics of NPs. In this regard, ornamentation of nanoparticles with specific molecules, such as DNA/RNA fragments, enzymes or antibiotics, facilitates the design of multimodal drugs and the improvement of the crucial parameters of stability or permeability. A multitargeted mechanism of action is the main benefit of metallic NPs as anti-biofilm agents. The generation of ROS and direct as well as indirect interference with efflux systems effectively serve as a double-edged sword against bacterial biofilms. On the other hand, the current lack of understanding of the holistic activity of efflux proteins hampers the introduction and development of new drugs.
6. Expert opinion
Efflux pumps are essential targets of antimicrobial drugs due to their contribution to antibiotic resistance. Despite intensive research, no drugs have been approved as EP inhibitors, although several other common drugs could possibly be repurposed [Citation91]. EPs play a critical role in biofilm formation, including adhesion, generation, and development of the extracellular matrix, as well as extrusion of quorum-sensing molecules. The efflux system is also a crucial control element for persistent cells, i.e. senescent cells in deeper layers of biofilm responsible for resistance and regeneration.
Further detailed and mechanistic investigations are essential to understand the potential of metallic NPs as anti-biofilm agents on desirable level. This knowledge is necessary to reveal plausible obstacles and hazards associated with nanosized anti-biofilm agents and ensure a more rational design of effective drugs. Antimicrobial nanoparticles are also susceptible to the development of resistance [Citation92]. Interestingly, this may involve different pathways and effectors to small-molecule antibiotics. Naturally, specific microorganisms habitat in lakes or soils rich in metals are evolutionarily capable of surviving levels that may be harmful to other bacteria. For example, biofilms of Shewanella oneidensis and Cupriavidus metallidurans have been shown to develop resistance against metallic copper as well as Cu-NPs topped with TiO2 rapidly [Citation93–95]. These bacteria are irrelevant from a medical viewpoint, as no infections in humans have been reported so far. Nevertheless, the presence of genes responsible for resistance against NPs in the environment increases the risk of its emergence in other pathogenic organisms. In particular, S. oneidensis used in wastewater treatment and other environmental purposes or even the production of metallic nanoparticles [Citation96], may increase the risk of spreading resistance genes. Silver in various forms, including incorporation into nanoparticles, has the most extended history of exploitation for antibacterial activity, and its susceptibility to resistance has been extensively investigated [Citation97]. Panacek et al. [Citation98] recently reported interesting findings on resistance mechanisms to Ag-NPs in pathogenic bacteria. E. coli and P. aeruginosa promote aggregation of NPs by secretion of flagellin and fimbrial proteins responsible for bacterial mobility, invasion ability and biofilm formation and maturation [Citation99]. These findings show both the possible hazards and benefits achieved by targeting the efflux system with metallic nanodrugs. One considerable advantage of NPs is that these molecules can be easily modified in diverse ways to alter their stability and affinity to specific targets and introduce additional molecules with other functional activities.
The era of chaotic searching for NPs with beneficial activities is ending and future efforts should focus on an in-depth analysis of antimicrobial mechanisms. Insights into the underlying pharmacological mechanisms of action are crucial to provide practical breakthroughs in managing stubborn biofilm infections.
Article highlights
Biofilms constitute a severe medical problem, and their incidence is rising.
Efflux pumps are crucial not only for drug resistance but also for the development and maturation of biofilm
Efflux pumps are ideal targets for anti-biofilm agents
Nanoparticles based on metals may have inhibitory activity on efflux pumps
Declaration of interests
The authors have no relevant affiliations or financial involvement with any organization or entity with a financial interest in or financial conflict with the subject matter or materials discussed in the manuscript. This includes employment, consultancies, honoraria, stock ownership or options, expert testimony, grants or patents received or pending, or royalties.
Reviewer disclosures
Peer reviewers on this manuscript have no relevant financial or other relationships to disclose.
