ABSTRACT
Objectives
Radioiodine-refractory differentiated thyroid cancer (RAI-rDTC) has frequently been associated with poor prognosis. We conducted a meta-analysis of published randomized controlled trials to evaluate multi-kinase inhibitors’ efficacy and safety profile treatment.
Methods
A comprehensive search was conducted using PubMed, Embase, Cochrane, and Medline databases. The quality of literature and trial risk of bias was assessed using the Cochrane risk of bias tool, while the results of progression-free survival (PFS), overall survival (OS), and adverse events (AEs) were evaluated using RevMan5.3 software.
Results
Treatment with MKIs significantly improved PFS and OS, but AEs were significantly higher than those in the control group (P < 0.01). The studies demonstrated the median PFS (HR 0.30, 95% CI: 0.18–0.50, P < 0.00001) and OS (HR 0.70, 95% CI: 0.57–0.88, P = 0.002) in RAI-rDTC patients treated with MKIs, and the median PFS of papillary thyroid carcinoma (HR0.28, 95% CI: 0.22–0.37, P < 0.00001) along with follicular thyroid carcinoma (HR0.14, 95%CI 0.09–0.24, P < 0.00001) were extended.
Conclusion
MKIs significantly prolonged PFS and OS in patients with RAI-rDTC (P < 0.01). Our recommendation is to use MKIs carefully in patients after evaluating their health status to maximize treatment benefits and minimize adverse effects.
1. Introduction
Thyroid cancer (TC) is the most common endocrine cancer, accounting for approximately 1–5% of all cancers in women, accounting for less than 2% in men [Citation1]. Differentiated thyroid carcinoma (DTC) accounts for more than 90% of all thyroid cancers, comprising papillary thyroid carcinoma (PTC), follicular thyroid carcinoma (FTC, including Hürthle cell), and poorly differentiated thyroid carcinoma (PDTC) [Citation2]. Women are more likely to develop DTC, but have a better prognosis [Citation3–5]. DTC responds well to current treatments, including surgery, l-thyroxine therapy, and radioactive iodine (RAI). However, after refractoriness to radioactive iodine, metastasis occurs in up to 15% of cases, followed by a poor prognosis [Citation6,Citation7]. The sorafenib and lenvatinib of multi-kinase inhibitors (MKIs) have been approved by FDA for treating RAI-rDTC. Vandetanib and cabozantinib were previously only approved for treating medullary thyroid carcinoma (MTC). Exelixis announced in September 2021 that FDA had approved cabozantinib for RAI-rDTC treatment. Apatinib, developed in China and released in December of that year, significantly improved PFS (P < 0.001) and OS (P = 0.04) in patients with RAI-rDTC [Citation8–13]. RAI-rDTC remained in clinical development yet unfavorable prognosis. We conducted a meta-analysis of published articles to evaluate strengths and weaknesses of MKIs medications, providing clinical patients with a reference point for selecting them. The mechanisms of action of these MKIs depend on the following signaling pathways, including phosphatidylinositol-3 kinase (PI3K)/AKT/mammalian target of rapamycin (mTOR), rearranged during transfection (RET)/RAS/RAF-mitogen-activated protein kinase (MAPK), janus kinase (JAK)/signal transducer and transcription activator (STAT). Vandetanib is a small molecule MKI that inhibits the vascular endothelial growth factor receptor (VEGFR)-2, the epidermal growth factor receptor (EGFR), and RET [Citation14]. Both sorafenib and lenvatinib inhibit the signaling pathways through VEGFRs 1–3, c-KIT, and RET genes. Furthermore, sorafenib targets platelet-derived growth factor receptor‐beta (PDGFR β), RAF-1, and BRAF, while lenvatinib targets fibroblast growth factor receptor1-4 (FGFR 1–4) and platelet‐derived growth factor receptor‐alpha (PDGFR-α) [Citation15]. Cabozantinib and apatinib inhibit VEFGR-2, but cabozantinib is also an MKI that targets MET, KIT, RET, AXL, and (FMS-like tyrosine kinase 3, FLT-3) [Citation16,Citation17]. Angiogenesis inhibitors inhibit endothelial cell proliferation, migration, survival, as well as vessel permeability, while EGFR blocks cell proliferation, differentiation, and survival. Inhibiting mutant genes or fusion genes can increase hypoxia, reduce tumor activity, and stimulate apoptosis in tumor cells [Citation8–13] ().
