ABSTRACT
Background
Ovarian cancer (OC) is the most lethal gynecological tumor, but it currently lacks effective therapeutic targets. CD147, which is overexpressed in OC, plays a crucial role in promoting malignant progression and is associated with poor prognosis in patients. Therefore, CD147 has been identified as a potential therapeutic target. However, there is a limited amount of research on the development of CD147 inhibitors.
Methods
Surface plasmon resonance (SPR) assay and virtual molecular docking analysis were performed to identify potential natural compounds targeting CD147. The anti‑tumor effects of myricetin were evaluated using various assays, including CCK8, Alkaline comet, immunofluorescence and xenograft mouse models. The underlying mechanism was investigated through western blot analysis and lentivirus short hairpin RNA (LV-shRNA) transfection.
Results
Myricetin, a flavonoid commonly found in plants, was discovered to be a potent inhibitor of CD147. Our findings demonstrated that myricetin exhibited a strong affinity for CD147 and down-regulated the protein level of CD147 by facilitating its proteasome-dependent degradation. Additionally, we observed synergistic antitumor effects of myricetin and cisplatin both in vivo and in vitro. Mechanistically, myricetin suppressed the expression of FOXM1 and its downstream DNA damage response (DDR) genes E×O1and BRIP1, thereby enhancing the DDR induced by cisplatin.
Conclusion
Our data demonstrate that myricetin, a natural inhibitor of CD147, may have clinical utility in the treatment of OC due to its ability to increase genomic toxicity when combined with cisplatin.
1. Introduction
OC is the deadliest gynecological tumor in the world [Citation1]. It is initially responsive to platinum-based chemotherapy, however, most of patients relapse and develop resistance to platinum drugs. More recently, anti-angiogenic drugs and poly (ADP-ribose) polymerase (PARP) inhibitors have been approved as ‘maintenance’ treatment for platinum-sensitive relapsed OC because they obviously prolong progression-free survival of OC patients [Citation2], however, most patients develop resistance to anti-angiogenic drugs and PARP inhibitors. Drug resistance is a serious problem in OC therapy, often leading to treatment failure and relapse. Therefore, it is necessary to find novel therapeutic targets and strategies.
CD147, a transmembrane glycoprotein, mainly expressed in epithelium and immune cells [Citation3]. CD147 was found overexpressed in tumor cells and promoted tumor migration, invasion, drug resistance, and angiogenesis. CD147 stimulates adjacent stromal cells to product matrix metalloproteinases (MMPs) [Citation4], which degrade extracellular matrix and promote tumor cell migration and invasion. As a partners of monocarboxylate transporter (MCT), CD147 plays an important role in glucose metabolism of tumor cells by regulating lactic acid export [Citation5,Citation6]. Furthermore, the interaction of CD147 and integrin contributes to the adhesion and metastasis of tumor cells. Some studies found CD147 and α3β1 integrin co-localize on the surface of tumor cells, and CD147 formed complexes with α3β1 and α6β1 integrins [Citation7,Citation8]. CD147 has been demonstrated to involve in tumor angiogenesis through promoting the expression of VEGF and VEGFR in endothelial cells and tumor cells [Citation9,Citation10]. Moreover, CD147 also mediates multidrug resistance by promoting the expression and dimerization of ABC transporter G2 (ABCG2) in breast cancer [Citation11]. Therefore, CD147 is a potential target for anti-cancer drug development.
Licartin, a monoclonal antibody (mAb) that targets CD147, has shown effectiveness in treating liver cancer and preventing recurrence after liver transplantation [Citation12]. However, its radioactive I131 component and high cost limit its widespread clinical application. AC-73, the first small molecule that targets CD147, has been proven to inhibit the mobility and invasion of hepatocellular carcinoma by inhibiting CD147 dimerization and the production of MMP2 [Citation13]. Building upon this, a new drug called HA-08 was synthesized and found to be more active than AC-73 [Citation14]. However, the anti-tumor effects of both compounds need to be confirmed in other types of tumors. Additionally, a study found that CD147 may be a target for shikonin, a traditional Chinese medicine, as shikonin downregulated the expression of CD147 and CD147 overexpression decreased shikonin-induced apoptosis in glioma cells [Citation15]. However, the study lacked important affinity data. Therefore, it is necessary to develop other more effective CD147 inhibitors.
Natural products offer the advantages of being multi-targeted and low-toxicity, making them an important source for the development of targeted anti-tumor drugs. Combining targeted drugs with traditional chemotherapy agents is a viable approach to enhance efficacy. However, it is worth noting that there are currently no known natural products that specifically target CD147.
Myricetin (3, 5, 7, 3‘, 4,’ 5’−hexahydroxyflavone) is a natural flavonoid widely found in many natural plants [Citation16], and has been considered to have great medicinal value. More and more studies have demonstrated that myricetin has anti-tumor effects on a variety of tumors. Myricetin is a multiple-target drug and intervenes some signaling pathways in tumor development, such as MEK/ERK, JAK1/STAT3 and PI3K/AKT signals, etc. Previous studies have demonstrated that myricetin acts as a natural inhibitor of MEK1, a crucial cytokine involved in cell transformation and tumor development. It has been observed that myricetin effectively suppresses the phosphorylation of downstream targets of MEK, surpassing the effectiveness of the MEK inhibitor PD098059 [Citation17]. Myricetin directly targeted JAK1 and inhibited the phosphorylation of JAK1/STAT3 signals, thus blocking the transformation of mouse JB6 cells [Citation18]. Molecular docking suggested that myricetin bound to the ATP-binding site of AKT by competing with ATP and inhibited the kinase activity of Akt [Citation19]. In addition, myricetin suppressed the activation of PI3K/AKT signaling and induced apoptosis of pancreatic cancer cells [Citation20]. However, there are currently no reports discussing the interaction of myricetin and CD147.
In this study, we measured the affinity of myricetin and CD147 using surface plasmon resonance (SPR) and molecule docking analysis. The results indicated that myricetin exhibited a higher affinity to CD147 compared to the CD147 inhibitor AC-73. Furthermore, we observed that myricetin promoted the degradation of CD147, thus identifying it as a natural inhibitor of CD147. Additionally, we assessed the effectiveness of combining myricetin with cisplatin in treating OC. Our findings demonstrated that myricetin enhanced the sensitivity of OC to cisplatin both in vitro and in vivo. The mechanism underlying myricetin-induced DNA damage may involve the downregulation of DDR gene expression.
2. Materials and methods
2.1. Reagents
Myricetin (70050-25 mg, purity ≥ 96%), dihydromyricetin (DHM, PHL80431-20 mg, purity ≥ 96%) were purchased from Sigma Aldrich. AC-73 (Glpbio), SP-8356 (Selleck) and protein biosynthesis inhibitor cycloheximide (CHX, Selleck) were dissolved in dimethyl sulfoxide (DMSO). Cisplatin (Sigma Aldrich) and MG132 (Selleck) were dissolved in sterilized double-distilled H2O. All reagents were stored at −20°C for less than three months. The final concentration of DMSO was 1:2000 or less.
2.2. Cell lines
A2780, SKOV3 and HEK 293T cell lines were obtained from the Institute of Cell Biology of Academia Sinica (Shanghai, China). ID8 cell line was purchased from the Cell Bank of the Type Culture Collection of the Chinese Academy of Sciences (Shanghai, China). A2780 and SKOV3 cells were cultured in RPMI-1640 medium (Biological Industries). HEK293T and ID8 cells were cultured in DMEM medium (Procell, PM15040). All media were supplemented with 10% fetal bovine serum (ExCell Bio) and 1% penicillin/streptomycin (Beyotime, China). Cells were incubated at 37°C and 5% CO2.