Additional information
Funding
References
- Schulze A, Mitterer F, Pombo JP, et al. Biofilms by bacterial human pathogens: clinical relevance - development, composition and regulation - therapeutical strategies. Microb Cell. 2021 Feb 1;8(2):28–56. doi: 10.15698/mic2021.02.741
- Bryers JD. Medical biofilms. Biotechnol Bioeng. 2008 May 1;100(1):1–18. doi: 10.1002/bit.21838
- An D, Parsek MR. The promise and peril of transcriptional profiling in biofilm communities. Curr Opin Microbiol. 2007 Jun;10(3):292–296. doi: 10.1016/j.mib.2007.05.011
- Lu H, Que Y, Wu X, et al. Metabolomics deciphered metabolic reprogramming required for biofilm formation. Sci Rep. 2019 Sep 11;9(1):13160. doi: 10.1038/s41598-019-49603-1
- Olivares E, Badel-Berchoux S, Provot C, et al. Clinical impact of antibiotics for the treatment of Pseudomonas aeruginosa biofilm infections. Front Microbiol. 2019;10:2894. doi: 10.3389/fmicb.2019.02894
- Clinton A, Carter T. Chronic wound biofilms: pathogenesis and potential therapies. Lab Med. 2015;46(4):277–284. Fall. doi: 10.1309/LMBNSWKUI4JPN7SO
- Schultz GS, Sibbald RG, Falanga V, et al. Wound bed preparation: a systematic approach to wound management. Wound Repair Regen. 2003 Mar;11 Suppl 1:S1–28.
- Rakotonirainy MS, Dubar P. Application of bioluminescence ATP measurement for evaluation of fungal viability of foxing spots on old documents. Luminescence. 2013 May;28(3):308–312. doi: 10.1002/bio.2382
- Cheong JZA, Johnson CJ, Wan H, et al. Priority effects dictate community structure and alter virulence of fungal-bacterial biofilms. Isme J. 2021 Jul;15(7):2012–2027. doi: 10.1038/s41396-021-00901-5
- Hofer U. Candida auris’ potential link to climate change. Nat Rev Microbiol. 2019 Oct;17(10):588. doi: 10.1038/s41579-019-0254-x
- Horton MV, Nett JE. Candida auris infection and biofilm formation: going beyond the surface. Curr Clin Microbiol Rep. 2020 Sep;7(3):51–56. doi: 10.1007/s40588-020-00143-7
- Gulmez D, Brown JL, Butcher MC, et al. Investigating dual-species Candida auris and staphylococcal biofilm antiseptic challenge. Antibiotics. 2022 Jul 11;11(7):931. doi: 10.3390/antibiotics11070931
- Kean R, McKloud E, Townsend EM, et al. The comparative efficacy of antiseptics against Candida auris biofilms. Int J Antimicrob Agents. 2018 Nov;52(5):673–677. doi: 10.1016/j.ijantimicag.2018.05.007
- Nnadi NE, Carter DA, Jabra-Rizk MA. Climate change and the emergence of fungal pathogens. PLOS Pathog. 2021 Apr;17(4):e1009503. doi: 10.1371/journal.ppat.1009503
- Alav I, Sutton JM, Rahman KM. Role of bacterial efflux pumps in biofilm formation. J Antimicrob Chemother. 2018 Aug 1;73(8):2003–2020. doi: 10.1093/jac/dky042
- Baugh S, Ekanayaka AS, Piddock LJ, et al. Loss of or inhibition of all multidrug resistance efflux pumps of Salmonella enterica serovar typhimurium results in impaired ability to form a biofilm. J Antimicrob Chemother. 2012 Oct;67(10):2409–2417. doi: 10.1093/jac/dks228
- Paluch E, Rewak-Soroczynska J, Jedrusik I, et al. Prevention of biofilm formation by quorum quenching. Appl Microbiol Biotechnol. 2020 Mar;104(5):1871–1881. doi: 10.1007/s00253-020-10349-w
- Baugh S, Phillips CR, Ekanayaka AS, et al. Inhibition of multidrug efflux as a strategy to prevent biofilm formation. J Antimicrob Chemother. 2014 Mar;69(3):673–681. doi: 10.1093/jac/dkt420
- Dawan J, Li Y, Lu F, et al. Role of efflux pump-mediated antibiotic resistance in quorum sensing-regulated biofilm formation by salmonella typhimurium. Pathogens. 2022 Jan 24;11(2):147. doi: 10.3390/pathogens11020147