Figure 1. Multi-kinase inhibitors signaling pathway for vandetanib, sorafenib, lenvatinib cabozantinib, and apatinib. FL, FLT3 ligand; FLT3, FMS-like tyrosine kinase 3; GDNF, glial cell-derived neurotrophic factor; GFR, GFRα, GDNF family receptor-α; RET, rearranged during transfection; HGF, hepatocyte growth factor; MET, mesenchymal-epithelial transition factor; SCF, stem cell factor; c-KIT, Proto-oncogene proteins c-kit;c-SRC, c-SRC tyrosine kinase; STAT, signal transducer and activator of transcription; JAK, janus kinase; MKIs, multi-kinase inhibitors; EGF, epidermal growth factor; EGFR, epidermal growth factor receptor; VEGF, vascular endothelial growth factor; VEGFR, vascular endothelial growth factor receptor; PDGF, platelet‐derived growth factor; PDGFR, platelet‐derived growth factor receptor; FGFR, fibroblast growth factor receptor; PI3K (phosphatidylinositol-3 kinase) /AKT/ mammalian target of rapamycin (mTOR) signaling pathway; RAS/RAF/MEK/ERK signaling pathway.
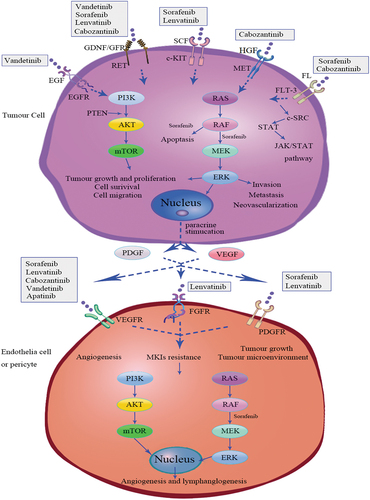
2. Methods
The literature search was conducted by using PubMed, Embase, Cochrane, and Medline databases in accordance with PRISMA guidelines. Data up to 20 December 2021, were analyzed. Medical Subject Headings (MeSH) terms and free terms were used ((Thyroid Neoplasms) OR (Neoplasm, Thyroid) OR (Thyroid Neoplasm) OR (Neoplasms, Thyroid) OR (Thyroid Carcinoma) OR (Carcinoma, Thyroid) OR (Carcinomas, Thyroid) OR (Thyroid Carcinomas) OR (Cancer of Thyroid) OR (Thyroid Cancers) OR (Thyroid Cancer) OR (Cancer, Thyroid) OR (Cancers, Thyroid) OR (Cancer of the Thyroid) OR (Thyroid Adenoma) OR (Adenoma, Thyroid) OR (Adenomas, Thyroid) OR (Thyroid Adenomas)) AND (Randomized controlled trial). Only those patients who had ‘radioiodine-refractory differentiated thyroid cancer’ and were treated with ‘multi-kinase inhibitors’ were selected in this study. Only studies designated as ‘randomized controlled clinical trials’ were included in the search strategy. Detailed search strategy is displayed in Supplementary Appendix 1.
2.1. Inclusion criteria
Clinical trials involving MKI use in treating RAI-rDTC were included if they met the following criteria: (a) Aged 18 and above; (b) DTC confirmed by histological or cytological diagnosis with no response to 131-I treatment or unable to absorb iodine or post-131-I body scan is negative [Citation18]; (c) diseases that can be measured according to Response Evaluation Criteria in Solid Tumors version1.1 (RECIST 1.1); (d) randomized controlled clinical trials with evidence of disease progression prior to enrollment and the control group was treated with placebo; (e) prior treatment no more than two MKIs; (f) adequate function of an organ, bone marrow, and blood coagulation, with serum thyroid stimulating hormone (TSH) less than the lower limit of the reference range or below the levels of 0.50 mIU/L.
