ABSTRACT
Background
We wanted to evaluate if event-free survival (EFS) is a reliable surrogate for overall survival (OS) in patients with resectable non-small cell lung cancer (r-NSCLC) receiving neoadjuvant therapy. We conducted a systematic literature review and meta-analysis to investigate the statistical association between EFS and OS.
Research design and methods
Electronic databases were searched on 30 July 2021 to identify sources reporting both EFS and OS data in patients with stage I–IIIB r-NSCLC receiving neoadjuvant therapy. Correlation and regression analyses evaluated the association between the effect of treatment on EFS and OS using log-hazard ratios (HRs). Sources in which the entire population had epidermal growth factor receptor mutations were excluded from the analyses.
Results
We identified 74 sources, of which 8 reported EFS and OS HRs from randomized controlled trials. Based on these, we found a positive linear correlation and a strong association between EFS and OS log-HRs (weighted Pearson’s correlation coefficient r = 0.864; 95% confidence interval 0.809–0.992; P = 0.006; random-effects meta-regression, R2 = 0.777).
Conclusions
We found a strong association between treatment effects for EFS and OS, indicating that improvements in EFS are likely to be predictive of improvements in OS. EFS may therefore be a reliable surrogate for OS after neoadjuvant therapy in r-NSCLC.
1. Introduction
Lung cancer is the leading cause of cancer mortality worldwide [Citation1], with non-small cell lung cancer (NSCLC) accounting for 82% of lung cancer diagnoses [Citation2]. Patients whose tumors are resectable may receive neoadjuvant therapy prior to surgical resection [Citation3–5], traditionally with platinum-based chemotherapy. However, clinical trials in resectable NSCLC (r-NSCLC) are currently evaluating immunotherapy in combination with chemotherapy as neoadjuvant therapy [Citation6–10]. The first immunotherapy for r-NSCLC was approved for use in combination with chemotherapy in 2022 [Citation11]. Following surgery, patients with r-NSCLC may receive additional systemic treatment with adjuvant therapy. Radiotherapy may also be used alongside chemotherapy, either as part of neoadjuvant or adjuvant therapy [Citation5].
Overall survival (OS) is the gold standard for assessing the efficacy of treatments in NSCLC [Citation12], but for early-stage disease, such as r-NSCLC, there is a long wait for mature OS data, during which time patients may receive several lines of treatment. Waiting for mature OS data would significantly delay patient access to novel treatments. Instead, event-free survival (EFS), which measures the duration of time from randomization or the start of neoadjuvant therapy to the point of disease progression or death, is often accepted by regulators as an alternative endpoint to assess treatment efficacy in r-NSCLC [Citation13]. Pathological complete response (pCR) is another endpoint that is used to determine the response to neoadjuvant therapy, showing whether there are any viable tumor cells in the resected tissue from the primary tumor or lymph nodes following surgery.
While regulators accept alternative endpoints, payers and health technology assessment agencies may still prefer OS data to evaluate the effects of novel treatments on mortality and how this might translate to a potential gain in life-years [Citation12]. Consequently, there is interest in whether outcomes, such as EFS, can be considered as surrogates for OS, in addition to being meaningful endpoints in their own right. For example, in recent evaluations of adjuvant therapy for r-NSCLC by the National Institute for Health and Care Excellence in the UK, consideration was given to how improvements in disease-free survival (DFS), which is similar to EFS but is evaluated in the adjuvant setting, may translate to improvements in OS, in the absence of mature OS data [Citation14,Citation15].
The aim of this study was to evaluate the association between EFS and OS outcomes in patients with r-NSCLC receiving neoadjuvant therapy before surgery. We conducted a systematic literature review (SLR) to identify studies reporting both EFS and OS data in patients with r-NSCLC receiving neoadjuvant therapy, and these studies were then used in a meta-analysis to assess the association between EFS and OS.
2. Methods
2.1. SLR
A protocol for the SLR was prepared ahead of the initiation of the project and was registered with PROSPERO (CRD42021270955). Electronic databases (Embase, MEDLINE, and the Cochrane Library) were searched on 30 July 2021 using the search strings detailed in the Supplementary materials. In addition, three key congresses were searched: the World Conference on Lung Cancer, the American Society of Clinical Oncology, and the European Society for Medical Oncology. Congresses that took place between 2018 and 2021 were searched in October 2021.