- Du D, Wang-Kan X, Neuberger A, et al. Multidrug efflux pumps: structure, function and regulation. Nat Rev Microbiol. 2018 Sep;16(9):523–539. doi: 10.1038/s41579-018-0048-6
- Sun J, Deng Z, Yan A. Bacterial multidrug efflux pumps: mechanisms, physiology and pharmacological exploitations. Biochem Biophys Res Commun. 2014 Oct 17;453(2):254–267. doi: 10.1016/j.bbrc.2014.05.090
- Dever LA. Mechanisms of bacterial resistance to antibiotics. Arch Internal Med. 1991;151(5):886. doi: 10.1001/archinte.1991.00400050040010
- Martinez JL. Natural antibiotic resistance and contamination by antibiotic resistance determinants: the two ages in the evolution of resistance to antimicrobials. Front Microbiol. 2012;3:1. doi: 10.3389/fmicb.2012.00001
- Ryan BM, Dougherty TJ, Beaulieu D, et al. Efflux in bacteria: what do we really know about it? Expert Opin Investig Drugs. 2001 Aug;10(8):1409–1422. doi: 10.1517/13543784.10.8.1409
- Saier MH Jr., Paulsen IT, Sliwinski MK, et al. Evolutionary origins of multidrug and drug-specific efflux pumps in bacteria. FASEB J. 1998 Mar;12(3):265–274. doi: 10.1096/fasebj.12.3.265
- Horiyama T, Nishino K, Cloeckaert A. AcrB, AcrD, and MdtABC multidrug efflux systems are involved in enterobactin export in Escherichia coli. PLoS One. 2014;9(9):e108642. doi: 10.1371/journal.pone.0108642
- Blanco P, Hernando-Amado S, Reales-Calderon JA, et al. Bacterial multidrug efflux pumps: much more than antibiotic resistance determinants. Microorganisms. 2016 Feb 16;4(1):14. doi: 10.3390/microorganisms4010014
- Pasqua M, Grossi M, Zennaro A, et al. The varied role of efflux pumps of the MFS family in the interplay of bacteria with animal and plant cells. Microorganisms. 2019 Aug 22;7(9):285. doi: 10.3390/microorganisms7090285
- Silver S, Phung LT. Bacterial heavy metal resistance: new surprises. Annu Rev Microbiol. 1996;50(1):753–789. doi: 10.1146/annurev.micro.50.1.753
- Silver S. Bacterial resistances to toxic metal ions–a review. Gene. 1996 Nov 7;179(1):9–19. doi: 10.1016/S0378-1119(96)00323-X
- Yang J, Rawat S, Stemmler TL, et al. Arsenic binding and transfer by the ArsD as(III) metallochaperone. Biochemistry. 2010 May 4;49(17):3658–3666. doi: 10.1021/bi100026a
- Cervantes C, Ji G, Ramirez JL, et al. Resistance to arsenic compounds in microorganisms. FEMS Microbiol Rev. 1994 Dec;15(4):355–367. doi: 10.1111/j.1574-6976.1994.tb00145.x
- Long F, Su CC, Zimmermann MT, et al. Crystal structures of the CusA efflux pump suggest methionine-mediated metal transport. Nature. 2010 Sep 23;467(7314):484–488. doi: 10.1038/nature09395
- Delmar JA, Su CC, Yu EW. Heavy metal transport by the CusCFBA efflux system. Protein Sci. 2015 Nov;24(11):1720–1736. doi: 10.1002/pro.2764
- Nies DH. Heavy metal-resistant bacteria as extremophiles: molecular physiology and biotechnological use of Ralstonia sp. CH34. Extremophiles. 2000 Apr;4(2):77–82. doi: 10.1007/s007920050140
- Lewis K. Persister cells, dormancy and infectious disease. Nat Rev Microbiol. 2007 Jan;5(1):48–56. doi: 10.1038/nrmicro1557
- Leung V, Levesque CM. A stress-inducible quorum-sensing peptide mediates the formation of persister cells with noninherited multidrug tolerance. J Bacteriol. 2012 May;194(9):2265–2274. doi: 10.1128/JB.06707-11
- El Meouche I, Dunlop MJ. Heterogeneity in efflux pump expression predisposes antibiotic-resistant cells to mutation. Science. 2018 Nov 9;362(6415):686–690. doi: 10.1126/science.aar7981