2.2. Exclusion criteria
Clinical trials were excluded if they (a) included anaplastic thyroid cancer (ATC) or medullary thyroid cancer (MTC); (b) had more than two points according to Eastern Cooperative Oncology Group performance status (ECOG PS); (c) any anticancer treatment within one month prior to randomization; (d) animal experiment and in vitro experiments, systematic reviews, meta-analyses, conference abstracts, other publications of the same experiment, and subgroup analysis; (e) literature with incomplete and inaccurate statistics; studies that did not report major outcomes; (f) sample size less than 30 patients.
2.3. Data extraction
Two reviewers (JY and YF) independently screened titles, keywords, and abstracts, then, according to the selection criteria, they performed a secondary screening of the retrieved articles by reading their complete texts, followed by a final cross-check of the included information. A third reviewer (JW) would be involved in judging any possible questions. The information obtained from the articles featured the name of the first author, publication year, study design, MKIs name, earlier treatment, study characteristics (mean age and sample size), treatment (treatment of intervention group and control group, median PFS, median follow-up period and OS), in addition to outcomes on different pathological types and major AEs.
2.4. Assessment of risk of bias
Two evaluators (JY and YF) independently assessed the risk of bias using the Cochrane Risk of Bias tool. They evaluated the quality of trials in terms of random sequence generation, allocation concealment, blinding of participants and personnel, blinding of outcome assessment, data integrity, selective reporting, and other biases. Each item was evaluated as low risk, unclear risk, or high risk.
2.5. Data synthesis and analysis
Data obtained from the studies were managed using Excel 2019. RevMan5.3 was used to evaluate the heterogeneity of direct comparisons and calculate HR and 95% CI. The Chi-square test tested heterogeneity for P-value and I2 statistics. P-value was bound by 0.1 and 50% for I2 statistic. In the case of the Chi-square test P < 0.10 or I2 > 50%, which indicates a high heterogeneity, the random-effects model would be adopted, along with sensitivity analysis and subgroup analysis. Other than that, a fixed-effect model would be used when a low heterogeneity is considered.
3. Results
3.1. Search process
The literature search was conducted by the example of the established retrieval strategy through a database search of PubMed, Embase, Cochrane, and Medline, which resulted in 3747 articles. A total of 2325 studies remained after removing duplicates. After further screening, 64 studies remained, and only six met the inclusion criteria, and none met the exclusion criteria. An illustration of PRISMA selection process and PRISMA-NMA (Network Meta-Analyses) can be found in the PRISMA flow diagram as shown in . A checklist of Items is documented in Supplementary Appendix 1.
3.2. Quality assessment
Two reviewers independently reviewed six articles, all of which were double-blinded, randomized controlled trials. The blinding of outcome assessment was unclear. Only one article failed to mention the measure of allocation concealment and was thus considered unclear, as demonstrated in .
3.3. Study characteristics
A total of 1384 patients were included in six studies, including five MKIs, vandetanib, sorafenib, lenvatinib, cabozantinib, and apatinib. All studies were randomized, double-blind Phase III studies, except the vandetanib study, a randomized double-blind Phase II study. Across all studies, the sample size was greater than 30 participants, and whether MKIs were administered or not, the median PFS was significantly prolonged (P < 0.01) in . The hazard ratio of median PFS after the application in patients with different pathological types and AEs are presented in .
Table 1. Overview of literature characteristics.
Table 2. Different types of thyroid cancer and adverse events.
3.4. Clinical outcomes
3.4.1. Progression‐free survival in RAI-rDTC
The influence of the intervention groups on the median PFS of patients with RAI-rDTC was statistically significant compared to the control group (HR 0.30, 95% CI: 0.18–0.50, P < 0.00001), and the risk of death was only 30% of the control group as illustrated in ).
3.4.2. Overall survival in RAI-rDTC
P > 0.77 and I2 = 0% in the Chi-square test indicated no heterogeneity, so the random-effects model was used. Analysis results indicate that MKIs intervention of RAI-refractory cancer significantly improved OS (HR 0.70, 95% CI: 0.57–0.88, P = 0.002), whereas its risk of death was only 70% compared to the control group as displayed in ).
