ABSTRACT
Introduction
Despite great advances, novel therapeutic targets and strategies are still needed, in particular for some carcinomas in the metastatic stage (breast cancer, colorectal cancer, pancreatic ductal adenocarcinoma and the clear cell renal carcinoma). Ion channels may be considered good cancer biomarkers and targets for antineoplastic therapy. These concepts are particularly relevant considering the hERG1 potassium channel as a novel target for antineoplastic therapy.
Areas covered
A great deal of evidence demonstrates that hERG1 is aberrantly expressed in human cancers, in particular in aggressive carcinomas. A relevant cornerstone was the discovery that, in cancer cells, the channel is present in a very peculiar conformation, strictly bound to the β1 subunit of integrin receptors. The hERG1/β1 integrin complex does not occur in the heart. Starting from this evidence, we developed a novel single chain bispecific antibody (scDb-hERG1-β1), which specifically targets the hERG1/β1 integrin complex and exerts antineoplastic effects in preclinical experiments.
Expert opinion
Since hERG1 blockade cannot be pursued for antineoplastic therapy due to the severe cardiac toxic effects (ventricular arrhythmias) that many hERG1 blockers exert, different strategies must be identified to specifically target hERG1 in cancer. The targeting of the hERG1/β1 integrin complex through the bispecific antibody scDb-hERG1-β1 can overcome such hindrances.
1. Introduction
Despite great advancements in the understanding of cancer biology and molecular features, which have led to improved both early diagnosis and therapeutic options in many cancer types, novel therapeutic strategies are still needed, especially for some carcinomas. In particular, the metastatic stage of many cancers, i.e. breast cancer, colorectal cancer, pancreatic ductal adenocarcinoma, lung cancer, and the clear cell renal carcinoma [Citation1] still need novel and improved therapies. In particular, novel and more efficient targeted therapies and immunotherapies are needed to fight the most aggressive carcinomas, especially in the metastatic stage. Indeed, an increasing number of novel antibodies, comprising both Antibody Drug Conjugates (ADCs) and novel antibody formats, as well as novel cellular therapies, such as CAR-T/CAR-NK cells, are being released on the market to push cancer therapeutic options forward [Citation2]. This huge amount of work from Academia and Pharma Companies (often in conjunction) is based on both the improvement/amelioration of therapeutics directed against ‘classical’ oncological targets (i.e. HER2/Neu antigens in breast [Citation3]) and the identification of novel targets, new tumor-associated or tumor-specific antigens, which can be addressed by novel therapeutics [Citation4]. Among new tumor antigens, whose relevance in cancer is emerging in the last years, ion channels must be included [Citation5]. Indeed, an increasing number of studies has led to show that ion channels are frequently mis-expressed in cancer cells where they contribute to regulate different cancer hallmarks (e.g. cell proliferation, survival and apoptosis, migration, invasion, and angiogenesis). Overall, ion channels can nowadays be considered novel cancer biomarkers and novel targets for therapy [Citation6–9]. Among ion channels which are frequently dysregulated in tumors, K+ channels are pivotal, being broadly expressed in several types of tumors [Citation10,Citation11], where they collectively modulate the cancerogenic processes through different mechanisms [Citation5,Citation12,Citation13]. Particularly relevant in this context is the EAG family of voltage-gated K+ channels, whose most important members KV10.1, also known as EAG1, encoded by the KCNH1 gene [Citation14,Citation15] and KV11.1, also known as hERG1, encoded by the KCNH2 gene [Citation16,Citation17], are highly represented in human cancers and deeply involved in the regulation of different cancer hallmarks. Indeed, hERG1 is the main subject of the present review and we will discuss its functional characteristics in normal and cancer cells, as well as the struggles encountered over the years for its targeting in cancer.
Overall, the novel therapeutic strategies to beat cancers, and in particular metastatic cancers, should take into account the targeting of ion channels, K+ channels in particular. However, the targeting of ion channels has several issues, due to the broad expression of channel proteins and their functional relevance in normal cells and tissues. Hence, efforts must be devoted to find more selective and cancer specific ion channels’ modulators, both small molecules and biologics. In this scenario, antibodies represent a great advantage in targeting ion channels [Citation18]. An emblematic example in this field is represented by hERG1, which is broadly expressed in cancers, and in particular in hard to treat and metastatic carcinomas, but in the meanwhile represent ‘an antitarget’ because of the potentially life-threatening effects of its blockade. We will describe below how it is possible to overcome challenges to target hERG1 in cancer.
2. Area covered
2.1. hERG1: structure, expression and physiological functions
hERG1 is an outward rectifying K+ channel belonging to the EAG family, encoded by the ether-à-go-go related gene 1 (KCNH2). The gene was cloned in 1994 from a human hippocampus cDNA library and mapped on chromosome 11, in q35–36 position [Citation19]. The corresponding protein is composed by 1159 amino-acids, with both amino- and carboxy-terminals located in the cytoplasm. hERG1 channels assemble as tetramers, each monomer being made by 6 transmembrane domains (S1-S6, with S4 representing the voltage sensor [Citation20]) and a long loop forming the pore. The four loops contribute to form the aqueous pore in the center of the tetrameric structure. The amino-terminal domain is typical of the EAG channel family and comprises a PAS (Per Arnt Sim) domain [Citation21]. Adjacent to the PAS domain there is a specific hERG1 domain called the proximal domain [Citation19]. hERG1 structure is shown in .
Figure 1. a) hERG1 potassium channel structure. PAS: PAS (acronym of per Arnt Sim) domain; cNBD (cyclic nucleotide binding domain). b) Ionic currents contributing to the ventricular action potential (top panel) and ECG (bottom panel); the action potential is aligned with its approximative time of action during the ECG. c) hERG1 in normal adult cells in collaboration with its canonical accessory subunits MinK1 and MirP1. Created with BioRender.com.
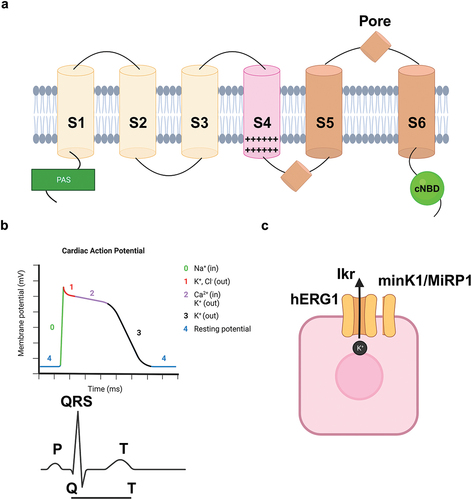
hERG1 shows peculiar voltage-dependent gating properties, which justify its physiological roles in normal excitable cells. Briefly, as the membrane potential (Vm) depolarizes, hERG1 rapidly activates but quickly inactivates. However, when Vm repolarizes, hERG1 inactivation is quickly removed and the channel hence sustains strong and long lasting outward, repolarizing K+ currents. Next, the channel slowly deactivates and then closes [Citation22]. These cycles of activation/inactivation/deactivation/closure make hERG1 an effective regulator of action potential (AP) in mature excitable cells, as cardiac myocytes. Indeed, the heart is the main tissue where hERG1 is physiologically expressed. In the heart, hERG1 underlies the cardiac repolarizing current IKr, which is mirrored by the QT interval in the superficial ECG (). This explains why mutations in the KCNH2 gene retard the cardiac AP repolarization phase, leading to a longer QT interval and hence to the onset of a cardiac arrhythmia, known as the long QT type 2 (LQT2) syndrome [Citation23,Citation24]. In other excitable cells hERG1 contributes to regulate (i) hormone release (e.g. in pancreatic neuroendocrine cells) [Citation25,Citation26]; (ii) neuronal spike frequency adaptation [Citation27] and other aspects of excitability (reviewed in [Citation28]); (iii) contractility in smooth muscle cells [Citation29]. It is worth noting that, in normal excitable cells hERG1 associates with its canonical accessory subunits MinK1 (KCNE1) and MirP1 (KCNE2) [Citation20] (). Thanks to this association, functional repolarizing currents can be generated. This point has a great relevance for the purposes of hERG1 targeting in cancer, and this point will hence be detailed below.