- Ebbensgaard AE, Lobner-Olesen A, Frimodt-Moller J. The role of efflux pumps in the transition from low-level to clinical antibiotic resistance. Antibiotics. 2020 Nov 30;9(12):855. doi: 10.3390/antibiotics9120855
- Van Acker H, Coenye T. The role of efflux and physiological adaptation in biofilm tolerance and resistance. J Biol Chem. 2016 Jun 10;291(24):12565–12572. doi: 10.1074/jbc.R115.707257
- Bas S, Kramer M, Stopar D. Biofilm surface density determines biocide effectiveness. Front Microbiol. 2017;8:2443. doi: 10.3389/fmicb.2017.02443
- Ferrer-Espada R, Shahrour H, Pitts B, et al. A permeability-increasing drug synergizes with bacterial efflux pump inhibitors and restores susceptibility to antibiotics in multi-drug resistant Pseudomonas aeruginosa strains. Sci Rep. 2019 Mar 5;9(1):3452. doi: 10.1038/s41598-019-39659-4
- Kvist M, Hancock V, Klemm P. Inactivation of efflux pumps abolishes bacterial biofilm formation. Appl Environ Microbiol. 2008 Dec;74(23):7376–7382. doi: 10.1128/AEM.01310-08
- Mba IE, Nweze EI. Nanoparticles as therapeutic options for treating multidrug-resistant bacteria: research progress, challenges, and prospects. World J Microbiol Biotechnol. 2021 May 28;37(6):108. doi: 10.1007/s11274-021-03070-x
- Abu Lila AS, Ishida T. Liposomal delivery systems: design optimization and Current applications. Biol Pharm Bull. 2017;40(1):1–10. doi: 10.1248/bpb.b16-00624
- Tang S, Zheng J. Antibacterial activity of silver nanoparticles: structural effects. Adv Healthc Mater. 2018 Jul;7(13):e1701503. doi: 10.1002/adhm.201701503
- Reshma VG, Syama S, Sruthi S, et al. Engineered nanoparticles with antimicrobial property. Curr Drug Metab. 2017;18(11):1040–1054. doi: 10.2174/1389200218666170925122201
- Hsueh YH, Hsieh CT, Chiu ST, et al. Antibacterial property of composites of reduced graphene oxide with nano-silver and zinc oxide nanoparticles synthesized using a microwave-assisted approach. Int J Mol Sci. 2019 Oct 29;20(21):5394. doi: 10.3390/ijms20215394
- Deng H, Yang Y, Zuo T, et al. Multifunctional ZnO@CuS nanoparticles cluster synergize chemotherapy and photothermal therapy for tumor metastasis. Nanomedicine. 2021 Jun;34:102399.
- Iqbal G, Faisal S, Khan S, et al. Photo-inactivation and efflux pump inhibition of methicillin resistant staphylococcus aureus using thiolated cobalt doped ZnO nanoparticles. J Photochem Photobiol B. 2019 Mar;192:141–146.
- Nejabatdoust A, Zamani H, Salehzadeh A. Functionalization of ZnO nanoparticles by glutamic acid and conjugation with thiosemicarbazide alters expression of efflux pump genes in multiple drug-resistant staphylococcus aureus strains. Microb Drug Resist. 2019 Sep;25(7):966–974. doi: 10.1089/mdr.2018.0304
- Lee JH, Kim YG, Cho MH, et al. ZnO nanoparticles inhibit Pseudomonas aeruginosa biofilm formation and virulence factor production. Microbiol Res. 2014 Dec;169(12):888–896. doi: 10.1016/j.micres.2014.05.005
- Nikaido H. Prevention of drug access to bacterial targets: permeability barriers and active efflux. Science. 1994 Apr 15;264(5157):382–388. doi: 10.1126/science.8153625
- Khare T, Mahalunkar S, Shriram V, et al. Embelin-loaded chitosan gold nanoparticles interact synergistically with ciprofloxacin by inhibiting efflux pumps in multidrug-resistant Pseudomonas aeruginosa and Escherichia coli. Environ Res. 2021 Aug;199:111321.