3.4.3. Overall survival in PTC or FTC
MKI treatment was used in thyroid cancer of various types, and the median PFS in PTC was (HR 0.28, 95% CI: 0.22–0.37, P < 0.00001) as depicted in ). The median PFS in FTC (HR 0.14, 95% CI: 0.09–0.24, P < 0.00001) were prolonged, as demonstrated in ).
3.4.4. Adverse events
AEs associated with tyrosine kinase inhibitors (TKIs) have been investigated in all clinical trials. Six AEs were analyzed, including hypertension, hand-foot skin reaction (HFSR), diarrhea, proteinuria, decreased appetite, and weight loss. In all studies, the intervention group experienced significantly more adverse events than the control group (hypertension: risk ratio (RR) 4.19, 95% CI: 2.89–6.08, P < 0.00001; HFSR: RR 14.95, 95% CI: 6.79–32.93, P < 0.00001; diarrhea: RR 6.09, 95% CI: 4.20–8.82, P < 0.00001; proteinuria: RR 10.30, 95% CI: 5.75–18.42, P < 0.00001; decreased appetite: RR 2.69, 95% CI: 1.55–4.69, P = 0.0005; decreased weight: RR 3.93, 95% CI: 2.98–5.20, P < 0.00001) as depicted in .
4. Discussion
The current meta-analysis summarizes the efficacy of MKIs and their associated AEs in RAI-rDTC. Our study is known to be the first RCTS meta-analysis to demonstrate the efficacy of MKIs in patients with RAI-rDTC. HRs of median PFS and OS were statistically significantly higher in the MKI treatment group than in the control group (P < 0.01). On the other hand, the outcome of median PFS in RAI-rDTC with P < 0.10 and I2 > 50% in the heterogeneity test exhibited severe heterogeneity. Further analysis was conducted applying the random-effects model. The sensitivity analysis found no heterogeneity. In the subgroup analysis of MKI studies, there was no heterogeneity between vandetanib and sorafenib (HR 0.60, 95% CI: 0.49–0.75, P = 0.78, I2 = 0%, P < 0.00001) and lenvatinib, cabozantinib, and apatinib studies (HR 0.20, 95% CI: 0.16–0.26, P = 0.63, I2 = 0%, P < 0.00001). Due to the earlier start of the vandetanib and sorafenib studies, a high number of patients discontinued the drugs for severe adverse reactions, resulting in a low median progression-free survival (PFS). Moreover, no other MKIs drugs were available at the time. Patients had not received MKIs prior to enrollment in vandetanib and sorafenib studies. However, in lenvatinib, cabozantinib, and apatinib studies, the median PFS and OS were satisfactory even though some or all patients in the previous study had received one or two TKI drugs. Tumor cells may be resistant to various drugs. We need to compare the pharmacological effects of different drugs. The absence of randomized trials comparing two TKI agents could complicate the selection of initial treatment references for patients with RAI-rDTC.