2.2. hERG1 in cancer: expression and functions
The first indication concerning the aberrant expression of hERG1 in neoplastic cells, and of its role in the control of cell proliferation was obtained in 1995 in neuroblastoma cells. From these studies it emerged that the biophysical characteristics of the channel, and in particular its activation kinetics, are correlated with the cell cycle phases [Citation30]. It is well known that neoplastic cells are characterized by lower values of the resting membrane potential (Vrest) than cells of the corresponding healthy tissues and it has been proposed that this is an essential prerogative for the uncontrolled growth typical of tumors [Citation31]. Starting from the assumption that hERG1 regulates Vm, studies have been conducted to evaluate its role in neoplastic cells. In a paper published in 1998 [Citation32] it was first shown that the KCNH2 transcript is overexpressed in neoplastic cell lines of different histogenesis and peculiar hERG1 currents (, inset) can be registered in these cells. The first demonstration of the presence of the KCNH2 gene transcript and its protein in human primary solid neoplasms was obtained from the analysis of endometrial adenocarcinoma samples [Citation33]. Subsequently it was demonstrated that hERG1 is also aberrantly expressed in several primary solid tumors [Citation9] [Citation33–50].
Figure 2. hERG1 potassium channel main functions in neoplastic cells and peculiar hERG1 currents (inset). ↑: increase; ↓: decrease. Created with BioRender.com.
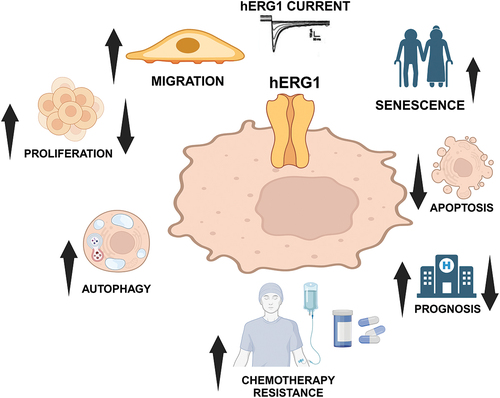
Overall, nowadays we know that hERG1 is aberrantly overexpressed in different types of human cancers where it contributes to regulate different cancer hallmarks, covering almost all stages of tumorigenesis (from cell proliferation and survival to the modulation of angiogenesis, cell invasiveness and metastasis [Citation51]; [references [Citation52–73] in ] (). For example, in lymphoid leukemias, hERG1 mainly regulates cell survival, while it is implicated in the control of transendothelial migration in myeloid leukemia. In colorectal and gastric cancer, it regulates the secretion of the angiogenic factor VEGF-A, and in turn affects neo angiogenesis and the tumor metastatic spread [Citation50]. What is particularly important in the present context is that growing evidence reviewed in [Citation17,Citation74,Citation75] indicates that hERG1 is implicated mainly in those processes which are regulated by cell adhesion to the Extra Cellular Matrix (ECM). This suggests that a unifying mechanism in the hERG1 role in neoplastic progression might be represented by the functional interaction of the channel with adhesion receptors, especially those of the integrin family [Citation17]. Indeed, the interplay between the channel and the integrin can modulate different ECM-triggered, cancer-related signaling pathways, such as FAK, ERK, and AKT phosphorylation, the activation and nuclear translocation of NFkB and HIF-1α, the modulation of f-actin organization and dynamics, either directly or through the involvement of small GTPases [Citation60,Citation74,Citation76]. In addition, hERG1 regulates the Vrest in cancer cells, because of its steady-state properties. The hERG1 activation and inactivation curves cross around −40 mV. Hence, the hERG1 ‘window’ current is centered around the typical Vrest of cycling and tumor cells [Citation20]. In conclusion, hERG1 appears to exert peculiar roles in cancer cells, which are apparently different from those typically observed in those adult organs where the channel is present, i.e. the modulation of action potential shape and firing. These hERG1 roles in cancer cells are mainly related to its interaction with integrin receptors and ECM interaction (see below, paragraph 2.4). Finally and more relevant, growing preclinical evidence indicated that blocking hERG1 currents with specific hERG1 blockers (see below) had antineoplastic effects in vivo, both in leukemias and solid cancers [Citation58,Citation61].
Table 1. _hERG1 expression and function in tumors.
2.3. Targeting hERG1 for antineoplastic therapy: a big challenge
The numerous preclinical evidence showing that hERG1 blockers exerted antineoplastic activity in vivo, would encourage to consider the channel a good target for antineoplastic therapy, if many hERG1 blockers did not produce cardiotoxic effects in humans. Indeed, it is well known that blocking hERG1 can cause serious cardiac arrhythmias by retarding cardiac repolarization, lengthening the electrocardiographic QT interval, which can give rise to torsade de pointes, a ventricular arrhythmia that may lead to ventricular fibrillation [Citation77]. These effects are related to the physiological role exerted by hERG1 in the human heart (see above). Among hERG1 blockers, which can trigger life- threatening arrhythmias, we must consider class III antiarrhythmic drugs, such as dofetilide and E4031, first. However, also structurally different compounds which can interact with specific amino acids usually in the pore sequence, can block ion conduction through the channel. Although some compounds effectively block hERG1 without facilitating arrhythmia (addressed as ‘nontorsadogenic’ hERG1 blockers [Citation78]), for the hindrances considered above, hERG1 is generally considered an undesirable pharmacologic target [Citation79]. Given this, one can hypothesize different possible pharmacologic approaches in clinical trials to exploit the anticancer effects of hERG1 inhibition in cancer cells while avoiding cardiotoxicity. The simplest possibility is using the above mentioned ‘nontorsadogenic’ hERG1 blockers, such as fluoxetine (Prozac; Eli Lilly) as suggested by Pointer [Citation80], or macrolide antibiotics, which were found to have hERG1-dependent antineoplastic effects in preclinical studies [Citation59,Citation81].
Another possible therapeutic strategy is seeking to target tumor-specific hERG1 features. We tested this possibility exploiting the fact that hERG1 has two isoforms, hERG1A and hERG1B [Citation82] and that leukemias preferentially express the isoform hERG1B. The latter is less relevant than hERG1A in heart physiology [Citation83]. On this basis we studied a pyrimidoindole derivative with a more selective inhibitory activity on hERG1B, CD160130 [Citation84], which showed to exert very good antileukemic activity. CD160130 could hence represent a first in- class compound to develop isoform-specific hERG1 blockers.
However, the most relevant and tumor-specific feature of hERG1 channels is that, in neoplastic cells, they do not associate with the classical accessory subunits MinK1\MIRP1, as occurs in cardiac cells. The discovery that a common molecular partner of hERG1 in tumor cells is the β1 subunit of integrin receptors [Citation74–76,Citation85]; represented a relevant breakthrough to envisage different strategies to specifically target hERG1 in cancers avoiding the blockade of hERG1 currents in the heart.