2.3. Molecular docking
Molecular docking analysis was carried out on the crystal structure of the CD147 human protein (PDB ID:3B5H) using the AutoDock 4.2 software (Scripps Research, U.S.A.). 3D structures of small molecules were downloaded from the PubChem database. Subsequently, the structure of CD147 protein was optimized through dehydration, ligand separation, and hydrogenation. Grid box was structured for each molecule, followed by semi-flexible docking. The docking scores were automatically generated by the software. The Pymol 2.7 software was used to analyze and visualize the binding patterns and hydrogen bonds.
2.4. Surface plasma resonance (SPR)
The SPR system (Biacore X100, U.S.A.) and CM5 chip (GE Healthcare, U.S.A.) were used to measure the affinity of small-molecules to CD147 at 25°C. Briefly, CD147 protein (ab151844, Abcam) and FOXM1 protein (ab158447, Abcam) were dissolved in sodium acetate buffer (pH 4.0) at a concentration of 100 µg/mL, and then the proteins were immobilized on two activated CM5 sensor chips using the amine coupling kit supplied by the manufacturer. The small molecules were dissolved in working buffer (1×PBS, 2% DMSO), respectively. Small molecule solutions with concentrations of 1.5625, 3.125, 6.25, 12.5, 25 and 50 μM were injected into the chip surface at a flow rate of 30 μL/min for 200 s, followed by the dissociation cycle for 300 s. Equilibrium dissociation (KD) constants were calculated based on a simple 1:1 interaction model using Biacore Insight scoring software.
2.5. Western blot and protein stability analysis
Extraction of whole protein from cells using RIPA buffer containing protease inhibitors and phosphatase inhibitors (Selleck, China). Proteins were separated on 10% SDS-PAGE gels and transferred to PVDF membranes (Millipore, U.S.A.). After PVDF membranes were blocking with 5% skim milk for 1 hour, they were incubated with primary antibodies (listed in Supplementary Table S1) and horseradish peroxidase (HRP)-conjugated secondary antibodies, respectively. The result visualized using an enhanced chemiluminescence (ECL) system (Bio-Rad).
For the detection of CD147 protein stability, 100 µg/mL Cycloheximide (CHX) was used to inhibit protein synthesis, and cells were harvested at 0 h, 2 h, 4 h and 6 h for western blot assay. OC cells were treated with MG132 (20 µM) 6 hours to block proteasomal-dependent degradation.
2.6. Quantitative real-time PCR (qRT-PCR)
Total RNA was extracted from OC cells using Trizol reagent (OMEGA Bio-Tek). Reverse transfection was performed using the PrimeScript RT Master Mix kit (Takara Bio, China) to synthesize cDNA. The PCR reaction was obtained using the SYBR Premix Ex Taq kit (Takara Bio, China), according to the manufacturer’s instructions. The mRNA expression level of actin gene was used as a control. The primer sequences used were listed in the supplement table S2.
2.7. CCK8 assay
Cell viability was measured using the Cell Counting Kit-8 (Beyotime, China). Cells were treated with graded concentrations of myricetin (0, 20, 40,80,160, 320 μM) or cisplatin (0, 0.25, 0.5, 1, 2, 4, 8, 16 μg/mL) for 48 hours followed by CCK8 for 2–4 hours. The cell viability was quantified through absorbance detection at 450 nm. The 50% inhibitory concentration (IC50) value was calculated by GraphPad Prism 8.0 software. As for combination treatment, two OC cell lines were treated with cisplatin (2 μg/mL) and myricetin (80 μM) for 48 h.
2.8. Alkaline comet assay
Cells were treated with DMSO, myricetin (80 μM) or cisplatin (2 μg/mL) 48 h before harvesting. Alkaline comet assay was performed using the Comet Assay Kit (Trevigen, 4250–050-K) according to the manufacturer’s instructions. For nuclear imaging, stain with DAPI (50 μg/mL, Yeasen) for 20 min. DNA damage level was observed under a fluorescent confocal microscope (Olympus), and the percentage of DNA in the tail (% Tail DNA) and tail length are indicative parameters of DNA damage. For each sample, at least 50 cells were analyzed using CometScore 2.0 software.
2.9. Immunofluorescence experiments
Cells treated with myricetin or cisplatin were fixed in 4% formalin, permeabilized in 0.3% Triton X-100, and then blocked with 5% BSA for 1 hour. Thereafter, the slides were incubated overnight with primary antibodies (γH2AX; 1:300 in 1% BSA) at 4°C, and then incubated with the fluorochrome-conjugated secondary antibody (1:500 in 1% BSA) 1 hour at room temperature, followed by staining with DAPI (Yeasen, China). Images were captured using a fluorescence microscope (CellSense, U.S.A.). The fluorescence intensity of γH2AX was quantitated using ImageJ software, and 200s cell per sample were evaluated.
2.10. Plasmids and lentivirus infection
We constructed pcDNA3.1-CD147 plasmid by inserting the open reading frame sequence of CD147 (Entrez Gene ID:682) into pcDNA3.1 plasmid (Invitrogen, V79520) to forced expression of CD147. Transient transfection was performed using Lipofectamine 2000 transfection reagent (Thermo Fisher Scientific, U.S.A.). 500,000 cells were transfected using 1 μg of empty pcDNA3.1 plasmid as a control or 1 μg pcDNA3.1-CD147 plasmid for 6 h.
To silence the expression of CD147, we transfected HEK 293T cells using VSVG/Gag-Pol lentiviral packaging system to produce lentiviral particles. The small hairpin RNA (shRNA) targeting CD147 was cloned into aPKLO.1 puro vector (Addgene, #8453). We harvested lentiviral particles after transfection for 48 hours, and stored them at −80°C.
2.11. In vivo experiments
All mouse experiments were performed in accordance with ethical guidelines approved by the institute of medical research of(Anonymised). ID8 cells were suspended in PBS at 5 × 106 cells/mL, and injected intraperitoneally into 6-week-old C57BL/6 mice. When the tumor can be monitored by ultrasound, the mice were randomly divided into four groups (n = 4). Mice were treated as followed: (a) control group (equal volume of saline); (b) myricetin (30 mg/Kg/2 days); (c) cisplatin (3 mg/Kg/7 days); (d) myricetin (30 mg/Kg/2 days) and cisplatin (3 mg/Kg/7 days) co-treated group. Myricetin was dissolved in a combination solution of 5% DMSO + 30% PEG300 + 5% Tween 80 + 60% sterilized water at a concentration of 6 mg/mL, and cisplatin was dissolved in sterilized PBS solution at a concentration of 1 mg/mL. All drugs were administered intraperitoneally. After 40 days of treatment, the mice were euthanized, and tumors were surgically dissected and fixed for IHC staining and western blot assays.
2.12. Statistical analysis
We performed statistical analysis using GraphPad Prism 8.0 software. All in vitro experiments were performed three times and data were presented as the mean ± SD. Data were analyzed using t-tests and one-way analysis of variance (ANOVA). IC50 was analyzed by nonlinear regression in the GraphPad Prism 8.0 software. A difference was considered statistically significant at *p < 0.05, **p < 0.01, ***p < 0.001.
3. Results
3.1. Myricetin is identified as an inhibitor of CD147
The role of CD147 in cancer treatment has been extensively studied, and the development of CD147 small molecule inhibitors is a key strategy. Natural products have the advantage of targeting multiple aspects and causing fewer side effects, making them a valuable resource for targeted drug development. However, there is limited research on the interaction between CD147 and natural products. Flavonoids, particularly myricetin and dihydromyricetin (DHM), have been widely reported for their anti-tumor effects [Citation21]. These two natural molecules have the same molecular formula and similar structural formula, prompting us to investigate their affinities with CD147.