Among the most common or severe (but less common) AEs of antiangiogenic drugs are hypertension, fatigue, decreased weight, diarrhea, QTc prolongation, congestive heart failure, proteinuria, liver damage, bone marrow suppression, HFSR, and other rashes. During this phase II trial, vandetanib was associated with diarrhea (74%), hypertension (34%), decreased appetite (26%), and QTc prolongation (23%) [Citation8]. QTc prolongation (14%) ranked first for AEs above grade 3, indicating that cardiotoxicity was the most severe AE of vandetanib. The phase III clinical trial (NCT01876784) of vandetanib showed no statistical significance in PFS (10.0 versus 5.7 months, P = 0.080) compared with that of the placebo group. The high drop-out ratio of participants could be attributed to the cardiotoxicity of vandetanib [Citation19]. Sorafenib was the first targeted drug approved by FDA to treat RAI-rDTC in November 2013 [Citation20]. HFSR (76.3%), the most common AE, can interrupt the dose of sorafenib [Citation9]. Kinase inhibitors are well-known to cause cutaneous and mucosal side effects [Citation21]. Based on a meta-analysis, HFSR was found to be an effective indicator for the treatment of hepatocellular carcinoma with sorafenib [Citation22]. Lenvatinib has a higher average PFS and OS values, a higher objective response rate, and some manageable side effects despite these benefits [Citation9,Citation10,Citation23–25]. In the treatment guidelines for thyroid carcinoma published by the National Comprehensive Cancer Network (NCCN) in September 2021, lenvatinib was listed as the first recommended regimen for RAI-rDTC. Lenvatinib benefited patients with a small baseline tumor, low tumor burden, and lung metastases above 1 cm in size [Citation26–28]. SELECT study participants reported specific AEs with lenvatinib including hypertension (67.8%), HFSR (31.8%), and proteinuria (31.0%). According to Kiyota et al. [Citation29], Japanese patients had a higher incidence of treatment-related hypertension in the SELECT study, but its efficacy and safety were generally comparable to the general population. Cabozantinib has been reported as a TKI regardless of the previous VEGFR targeted therapy [Citation30]. AEs of cabozantinib are mainly related to the digestive system, including diarrhea (51%), nausea (24%), and decreased appetite (23%) [Citation11]. Elisei et al. [Citation31]reported gastrointestinal perforations (3.3%), hemorrhage (3.3%), and fistula development (0.5%) in the cabozantinib arm. Apatinib and lenvatinib are highly selective VEFGR-2 inhibitors [Citation10,Citation17], and the common AEs reported of apatinib were also hypertension (87.0%), HFSR (87.0%), and proteinuria (76.1%). A randomized controlled clinical phase II clinical study of the anlotinib in China has demonstrated significant benefit for PFS (40.54 versus 8.38 months, HR 0.21, 95% CI: 0.12–0.37, P < 0.0001) [Citation32]. Studies on donafenib, pazopanib, sunitinib, selumetinib, and axitinib have shown promising results, but it is expected that further studies will be conducted to ascertain the clinical effectiveness of these drugs [Citation33–37].
Similarly, we analyzed the median PFS of patients with various pathologies and found that MKIs significantly correlated with PTC and FTC (P < 0.00001). The recurrence and metastasis of thyroid cancer are associated with several specific genetic alterations. PTC is associated with BRAF mutations (29–83%), RAS mutations (0–21%), RET rearrangements (14–43%), and NTRK rearrangements (3–13%), FTC is associated with RAS mutations (40–53%) [Citation38]. Studies have revealed that BRAF and RAS mutations were linked to poor prognosis in DTC patients [Citation39,Citation40]. Both studies [Citation9,Citation10] reported that sorafenib and lenvatinib demonstrated a higher PFS than the control group regardless of the status of BRAF and RAS. Vemurafenib and dabrafenib are type I BRAF kinase inhibitors, functioning as ATP-competitive inhibitors [Citation41]. Two cohorts of patients in a phase II trial with metastatic BRAF V600E mutant papillary carcinoma and refractory RAI received vemurafenib in a phase II trial. A 38.5% partial response (PR) and a median progression-free survival (PFS) of 18.2 months were reported in 26 patients who had never previously taken antiangiogenic multitargeted kinase inhibitors. In 25 patients treated with antiangiogenic multitarget kinase inhibitors, 27.3% achieved a PR, and the median PFS was 8.9 months [Citation42]. In another clinical trial conducted on 14 patients with progressive radioiodine-refractory disease, the partial response rate (PRR) of treatment with dabrafenib was 29%, and the median PFS was 11.3 months [Citation43]. Although BRAF kinase inhibitors are used as second-line therapeutic agents after antiangiogenic kinase inhibitors, more research and data are needed to confirm their efficacy. For patients with a RET fusion gene, selpercatinib or pralsetinib is an option [Citation25]. In Europe, larotrectinib [Citation44] and entrectinib [Citation45] have been approved for treating NTRK fusion gene-positive patients with DTC. Pembrolizumab can be recommended for patients with a high mutation load.