- Poojari R. Embelin - a drug of antiquity: shifting the paradigm towards modern medicine. Expert Opin Investig Drugs. 2014 Mar;23(3):427–444. doi: 10.1517/13543784.2014.867016
- Opata MR, Dreuw A. Embelin’s versatile photochemistry makes it a potent photosensitizer for photodynamic therapy. J Phys Chem B. 2021 Apr 15;125(14):3527–3537. doi: 10.1021/acs.jpcb.1c00330
- Manju S, Malaikozhundan B, Vijayakumar S, et al. Antibacterial, antibiofilm and cytotoxic effects of Nigella sativa essential oil coated gold nanoparticles. Microb Pathog. 2016 Feb;91:129–135.
- Mrozek-Wilczkiewicz A, Musiol R. Nigella sativa – a functional spice from a Pharaoh’s tomb to modern healthcare. Nat Prod J. 2016;6(1):13–26. doi: 10.2174/2210315506999151209100649
- Mouwakeh A, Telbisz A, Spengler G, et al. Antibacterial and resistance modifying activities of Nigella sativa essential oil and its active compounds against listeria monocytogenes. Vivo. 2018 Jul;32(4):737–743. doi: 10.21873/invivo.11302
- Khosravi M, Mirzaie A, Kashtali AB, et al. Antibacterial, anti-efflux, anti-biofilm, anti-slime (exopolysaccharide) production and urease inhibitory efficacies of novel synthesized gold nanoparticles coated Anthemis atropatana extract against multidrug- resistant Klebsiella pneumoniae strains. Arch Microbiol. 2020 Oct;202(8):2105–2115. doi: 10.1007/s00203-020-01930-y
- Chemsa AE, Zellagui A, Ozturk M, et al. Chemical composition, antioxidant, anticholinesterase, antimicrobial and antibiofilm activities of essential oil and methanolic extract of Anthemis stiparum subsp. sabulicola (Pomel) Oberpr. Microb Pathog. 2018 Jun;119:233–240.
- Dehghanizade S, Arasteh J, Mirzaie A. Green synthesis of silver nanoparticles using Anthemis atropatana extract: characterization and in vitro biological activities. Artif Cells Nanomed Biotechnol. 2018 Feb;46(1):160–168. doi: 10.1080/21691401.2017.1304402
- Foroohimanjili F, Mirzaie A, Hamdi SMM, et al. Antibacterial, antibiofilm, and antiquorum sensing activities of phytosynthesized silver nanoparticles fabricated from Mespilus germanica extract against multidrug resistance of Klebsiella pneumoniae clinical strains. J Basic Microbiol. 2020 Mar;60(3):216–230. doi: 10.1002/jobm.201900511
- Wang Y, Liu B, Grenier D, et al. Regulatory mechanisms of the LuxS/AI-2 system and bacterial resistance. Antimicrob Agents Chemother. 2019 Oct;63(10). doi: 10.1128/AAC.01186-19
- Behdad R, Pargol M, Mirzaie A, et al. Efflux pump inhibitory activity of biologically synthesized silver nanoparticles against multidrug-resistant Acinetobacter baumannii clinical isolates. J Basic Microbiol. 2020 Jun;60(6):494–507. doi: 10.1002/jobm.201900712
- Ahmed FY, Aly UF, Abd El-Baky RM, et al. Effect of titanium dioxide nanoparticles on the expression of efflux pump and quorum-sensing genes in MDR Pseudomonas aeruginosa isolates. Antibiotics. 2021 May 24;10(6):625. doi: 10.3390/antibiotics10060625
- Zhou T, Ma Y, Kong X, et al. Design of iron chelators with therapeutic application. Dalton Trans. 2012 Jun 7;41(21):6371–6389. doi: 10.1039/c2dt12159j
- Richardson DR. Iron chelators as therapeutic agents for the treatment of cancer. Crit Rev Oncol Hematol. 2002;42(3):267–281. doi: 10.1016/S1040-8428(01)00218-9
- Christena LR, Mangalagowri V, Pradheeba P, et al. Copper nanoparticles as an efflux pump inhibitor to tackle drug resistant bacteria. RSC Adv. 2015;5(17):12899–12909. doi: 10.1039/C4RA15382K
- Chatterjee AK, Chakraborty R, Basu T. Mechanism of antibacterial activity of copper nanoparticles. Nanotechnology. 2014 Apr 4;25(13):135101. doi: 10.1088/0957-4484/25/13/135101
- Geilich BM, Gelfat I, Sridhar S, et al. Superparamagnetic iron oxide-encapsulating polymersome nanocarriers for biofilm eradication. Biomaterials. 2017 Mar;119:78–85.