2.4. The hERG1/β1 integrin complex in cancer
Co-immunoprecipitation experiments, further confirmed by fluorescence resonance energy transfer (FRET) data, clearly showed that hERG1 interacts with β1 integrin on the plasma membrane of human cancer cells, giving rise to a hERG1/β1 integrin macromolecular complex [Citation17,Citation74,Citation75]. The complex assembly does not involve the intracellular domains of hERG1 or the cytoplasmic C-terminal domain of the β1 integrin [Citation86]. Hence, it is the transmembrane domain of the β1 integrin that putatively interacts with the hERG1 voltage sensor [Citation86]. Interestingly, the presence of KCNE1 (MinK) accessory subunit in cardiomyocytes alters the interaction between hERG1 and β1 integrin [Citation86]. Since KCNE1 is not expressed in cancer cells, this lack may explain the presence of the hERG1/β1 integrin complex in cancers [Citation86]. Recently another explanation has been provided for the frequent overexpression of hERG1 as well as of the hERG1/β1 integrin complex in cancer. In fact, a mathematical model developed to interpret the data related to the dynamics of hERG1 interaction with the β1 integrin, focused on the role of the tumor microenvironment. The latter would trigger the translocation of hERG1 channels to the plasma membrane, and hence their over-expression, through the engagement of cellular integrins by ECM proteins [Citation87]. The same paper also clarified the dynamics of the mutual relationship between hERG1 and integrins: when the β1 integrin is activated by cell adhesion onto the ECM or by specific antibodies, hERG1-mediated currents increase which in turn is accompanied by the triggering of several signaling pathways mediated by integrin receptors [Citation75,Citation76,Citation88]. Such integrin-dependent hERG1 stimulation of hERG1 conductive functions determines Vrest hyperpolarization [Citation88] and regulates FAK phosphorylation and cell proliferation [Citation75,Citation86]; Next, hERG1 associates with the β1 integrin subunit, which preferentially recruits hERG1 in the closed state [Citation59,Citation86], which progressively restores the initial Vrest [Citation87]. The recruitment of hERG1 in the closed state within the hERG1/β1 integrin complex suggests that the signaling pathways triggered by the complex mainly rely on signal transfer by conformational coupling, more than to the ion flux [Citation17]. For example, in colorectal cancer (CRC) cells integrin-dependent cell adhesion triggers the recruitment of the PI3K p85 subunit within the hERG1/β1 integrin complex, specifically to the hERG1 protein. This stimulates AKT phosphorylation and thus regulates autophagy [Citation59], through a mechanism that we refer to conformational coupling. In addition, we provided evidence that the hERG1/β1 integrin complex can recruit other ion channels and transporters, such as the Na+/H+ antiporter NHE1, thus controlling intracellular pH and in turn cell motility and migration [Citation57]. In pancreatic adenocarcinoma (PDAC) cells, a different hERG1-centered mechanism sustains cell motility, which occurs through the reorganization of f-actin in stress fibers and the modulation of filopodia formation and dynamics, thanks to the interplay with small GTPases and the modulation of [Ca2+]i [Citation60]. In leukemia cells, the hERG1/β1 integrin complex also comprises the chemokine receptor CXCR4, to trigger pro-survival signaling pathways and resistance to chemotherapy-induced apoptosis [Citation61]. Finally, the hERG1/β1 integrin complex comprises the Carbonic Anhydrase IX (CA-IX), in clear-cell renal carcinoma (ccRC) cells and primary tissues [Citation38], where hERG1 and CAIX together represent biomarkers of ccRC progression [Citation38]. Overall, the hERG1/β1-integrin/NHE1 and hERG1/β1-integrin/CA-IX complexes may represent functional hubs to locally control intra- and extra-cellular pH, especially in hypoxic conditions, hence driving pro-metastatic signaling pathways. summarizes all these findings.
Figure 3. hERG1/β1 signaling pathways in different types of cancer. From left to right: in CRC hERG1/β1 complex triggers AKT-HIF1α centered signaling sustaining cancer cell neoangiogenesis; in PDAC hERG1/β1 complex controls RHO-GTPase actin signaling mediating Ca+2 release; in acute myeloid leukemia hERG1/β1 complex sustains proliferation, migration and neoangiogenesis via VEGFR-1 interaction through MAPK, PI3K/AKT signaling. Created with BioRender.com.
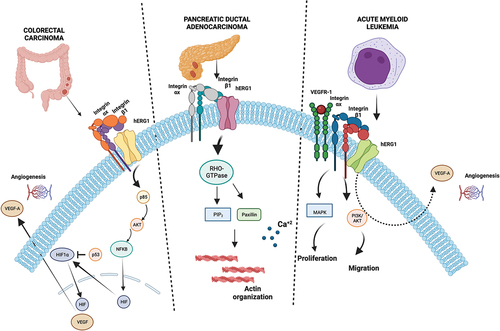
2.5. The hERG1/β1 integrin complex is a novel tumor antigen and target for antineoplastic therapy
All the data described above lead to the conclusion that the hERG1/β1 integrin complex can be considered a novel tumor antigen. First, the complex does not occur in the heart, where hERG1 assembles with its canonical accessory subunits as depicted in [Citation88]. Second, the hERG1/β1 integrin complex is present exclusively in tumors, especially in those advanced cancers where a hERG1 overexpression was previously detected. To this purpose we have exploited the possibility to use the scDb antibody as a diagnostic tool performing immunohistochemistry (IHC) to assess the expression of the complex in patients’ derived sections of different histogenesis. shows a list of all the human cancers where the hERG1/β1 integrin complex was detected, thanks to different techniques, i.e. co-immunoprecipitation and IHC exploiting the hERG1/β1 integrin-specific antibody which will be described in the next paragraph. A positive statistically significant correlation emerged between hERG1 and hERG1/β1 integrin complex in CRC, PDAC, breast cancer, neuroendocrine tumors of ileum and pancreas, and MALT lymphoma primary samples (see and related references [Citation89–97]).
Table 2. hERG1/β1 integrin complex expression in tumor.
Finally, the hERG1/β1 integrin complex is the true cancer biomarker, which can be exploited not only for diagnostic/prognostic purposes, but also for a novel therapeutic approach to cancer. In fact, depending on the pathophysiological context, the hERG1/β1 integrin complex can modulate different cancer-related, cancer-relevant, signaling pathways, which have a relevant functional impact on cancer cell proliferation, survival, motility, etc [Citation17,Citation75]. When the interplay between the integrin and the channel involves the activation of ion conduction, mainly FAK and ERK phosphorylation are switched on, and cell proliferation mechanisms are triggered [Citation17,Citation75]. When such interplay involves the formation of multiprotein complexes, signal transfer mainly occurs by conformational coupling [Citation17]. This has been proven in the case of the AKT-centered pathways, in CRC and PDAC cells [Citation50,Citation60,Citation89], which overall indicates that the hERG1/β1 integrin complex is mostly implicated in controlling cell motility.
Overall, it is not hERG1 but the hERG1/β1 integrin complex that can be targeted to obtain a good therapeutic response in cancer without affecting the cardiac hERG1, and hence without cardiotoxicity. How can this specific targeting be obtained? The development of novel, specific antibodies was the answer to this question.
2.6. Targeting the complex: the hERG1/β1 integrin bispecific antibody
Moving from an anti-hERG1 monoclonal antibody developed in 2006, a single chain variable fragment (scFv) antibody, anti-hERG1scFv, was derived. The antibody was mutagenized, to substitute a Phenylalanine residue in the third framework of the VH domain with a Cysteine residue. The resulting scFv-hERG1-Cys had higher stability, also mirrored by much higher protein yield, and increased affinity for the antigen, compared to the ‘native’ anti-hERG1scFv. The scFv-hERG1-Cys was hence chosen and characterized both in vitro and in vivo, allowing us to conclude that it possesses features which make it a suitable tool for application in cancer molecular imaging [Citation98].