The meta-analysis showed low heterogeneity and high reliability, but our study has limitations. First, the clinical trials we selected included fewer patients with Hürthle cell and poorly differentiated thyroid carcinoma, so they may not apply to these patients. Second, three trials did not make it to the final analysis time for OS, so the results may be biased. Although apatinib has the highest efficacy of the included studies, a third consideration is that this study was only conducted in China and may not apply to other races. Lastly, no randomized trials comparing MKIs with second-line agents or any two TKI agents can complicate the selection of initial treatment references for patients with RAI-rDTC. More studies are needed, to determine a most effective MKIs drug.
5. Conclusion
MKIs are a promising treatment for patients with RAI-rDTC. Currently, MKIs are the preferred treatment for RAI-rDTC patients, the use of MKIs can significantly improve PFS and OS (P < 0.01). Both PTC and FTC patients can use MKIs, but it is necessary to pay attention to the side effects of related medications and choose medications based on their economic and health status. To achieve the optimal outcome, the goal of treatment is to improve the patient’s quality of life. To achieve a long-term sustainable outcome, efforts should also be made to manage adverse events and focus on specific efficacy, with ongoing research.
Declaration of interest
The authors have no relevant affiliations or financial involvement with any organization or entity with a financial interest in or financial conflict with the subject matter or materials discussed in the manuscript. This includes employment, consultancies, honoraria, stock ownership or options, expert testimony, grants or patents received or pending, or royalties.
Reviewer disclosures
A reviewer on this paper has received honoraria from Exelixis.
Another reviewer on this paper has served as an invited speaker or participated on the advisory board for EISAI, Bayer, Lilly and Ipsen.
The remaining reviewers have no other relevant financial relationships or otherwise to disclose.
Supplemental Material
Download MS Word (37.4 KB)Supplementary material
Supplemental data for this article can be accessed here
Additional information
Funding
References
- La Vecchia C, Malvezzi M, Bosetti C, et al. Thyroid cancer mortality and incidence: a global overview. Int J Cancer. 2015;136(9):2187–2195.
- Prete A, Borges de Souza P, Censi S, et al. Update on fundamental mechanisms of thyroid cancer. Front Endocrinol (Lausanne). 2020;11:102.
- Suteau V, Munier M, Briet C, et al. Sex bias in differentiated thyroid cancer. Int J Mol Sci. 2021;22(23):12992.
- Zahedi A, Bondaz L, Rajaraman M, et al. Risk for thyroid cancer recurrence is higher in men than in women independent of disease stage at presentation. Thyroid. 2020;30(6):871–877.
- Zhang D, Tang J, Kong D, et al. Impact of gender and age on the prognosis of differentiated thyroid carcinoma: a retrospective analysis based on SEER. Horm Cancer. 2018;9(5):361–370.
- Lirov R, Worden FP, Cohen MS. The treatment of advanced thyroid cancer in the age of novel targeted therapies. Drugs. 2017;77(7):733–745.
- Durante C, Haddy N, Baudin E, et al. Long-term outcome of 444 patients with distant metastases from papillary and follicular thyroid carcinoma: benefits and limits of radioiodine therapy. J Clin Endocrinol Metab. 2006;91(8):2892–2899.
- Leboulleux S, Bastholt L, and Krause T, et al. Vandetanib in locally advanced or metastatic differentiated thyroid cancer: a randomised, double-blind, phase 2 trial. Lancet Oncol. 2012;13(9):897–905.
- Brose MS, Nutting CM, and Jarzab B, et al. Sorafenib in radioactive iodine-refractory, locally advanced or metastatic differentiated thyroid cancer: a randomised, double-blind, phase 3 trial. Lancet. 2014;384(9940):319–328.
- Schlumberger M, Tahara M, and Wirth LJ, et al. Lenvatinib versus placebo in radioiodine-refractory thyroid cancer. N Engl J Med. 2015;372(7):621–630.
- Brose MS, Robinson B, and Sherman SI, et al. Cabozantinib for radioiodine-refractory differentiated thyroid cancer (COSMIC-311): a randomised, double-blind, placebo-controlled, phase 3 trial. Lancet Oncol. 2021;22(8):1126–1138.
- Zheng X, Xu Z, Ji Q, et al. A randomized, Phase III Study of lenvatinib in Chinese patients with radioiodine-refractory differentiated thyroid cancer. Clin Cancer Res. 2021;27(20):5502–5509.