- Pham DTN, Khan F, Phan TTV, et al. Biofilm inhibition, modulation of virulence and motility properties by FeOOH nanoparticle in Pseudomonas aeruginosa. Braz J Microbiol. 2019 Jul;50(3):791–805. doi: 10.1007/s42770-019-00108-z
- Nguyen TK, Duong HT, Selvanayagam R, et al. Iron oxide nanoparticle-mediated hyperthermia stimulates dispersal in bacterial biofilms and enhances antibiotic efficacy. Sci Rep. 2015 Dec 18;5:18385. doi: 10.1038/srep18385
- Khalid HF, Tehseen B, Sarwar Y, et al. Biosurfactant coated silver and iron oxide nanoparticles with enhanced anti-biofilm and anti-adhesive properties. J Hazard Mater. 2019 Feb 15;364:441–448. doi: 10.1016/j.jhazmat.2018.10.049
- Noordman WH, Janssen DB. Rhamnolipid stimulates uptake of hydrophobic compounds by Pseudomonas aeruginosa. Appl Environ Microbiol. 2002 Sep;68(9):4502–4508. doi: 10.1128/AEM.68.9.4502-4508.2002
- Vaillancourt M, Limsuwannarot SP, Bresee C, et al. Pseudomonas aeruginosa mexR and mexEF antibiotic efflux pump variants exhibit increased virulence. Antibiotics. 2021 Sep 25;10(10):1164. doi: 10.3390/antibiotics10101164
- Sabella S, Carney RP, Brunetti V, et al. A general mechanism for intracellular toxicity of metal-containing nanoparticles. Nanoscale. 2014 Jun 21;6(12):7052–7061. doi: 10.1039/c4nr01234h
- Siritongsuk P, Hongsing N, Thammawithan S, et al. Two-phase bactericidal mechanism of silver nanoparticles against Burkholderia pseudomallei. PLoS One. 2016;11(12):e0168098. doi: 10.1371/journal.pone.0168098
- Mishra M, Kumar S, Majhi RK, et al. Antibacterial efficacy of polysaccharide capped silver nanoparticles is not compromised by AcrAB-TolC efflux pump. Front Microbiol. 2018;9:823. doi: 10.3389/fmicb.2018.00823
- Saha RP, Samanta S, Patra S, et al. Metal homeostasis in bacteria: the role of ArsR-SmtB family of transcriptional repressors in combating varying metal concentrations in the environment. Biometals. 2017 Aug;30(4):459–503. doi: 10.1007/s10534-017-0020-3
- Chandrangsu P, Rensing C, Helmann JD. Metal homeostasis and resistance in bacteria. Nat Rev Microbiol. 2017 Jun;15(6):338–350. doi: 10.1038/nrmicro.2017.15
- Mathivanan K, Chandirika JU, Vinothkanna A, et al. Bacterial adaptive strategies to cope with metal toxicity in the contaminated environment - a review. Ecotoxicol Environ Saf. 2021 Dec 15;226:112863. doi: 10.1016/j.ecoenv.2021.112863
- Begg SL. The role of metal ions in the virulence and viability of bacterial pathogens. Biochem Soc Trans. 2019 Feb 28;47(1):77–87. doi: 10.1042/BST20180275
- Ye Q, Chen W, Huang H, et al. Iron and zinc ions, potent weapons against multidrug-resistant bacteria. Appl Microbiol Biotechnol. 2020 Jun;104(12):5213–5227. doi: 10.1007/s00253-020-10600-4
- Becker KW, Skaar EP. Metal limitation and toxicity at the interface between host and pathogen. FEMS Microbiol Rev. 2014 Nov;38(6):1235–1249. doi: 10.1111/1574-6976.12087