We conducted a surface plasmon resonance (SPR) assay to measure the binding affinities of myricetin and DHM to CD147. Using the Biacore X100 system, the equilibrium dissociation constants (KD values) of the different molecules to CD147 were automatically calculated based on the kinetic data obtained from various concentrations (0.78125–50 μM) of the interacting molecules. The results revealed that myricetin exhibited a higher affinity to CD147, with a KD value of 3.718 × 10−6 M (), whereas DHM displayed a lower affinity with a KD value of 5.440 × 10−5 M (). Interestingly, despite the structural similarity between myricetin and DHM, the response value of the interaction between myricetin and CD147 was higher than that of DHM at the same concentration. Further analysis of the interaction kinetics data revealed that myricetin exhibited a rapid binding to CD147 with an association constant of 203.0 M−1s−1, which is 9.3 times higher than that of DHM (21.7 M−1s−1). These findings suggest that myricetin has a stronger affinity with CD147 compared to DHM and is more likely to impact the activity of CD147. To further identify potential binding sites of myricetin and CD147, we carried out virtual molecular docking analysis. The crystal structure of the human CD147 protein was downloaded from the RCSB PDB database (PDB ID:3B5H). The binding mode and docking score of the compounds were evaluated using AutoDock 4.2 software. As show in , myricetin occupied to the N-terminal domain of CD147 and formed 6 hydrogen bonds with amino acid residues of GLU73, LYS75, ASP77 and ASP80. The interaction patterns of myricetin-CD147 and DHM-CD147 showed the similar minimum binding energy, −5.52 kcal/mol () and −4.95 kcal/mol (Fig. S1B) respectively, suggesting that both flavonoids have the potential to bind to CD147. Thus, it is important to combine the structure-based molecular docking technique with in vitro experiments to analyze the binding ability and binding sites between molecules.
Figure 1. Binding affinity between CD147 and small molecules. (a) The binding dissociation curve of CD147-myricetin was obtained at different concentrations (3.125 μM-50 μM) by SPR technology. RU: response unites. (b)The KD values of CD147-myricetin were calculated by fitting a steady-state affinity curve. Equilibrium dissociation constant (KD) = 3.718 × 10–6 M. (c) The binding dissociation curve of CD147- DHM was obtained at different concentrations (3.125 μM-50 μM) by SPR technology. KD = 5.440 × 10–5 M. RU: response unites. (d) Docking pattern of CD147 crystal (PDB:3B5H, blue ribbons) and myricetin (red sticks) with minimum energy conformation. The amino acid residues involving the hydrogen-bonding interactions (yellow dotted lines) are shown. Surface model of CD147-myricetin binding (left). (e) Comparison of binding kinetic parameters of myricetin, AC-73 and SP-8356 to CD147. Ka, association constant; kd, dissociation constant; RU,response units.
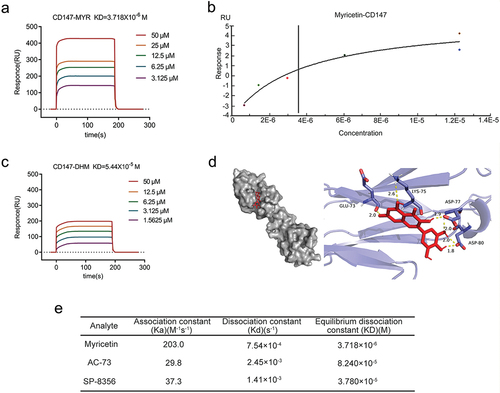
Previous studies have shown that AC-73 [Citation13] and SP-8356 [Citation22] target CD147 and inhibit CD147 dimerization. In this study, we compared the binding kinetics of myricetin with those of the known CD147 inhibitors. Our results revealed that myricetin exhibited a higher affinity for CD147 compared to AC-73 (KD = 8.240 × 10–5 M) and SP-8356 (KD = 3.780 × 10−5 M) ( and Fig. S1C-F). These findings suggest that myricetin binds to the catalytic pocket of CD147 and has a stronger affinity for CD147 than the two known inhibitors. Therefore, further investigation is necessary to understand the impact of myricetin on CD147 activity.
3.2. Myricetin accelerates proteasome-dependent degradation of CD147
CD147, a transmembrane glycoprotein, is primarily found in normal epithelial cells and immune cells. However, it is highly expressed on the surface of various tumor cells and plays a role in tumor cell invasion and metastasis by promoting the expression of MMP [Citation23]. Previous studies have shown that myricetin can inhibit the invasion and migration of lung cancer and breast cancer by downregulating the expression of MMP-2/9 [Citation24]. However, the specific mechanism through which myricetin affects MMP expression remains unclear. It is hypothesized that myricetin may affect MMP expression by forming a complex with CD147 and influencing its expression. To test this hypothesis, western blot and qRT-PCR assays were performed to detect the effects of myricetin on CD147 protein and mRNA levels, respectively. The results showed that treatment with myricetin led to a dose-dependent decrease in CD147 protein levels in A2780 and SKOV3 OC cells (p < 0.05) (). However, treatment with myricetin did not significantly reduce the mRNA expression of CD147 in both OC cells (p > 0.05) (), suggesting that myricetin may regulate the expression of CD147 at the protein level.
Figure 2. Myricetin accelerates degradation of CD147 protein via the proteasomal pathway. (a-b) The protein level of CD147 in A2780 (a) and SKOV3 cells (b) treated with different concentrations of myricetin (20,40, and 80 μM) or DMSO. Histograms showing the densitometric analysis of the bands normalized to GAPDH. (c-d) The mRNA level of CD147 in A2780 (c) and SKOV3 cells (d) Treated with different concentrations of myricetin (20,40, and 80 μM). (e-f) the protein stability of CD147 in the presence or absence of myricetin was measured by WB. Cycloheximide (CHX, 100 μg/mL) was used to block synthesis of protein. The line charts showing CD147 protein levels at different time points.(g-h) CD147 protein levels in A2780 cells (g) and SKOV3 cells (H) after proteasomal degradation was blocked by MG132 (20 μM). All data were presented as mean ± SD. one-way ANOVA, *p < 0.05, **p < 0.01, ***p < 0.001. ns: not significant.
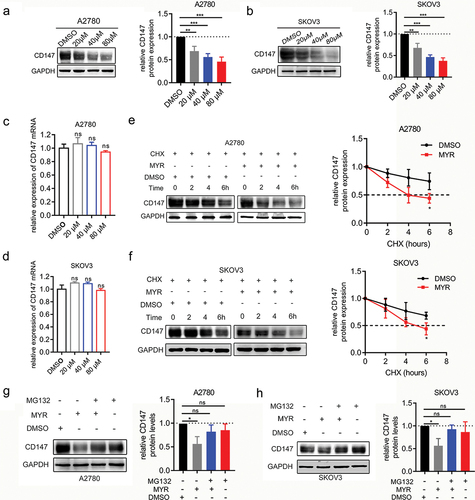
The effect of myricetin on the stability of the CD147 protein was investigated by treating A2780 and SKOV3 cells with CHX 100 µg/mL to block protein synthesis for 0, 2, 4, and 6 hours in the presence or absence of myricetin (80 µM) for 48 hours. It was observed that the rate of decrease in CD147 protein levels in A2780 and SKOV3 cells treated with myricetin was faster compared to the control cells (p < 0.05) (). Line graphs representing CD147 protein stability clearly showed the degradation rate of the protein, and it was found that the half-life of CD147 protein was significantly shortened in A2780 and SKOV3 cells (p < 0.05). These findings suggest that myricetin may accelerate the degradation of CD147 protein in A2780 and SKOV3 OC cells.