Furthermore, the scFv-hERG1-Cys was used to develop a single chain antibody targeting the hERG1/β1 integrin complex in the format of a Diabody (scDb). Indeed, the scDb-hERG1-β1 construct was then generated starting from the scFv-hERG1-Cys and an anti β1 integrin scFv, obtained from to TS2/16 monoclonal antibody. The two scFvs were linked by three peptide linkers and expressed in yeast. The scDb-hERG1-β1 bound hERG1 and β1 when they were complexed (i.e. in cells seeded onto Fibronectin) at higher strength compared to the single proteins, as demonstrated by immunofluorescence (IF) data and cell-Enzyme-Linked Immunosorbent Assay (ELISA). The latter data were obtained on a reconstituted cellular model, GD25 cells, selectively expressing either hERG1 or β1, or both. The antibody has also been characterized for its stability in mouse plasma, showing it to remain stable even when tested at different timepoints (0, 6, 24, 48, 72, 96 h). In vivo evidence was also given concerning the lack of general or cardiac toxicity when administered at 20 µg/mice, assessing that no changes occurred either in ECG (no lengthening of the QT interval) or in kidney perfusion.
The scDb-hERG1-β1 antibody was then characterized in vitro on HCT116 CRC cell line. It had an effect of reduction on growth rate on a 3D culture of spheroids and it also had a deep effect on lateral motility. It is able to decrease AKT phosphorylation and HIF1α expression, which resulted to be completely shut down, while having mild or null effects on FAK and ERK phosphorylation. Such findings allow us to suggest a possible ‘freezing’ effect of the antibody on the complex.
Finally, in order to improve the effect obtained using the nude scDb-hERG1-β1, we have developed a trifunctional recombinant protein able to target the hERG1/β1 integrin complex and, at the same time, to induce apoptosis through the ‘tumor necrosis factor-related apoptosis-inducing ligand’ (TRAIL) mediated pathway. The recombinant protein is composed by our scDb-hERG1-β1 N-terminally fused to a single chain TRAIL (scTRAIL). The fusion between scDb-hERG1-β1 and TRAIL occurred in two PCR steps: the first one to allow the linkage between one segment of the scDb-hERG1-β1 and TRAIL; the second one to allow the linkage between the other segment of the scDb-hERG1-β1 and product of the above-mentioned PCR, using appropriate primers. The full construct of the scDb-hERG1-β1-TRAIL, cloned into the mammalian expression vector pSectag2A, resulted to be of 2400 bp in length, consistently with what expected and, hence, sequenced. It presented a point mutation into the scDb-hERG1-β1 portion which was reverted via in situ-mutagenesis, followed by a further sequencing step, with a positive outcome. The scDb-hERG1-β1-TRAIL was then transfected into CHO-K1 cells using Lipofectamine 2000. Transfected cells were selected using Zeocin and the supernatant containing the fusion protein was collected for two weeks. We concentrated the antibody supernatant using Centricon and tested it on 697 cells, using supernatant derived from non-transfected CHO-K1 cells as negative control.
Moreover, it was possible to upgrade the scDb-hERG1-β1 antibody, obtaining a potentially more powerful molecule, ADC based, which has already been preliminarily tested in vitro on ccRCC (clear cell renal carcinoma) Caki1 cells, showing a good anticancer activity. In fact, the scDb-hERG1-β1 antibody has been conjugated with the anti-CAIX AA38–08 molecule, chosen among different CAIX inhibitors (e.g. PS, IR, SLC, specific inhibitors synthetized by Claudiu T Supuran Group University of Florence) that have been tested on ccRCC Caki1 cells for their effects.
The strategy used to develop the scDb-hERG1-β1 and the different formats which can be produced to improve its efficacy are depicted in .
Figure 4. Schematic structure of antibodies directed against hERG1/β1 complex. From top, schematic structure of monoclonal antibodies directed against hERG1 and β1 (mAb), respectively; below schematic structure of the mAbs’ derived single chain diabody directed against hERG1/β1 complex (scDb-hERG1-β1). On the lower panel some of the antibodies derived from the scDb-hERG1-β1 antibody engineering are reported: from left, scDb-hERG1-β1-TRAIL, scDb-hERG1-β1-CAR-T and scDb-hERG1-β1-ADC (antibody drug conjugate). Created with BioRender.com.
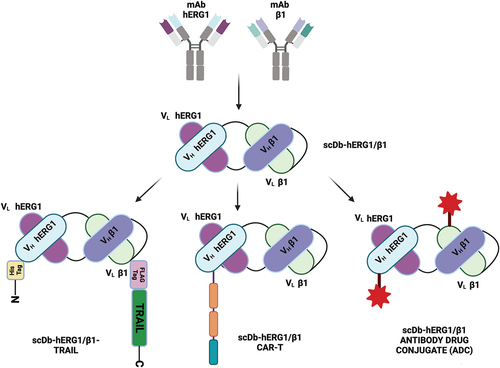
3. Conclusion
Ion channels are becoming more and more relevant in cancer physiology, and their specific targeting must be pursued. Among ion channels aberrantly expressed in cancer, the hERG1 channel must be taken into account. Searching for hERG1 targeting strategies, relevant molecular differences between the channel expressed in normal tissues (mainly in the heart) compared to that present in cancers emerged. In particular, it was found the hERG1 forms a macromolecular complex with the β1 subunit of integrin receptors, exclusively in cancer cells. Hence, the hERG1/β1 integrin complex (and not the channel per se) is the proper therapeutic target to be addressed for anti-neoplastic therapy. This discovery opened the way for a novel strategy to target the hERG1/β1 integrin complex, through bispecific antibodies capable of harnessing the complex, blocking its assembly and its signaling activity, such as through the PI3K-Akt pathway. The developed antibody (scDb-hERG1-β1) and its derivatives turned out to inhibit relevant cancer functions such as cell motility, angiogenesis, and metastasis in the preclinical setting, both in vitro and in vivo. Overall, the strategy described in the present review is envisaged to improve the therapeutic options for cancers, in particular for metastatic carcinomas.
4. Expert opinion
Given the wide aberrant expression of hERG1 in different cancer types, and in particular in hard to treat carcinomas, it became immediately clear that novel and non-cardiotoxic strategies to target hERG1 should be identified, if hERG1 would be considered a proper oncological target. In fact, hERG1 exerts a pivotal role in cardiac physiology, since it constitutes the molecular correlate of the rapid repolarizing current IKr which starts the repolarizing phase of the cardiac action potential. Hence targeting hERG1 for cancer treatment must avoid the block of its ion conductive function, since blocking hERG1 currents lengthens cardiac repolarization, lengthens the ECG QT interval and predisposes to fatal ventricular arrhythmias [Citation20,Citation77,Citation79]. This effect is identical to what happens in patients whose hERG1 encoding gene KCNH2 is mutated, and hence can develop a genetic Long QT syndrome (type 2). What is more, once the role of hERG1 in cardiac physiology and pathology [Citation99] became clear, it emerged that several drugs, even structurally unrelated to class III antiarrhythmic drugs and with completely different therapeutic applications, could cause cardiac arrythmias (similar to the type 2 Long QT syndrome) because they were able to block hERG1 currents. The discovery of many ‘torsadogenic’ hERG1 blocking drugs focused the pharma market for years to screen potentially cardiotoxic drugs based on testing their effects on hERG1 currents. Indeed a ‘hERG1 test’ is still mandatory during the early, preclinical phases of drug development [Citation100]. Hence the question arises: is it possible to target hERG1 for antineoplastic therapy? The answer is no, if we block the conductive properties of the channel. Indeed, this could also block hERG1 currents in the heart, inhibit IKr, retard cardiac repolarization, hence facilitating the onset of ventricular arrythmias. For these reasons the discovery that hERG1 not only is aberrantly expressed in cancer cells, but resides in tumor cells, especially in carcinomas, in a very peculiar conformation, i.e. tightly bound to the β1 subunit of integrin receptors to form a macromolecular complex, represented a relevant cornerstone. What is more, hERG1 within the hERG1/β1 integrin complex is preferentially in the closed, non-conductive conformation, i.e. it does not conduct potassium ions.