- Lin Y, Qin S, and Li Z, et al. Apatinib vs placebo in patients with locally advanced or metastatic, radioactive iodine-refractory differentiated thyroid cancer: the reality randomized clinical trial. JAMA Oncol. 2022;8(2):242–250.
- Morabito A, Piccirillo MC, Falasconi F, et al. Vandetanib (ZD6474), a dual inhibitor of vascular endothelial growth factor receptor (VEGFR) and epidermal growth factor receptor (EGFR) tyrosine kinases: current status and future directions. Oncologist. 2009;14(4):378–390.
- Matsui J, Funahashi Y, Uenaka T, et al. Multi-kinase inhibitor E7080 suppresses lymph node and lung metastases of human mammary breast tumor MDA-MB-231 via inhibition of vascular endothelial growth factor-receptor (VEGF-R) 2 and VEGF-R3 kinase. Clin Cancer Res. 2008;14(17):5459–5465.
- Santoni M, Iacovelli R, Colonna V, et al. Antitumor effects of the multi-target tyrosine kinase inhibitor cabozantinib: a comprehensive review of the preclinical evidence. Expert Rev Anticancer Ther. 2021;21(9):1029–1054.
- Tian S, Quan H, Xie C, et al. YN968D1 is a novel and selective inhibitor of vascular endothelial growth factor receptor-2 tyrosine kinase with potent activity in vitro and in vivo. Cancer Sci. 2011;102(7):1374–1380.
- Vaisman F, Carvalho DP, Vaisman M. A new appraisal of iodine refractory thyroid cancer. Endocr Relat Cancer. 2015;22(6):R301–10.
- Al-Jundi M, Thakur S, Gubbi S, et al. Novel targeted therapies for metastatic thyroid cancer-A comprehensive review. Cancers (Basel). 2020;12(8):2104.
- White PT, Cohen MS. The discovery and development of sorafenib for the treatment of thyroid cancer. Expert Opin Drug Discov. 2015;10(4):427–439.
- Lupu I, Voiculescu N, Bacalbasa N, et al. Cutaneous complications of molecular targeted therapy used in oncology. J Med Life. 2016;9(1):19–25.
- Wang P, Tan G, Zhu M, et al. Hand-foot skin reaction is a beneficial indicator of sorafenib therapy for patients with hepatocellular carcinoma: a systemic review and meta-analysis. Expert Rev Gastroenterol Hepatol. 2018;12(1):1–8.
- Verburg FA, Amthauer H, Binse I, et al. Questions and controversies in the clinical application of tyrosine kinase inhibitors to treat patients with radioiodine-refractory differentiated thyroid carcinoma: expert perspectives. Horm Metab Res. 2021;53(3):149–160.
- Yu ST, Ge JN, Luo JY, et al. Treatment-related adverse effects with TKIs in patients with advanced or radioiodine refractory differentiated thyroid carcinoma: a systematic review and meta-analysis. Cancer Manag Res. 2019;11:1525–1532.
- Tsoli M, Alexandraki KI, Spei ME, et al. Anti-tumor activity and safety of multikinase inhibitors in advanced and/or metastatic thyroid cancer: a systematic review and network meta-analysis of randomized controlled trials. Horm Metab Res. 2020;52(1):25–31.
- Kiyota N, Tahara M, Robinson B, et al. MO3-4 Impact of baseline tumor size on overall survival in patients with RR-DTC treated with lenvatinib (SELECT) [Conference Abstract]. Ann Oncol. 2021;32:S296.
- Reuter C, Yu X, Baig M, et al. Baseline characteristics of responders and nonresponders from the phase 3 study of (E7080) lenvatinib in differentiated cancer of the thyroid (SELECT) [Conference Abstract]. Eur J Cancer. 2017;72:S112–S3.
- Tahara M, Kiyota N, Hoff AO, et al. Impact of lung metastases on overall survival in the phase 3 SELECT study of lenvatinib in patients with radioiodine-refractory differentiated thyroid cancer. Eur J Cancer. 2021;147:51–57.