Since it has been reported that CD147 is degraded by the proteasome pathway [Citation25], we conducted a disruption assay to investigate whether blocking proteasome activity could reverse the effects of myricetin. We added MG132 (20 µM) in the presence or absence of myricetin to inhibit proteasomal degradation for a duration of 6 hours. Our findings revealed that MG132 was able to reverse the myricetin-induced decrease in CD147 protein levels in both OC cells (p < 0.05) (). These results suggest that myricetin promotes proteasome-dependent degradation of CD147, which may serve as an important mechanism for reducing the expression of MMPs in tumor cells. Therefore, myricetin holds promise as a potential compound for the development of new CD147 inhibitors.
3.3. Myricetin increases the sensitivity of OC cells to cisplatin
Platinum-based chemotherapy is the first-line treatment option for OC. However, many OC patients experience relapse and develop platinum-resistant disease [Citation26]. To improve chemosensitivity and prevent recurrence of OC, a combination of targeted therapy and chemotherapy has been suggested. Myricetin, a compound with anti-tumor effects, has shown promise in inhibiting oxidative stress, inducing tumor cell apoptosis, blocking cell cycle progression, and suppressing tumor angiogenesis in OC. A study found that myricetin promoted apoptosis in OC cells by inducing endoplasmic reticulum stress and DNA double-strand breaks [Citation27]. Moreover, myricetin increased the sensitivity of OC cells to paclitaxel by down-regulating multidrug resistance (MDR)-1 [Citation28]. Interestingly, myricetin had little cytotoxicity on the normal ovarian cell line IOSE-364, but it exhibited greater cytotoxicity than cisplatin in cisplatin-resistant cancer cell lines OVCAR-3 and A2780/CP70 [Citation29]. However, there is a lack of data on the combined use of myricetin and platinum in the treatment of OC.
To evaluate the potential synergistic anti-tumor effect of myricetin and cisplatin in OC cell lines, we conducted an experiment to examine the impact of myricetin on the cytotoxicity of cisplatin in A2780 and SKOV3 OC cell lines. Initially, we assessed the effect of myricetin on the cell viability of A2780 and SKOV3 cells using the CCK8 cytotoxicity assay to determine the appropriate concentration. Following a 48-hour treatment of the OC cell lines with varying concentrations of myricetin, we measured the absorbance and calculated the IC50 value. The results indicated that myricetin dose-dependently inhibited cell viability in both cell lines. Notably, A2780 cells exhibited greater sensitivity to myricetin (IC50: 110.5 ± 2.4 μM) compared to SKOV3 cells (IC50: 147.0 ± 9.5 μM) (Fig. S2A-B). To minimize the toxicity of the solvent DMSO on OC cells, we utilized a myricetin concentration (80 μM) lower than the IC50 value as the working concentration. Our study also revealed that 80 μM myricetin significantly down-regulated the CD147 protein level in OC cells (p < 0.05). Subsequently, we assessed the impact of myricetin on cisplatin sensitivity by treating the two cell lines with cisplatin alone (concentration gradient 0.25–16 μg/mL) or in combination with myricetin (80 μM) for 48 hours. The results demonstrated that combination therapy significantly reduced the IC50 value of cisplatin in OC cell lines (p < 0.05). Specifically, the mean IC50 value of cisplatin in A2780 cells decreased from 3.33 ± 0.405 μg/mL to 1.90 ± 0.275 μg/mL, and the mean IC50 value of cisplatin in SKOV3 cells decreased from 3.98 ± 0.272 μg/mL to 1.85 ± 2.242 μg/mL ().
Figure 3. Myricetin increases cisplatin sensitivity of OC cell lines. (a-b) The cisplatin sensitivity of A2780 and SKOV3 cells in the presence or absence of myricetin were measured by CCK8 assays. Histograms showing the IC50 values of triplicate measurements. (c) Representative images of alkaline comet assay showing the DNA damage level in A2780 and SKOV3 cells. Cells were treated with DMSO, myricetin, cisplatin, or myricetin-cisplatin combination for 48 h. Scale bar, 20 μm. (d-e) Individual data point in the scatter plot represented the comet tail DNA content of OC cells from C. 50 cells per sample were measured. (f) Representative images of γH2AX-immunofluorescence in A2780 and SKOV3 cells. Cells were treated with DMSO, myricetin, cisplatin, or myricetin-cisplatin combination for 48 h. Scale bar, 50 μm.(g-h) Proportion of γH2AX-positive cells in OC cells from F. 200 cells per sample were examined. Data were presented as means ± SD. one-way ANOVA. *p < 0.05, **p < 0.01, ***p < 0.001, ns: not significant.
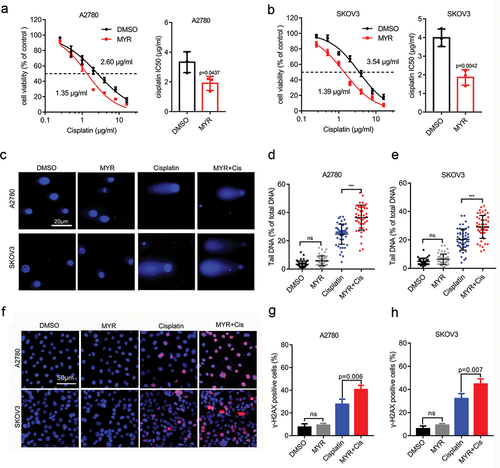
Given that cisplatin is a DNA-damaging drug, we performed an alkaline comet assay to investigate the effect of myricetin on cisplatin-induced DNA damage in OC cells. It is known that the length and DNA content of comet tails are indicators of DNA damage [Citation30]. Therefore, we assessed the degree of DNA damage by measuring the DNA content in the comet tail. Our results demonstrated that the combination treatment of cisplatin and myricetin caused more pronounced DNA damage compared to cisplatin alone in both OC cell lines (p < 0.001) (). Furthermore, we found that myricetin alone did not increase the level of DNA damage in OC cells. To confirm this finding, we examined the expression level of the DNA damage marker ɣ-H2AX using immunofluorescence assay. Consistent with the alkaline comet assay data, we observed a higher percentage of ɣ-H2AX-positive cells in OC cells treated with the combination therapy compared to cisplatin alone (). However, myricetin monotherapy did not increase the expression of ɣ-H2AX. Overall, these results suggest that myricetin enhances the toxicity of cisplatin to DNA in OC cell lines, but it does not directly damage DNA. Further research is necessary to elucidate the precise mechanism of the synergistic effect between myricetin and cisplatin.
3.4. Myricetin increases the sensitivity of cisplatin in OC cells
Previous studies have demonstrated a close relationship between CD147 and cisplatin resistance in various tumors. It has been shown that knocking down CD147 using small interfering RNA (siRNA) not only downregulates the expression of MCT1/4, but also improves the sensitivity of bladder cancer to cisplatin [Citation31]. Similarly, silencing CD147 with short hairpin RNA (shRNA) has been found to inhibit invasion of gastric tumor cells and increase the sensitivity of gastric cancer to cisplatin [Citation32]. Additionally, it has been observed that CD147 polyubiquitination and degradation can reverse cisplatin resistance in tumor cells [Citation33]. Therefore, the objective of our study is to investigate whether myricetin can regulate cisplatin sensitivity of OC cell lines, with a specific focus on its dependence on CD147.
To inhibit the expression of CD147, we used lentivirus transfection technique to introduce shRNA into A2780 and SKOV3 OC cells (). We then examined whether myricetin could enhance cisplatin-induced DNA damage in OC cells after CD147 knockdown. Immunofluorescence analysis of ɣ-H2AX revealed that cisplatin-induced DNA damage was significantly increased in CD147 knockdown cells, indicating that CD147 plays a protective role in maintaining genome stability. However, myricetin did not upregulate the expression of H2AX after CD147 knockdown in OC cells (). Additionally, we observed that CD147-knockdown OC cells exhibited increased sensitivity to cisplatin (IC50 dropped from 3.46 ± 0.596 μg/mL to 2.24 ± 0.104 μg/mL in A2780 cells, IC50 dropped from 4.91 ± 0.307 μg/mL to 2.65 ± 0.290 μg/mL in SKOV3 cells). However, myricetin did not further enhance the sensitivity of CD147-knockdown cells to cisplatin (), suggesting that the knockout of CD147 abolishes the effect of myricetin. These findings indicate that CD147 is involved in maintaining genome stability, and myricetin improves cisplatin sensitivity by down-regulating CD147.