Overall, completely novel strategies must be figured out to target hERG1 in carcinomas, when the channel is bound to integrins, in the hERG1/β1 integrin complex. As stated above and in Duranti & Iorio [Citation87] this is the usual conformation in these tumor types, where the ECM proteins (such as Fibronectin and Collagen I) are the main triggers of hERG1 over expression and hERG1/β1 integrin complex formation. One of these strategies could be the repurposing of ‘old’ drugs such as Clarythromycin which targets the closed hERG1 [Citation59] and dissociates the hERG1/β1 integrin complex (Iorio et al., personal communication). Another strategy was the bispecific antibody developed by us, scDb-hERG1-β1, which specifically binds hERG1 and the β1 integrin, but only when the two proteins are complexed together. The antibody was developed in the format of a diabody, with a peculiar length of the linker between each variable domains, in order to assure a proper folding, which was predicted through an appropriate structure modeling. Such tuning resulted in a protein capable of binding only the complex and not the two proteins at distances higher than 1.6 nm, i.e. the distance we determined for the complex from FRET experiments [Citation86]. The strategy to develop the scDb-hERG1-β1 was quite innovative, since two scFv antibodies, specifically binding extracellular domains of either hERG1 or the β1 integrin [Citation89,Citation101,Citation102] were engineered, and the resulting product was expressed in yeasts. This strategy allows an easy protocol for its production, with low costs, lower than those necessary to produce recombinant antibodies in eukaryotic cells, such as CHO cells [Citation103]. What is more, the scDb-hERG1-β1 shows a good toxicologic, biodistribution, and therapeutic profile, especially in CRC and PDAC [Citation89]. In particular, no cardiac toxicity of the scDb emerged either in mice [Citation89] or humans [Citation104]. When tested both in vitro and in vivo on different cancer models (e.g. CRC, PDAC, and breast cancer) the diabody was shown to significantly decrease tumor burden and metastatic spread [Citation89,Citation93]. Moreover, when tested in combination with different chemotherapeutic agents, such as gemcitabine [Citation93] and cisplatin derivatives [Citation105] the antibody has demonstrated to potentiate the effect of the drug, which could be used at lower doses, preventing severe toxicity of such pharmacological treatments.
Another relevant discovery was the fact that hERG1 associates with β1 integrin prevalently in the closed state [Citation86], suggesting a non-conductive function of hERG1 in cancers, which can transduce the ECM signals by conformational coupling. In agreement, and interestingly relevant from the therapeutic point of view, is the fact that the newly developed scDb-hERG1-β1 antibody impairs complex formation and prefers the hERG1 closed conformation [Citation100].
Which steps are missing to reach the goal of treating carcinoma patients with the scDb targeting the hERG1/β1 integrin complex? First of all, the antibody is fully patent protected (‘Novel Antibodies’ IP WO2019015936A1), and the patent was licensed to an academic spin-off of the University of Florence MCK Therapeutics Srl. Furthermore, the preclinical not regulatory steps of drug development have been fully accomplished, whereas preclinical, regulatory steps (mainly toxicological tests) must be completed before reaching the clinical Phase 1. This step requires more time and will be accomplished in the next years.
Overall, the identification of a novel target, of a novel tumor antigen, the hERG1/β1 integrin complex, has led to the development of a completely new bispecific antibody, and it is envisaged to tempt researchers (both in Academia and in Pharma Industry) to design and develop novel product (e.g. small molecules), given the promising results obtained in specifically targeting the complex and hard to treat carcinomas, such as PDAC and CRC [Citation87,Citation89].
Article highlights
Ion channels must be included among new tumor antigens and their relevance in cancer is emerging in the last years.
Particularly relevant is the example represented by hERG1 (KV11.1) which is broadly expressed in cancers, but it is considered ‘an antitarget’ because of the potentially life-threatening effects of its blockade.
The most relevant and tumor-specific characteristic of hERG1 channels is that a common molecular partner of hERG1 in tumor cells is the β1 subunit of integrin receptors.
hERG1/β1 integrin complex can be considered a novel tumor antigen, as it does not occur in the heart, where hERG1 assembles with its canonical accessory subunits and it is present exclusively in tumors, especially in those advanced cancers where a hERG1 overexpression was previously detected.
It has paved the way for a novel strategy to target the hERG1/β1 integrin complex, through bispecific antibodies (scDb-hERG1-β1) capable of harnessing the complex. The scDb and its derivatives are able to inhibit relevant cancer functions, such as cell motility, angiogenesis, and metastasis in the preclinical setting.
Declaration of interest
A Arcangeli is founder and partner of MCK Therapeutics S.R.L. C Duranti is partner of MCK Therapeutics S.R.L. The authors have no other relevant affiliations or financial involvement with any organization or entity with a financial interest in or financial conflict with the subject matter or materials discussed in the manuscript apart from those disclosed.
Reviewer disclosure
Peer reviewers on this manuscript have no relevant financial or other relationships to disclose.
Additional information
Funding
References
- Globocan. 2020 [cited 2022 Jun 29]. Available from: https://gco.iarc.fr
- Kaplon H, Crescioli S, Chenoweth A, et al. Antibodies to watch in 2023. MAbs. 2023;15(1):2153410. doi: 10.1080/19420862.2022.2153410
- Wilkinson TC, Gardener MJ, Williams WA. Discovery of functional antibodies targeting ion channels. J Biomol Screen. 2015;20(4):454–467. doi: 10.1177/1087057114560698
- Zhang Z, Lu M, Qin Y, et al. Neoantigen: a new breakthrough in tumor immunotherapy. Front Immunol. 2021;12:672356. doi: 10.3389/fimmu.2021.672356
- Arcangeli A, Crociani O, Lastraioli E, et al. Targeting ion channels in cancer: a novel frontier in antineoplastic therapy. Curr Med Chem. 2009;16(1):66–93. doi: 10.2174/092986709787002835
- Prevarskaya N, Skryma R, Shuba Y. Ion channels in cancer: are cancer hallmarks oncochannelopathies? Physiol Rev. 2018;98(2):559–621. doi: 10.1152/physrev.00044.2016
- Fraser SP, Pardo LA. Ion channels: functional expression and therapeutic potential in cancer. Colloquium on Ion Channels and Cancer. EMBO Rep. 2008;9(6):512–515. doi: 10.1038/embor.2008.75
- Djamgoz MBA. Combinatorial therapy of cancer: possible advantages of involving modulators of ionic mechanisms. Cancers (Basel). 2022;14(11):2703. doi: 10.3390/cancers14112703
- Lastraioli E, Iorio J, Arcangeli A. Ion channel expression as promising cancer biomarker. Biochim Biophys Acta. 2015;1848(10 Pt B):2685–2702. doi: 10.1016/j.bbamem.2014.12.016
- Huang X, Jan LY. Targeting potassium channels in cancer. J Cell Bio. 2014;206(2):151–62. doi: 10.1083/jcb.201404136
- Pardo LA, Stühmer W. The roles of K(+) channels in cancer. Nat Rev Cancer. 2014;14(1):39–48. doi: 10.1038/nrc3635
- Arcangeli A. Ion channels and transporters in cancer. 3. Ion channels in the tumor cell-microenvironment cross talk. Am J Physiol Cell Physiol. 2011;301(4):C762–71. doi: 10.1152/ajpcell.00113.2011
- Becchetti A. Ion channels and transporters in cancer. 1. Ion channels and cell proliferation in cancer. Am J Physiol Cell Physiol. 2011;301(2):C255–65. doi: 10.1152/ajpcell.00047.2011
- Pardo LA, Brüggemann A, Camacho J, et al. Cell cycle-related changes in the conducting properties of r-eag K+ channels. J Cell Bio. 1998;143(3):767–775. doi: 10.1083/jcb.143.3.767
- Ouadid-Ahidouch H, Ahidouch A, Pardo LA. Kv10.1 K(+) channel: from physiology to cancer. Pflugers Arch. 2016;468(5):751–762. doi: 10.1007/s00424-015-1784-3
- Arcangeli A, Bianchi L, Becchetti A, et al. A novel inward-rectifying K+ current with a cell-cycle dependence governs the resting potential of mammalian neuroblastoma cells. J Physiol. 1995;489:455–471. doi: 10.1113/jphysiol.1995.sp021065
- Becchetti A, Duranti C, Arcangeli A. Dynamics and physiological meaning of complexes between ion channels and integrin receptors: the case of Kv11.1. Am J Physiol Cell Physiol. 2022;322(6):C1138–C1150. doi: 10.1152/ajpcell.00107.2022
- Wulff H, Christophersen P, Colussi P, et al. Antibodies and venom peptides: new modalities for ion channels. Nat Rev Drug Discov. 2019;18(5):339–357. doi: 10.1038/s41573-019-0013-8
- Warmke JW, Ganetzky B. A family of potassium channel genes related to eag in drosophila and mammals. Proc Natl Acad Sci USA. 1994;91:3438–3442. doi: 10.1073/pnas.91.8.3438
- Vandenberg JI, Perry MD, Perrin MJ, et al. hERG K(+) channels: structure, function, and clinical significance. Physiol Rev. 2012;92(3):1393–1478.