Title and abstract screening and full-text review were carried out by a reviewer according to the inclusion/exclusion criteria detailed in Supplementary Table S1, with 10% of references screened by a second independent reviewer. Discrepancies were resolved by a third reviewer. As per the inclusion criteria, references reporting both EFS and OS data in patients with stage I–IIIB r-NSCLC receiving neoadjuvant therapy (including chemotherapy and/or immunotherapy) were considered for inclusion. DFS, progression-free survival (PFS), and recurrence-free survival (RFS) are terms that, correctly or otherwise, may be used interchangeably, and sometimes as synonyms of EFS. Studies that reported endpoints such as DFS, PFS, or RFS and met all other inclusion criteria were included if the outcome was measured from the time prior to neoadjuvant therapy and surgery. To allow confirmation that the endpoints evaluated were all synonymous with EFS, studies were required to report the definition of EFS, DFS, PFS, and RFS, including the time point from which the endpoint was measured.
Data were extracted into a data extraction table in Microsoft Excel by one reviewer and independently checked for errors against the original publications by a second reviewer. Extracted outcomes included the definition of EFS and OS, hazard ratios (HRs) for EFS and OS, and median EFS (mEFS) and median OS (mOS). For studies with multiple treatment arms, data were only extracted for the treatment arms that included neoadjuvant therapy for use in the subsequent analysis. For studies reporting data from multiple subgroups, data were only extracted for the overall population, if available. When reported as a subgroup of the overall population, data were also extracted for all patients who underwent surgery, in addition to the overall population.
The risk of bias was evaluated for full publications reporting randomized controlled trials (RCTs) using the Risk of Bias 2 (RoB2) tool [Citation16], and for full publications reporting data from non-RCTs using the Risk Of Bias In Non-randomized Studies – of Interventions (ROBINS-I) tool [Citation17]. Bias assessments were carried out by a single reviewer.
mEFS and mOS were visualized using bar charts that were stratified by the type of treatment received in the different treatment arms (adjuvant therapy, surgery rates, and pre-operative radiotherapy). We then conducted a visual inspection of the bar charts to identify any trends in mEFS and mOS based on the type of treatments; no statistical analysis was conducted.
2.2. Meta-analysis
Studies in which the entire population had epidermal growth factor receptor (EGFR) mutations and had received tyrosine kinase inhibitors were excluded from the meta-analysis. This was based on the assumption that the relationship between EFS and OS may differ between studies with an exclusively EGFR mutation-positive population and studies with a mixed or wild-type EGFR population. For example, a meta-analysis found that the strength of the association between PFS and OS in patients with NSCLC was lower in those who had received EGFR inhibitors and had EGFR mutations than those who had received treatments with other mechanisms of action and patient populations not selected based on EGFR mutations [Citation18].
2.3. Primary analysis of treatment effects
Following published frameworks on surrogacy in healthcare decision-making [Citation19,Citation20], the primary analysis evaluated the study-level association between the treatment effect on EFS and OS. The aim of this analysis was to determine whether a treatment effect on a surrogate (e.g. EFS) is predictive of a treatment effect on the outcome of interest (e.g. OS). In line with similar surrogacy analyses [Citation18], the statistical analysis comprised assessments of both the correlation and linear relationships between the treatment effects for EFS and OS. These effects were based on the HRs for treatments versus controls, which were log-transformed to remove the lower boundary of the HR at 0 and support the normality assumptions of the regression analyses, described below.
Correlation was evaluated using weighted Pearson’s correlation analysis and weighted Spearman’s rank order correlation coefficient. Weights were based on the inverse-variance of the HRs for OS, which were calculated using the HR 95% confidence intervals (CIs) and assuming normality. Greater weights were assigned to studies reporting narrower CIs, with CI width depending on both the OS maturity and study sample size. Bootstrapping was used to determine the 95% CIs for the correlation coefficient. The correlation analyses were performed using the wCorr package in R version 4.0.2.