Figure 4. Myricetin increases cisplatin sensitivity of OC cells depending on CD147. (a) The protein level in CD147-knockdown A2780 and SKOV3 cells. (b) The RNA level in CD147-knockdown A2780 and SKOV3 cells. (c) Representative images of γH2AX-immunofluorescence in CD147-knockdown A2780. Cells were treated with DMSO, myricetin, cisplatin, or myricetin-cisplatin combination for 48 h. Scale bar, 50 μm. (d) Histogram showing the proportion of γH2AX-positive cells in OC cells from C. 200 cells per sample were examined. (e) Representative images of γH2AX-immunofluorescence in CD147-knockdown SKOV3 cells. Cells were treated like C. Scale bar, 50 μm. (f) Histogram showing the proportion of γH2AX-positive cells in OC cells from H. 200 cells per sample were examined. (g-h) Cisplatin sensitivity of CD147-knockdown OC cells in the presence or absence of myricetin. Histograms showing the IC50 of three independent experiments. Data were presented as means ± SD. one-way ANOVA. *p < 0.05, **p < 0.01, ***p < 0.001, ns: not significant.
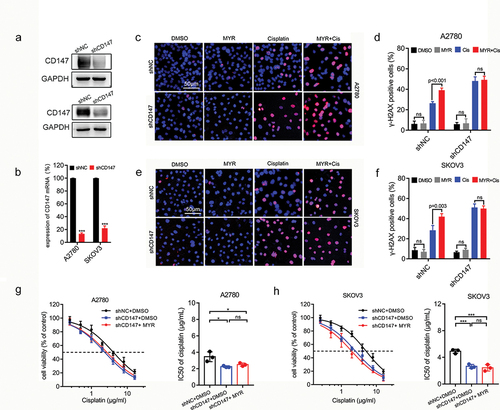
3.5. Myricetin inhibits DDR in OC cells
Increased DDR or DNA tolerance is an underlying mechanism of cellular adaptation to platinum drugs [Citation34]. Our study found that the direct damage of myricetin to DNA was not obvious. Therefore, we hypothesized that myricetin might improve cisplatin sensitivity by regulating the DDR pathway. A previous study has demonstrated that myricetin acts as an inhibitor of human flap endonuclease 1 (hFEN1), a structure-specific nuclease essential for DNA replication and repair processes. This inhibition leads to the downregulation of hFEN1 expression and sensitizes colon cancer cells to the treatment of paclitaxel [Citation35]. However, there are few studies that have reported the effect of myricetin on the expression of other DDR genes.
FOXM1, an essential transcription factor, is known to drive the transcription of DDR genes and contribute to drug resistance [Citation36,Citation37]. Previous studies have identified BRIP1 as a direct transcription target of FOXM1, and the FOXM1/BRIP1 axis has been shown to regulate DDR and drug resistance induced by epirubicin [Citation38]. Moreover, FOXM1 has been found to promote cisplatin resistance in OC by binding strongly to E×O1and regulating E×O1transcription [Citation39]. Therefore, we investigated the impact of myricetin on the expression of FOXM1 and its downstream DDR genes (EXO1 and BRIP1) in OC cells.
Our findings demonstrate that myricetin effectively downregulated the protein levels of FOXM1 (p < 0.01), E×O1(p < 0.05), and BRIP1 (p < 0.01) in both A2780 and SKOV3 cell lines (). Additionally, myricetin significantly reduced the mRNA levels of E×O1(p < 0.001) and BRIP1 (p < 0.001), while not affecting FOXM1 in both OC cell lines (). These results suggest that myricetin might induce genomic instability by suppressing the protein expression of FOXM1, thereby inhibiting the transcription of downstream DDR genes regulated by FOXM1.
Figure 5. Myricetin inhibits DDR in OC cell lines. (a-b) The protein levels of FOXM1, E×O1 and BRIP1 in A2780 (a) and SKOV3 (b) cells treated with myricetin or DMSO for 48 hours (left). Histograms showing the densitometric analysis of the bands normalized to GAPDH (right). (c-d) The mRNA levels of FOXM1, E×O1and BRIP1 in A2780 (c) and SKOV3 (d) Cells treated with myricetin or DMSO for 48 hours. (e-f) The protein levels of CD147, FOXM1, E×O1and BRIP1 in OC cells treated with myricetin for 48 hours and then forced to express CD147 for 24 hours. Histograms showing the densitometric analysis of the bands normalized to GAPDH (right). (g-h) the mRNA level of FOXM1, E×O1 and BRIP1 in A2780 (g) and SKOV3 (h) Cells treated with myricetin for 48 hours and then forced to express CD147 for 24 hours. Data were presented as means ± SD. one-way ANOVA. *p < 0.05, **p < 0.01, ***p < 0.001, ns: not significant.
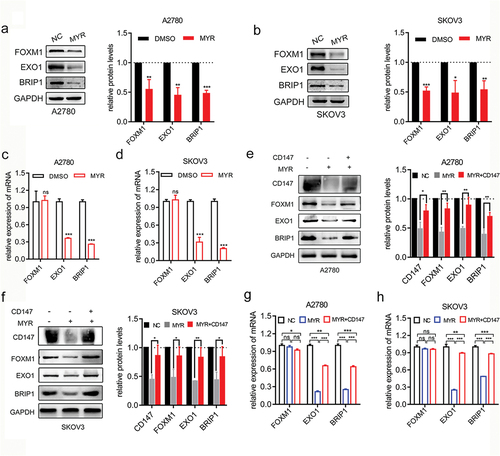
It has been discovered that CD147 plays a role in mediating the DDR by up-regulating the ATM/ATR signal, which contributes to the resistance of pancreatic cancer to gemcitabine [Citation40]. To investigate whether myricetin inhibits FOXM1-mediated DDR by down-regulating CD147, we conducted a repair experiment. We introduced pcDNA3.1-CD147 plasmid into OC cells treated with myricetin to induce the expression of CD147. Our observations revealed that the exogenous expression of CD147 partially rescued the reduction of FOXM1 protein levels caused by myricetin in OC cell lines (). Additionally, the exogenous expression of CD147 partially counteracted the inhibitory effects of myricetin on the transcription of FOXM1 downstream target genes, resulting in increased mRNA levels of E×O1and BRIP1 (). These findings demonstrate that myricetin suppresses the FOXM1-related DDR pathway by down-regulating CD147, leading to genomic instability in OC cells and enhancing the sensitivity of OC cells to cisplatin.
3.6. Myricetin and cisplatin synergistically inhibit the growth of OC in vivo.
Several studies have confirmed the inhibitory effects of myricetin on the growth and angiogenesis of subcutaneously transplanted OC through peripheral administration [Citation41,Citation42]. To better mimic the in vivo environment of OC, we utilized the ID8 mouse OC cell line to establish an abdominal cavity model. The animal models were treated with intraperitoneal administration to enhance the utilization rate of myricetin. After injecting the ID8 OC cell line for 2–3 weeks, multiple tumor foci were observed in the mouse abdominal cavity (). Both myricetin monotherapy (30 mg/kg) and cisplatin monotherapy (3 mg/kg) reduced the number of tumor metastatic foci compared to the control group. However, the efficacy of myricetin was not as significant as that of cisplatin (). For quantitative analysis, we removed all visible tumors in the abdominal cavity, weighed them, and performed statistical analysis. The results indicate that the combination treatment of myricetin (30 mg/kg) and cisplatin (3 mg/kg) resulted in the smallest tumor weight compared to cisplatin monotherapy (p = 0.0058) (). This suggests that myricetin and cisplatin have a synergistic effect in inhibiting the growth of OC in vivo.