- Morais Cabral JH, Lee A, Cohen SL, et al. Crystal structure and functional analysis of the HERG potassium channel N terminus: a eukaryotic PAS domain. Cell. 1998;95(5):649–655. doi: 10.1016/s0092-8674(00)81635-9
- Bett GC, Rasmusson RL. Functionally-distinct proton-binding in HERG suggests the presence of two binding sites. Cell Biochem Biophys. 2003;39(3):183–194. doi: 10.1385/CBB:39:3:183
- Bezanilla F. The voltage sensor in voltage-dependent ion channels. Physiol Rev. 2000;80:555–592. doi: 10.1152/physrev.2000.80.2.555
- Bezzina CR, Verkerk AO, Busjahn A, et al. A common polymorphism in KCNH2 (HERG) hastens cardiac repolarization. Cardiovasc Res. 2003;59(1):27–36.
- Bhuiyan ZA, Momenah TS, Gong Q, et al. Recurrent intrauterine fetal loss due to near absence of HERG: clinical and functional characterization of a homozygous nonsense HERG Q1070X mutation. Heart Rhythm. 2008;5:553–561. doi: 10.1016/j.hrthm.2008.01.020
- Bhuvanagiri M, Schlitter AM, Hentze MW, et al. NMD: RNA biology meets human genetic medicine. Biochem J. 2010;430:365–377. doi: 10.1042/BJ20100699
- Bian J, Cui J, McDonald TV. HERG K+ channel activity is regulated by changes in phosphatidyl inositol 4,5-bisphosphate. Circ Res. 2001;89:1168–1176. doi: 10.1161/hh2401.101375
- Bian JS, Kagan A, McDonald TV. Molecular analysis of PIP2 regulation of HERG and IKr. Am J Physiol Heart Circ Physiol. 2004;287:H2154–H2163. doi: 10.1152/ajpheart.00120.2004
- Bian JS, McDonald TV. Phosphatidylinositol 4,5-bisphosphate interactions with the HERG K+ channel. Pflügers Arch. 2007;455:105–113. doi: 10.1007/s00424-007-0292-5
- Arcangeli A, Bianchi L, Becchetti A, et al. A novel inward-rectifying K+ current with a cell-cycle dependence governs the resting potential of mammalian neuroblastoma cells. J Physiol. 1995;489(2):455. doi: 10.1113/JPHYSIOL.1995.SP021065
- Binggeli R, Weinstein R. Membrane potentials and sodium channels: hypotheses for growth regulation and cancer formation based on changes in sodium channels and gap junctions. J Theor Biol. 1986;123:377–401. doi: 10.1016/S0022-5193(86)80209-0
- Bianchi L, Wible B, Arcangeli A, et al. Herg encodes a K+ Current highly conserved in tumors of different histogenesis: a selective advantage for cancer cells? Cancer Res. 1998;58(4):815–822.
- Cherubini A, Taddei GL, Crociani O, et al. HERG potassium channels are more frequently expressed in human endometrial cancer as compared to non-cancerous endometrium. Br J Cancer. 2000;83:1722–1729. doi: 10.1054/bjoc.2000.1497
- Muratori L, Petroni G, Antonuzzo L, et al. HERG1 positivity and glut-1 negativity identifies high-risk TNM stage I and II colorectal cancer patients, regardless of adjuvant chemotherapy. Onco Targets Ther. 2016;9. doi: 10.2147/OTT.S114090
- Iorio J, Lastraioli E, Tofani L, et al. hERG1 and HIF-2α behave as biomarkers of positive response to Bevacizumab in metastatic colorectal cancer patients. Translational Oncology. 2020;13(3):100740.
- Lastraioli E, Romoli MR, Iorio J, et al. the hERG1 potassium channel behaves as prognostic factor in gastric dysplasia endoscopic samples. OTT. 2019;12:9377–9384. doi: 10.2147/OTT.S226257
- Iorio J, Meattini I, Bianchi S, et al. hERG1 channel expression associates with molecular subtypes and prognosis in breast cancer. Cancer Cell Int. 2018;18(1). doi: 10.1186/s12935-018-0592-1
- Lastraioli E, Pillozzi S, Mari A, et al. hERG1 and CA IX expression are associated with disease recurrence in surgically resected clear cell renal carcinoma. European J Surg Oncol. 2019;46(1):209–215. doi: 10.1016/j.ejso.2019.10.031
- Lastraioli E, Gasperi Campani F, Taddei A, et al. hERG1 channels are overexpressed in human gastric cancer and their activity regulates cell proliferation: a novel prognostic and therapeutic target? In Proceedings of 6th IGCC; Yokohama, Japan; 2005. p. 151–154.
- Shao XD, Wu KC, Guo XZ, et al. Expression and significance of HERG protein in gastric cancer. Cancer Biol Ther. 2008;7(1):45–50.
- Ding X, Luo H, Luo B, et al. Overexpression of hERG1 in resected esophageal squamous cell carcinomas: a marker for poor prognosis. J Surg Oncol. 2008;97:57–62. doi: 10.1002/JSO.20891
- Ding X, Yang W, Gao S, et al. Prognostic significance of hERG1 expression in gastric cancer. Dig Dis Sci. 2010;55:1004–1010. doi: 10.1007/S10620-009-0834-0
- Dolderer JH, Schuldes H, Bockhorn H, et al. HERG1 gene expression as a specific tumor marker in colorectal tissues. Eur J Surg Oncol. 2010;36:72–77. doi: 10.1016/j.ejso.2009.05.009
- Lastraioli E, Guasti L, Crociani O, et al. herg1 gene and HERG1 protein are overexpressed in colorectal cancers and regulate cell invasion of tumor cells. Cancer Res. 2004;64(2):606–611.