Following previous studies [Citation21], the linear relationship between the log HRs for EFS and OS was estimated using a random-effects meta-regression (REMR) model. In the REMR, the log HRs for OS were analyzed using a random-effects model that allowed for heterogeneity in the treatment effect across studies. The log HRs for EFS were treated as linear fixed moderators of the log HR for OS. The resulting linear regression estimated the log HR for OS as an intercept term plus a variable multiplied by the log HR of EFS. The REMR analysis was performed using the metafor package in R version 4.02. The strength of the association between the log HRs for EFS and OS were assessed using the coefficient of determination from the REMR (R2), which corresponds to the amount of variation (or heterogeneity) in OS treatment effect that is explained by the surrogate (i.e. the EFS treatment effect). According to the reporting of surrogate endpoint evaluation using meta-analyses (ReSEEM) guidelines [Citation22] and Conforti et al. [Citation23], an R2 of > 0.7 was assumed to indicate a strong association, 0.5–0.69 a moderate association and < 0.5 a weak association.
2.4. Secondary analysis of mEFS and mOS
The secondary analysis evaluated the association between mEFS and mOS, to support the primary analysis. Correlation was evaluated using the same methods as used for the primary analysis (weighted Pearson’s correlation coefficient and weighted Spearman’s rank order correlation coefficient, using bootstrapping to determine the 95% CIs). Weights were based on the sample size in each study arm because not all studies reported variance measures for mOS. Associations between mEFS and mOS were evaluated using weighted linear regression using the total sample size of each study as weights.
3. Results
3.1. SLR results
The SLR identified 74 sources that met the inclusion criteria, of which 18 reported RCTs, 26 reported single-arm studies, and 30 reported observational studies (). No sources identified by the SLR evaluated whether there was an association between EFS and OS. The majority of studies were conducted in the USA or Europe, with other studies conducted in Asia (China, Japan, South Korea, and Taiwan) or the Middle East (Israel) or internationally. The characteristics of the included sources can be found in Supplementary Table S2. The quality assessment using the RoB2 and ROBINS-I tools generally found there to be a low risk of bias and the full results can be found in the Supplementary materials. The rationale for excluding studies at full text review can also be found in the Supplementary materials.
Figure 1. PRISMA diagram summarizing the flow of literature.
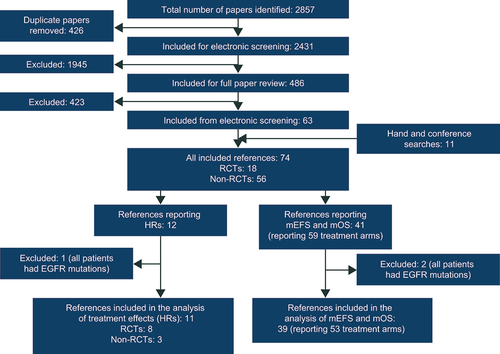
In total, 41 sources (including 59 treatment arms) reported mEFS and mOS following neoadjuvant therapy, and these sources all evaluated EFS and OS from diagnosis, enrollment, randomization, or initiation of neoadjuvant therapy. The majority of treatment arms (40 out of 55) evaluating mEFS and mOS following neoadjuvant therapy reported the use of adjuvant therapy, and these treatment arms appeared to have a longer mEFS and mOS than those that did not report the use of adjuvant therapy (). Out of the 12 treatment arms with the longest mEFS and mOS, 11 reported the use of adjuvant therapy in addition to neoadjuvant therapy. In addition, surgery rates were generally high, with most treatment arms (40 out of 55) reporting that ≥ 80% of patients underwent surgery. Treatment arms in which ≥ 80% of patients had undergone surgery appeared to have a longer mEFS and mOS than those in which < 80% of patients had undergone surgery (), and six out of the seven treatment arms with the longest mEFS and mOS had surgery rates ≥ 80%. The majority of treatment arms (41 out of 59) reporting mEFS and mOS did not receive radiotherapy as part of neoadjuvant therapy, and the distributions of mEFS and mOS were similar between treatment arms that included neoadjuvant radiotherapy and those that did not (). For example, the treatment arm with the longest mEFS received CRT [Citation24] while the treatment arm with the longest mOS received chemotherapy only [Citation25].
Figure 2. (a) mEFS and mOS in patients who received both neoadjuvant and adjuvant therapy and patients who received neoadjuvant therapy only. (b) mEFS and mOS in treatment arms in which ≥ 80% or < 80% of patients underwent surgery. (c) mEFS and mOS in treatment arms in which patients received chemoradiotherapy or chemotherapy only.