Figure 6. Myricetin and cisplatin synergistically inhibit the growth of OC in vivo. (a) Representative images of mouse abdominal tumor from each treatment group. The yellow arrows point to the tumor focus. (b) Tumor weight per mouse was measured after the tumors were surgically dissected (n = 4). Individual data point in the scatter plot represents total weight of all tumor focus per mouse (right). (c) The protein levels of CD147, FOXM1, E×O1 and BRIP1 in the tumor was examined by western blot assay (left). Histogram showing the protein quantification from each group (right). (d) The protein levels of FOXM1, E×O1 and BRIP1 in the tumor was examined by qRT-PCR. Data were presented as means ± SD. *p < 0.05, **p < 0.01, ***p < 0.001, one-way ANOVA.
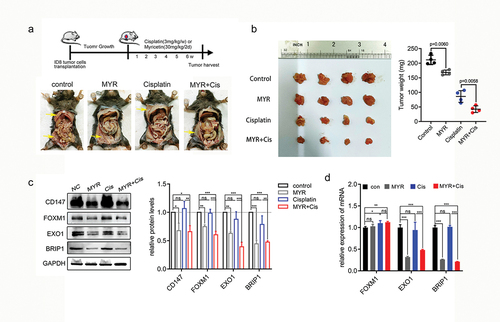
To validate the outcomes of our in vitro experiments, we performed western blot and qPCR experiments using protein and RNA extracted from tumor tissue. The results indicated that myricetin treatment significantly reduced the protein levels of CD147 (p = 0.0128) and FOXM1 (p = 0.0076), and inhibited the transcription of FOXM1 downstream target genes E×O1(p = 0.0032) and BRIP1 (p = 0.0005). Interestingly, we observed that cisplatin treatment led to an increase in the mRNA level of FOXM1, although there was no statistically significant difference in the protein levels of FOXM1 (p = 0.0553), EXO1 (p = 0.0619), and BRIP1 (p = 0.0690) (). This discrepancy could potentially be attributed to the cellular DDR.
The combination treatment of myricetin and cisplatin resulted in a significant decrease in the protein levels of CD147 (p = 0.0250) and FOXM1 (p = 0.0037). Additionally, the transcript levels of DDR genes E×O1(p = 0.0005) and BRIP1 (p = 0.0001) were also significantly reduced in the combined treatment group (). This suggests that myricetin has the ability to inhibit DNA damage caused by cisplatin. Overall, these findings indicate that myricetin can effectively suppress the growth of OC in synergy with cisplatin by down-regulating the expression of CD147 and FOXM1-related DDR genes.
4. Discussion
As a food ingredient, myricetin is believed to have various positive effects on human health, including antioxidant, anti-inflammatory, and anti-tumor properties [Citation43]. On one hand, myricetin is considered a potent antioxidant capable of scavenging reactive oxygen species (ROS) and activating antioxidant enzymes. This helps inhibit DNA oxidative damage and lipid peroxidation caused by hydrogen peroxide [Citation44]. On the other hand, flavonoids like myricetin, quercetin, and apigenin can interact with DNA, leading to nuclear DNA damage, especially in the presence of Cu2+ or under lower pH conditions [Citation45,Citation46]. A study has suggested that the effects of myricetin are dependent on its concentration and the microenvironment. At low concentrations (less than or equal to 1.5 mM), myricetin acts as an antioxidant, whereas at high concentrations, it can promote the generation of hydroxyl radicals (.OH) through hydrogen peroxide and induce DNA damage in the presence of Fe3+ [Citation47]. Therefore, some individuals believe that myricetin exhibits both antioxidant and pro-oxidation effects. In our study, we observed that myricetin (80 μM) did not exhibit significant toxicity to the genome under basic conditions. However, when combined with cisplatin, it resulted in significant DNA damage. The interpretation of the presented findings has some limitations. The main limitation is the small number of animals enrolled in the study. To verify this conclusion, a larger number of in vivo experiments are needed. Secondly, this experiment focused solely on cisplatin due to limited research funds. However, it is important to note that further investigation is warranted to explore the efficacy of carboplatin, which is more commonly used in clinical practice. Additionally, it should be noted that the study utilized diluted myricetin for intraperitoneal injection, as higher utilization rates of myricetin derivatives have not yet been synthesized. It is worth considering that this dilution may potentially impact the absorption effectiveness of myricetin.
Platinum-induced intra-strand and inter-strand crosslinks adducts are repaired through various DDR pathways [Citation34]. Targeting DDR pathways is a viable strategy to enhance platinum sensitivity. Studies have shown that myricetin inhibits key enzymes in DDR pathways, such as apurinic/apyrimidinic endonuclease 1 (APE1) [Citation48], which is an essential enzyme in DNA base excision repair, and human flap endonuclease 1 (hFEN1), a target for anti-cancer drug development [Citation35]. Furthermore, myricetin has been identified as a DNA-topoisomerase I poison, leading to DNA double-strand breaks and chromosome translocation by stabilizing the topoisomerase-DNA complex [Citation49]. Inhibition of DDR results in cell cycle arrest and apoptosis, which are crucial anti-tumor mechanisms of myricetin.
FOXM1, a member of the forkhead family proteins, consists of four isoforms (FOXM1A, B, C, D). Among these isoforms, FOXM1B is overexpressed in various tumor tissues and plays a critical role in embryonic development and tumor progression [Citation50]. FOXM1 regulates the transcription of genes related to DDR, including those involved in DNA damage sensing, signal transduction, and repair. Specifically, FOXM1 activates the transcription of E×O1and PolE2, which are responsible for DNA single-strand damage repair [Citation51]. Additionally, FOXM1 directly regulates the transcription of homologous recombination (HR) -related genes such as BRCA1/2, RAD51, and BRIP1, which are involved in HR, a highly accurate DNA double-strand break repair mechanism [Citation52]. Given its central role in DDR, FOXM1 has been identified as a potential target for tumor therapy. Our data shows that myricetin inhibits the expression of FOXM1 and its downstream DDR genes (EXO1 and BRIP1) through its interaction with CD147. This may explain why myricetin enhances the sensitivity of cisplatin in OC.
It has been confirmed that FOXM1 mediates cisplatin resistance of OC by activating the PI3K/AKT/mTOR signal [Citation53]. Our results indicate that CD147 regulates the protein level of FOXM1. Therefore, we hypothesize that CD147 acts as a signal molecule upstream of FOXM1. However, further investigation is needed to determine the specific mechanism.
Cisplatin and carboplatin are both platinum chemotherapeutic drugs with different curative effects. Cisplatin has been found to have a better curative effect compared to carboplatin. Additionally, cisplatin exhibits better radiosensitization and synergistic effects than carboplatin. Since this study focuses on basic cellular and animal experimentation, it is important to choose chemotherapeutic drugs with higher efficacy to effectively observe the combined action with myricetin. This design not only facilitates the observation of curative effects but also enables a deeper investigation into the underlying mechanisms.
The combination of myricetin and cisplatin has been shown to enhance the sensitivity of cisplatin and reduce its side effects. Previous studies have demonstrated that myricetin can alleviate cisplatin-induced colon toxicity and nephrotoxicity by increasing antioxidant enzyme levels and up-regulating inflammatory markers such as NF-κB, IL-6, and TNF-α [Citation54]. Additionally, myricetin-loaded nanomicelles have been found to mitigate cisplatin-induced acute kidney injury (AKI) by inhibiting oxidative stress and DNA damage [Citation55]. However, myricetin’s poor water solubility, incomplete absorption in the intestine, and low bioavailability pose limitations on its development and utilization. In recent years, researchers have made significant progress in improving the bioavailability of myricetin by synthesizing derivatives or encapsulating it with nanomaterials. These advancements will facilitate further investigation into the functions and mechanisms of myricetin. The synthesis of myricetin derivatives holds great significance in the treatment of tumors and other diseases that exhibit high expression of CD147, such as OC and liver cancer.