- White C, Lee J, Kambe T, et al. A role for the ATP7A copper-transporting ATPase in macrophage bactericidal activity. J Biol Chem. 2009 Dec 4;284(49):33949–33956. doi: 10.1074/jbc.M109.070201
- Djoko KY, Ong CL, Walker MJ, et al. The role of copper and zinc toxicity in innate immune defense against bacterial pathogens. J Biol Chem. 2015 Jul 31;290(31):18954–18961. doi: 10.1074/jbc.R115.647099
- Thevenod F. Catch me if you can! Novel aspects of cadmium transport in mammalian cells. Biometals. 2010 Oct;23(5):857–875. doi: 10.1007/s10534-010-9309-1
- Valente A, Podolski-Renic A, Poetsch I, et al. Metal- and metalloid-based compounds to target and reverse cancer multidrug resistance. Drug Resist Updat. 2021 Sep;58:100778.
- Sheldon JR, Skaar EP. Metals as phagocyte antimicrobial effectors. Curr Opin Immunol. 2019 Oct;60:1–9. doi: 10.1016/j.coi.2019.04.002
- Abdel-Karim SAM, El-Ganiny AMA, El-Sayed MA, et al. Promising FDA-approved drugs with efflux pump inhibitory activities against clinical isolates of staphylococcus aureus. PLoS One. 2022;17(7):e0272417. doi: 10.1371/journal.pone.0272417
- Nino-Martinez N, Salas Orozco MF, Martinez-Castanon GA, et al. Molecular mechanisms of bacterial resistance to metal and metal oxide nanoparticles. Int J Mol Sci. 2019 Jun 8;20(11):2808. doi: 10.3390/ijms20112808
- Pelgrift RY, Friedman AJ. Nanotechnology as a therapeutic tool to combat microbial resistance. Adv Drug Deliv Rev. 2013 Nov;65(13–14):1803–1815. doi: 10.1016/j.addr.2013.07.011
- Wu B, Huang R, Sahu M, et al. Bacterial responses to Cu-doped TiO(2) nanoparticles. Sci Total Environ. 2010 Mar 1;408(7):1755–1758. doi: 10.1016/j.scitotenv.2009.11.004
- Simon-Deckers A, Loo S, Mayne-L’hermite M, et al. Size-, composition- and shape-dependent toxicological impact of metal oxide nanoparticles and carbon nanotubes toward bacteria. Environ Sci Technol. 2009 Nov 1;43(21):8423–8429. doi: 10.1021/es9016975
- Ng CK, Sivakumar K, Liu X, et al. Influence of outer membrane c-type cytochromes on particle size and activity of extracellular nanoparticles produced by shewanella oneidensis. Biotechnol Bioeng. 2013 Jul;110(7):1831–1837. doi: 10.1002/bit.24856
- Krutyakov YA, Khina AG. Bacterial resistance to nanosilver: molecular mechanisms and possible ways to overcome them. Appl Biochem Microbiol. 2022;58(5):493–506. doi: 10.1134/S0003683822050106
- Panacek A, Kvitek L, Smekalova M, et al. Bacterial resistance to silver nanoparticles and how to overcome it. Nat Nanotech. 2018 Jan;13(1):65–71. doi: 10.1038/s41565-017-0013-y
- Gibson K, Chu JK, Zhu S, et al. A tripartite efflux system affects flagellum stability in Helicobacter pylori. Int J Mol Sci. 2022 Oct 1;23(19):11609. doi: 10.3390/ijms231911609