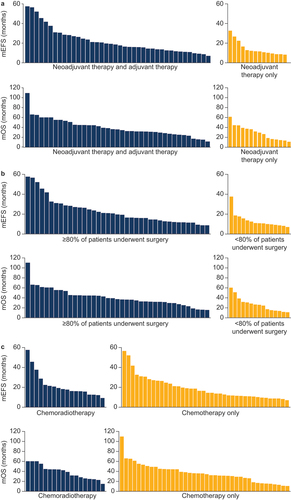
pCR, defined as no residual viable tumor cells in the resected primary tumor, was often reported alongside EFS and OS data (22 sources reporting 25 treatment arms), and a large range in pCR was observed across studies (0%–55%). Higher pCR rates were reported in treatment arms that had received CRT, with the 11 treatment arms reporting the highest pCR (>12%) all receiving CRT as part of neoadjuvant therapy. pCR ranged from 8% to 55% in patients who received CRT (13 treatment arms), and from 0% to 12% in those who received chemotherapy only (12 treatment arms).
3.2. Meta-analysis results
In total, one study was excluded from the primary analysis and two studies from the secondary analysis because the entire population had EGFR mutations (). No studies included in either analysis reported the use of immunotherapy.
3.3. Primary analysis of treatment effects
Eight RCTs [Citation26–33] reported HRs for EFS and OS, and were included in the primary analysis of treatment effects (). Seven out of the eight RCTs generated the HRs using Cox proportional hazard models [Citation26–32], and the remaining RCT did not report the method used [Citation33]. Across these studies, there was a positive linear correlation, according to the weighted Pearson’s correlation coefficient (r = 0.864, 95% CI: 0.809–0.992; p = 0.006) and the Spearman’s rank correlation coefficient (ρ = 0.977, 95% CI: 0.699–1.000; p < 0.0001). There was also a strong association between the HRs for EFS and OS, according to the random-effects meta-regression (R2 = 0.777; and Supplementary Fig. S1A for the graph plotted on a logarithmic scale).
Figure 3. Linear relationship between HRs for EFS and OS in (a) RCTs (8 studies) and (b) RCTs and observational studies (11 studies).
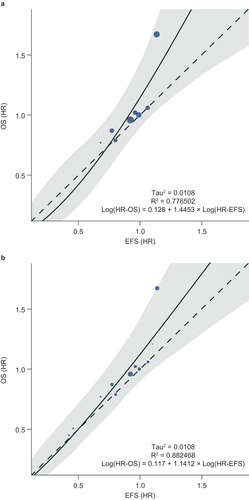
Table 1. Overview of sources included in the primary analysis of treatment effects.
The SLR also identified three observational studies with HRs for EFS and OS [Citation25,Citation34,Citation35]. All three studies generated the HRs using Cox proportional hazard models [Citation25,Citation34,Citation35] and two included propensity score matching to adjust for potential imbalances in the baseline characteristics between treatment groups [Citation25,Citation34]. When the three observational studies were included alongside the RCTs in a sensitivity analysis, the results were consistent with those from the primary analysis, and showed a linear correlation and a strong association between HRs for EFS and OS (weighted r = 0.888, 95% CI: 0.841–0.994; p = 0.0003; ρ = 0.981, 95% CI: 0.710–1.000; p < 0.0001; R2 = 0.882; and Supplementary Fig. S1B for the graph plotted on a logarithmic scale).
3.4. Secondary analysis of mEFS and mOS
In the secondary analysis of mEFS and mOS, 39 sources reporting 53 treatment arms were included (). There was a positive linear correlation according to weighted Pearson’s correlation coefficient (r = 0.819, 95% CI: 0.728–0.922; P < 0.0001) and Spearman’s rank correlation coefficient (ρ = 0.894, 95% CI: 0.746–0.955; p < 0.0001). There was also a moderate association between mEFS and mOS according to weighted linear regression (R2 = 0.664).
4. Discussion
We found a strong association between EFS and OS in the primary analysis of treatment effects, which evaluated the relationship between changes in the surrogate outcome (i.e. EFS) and the outcome of interest (i.e. OS). The R2 values (RCTs only, R2 = 0.777; RCTs and observational studies, R2 = 0.882) were above the threshold of 0.7 that indicates a strong association and surrogacy, as recommended by the ReSEEM guidelines [Citation22] and Conforti et al. [Citation23]. This suggests that improvements in EFS are likely to be predictive of improvements in OS. A moderate association was also found between mEFS and mOS in the secondary analysis, which included a larger number of studies than the primary analysis.