5. Conclusion
In this study, we have identified myricetin as a natural inhibitor of CD147. We found that myricetin promotes the degradation of CD147 protein through the proteasome pathway, and enhances the sensitivity of OC cells to cisplatin both in vivo and in vitro. Mechanistically, myricetin inhibits the repair of DNA damage by reducing the expression of FOXM1 and downstream DDR genes in OC cells. Our findings have important implications for the development of CD147 inhibitors and offer new possibilities for the treatment of OC.
Declaration of interests
The authors have no relevant affiliations or financial involvement with any organization or entity with a financial interest in or financial conflict with the subject matter or materials discussed in the manuscript. This includes employment, consultancies, honoraria, stock ownership or options, expert testimony, grants or patents received or pending, or royalties.
Reviewer disclosures
Peer reviewers on this manuscript have no relevant financial or other relationships to disclose.
Supplementary Information.docx
Download MS Word (305.9 KB)Acknowledgments
We thank Prof Hong Yang for helpful discussion and insightful comments on the manuscript.
Supplementary material
Supplemental data for this article can be accessed online at https://doi.org/10.1080/14728222.2024.2306345.
Additional information
Funding
References
- Siegel RL, Miller KD, Fuchs HE, et al. Cancer statistics, 2022. CA Cancer J Clin. 2022;72(1):7–33. doi: 10.3322/caac.21708
- Lheureux S, Gourley C, Vergote I, et al. Epithelial ovarian cancer. Lancet. 2019;393(10177):1240–1253. doi: 10.1016/S0140-6736(18)32552-2
- Radzikowska U, Ding M, Tan G, et al. Distribution of ACE2, CD147, CD26, and other SARS-CoV-2 associated molecules in tissues and immune cells in health and in asthma, COPD, obesity, hypertension, and COVID-19 risk factors. Allergy. 2020;75(11):2829–2845. doi: 10.1111/all.14429
- Biswas C, Zhang Y, DeCastro R, et al. The human tumor cell-derived collagenase stimulatory factor (renamed EMMPRIN) is a member of the immunoglobulin superfamily. Cancer Res. 1995;55(2):434–439.
- Lucke JN. Malignant hyperthermia in a parturient Poland China sow. Br J Anaesth. 1977;49(10):1070. doi: 10.1093/bja/49.10.1070
- Le Floch R, Chiche J, Marchiq I, et al. CD147 subunit of lactate/H+ symporters MCT1 and hypoxia-inducible MCT4 is critical for energetics and growth of glycolytic tumors. Proc Natl Acad Sci U S A. 2011;108(40):16663–16668. doi: 10.1073/pnas.1106123108
- Kay WW, Cameron MJ. Transport of C4-dicarboxylic acids in salmonella typhimurium. Arch Biochem Biophys. 1978;190(1):281–289. doi: 10.1016/0003-9861(78)90277-1
- Rodrigo R, Thielemann L, Olea M, et al. Effect of ethanol ingestion on renal regulation of water and electrolytes. Arch Med Res. 1998;29(3):209–218.
- Tang Y, Nakada MT, Kesavan P, et al. Extracellular matrix metalloproteinase inducer stimulates tumor angiogenesis by elevating vascular endothelial cell growth factor and matrix metalloproteinases. Cancer Res. 2005;65(8):3193–3199. doi: 10.1158/0008-5472.CAN-04-3605
- Bougatef F, Menashi S, Khayati F, et al. EMMPRIN promotes melanoma cells malignant properties through a HIF-2alpha mediated up-regulation of VEGF-receptor-2. PloS One. 2010;5(8):e12265. doi: 10.1371/journal.pone.0012265
- Zhou S, Liao L, Chen C, et al. CD147 mediates chemoresistance in breast cancer via ABCG2 by affecting its cellular localization and dimerization. Cancer Lett. 2013;337(2):285–292. doi: 10.1016/j.canlet.2013.04.025
- Xu J, Shen ZY, Chen XG, et al. A randomized controlled trial of Licartin for preventing hepatoma recurrence after liver transplantation. Hepatology. 2007;45(2):269–276. doi: 10.1002/hep.21465
- Fu ZG, Wang L, Cui HY, et al. A novel small-molecule compound targeting CD147 inhibits the motility and invasion of hepatocellular carcinoma cells. Oncotarget. 2016;7(8):9429–9447. doi: 10.18632/oncotarget.6990
- Fu ZG, Wang Y, Wang S, et al. Synthesis and evaluation of a novel Small-molecule Compound as an anticancer inhibitor of CD147. Biomed Environ Sci. 2019;32(9):673–686. doi: 10.3967/bes2019.086
- Guo N, Miao R, Gao X, et al. Shikonin inhibits proliferation and induces apoptosis in glioma cells via downregulation of CD147. Mol Med Rep. 2019;19(5):4335–4343. doi: 10.3892/mmr.2019.10101
- Javed Z, Khan K, Herrera-Bravo J, et al. Myricetin: targeting signaling networks in cancer and its implication in chemotherapy. Cancer Cell Int. 2022;22(1):239. doi: 10.1186/s12935-022-02663-2
- Lee KW, Kang NJ, Rogozin EA, et al. Myricetin is a novel natural inhibitor of neoplastic cell transformation and MEK1. Carcinogenesis. 2007;28(9):1918–1927. doi: 10.1093/carcin/bgm110
- Kumamoto T, Fujii M, Hou DX. Myricetin directly targets JAK1 to inhibit cell transformation. Cancer Lett. 2009;275(1):17–26. doi: 10.1016/j.canlet.2008.09.027
- Kumamoto T, Fujii M, Hou DX. Akt is a direct target for myricetin to inhibit cell transformation. Mol Cell Biochem. 2009;332(1–2):33–41. doi: 10.1007/s11010-009-0171-9
- Phillips PA, Sangwan V, Borja-Cacho D, et al. Myricetin induces pancreatic cancer cell death via the induction of apoptosis and inhibition of the phosphatidylinositol 3-kinase (PI3K) signaling pathway. Cancer Lett. 2011;308(2):181–188. doi: 10.1016/j.canlet.2011.05.002
- Sun Q, Liu Q, Zhou X, et al. Flavonoids regulate tumor-associated macrophages - from structure-activity relationship to clinical potential. Pharmacol Res. 2022;184:106419. doi: 10.1016/j.phrs.2022.106419
- Pahk K, Noh H, Joung C, et al. A novel CD147 inhibitor, SP-8356, reduces neointimal hyperplasia and arterial stiffness in a rat model of partial carotid artery ligation. J Transl Med. 2019;17(1):274. doi: 10.1186/s12967-019-2024-y
- Sun J, Hemler ME. Regulation of MMP-1 and MMP-2 production through CD147/extracellular matrix metalloproteinase inducer interactions. Cancer Res. 2001;61(5):2276–2281.