- Lastraioli E, Taddei A, Messerini L, et al. hERG1 channels in human esophagus: evidence for their aberrant expression in the malignant progression of Barrett’s esophagus. J Cell Physiol. 2006;209. doi: 10.1002/jcp.20748
- Crociani O, Lastraioli E, Boni L, et al. HERG1 channels regulate VEGF-A secretion in human gastric cancer: clinicopathological correlations and therapeutical implications. Clin Cancer Res. 2014;20(6):1502–1512.
- Lastraioli E, Perrone G, Sette A, et al. HERG1 channels drive tumour malignancy and may serve as prognostic factor in pancreatic ductal adenocarcinoma. Br J Cancer. 2015;112(6):1076–1087.
- Lastraioli E, Lottini T, Bencini L, et al. HERG1 potassium channels: novel biomarkers in human solid cancers. Biomed Res Int. 2015;2015:1–9. doi: 10.1155/2015/896432
- Lastraioli E, Bencini L, Bianchini E, et al. hERG1 channels and glut-1 as independent prognostic indicators of worse outcome in stage I and II colorectal cancer: a pilot study. Transl Oncol. 2012;5(2):105–112.
- Crociani O, Zanieri F, Pillozzi S, et al. Herg1 channels modulate integrin signaling to trigger angiogenesis and tumor progression in colorectal cancer. Sci Rep. 2013;3(1). doi: 10.1038/srep03308
- Arcangeli A, Becchetti A. Novel perspectives in cancer therapy: targeting ion channels, drug resistance updates. Drug Resist Updat. 2015;21–22:11–19. doi: 10.1016/j.drup.2015.06.002
- Thomas D, Gut B, Karsai S, et al. Inhibition of cloned HERG potassium channels by the antiestrogen tamoxifen Naunyn Schmiedebergs Arch. Pharmacol. 2003;368(1):41–48. doi: 10.1007/s00210-003-0766-8
- Capitani C. Ion channels and transporters in breast cancer: promising targets for novel recombinant antibodies. Siena, Italy: University of Siena. Genomec; 2022/2023.
- Lansu K, Gentile S. Potassium channel activation inhibits proliferation of breast cancer cells by activating a senescence program cell death dis. 2013. p. e652. doi: 10.1038/cddis.2013.174
- Glassmeier G, Hempel K, Wulfsen I, et al. Inhibition of HERG1 K(+) channel protein expression decreases cell proliferation of human small cell lung cancer cells pflugers arch. Pflügers Archiv – European J Physiol. 2012;463(2):365–376.
- Chen SZ, Jiang M, Zhen YS. HERG K + channel expression-related chemosensitivity in cancer cells and its modulation by erythromycin. Cancer Chemother Pharmacol. 2005;56(2):212–220. doi: 10.1007/s00280-004-0960-5
- Iorio J, Duranti C, Lottini T, et al. KV11.1 potassium channel and the Na+/H+ antiporter NHE1 modulate adhesion-dependent intracellular pH in colorectal cancer cells. Front Pharmacol. 2020;11:848. doi: 10.3389/fphar.2020.00848
- Pillozzi S, D’Amico M, Bartoli G, et al. The combined activation of KCa3.1 and inhibition of Kv11.1/hERG1 currents contribute to overcome Cisplatin resistance in colorectal cancer cells. Br J Cancer. 2018;118(2):200–212. doi: 10.1038/bjc.2017.392
- Petroni G, Bagni G, Iorio J, et al. Correction: clarithromycin inhibits autophagy in colorectal cancer by regulating the hERG1 potassium channel interaction with PI3K. Cell Death Dis. 2020;11(3):209. doi: 10.1038/s41419-020-2407-2
- Manoli S, Coppola S, Duranti C, et al. The activity of kv 11.1 potassium channel modulates F-Actin organization during cell migration of pancreatic ductal adenocarcinoma cells. Cancers (Basel). 2019;11(2):135. doi: 10.3390/cancers11020135
- Pillozzi S, Brizzi MF, Bernabei PA, et al. VEGFR-1 (FLT-1), beta1 integrin, and hERG K+ channel for a macromolecular signaling complex in acute myeloid leukemia: role in cell migration and clinical outcome. Blood. 2007;110(4):1238–1250. doi: 10.1182/blood-2006-02-003772
- Zhang R, Tian P, Chi Q, et al. Human ether-à-go-go-related gene expression is essential for cisplatin to induce apoptosis in human gastric cancer oncol. Rep. 2012;27(2):433–440. doi: 10.3892/or.2011.1515
- Zhao J, Wei XL, Jia YS, et al. Silencing of herg gene by shRNA inhibits SH-SY5Y cell growth in vitro and in vivo. Eur J Pharmacol. 2008;579(1–3):50–7. doi: 10.1016/j.ejphar.2007.10.008
- Lottini T, Iorio J, Lastraioli E, et al. Transgenic mice overexpressing the LH receptor in the female reproductive system spontaneously develop endometrial tumour masses. Sci Rep. 2021;11(1):8847. doi: 10.1038/s41598-021-87492-5
- Masi A, Becchetti A, Restano-Cassulini R, et al. hERG1 channels are overexpressed in glioblastoma multiforme and modulate VEGF secretion in glioblastoma cell lines. Br J Cancer. 2005;93(7):781–92. doi: 10.1038/sj.bjc.6602775
- Iorio J, Antonuzzo L, Scarpi E, et al. Prognostic role of hERG1 potassium channels in neuroendocrine tumours of the ileum and pancreas. Int J Mol Sci. 2022;23(18):10623. doi: 10.3390/ijms231810623
- Zeng W, Liu Q, Chen Z, et al. Silencing of hERG1 gene inhibits proliferation and invasion, and induces apoptosis in human osteosarcoma cells by targeting the NF-κB pathway. J Cancer. 2016;7(6):746–57. doi: 10.7150/jca.13289
- Menéndez ST, Villaronga MÁ, Rodrigo JP, et al. HERG1A potassium channel is the predominant isoform in head and neck squamous cell carcinomas: evidence for regulation by epigenetic mechanisms. Sci Rep. 2016;6:19666. doi: 10.1038/srep19666
- Fernández-Valle Á, Rodrigo JP, Rodríguez-Santamarta T, et al. HERG1 potassium channel expression in potentially malignant disorders of the oral mucosa and prognostic relevance in oral squamous cell carcinoma. Head Neck. 2016;38(11):1672–1678.
- Asher V, Khan R, Warren A, et al. The Eag potassium channel as a new prognostic marker in ovarian cancer. Diagn Pathol. 2010;5:78. doi: 10.1186/1746-1596-5-78
- Asher V, Warren A, Shaw R, et al. The role of Eag and HERG channels in cell proliferation and apoptotic cell death in SK-OV-3 ovarian cancer cell line. Cancer Cell Int. 2011;11:6. doi: 10.1186/1475-2867-11-6
- Cicek MS, Koestler DC, Fridley BL, et al. Epigenome-wide ovarian cancer analysis identifies a methylation profile differentiating clear-cell histology with epigenetic silencing of the HERG K+ channel. Hum Mol Genet. 2013;22:3038–3047. doi: 10.1093/hmg/ddt160
- Arcangeli A, Romoli MR, Boni L, et al. High hERG1 expression in advanced melanoma. Melanoma Res. 2013;23:185–190. doi: 10.1097/CMR.0b013e32835fc6c9
- Arcangeli A, Becchetti A. Complex functional interaction between integrin receptors and ion channels. Trends Cell Biol. 2006;16(12):631–9. doi:10.1016/j.tcb.2006.10.003
- Becchetti A, Petroni G, Arcangeli A. Ion channel conformations regulate integrin-dependent signaling. Trends Cell Biol. 2019;29:298–307. doi: 10.1016/j.tcb.2018.12.005
- Cherubini A, Hofmann G, Pillozzi S, et al. Human ether-a-go-go –related gene 1 channels are physically linked to β 1 integrins and modulate adhesion-dependent signaling. Mol Biol Cell. 2005;16(6):2972–2983.