Following disease recurrence after neoadjuvant therapy and surgery, patients may receive subsequent therapies, including treatment for more advanced stages of disease. Although these will likely impact OS, they are less likely to impact EFS. Differences in OS between treatment arms, and to a much lesser extent differences in EFS, may therefore be influenced by the type of subsequent therapy received. The studies included in the primary analysis reported limited information on subsequent therapies, and so we were unable to investigate their impact on the relationship between OS and EFS. Notably, all RCTs included in the primary analysis were published before 2015 and prior to the approval of the first immunotherapy (a programmed cell death-1/programmed cell death-ligand 1 inhibitor) for metastatic NSCLC in the USA [Citation36]. For patients with NSCLC without actionable driver mutations, immunotherapy now represents standard of care for subsequent lines of therapy and therefore the impact of immunotherapy in these subsequent therapy lines on OS, and therefore the relationship between EFS and OS, is unlikely to be captured by our analyses. EFS is typically designed to minimize confounding by subsequent therapies, making it a useful endpoint for assessing the efficacy of neoadjuvant therapies in r-NSCLC. Moreover, the avoidance or delay of disease progression or recurrence in this setting is a clinically relevant outcome that is independent of the potential use of EFS as a surrogate for OS.
One of the limitations of our analysis was that only a small number of RCTs (n = 8) reported HRs for EFS and OS, which affects the robustness of the results. Our analysis was also based on published literature, meaning that it may therefore have been subject to publication bias. In addition, no studies reporting mEFS and mOS in patients receiving neoadjuvant immunotherapy were identified in the SLR, and therefore immunotherapy could not be investigated in either the primary or secondary analyses. Several trials are currently evaluating immunotherapies as neoadjuvant therapy in r-NSCLC; however, no published data from these studies on both EFS and OS were available at the time of the SLR, with the results of the CheckMate 816 and NADIM II trials only published after the searches were conducted [Citation37,Citation38]. Further research is therefore needed to evaluate the strength of the association between EFS and OS in patients receiving immunotherapy. Finally, as described in the methods section, sources in which the entire population had EGFR mutations were excluded from the analyses, and therefore the association between EFS and OS could not be evaluated in these populations.
This study was designed to evaluate any association between EFS and OS, but we also summarized mEFS and mOS using bar charts and conducted a visual inspection to look at various factors relating to treatment, such as adjuvant therapy, surgery rates, and radiotherapy. No statistical analysis was conducted and therefore these results should be interpreted with caution due to the differences between the included studies, including the design, specific treatments, and timepoint from which EFS and OS were measured, which may have impacted EFS and OS. For example, treatment arms that reported the use of adjuvant therapy appeared to have longer mEFS and mOS than those that did not. However, it is worth noting that the proportion of patients receiving adjuvant therapy varied between treatment arms and that this may affect the conclusions that can be drawn. Another factor that seemed to affect mEFS and mOS was the proportion of patients who underwent surgery, with high surgery rates (≥80% undergoing surgery) appearing to improve mEFS and mOS.
In addition, we also looked at the number of studies reporting pCR. Although our SLR was not specifically designed to identify all studies reporting pCR, 22 (30%) studies included did report pCR rates; and these varied widely between studies. pCR rates were often higher than have been reported in more recent trials evaluating newer therapies, such as immunotherapy in the CheckMate 816 and NADIM II trials (pCR of 24.0% and 37.5%, respectively, in the immunotherapy arms) [Citation37,Citation38]. In addition to differences in neoadjuvant treatment (e.g. the use of CRT), this may be because recent trials use a more stringent definition of pCR, which includes no residual viable cells in the lymph nodes in addition to the tumor following resection, than was typically used in older studies, which predominantly looked only at residual viable cells in the tumor. The association between pCR and OS has been evaluated previously in a meta-analysis that found a consistent and strong association between pCR and survival when assessed within studies [Citation39]. Emerging data suggest that pCR may also be associated with EFS in patients receiving neoadjuvant immunotherapy [Citation37,Citation38].