- Kiyota N, Schlumberger M, Muro K, et al. Subgroup analysis of Japanese patients in a phase 3 study of lenvatinib in radioiodine-refractory differentiated thyroid cancer. Cancer Sci. 2015;106(12):1714–1721.
- Brose M, Robinson B, Sherman S, et al. Cabozantinib versus placebo in patients with radioiodine-refractory differentiated thyroid cancer (dtc) who have progressed after prior vegfr-targeted therapy: updated results from the phase 3 cosmic-311 trial and prespecified subgroup analyses based on prior vegfr-targeted therapy [Conference Abstract]. Thyroid. 2021;31(SUPPL 1):A125–A6.
- Elisei R, Schlumberger MJ, Müller SP, et al. Cabozantinib in progressive medullary thyroid cancer. J Clin Oncol. 2013;31(29):3639–3646.
- Chi Y, Gao M, Zhang Y, et al. Anlotinib in locally advanced or metastatic radioiodine-refractory differentiated thyroid carcinoma: a randomized, double-blind, multicenter phase II trial [Conference Abstract]. Ann Oncol. 2020;31:S1215.
- Lin YS, Yang H, Ding Y, et al. Donafenib in progressive locally advanced or metastatic radioactive iodine-refractory differentiated thyroid cancer: results of a randomized, multicenter Phase II trial. Thyroid. 2021;31(4):607–615.
- Bible KC, Suman VJ, Molina JR, et al. Efficacy of pazopanib in progressive, radioiodine-refractory, metastatic differentiated thyroid cancers: results of a phase 2 consortium study. Lancet Oncol. 2010;11(10):962–972.
- Bikas A, Kundra P, Desale S, et al. Phase 2 clinical trial of sunitinib as adjunctive treatment in patients with advanced differentiated thyroid cancer. Eur J Endocrinol. 2016;174(3):373–380.
- Hayes DN, Lucas AS, Tanvetyanon T, et al. Phase II efficacy and pharmacogenomic study of Selumetinib (AZD6244; ARRY-142886) in iodine-131 refractory papillary thyroid carcinoma with or without follicular elements. Clin Cancer Res. 2012;18(7):2056–2065.
- Capdevila J, Trigo JM, Aller J, et al. Axitinib treatment in advanced RAI-resistant differentiated thyroid cancer (DTC) and refractory medullary thyroid cancer (MTC). Eur J Endocrinol. 2017;177(4):309–317.
- Fukuda N, Takahashi S. Clinical indications for treatment with multi-kinase inhibitors in patients with radioiodine-refractory differentiated thyroid cancer. Cancers (Basel). 2021;13(9):2279.
- Xing M, Haugen BR, Schlumberger M. Progress in molecular-based management of differentiated thyroid cancer. Lancet. 2013;381(9871):1058–1069.
- Feng G, Luo Y, Zhang Q, et al. Sorafenib and radioiodine-refractory differentiated thyroid cancer (RR-DTC): a systematic review and meta-analysis. Endocrine. 2020;68(1):56–63.
- Holderfield M, Deuker MM, McCormick F, et al. Targeting RAF kinases for cancer therapy: BRAF-mutated melanoma and beyond. Nat Rev Cancer. 2014;14(7):455–467.
- Brose MS, Cabanillas ME, Cohen EE, et al. Vemurafenib in patients with BRAF(V600E)-positive metastatic or unresectable papillary thyroid cancer refractory to radioactive iodine: a non-randomised, multicentre, open-label, phase 2 trial. Lancet Oncol. 2016;17(9):1272–1282.
- Falchook GS, Millward M, Hong D, et al. BRAF inhibitor dabrafenib in patients with metastatic BRAF-mutant thyroid cancer. Thyroid. 2015;25(1):71–77.
- Weitzman SP, Sherman SI. Novel drug treatments of progressive radioiodine-refractory differentiated thyroid cancer. Endocrinol Metab Clin North Am. 2019;48(1):253–268.
- Chu YH, Dias-Santagata D, Farahani AA, et al. Clinicopathologic and molecular characterization of NTRK-rearranged thyroid carcinoma (NRTC). Mod Pathol. 2020;33(11):2186–2197.