- Min X, Zhang X, Li Y, et al. HSPA12A unstabilizes CD147 to inhibit lactate export and migration in human renal cell carcinoma. Theranostics. 2020;10(19):8573–8590. doi: 10.7150/thno.44321
- Lheureux S, Braunstein M, Oza AM. Epithelial ovarian cancer: evolution of management in the era of precision medicine. CA Cancer J Clin. 2019;69(4):280–304. doi: 10.3322/caac.21559
- Tavsan Z, Kayali HA. Flavonoids showed anticancer effects on the ovarian cancer cells: involvement of reactive oxygen species, apoptosis, cell cycle and invasion. Biomed Pharmacother. 2019;116:109004. doi: 10.1016/j.biopha.2019.109004
- Xu Y, Xie Q, Wu S, et al. Myricetin induces apoptosis via endoplasmic reticulum stress and DNA double-strand breaks in human ovarian cancer cells. Mol Med Rep. 2016;13(3):2094–2100. doi: 10.3892/mmr.2016.4763
- Zheng AW, Chen YQ, Zhao LQ, et al. Myricetin induces apoptosis and enhances chemosensitivity in ovarian cancer cells. Oncol Lett. 2017;13(6):4974–4978. doi: 10.3892/ol.2017.6031
- Huang H, Chen AY, Ye X, et al. Myricetin inhibits proliferation of cisplatin-resistant cancer cells through a p53-dependent apoptotic pathway. Int J Oncol. 2015;47(4):1494–1502. doi: 10.3892/ijo.2015.3133
- Wang W, Li J, Tan J, et al. Endonuclease G promotes autophagy by suppressing mTOR signaling and activating the DNA damage response. Nat Commun. 2021;12(1):476. doi: 10.1038/s41467-020-20780-2
- Afonso J, Santos LL, Miranda-Goncalves V, et al. CD147 and MCT1-potential partners in bladder cancer aggressiveness and cisplatin resistance. Mol Carcinog. 2015;54(11):1451–1466. doi: 10.1002/mc.22222
- Wang B, Xu YF, He BS, et al. RNAi-mediated silencing of CD147 inhibits tumor cell proliferation, invasion and increases chemosensitivity to cisplatin in SGC7901 cells in vitro. J Exp Clin Cancer Res. 2010;29(1):61. doi: 10.1186/1756-9966-29-61
- Wu B, Liu ZY, Cui J, et al. F-Box protein FBXO22 mediates polyubiquitination and degradation of CD147 to reverse cisplatin resistance of tumor cells. Int J Mol Sci. 2017;18(1):212. doi: 10.3390/ijms18010212
- Rabik CA, Dolan ME. Molecular mechanisms of resistance and toxicity associated with platinating agents. Cancer Treat Rev. 2007;33(1):9–23. doi: 10.1016/j.ctrv.2006.09.006
- Ma L, Cao X, Wang H, et al. Discovery of myricetin as a potent inhibitor of human flap endonuclease 1, which potentially can be used as sensitizing agent against HT-29 human colon cancer cells. J Agric Food Chem. 2019;67(6):1656–1665. doi: 10.1021/acs.jafc.8b05447
- Kwok JM, Peck B, Monteiro LJ, et al. FOXM1 confers acquired cisplatin resistance in breast cancer cells. Mol Cancer Res. 2010;8(1):24–34. doi: 10.1158/1541-7786.MCR-09-0432
- Tassi RA, Todeschini P, Siegel ER, et al. FOXM1 expression is significantly associated with chemotherapy resistance and adverse prognosis in non-serous epithelial ovarian cancer patients. J Exp Clin Cancer Res. 2017;36(1):63. doi: 10.1186/s13046-017-0536-y
- Monteiro LJ, Khongkow P, Kongsema M, et al. The forkhead box M1 protein regulates BRIP1 expression and DNA damage repair in epirubicin treatment. Oncogene. 2013;32(39):4634–4645. doi: 10.1038/onc.2012.491
- Zhou J, Wang Y, Wang Y, et al. FOXM1 modulates cisplatin sensitivity by regulating EXO1 in ovarian cancer. PloS One. 2014;9(5):e96989. doi: 10.1371/journal.pone.0096989
- Zhou Y, Zheng M, Liu Z, et al. CD147 promotes DNA damage response and gemcitabine resistance via targeting ATM/ATR/p53 and affects prognosis in pancreatic cancer. Biochem Biophys Res Commun. 2020;528(1):62–70. doi: 10.1016/j.bbrc.2020.05.005
- Huang H, Chen AY, Rojanasakul Y, et al. Dietary compounds galangin and myricetin suppress ovarian cancer cell angiogenesis. J Funct Foods. 2015;15:464–475. doi: 10.1016/j.jff.2015.03.051
- Varela-Rodriguez L, Sanchez-Ramirez B, Hernandez-Ramirez VI, et al. Effect of gallic acid and myricetin on ovarian cancer models: a possible alternative antitumoral treatment. BMC Complement Med Ther. 2020;20(1):110. doi: 10.1186/s12906-020-02900-z
- Dobrzynska M, Napierala M, Florek E. Flavonoid nanoparticles: a promising approach for cancer therapy. Biomolecules. 2020;10(9). doi: 10.3390/biom10091268
- Wang ZH, Ah KK, Zhang R, et al. Myricetin suppresses oxidative stress-induced cell damage via both direct and indirect antioxidant action. Environ Toxicol Pharmacol. 2010;29(1):12–18. doi: 10.1016/j.etap.2009.08.007
- Das A, Majumder D, Saha C. Correlation of binding efficacies of DNA to flavonoids and their induced cellular damage. J Photochem Photobiol B. 2017;170:256–262. doi: 10.1016/j.jphotobiol.2017.04.019
- Sahu SC, Gray GC. Interactions of flavonoids, trace metals, and oxygen: nuclear DNA damage and lipid peroxidation induced by myricetin. Cancer Lett. 1993;70(1–2):73–79. doi: 10.1016/0304-3835(93)90077-M
- Laughton MJ, Halliwell B, Evans PJ, et al. Antioxidant and pro-oxidant actions of the plant phenolics quercetin, gossypol and myricetin. Effects on lipid peroxidation, hydroxyl radical generation and bleomycin-dependent damage to DNA. Biochem Pharmacol. 1989;38(17):2859–2865. doi: 10.1016/0006-2952(89)90442-5
- Srinivasan A, Wang L, Cline CJ, et al. Identification and characterization of human apurinic/apyrimidinic endonuclease-1 inhibitors. Biochemistry. 2012;51(31):6246–6259. doi: 10.1021/bi300490r
- Lopez-Lazaro M, Martin-Cordero C, Toro MV, et al. Flavonoids as DNA topoisomerase I poisons. J Enzyme Inhib Med Chem. 2002;17(1):25–29. doi: 10.1080/14756360290011744
- Halasi M, Gartel AL, Li J. Suppression of FOXM1 sensitizes human cancer cells to cell death induced by DNA-damage. PloS One. 2012;7(2):e31761. doi: 10.1371/journal.pone.0031761
- Zhang P, Chen X, Zhang L, et al. POLE2 facilitates the malignant phenotypes of glioblastoma through promoting AURKA-mediated stabilization of FOXM1. Cell Death Dis. 2022;13(1):61. doi: 10.1038/s41419-021-04498-7
- Yao S, Fan LY, Lam EW. The FOXO3-FOXM1 axis: a key cancer drug target and a modulator of cancer drug resistance. Semin Cancer Biol. 2018;50:77–89. doi: 10.1016/j.semcancer.2017.11.018
- Jin C, Liu Z, Li Y, et al. PCNA-associated factor P15(PAF), targeted by FOXM1, predicts poor prognosis in high-grade serous ovarian cancer patients. Int J Cancer. 2018;143(11):2973–2984. doi: 10.1002/ijc.31800
- Hassan SM, Khalaf MM, Sadek SA, et al. Protective effects of apigenin and myricetin against cisplatin-induced nephrotoxicity in mice. Pharm Biol. 2017;55(1):766–774. doi: 10.1080/13880209.2016.1275704
- Qi X, Wang J, Fei F, et al. Myricetin-loaded nanomicelles protect against cisplatin-induced acute kidney injury by inhibiting the DNA damage-cGAS-STING signaling pathway. Mol Pharm. 2023;20(1):136–146. doi: 10.1021/acs.molpharmaceut.2c00520