5. Conclusions
We found a strong association between EFS and OS in the analysis of treatment effects, which evaluated the relationship between changes in the surrogate outcome (i.e. EFS) and changes in the outcome of interest (i.e. OS). This indicates that improvements in EFS are likely to be predictive of improvements in OS. EFS may therefore be a reliable surrogate for OS following neoadjuvant therapy in r-NSCLC.
Declaration of interest
RH, MB, and PM are employees of AstraZeneca UK. NG, PC, and IDP are employees of AstraZeneca, USA. AMC, CE, and PF are employees of, and PF holds shares in Oxford PharmaGenesis, Oxford, UK.
The authors have no other relevant affiliations or financial involvement with any organization or entity with a financial interest in or financial conflict with the subject matter or materials discussed in the manuscript apart from those disclosed.
Reviewer disclosures
Peer reviewers on this manuscript have no relevant financial or other relationships to disclose.
Author contribution statement
Gyula Ostoros: Methodology, Writing- Reviewing and Editing
Robert Hettle: Conceptualization, Methodology, Data analysis, Writing- Reviewing and Editing
Nefeli Georgoulia: Conceptualization, Methodology, Writing- Reviewing and Editing
Mehmet Berktas: Conceptualization, Methodology, Writing- Reviewing and Editing
Pratibha Chander: Conceptualization, Methodology, Writing- Reviewing and Editing
Ignacio Diaz Perez: Conceptualization, Methodology, Writing- Reviewing and Editing
Anne-Marie Couto: Methodology, Data collection, Data analysis, Writing- Reviewing and Editing
Christian Eichinger: Methodology, Data collection, Data analysis, Writing- Reviewing and Editing
Polly Field: Methodology, Data collection, Data analysis, Writing- Reviewing and Editing
Peter Morten: Conceptualization, Methodology, Data analysis, Writing- Reviewing and Editing
Supplemental Material
Download MS Word (314.2 KB)Acknowledgments
The authors thank Helen Fishpool at Oxford PharmaGenesis, Oxford, UK, for medical writing support (funded by AstraZeneca, Cambridge, UK).
Supplementary material
Supplemental data for this article can be accessed online at https://doi.org/10.1080/14737140.2023.2272645
Additional information
Funding
References
- Sung H, Ferlay J, Siegel RL, et al. Global cancer statistics 2020: GLOBOCAN estimates of incidence and mortality worldwide for 36 cancers in 185 countries. CA Cancer J Clin. 2021 May;71(3):209–249. doi: 10.3322/caac.21660
- Cancer.Net. Lung cancer - non-small cell: statistics. 2022 [Accessed 2023 Nov 16]. Available at: https://www.cancer.net/cancer-types/lung-cancer-non-small-cell/statistics.
- Daly ME, Singh N, Ismaila N, et al. Management of stage III non-small-cell lung cancer: ASCO guideline. J Clin Oncol. [2022 Apr 20];40(12):1356–1384. doi: 10.1200/JCO.21.02528
- Postmus PE, Kerr KM, Oudkerk M, et al. Early and locally advanced non-small-cell lung cancer (NSCLC): ESMO clinical practice guidelines for diagnosis, treatment and follow-up. Ann Oncol. [2017 Jul 1];28(suppl_4):iv1–iv21. doi: 10.1093/annonc/mdx222
- National Comprehensive Cancer Network. NCCN guidelines version 5.2022. Non-small cell lung cancer. [Accessed 2022 Nov 16]. Available at: https://www.nccn.org/professionals/physician_gls/pdf/nscl.pdf.
- Clinicaltrials.gov. A study of neoadjuvant/adjuvant durvalumab for the treatment of patients with resectable non-small cell lung cancer (AEGEAN). [Accessed 2022 Jul 5]. Available at: https://www.clinicaltrials.gov/ct2/show/NCT03800134.
- Clinicaltrials.gov. A neoadjuvant study of nivolumab plus ipilimumab or nivolumab plus chemotherapy versus chemotherapy alone in early stage non-small cell lung cancer (NSCLC) (CheckMate 816). [Accessed 2022 Jul 5]. Available at: https://clinicaltrials.gov/ct2/show/NCT02998528.
- Clinicaltrials.gov. A study of neoadjuvant chemotherapy plus nivolumab versus neoadjuvant chemotherapy plus placebo, followed by surgical removal and adjuvant treatment with nivolumab or placebo for participants with surgically removable early stage non-small cell lung cancer. [Accessed 2022 Jul 7]. Available at: https://www.clinicaltrials.gov/ct2/show/NCT04025879.