- Sanguinetti MC, Tristani-Firouzi M. hERG potassium channels and cardiac arrhythmia. Nature. 2006;440(7083):463–9. doi: 10.1038/nature04710
- Raschi E, Vasina V, Poluzzi E, et al. The hERG Kfl channel: target and antitarget strategies in drug development. Pharmacol Res. 2008;57(3):181–195.
- Arcangeli A. Chapter 17 expression and role of hERG channels in cancer cells book. In: Chadwick DJ Goode J, editors. 64 book series:novartis foundation symposia series editor(s): novartis foundation. First published: 11 Feb 2005. doi: 10.1002/047002142X.ch17Citations
- Pointer KB, Clark PA, Eliceiri KW. Administration of non-torsadogenic human ether-à-go-go-related gene inhibitors is associated with better survival for high hERG-expressing glioblastoma patients. Clin Cancer Res. 2017;23(1):73–80. doi: 10.1158/1078-0432.CCR-15-3169
- Pillozzi S, Masselli M, Gasparoli L, et al. Macrolide antibiotics have an antileukemic effect by modulating the autophagic flux through the involvement of hERG1 potassium channels. Blood Cancer J. 2016;6(5):e423.
- Phartiyal P, Jones EMC, Robertson GA. Heteromeric assembly of human ether-à-go-go-related gene (hERG) 1a/1b channels occurs cotranslationally via N-terminal interactions. J Biol Chem. 2007;282(13):9874–9882. doi:10.1074/jbc.M610875200
- Jones DK, Liu F, Vaidyanathan R, et al. hERG 1b is critical for human cardiac repolarization. Proc Natl Acad Sci USA. 2014;111(50):18073–7. doi: 10.1073/pnas.1414945111
- Gasparoli L, D’Amico M, Masselli M, et al. New pyrimido-indole compound CD-160130 preferentially inhibits the K V 11.1B isoform and produces antileukemic effects without Cardiotoxicity. Mol Pharmacol. 2015;87(2):183–196.
- Hofmann G, Bernabei PA, Crociani O, et al. HERG K+ channels activation during β1 integrin-mediated adhesion to fibronectin induces an up-regulation of αvβ3 integrin in the preosteoclastic leukemia cell line FLG 29.1. J Biol Chem. 2001;276:4923–4931. doi: 10.1074/jbc.M005682200
- Becchetti A, Crescioli S, Zanieri F, et al. The conformational state of Kv11.1 channels determines integrin association, downstream signaling, and cancer progression. Sci Signal. 2017;10(473):eaaf3236. doi: 10.1126/scisignal.aaf3236
- Duranti C, Iorio J, Bagni G, et al. Integrins regulate hERG1 dynamics by girdin-dependent Gαi3: signaling and modeling in cancer cells. LSA. 2023;7(1):e202302135. doi: 10.26508/lsa.202302135
- Arcangeli A, Becchetti A, Mannini A, et al. Integrin-mediated neurite outgrowth in neuroblastoma cells depends on the activation of potassium channels. J Cell Bio. 1993;122:1131–1143. doi: 10.1083/jcb.122.5.1131
- Duranti C, Iorio J, Lottini T, et al. Harnessing the hErg1/β1 integrin complex via a novel bispecific single-chain antibody: an effective strategy against solid cancers. Mol Cancer Ther. 2021;20(8):1338–1349. doi: 10.1158/1535-7163.MCT-20-1111
- Capitani C, Iorio J, Duranti C, et al. Physical and functional association of potassium (hERG1) and sodium (neonatal Nav1.5) channel protein in breast cancer. EACR 2022 Congress: Innovative Cancer Science – Translating Biology to Medicine 20–23 June 2022 | Seville; Spain.
- Lastraioli E. Manuscript in preparation.
- Lottini T, Martinelli M, Duranti C, et al. Study of the effects of a combination therapy in subcutaneous mouse models of colorectal cancer (CRC) with ultrasound and photoacoustic imaging. World Molecular Imaging Society Congress (WMIC); 5–9 Sep 2023; Prague, Czech Republic.
- Lottini T, Duranti C, Iorio J, et al. Combination therapy with a bispecific antibody targeting the hErg1/β1 integrin complex and gemcitabine in pancreatic ductal adenocarcinoma. Cancers (Basel). 2023;15(7):2013. doi: 10.3390/cancers15072013
- Duranti CV, Iorio J, Bagni G, et al. Potassium channels affect tumor malignancy acting through lipid rafts: therapeutic effects of cholesterol lowering drugs in combination with potassium channel targeting antibodies. ISCaM2023 – 10th Annual Meeting Systemic Metabolism and Cancer; The Francis Crick Instistut, London, UK; 2023 Sep 17–19.
- Duranti C, Arcangeli A. Ion Channel Targeting with Antibodies and Antibody Fragments for Cancer Diagnosis. Antibodies (Basel). 2019;8(22):33 . doi: 10.3390/antib8020033
- Sala C, Staderini M, Lottini T, et al. Expression of the ether-a-gò-gò-related gene 1 channel during B and T lymphocyte development: role in BCR and TCR signaling. Front Immunol. 2023;14:1111471. doi: 10.3389/fimmu.2023.1111471
- Picchi M. Biotechnology Bachelor Thesis: Immunohistochemical evaluation of hERG1 and hERG1/β1 Integrin complex expression in a case series of MALT lymphomas: clinical correlations and response to clarithromycin therapy. Academic year 2020/2021. University of Florence.
- “Novel antibodies” n.102017000083637; PCT/EP2018/06764; n. WO2019/015936. Inventors: Annarosa Arcangeli, Claudia Duranti, Laura Carraresi, Silvia Crescioli.
- Sanguinetti MC, Jiang C, Curran ME, et al. A mechanistic link between an inherited and an acquired cardiac arrhythmia: HERG encodes the IKr potassium channel. Cell. 1995;81(2):299–307. doi: 10.1016/0092-8674(95)90340-2
- Garrido A, Lepailleur A, Mignani SM, et al. hERG toxicity assessment: useful guidelines for drug design. Eur J Med Chem. 2020;195:112290. doi: 10.1016/j.ejmech.2020.112290
- Duranti C, Carraresi L, Sette A, et al. Generation and characterization of novel recombinant anti-hERG1 scFv antibodies for cancer molecular imaging. Oncotarget. 2018;9(79):34972–34989. doi: 10.18632/oncotarget.26200
- Duranti C, Lastraioli E, Iorio J, et al. Expression and purification of a novel single-chain diabody (scDb-hErg1/β1) from Pichia pastoris transformants. Protein Expr Purif. 2021;184:105879. doi: 10.1016/j.pep.2021.105879
- Zhang JH, Shan LL, Liang F, et al. Strategies and considerations for improving recombinant antibody production and quality in Chinese hamster ovary cells. Front Bioeng Biotechnol. 2022;10:856049. doi: 10.3389/fbioe.2022.856049
- Santini L, Duranti C, Palandri C, et al. Cardiac safety assessment of a novel recombinant bispecific antibody targeting the ether-à-go-go related gene 1 (hERG1)-β1 integrin macromolecular complex. Front Pharmacol. 2023;14. doi: 10.3389/fphar.2023.1237431
- Colasurdo R, Duranti C, Iorio J, et al. Manuscript in preparation.