- Clinicaltrials.gov. A study of neoadjuvant atezolizumab plus chemotherapy versus placebo plus chemotherapy in patients with resectable stage II, IIIA, or select IIIB non-small cell lung cancer (IMpower030). [Accessed 2022 Jul 7]. Available at: https://www.clinicaltrials.gov/ct2/show/NCT03456063.
- Clinicaltrials.gov. Efficacy and safety of pembrolizumab (MK-3475) with platinum doublet chemotherapy as neoadjuvant/adjuvant therapy for participants with resectable stage II, IIIA, and resectable IIIB (T3-4N2) non-small cell lung cancer (MK-3475-671/KEYNOTE-671). [Accessed 2022 Jul 7]. Available at: https://www.clinicaltrials.gov/ct2/show/NCT03425643.
- FDA. FDA approves neoadjuvant nivolumab and platinum-doublet chemotherapy for early-stage non-small cell lung cancer. [Accessed 2022 Aug 23]. Available at: https://www.fda.gov/drugs/resources-information-approved-drugs/fda-approves-neoadjuvant-nivolumab-and-platinum-doublet-chemotherapy-early-stage-non-small-cell-lung.
- Lux MP, Ciani O, Dunlop WCN, et al. The impasse on overall survival in oncology reimbursement decision-making: how can we resolve this? Cancer Manag Res. 2021;13:8457–8471. doi: 10.2147/CMAR.S328058
- FDA. Clinical trial endpoints for the approval of cancer drugs and biologics. Guidance For Industry. 2018 [Accessed 2022 Jul 26]. Available at: https://www.fda.gov/media/71195/download.
- National Institute for Health and Care Excellence. Osimertinib for adjuvant treatment of EGFR mutation-positive non-small-cell lung cancer after complete tumour resection. [Accessed 2022 Aug 23]. Available at: https://www.nice.org.uk/guidance/ta761/resources/osimertinib-for-adjuvant-treatment-of-egfr-mutationpositive-nonsmallcell-lung-cancer-after-complete-tumour-resection-pdf-82611430864837.
- National Institute for Health and Care Excellence. Atezolizumab for adjuvant treatment of resected non-small-cell lung cancer. [Accessed 2022 Sep 29]. Available at: https://www.nice.org.uk/guidance/ta823.
- Risk of bias info. [Accessed 2022 Nov 28]. Available at: https://www.riskofbias.info/welcome.
- Cochrane Methods. Risk of bias in non-randomized studies - of interventions (ROBINS-I). [Accessed 2022 Nov 28]. Available at: https://methods.cochrane.org/bias/risk-bias-non-randomized-studies-interventions.
- Shameer K, Zhang Y, Jackson D, et al. Correlation between early endpoints and overall survival in non-small-cell lung cancer: a trial-level meta-analysis. Front Oncol. 2021;11:672916. doi: 10.3389/fonc.2021.672916
- Ciani O, Buyse M, Drummond M, et al. Time to review the role of surrogate end points in health policy: state of the art and the way forward. Value Health. 2017 Mar;20(3):487–495. doi: 10.1016/j.jval.2016.10.011
- Taylor RS, Elston J. The use of surrogate outcomes in model-based cost-effectiveness analyses: a survey of UK Health Technology assessment reports. Health Technol Assess. 2009;13(8):8. doi: 10.3310/hta13080
- Schürmann C, Sieben W. Differences in surrogate threshold effect estimates between original and simplified correlation-based validation approaches. Stat Med. 2016;35(7):1049–1062. doi: 10.1002/sim.6778
- Xie W, Halabi S, Tierney JF, et al. A systematic review and recommendation for reporting of surrogate endpoint evaluation using meta-analyses. JNCI Cancer Spectr. 2019 Mar;3(1):kz002. doi: 10.1093/jncics/pkz002
- Conforti F, Pala L, Sala I, et al. Evaluation of pathological complete response as surrogate endpoint in neoadjuvant randomised clinical trials of early stage breast cancer: systematic review and meta-analysis. BMJ. [2021 Dec 21];375:e066381. doi: 10.1136/bmj-2021-066381
- Albain KS, Swann RS, Rusch VW, et al. Radiotherapy plus chemotherapy with or without surgical resection for stage III non-small-cell lung cancer: a phase III randomised controlled trial [clinical trial, phase III comparative study randomized controlled trial research support, N.I.H., extramural research support, non-U.S. Gov’t]. Lancet. [2009 Aug 1];374(9687):379–386. doi: 10.1016/S0140-6736(09)60737-6
- Chen Z, Luo Q, Hong J, et al. Long-term results of a randomized controlled trial evaluating preoperative chemotherapy in resectable non-small cell lung cancer. Onco Targets Ther. 2013;6:645–650. doi: 10.2147/OTT.S44503
- Katakami N, Tada H, Mitsudomi T, et al. A phase 3 study of induction treatment with concurrent chemoradiotherapy versus chemotherapy before surgery in patients with pathologically confirmed N2 stage IIIA nonsmall cell lung cancer (WJTOG9903). Cancer. [2012 Dec 15];118(24):6126–6135. doi: 10.1002/cncr.26689
- Thomas M, Rube C, Hoffknecht P, et al. Effect of preoperative chemoradiation in addition to preoperative chemotherapy: a randomised trial in stage III non-small-cell lung cancer. Lancet Oncol. 2008 Jul;9(7):636–648. doi: 10.1016/S1470-2045(08)70156-6
- van Meerbeeck JP, Kramer GWPM, Van Schil PEY, et al. Randomized controlled trial of resection versus radiotherapy after induction chemotherapy in stage IIIA-N2 non-small-cell lung cancer. J Natl Cancer Inst. [2007 21 Mar];99(6):442–450. doi: 10.1093/jnci/djk093
- Felip E, Rosell R, Maestre JA, et al. Preoperative chemotherapy plus surgery versus surgery plus adjuvant chemotherapy versus surgery alone in early-stage non-small-cell lung cancer. J Clin Oncol. [2010 1 Jul];28(19):3138–3145. doi: 10.1200/JCO.2009.27.6204
- Pisters KM, Vallieres E, Crowley JJ, et al. Surgery with or without preoperative paclitaxel and carboplatin in early-stage non-small-cell lung cancer: Southwest Oncology group trial S9900, an intergroup, randomized, phase III trial [clinical trial, phase III multicenter study randomized controlled trial research support, N.I.H., extramural research support, non-U.S. Gov’t]. J Clin Oncol. [2010 Apr 10];28(11):1843–1849. doi: 10.1200/JCO.2009.26.1685
- Gilligan D, Nicolson M, Smith I, et al. Preoperative chemotherapy in patients with resectable non-small cell lung cancer: results of the MRC LU22/NVALT 2/EORTC 08012 multicentre randomised trial and update of systematic review. Lancet. [2007 Jun 9];369(9577):1929–1937. doi: 10.1016/S0140-6736(07)60714-4
- Brandt WS, Yan W, Zhou J, et al. Outcomes after neoadjuvant or adjuvant chemotherapy for cT2-4N0-1 non-small cell lung cancer: a propensity-matched analysis. J Thorac Cardiovasc Surg. 2019 Feb;157(2):743–753.e3. doi: 10.1016/j.jtcvs.2018.09.098
- Counago F, Rodriguez de Dios N, Montemuino S, et al. Neoadjuvant treatment followed by surgery versus definitive chemoradiation in stage IIIA-N2 non-small-cell lung cancer: a multi-institutional study by the oncologic group for the study of lung cancer (Spanish radiation Oncology Society). Lung Cancer. 2018 Apr;118:119–127.
- Vyfhuis MAL, Burrows WM, Bhooshan N, et al. Implications of pathologic complete response beyond mediastinal nodal clearance with high-dose neoadjuvant chemoradiation therapy in locally advanced, non-small cell lung cancer. Int J Radiat Oncol Biol Phys. [2018 1 Jun];101(2):445–452. doi: 10.1016/j.ijrobp.2018.02.003
- Shields MD, Marin-Acevedo JA, Pellini B. Immunotherapy for advanced non–small cell lung cancer: a decade of progress. Am Soc Clin Oncol Educ Book. 2021 Mar;41(41):e105–e127. doi: 10.1200/EDBK_321483
- Forde PM, Spicer J, Lu S, et al. Neoadjuvant nivolumab plus chemotherapy in resectable lung cancer. N Engl J Med. [2022 May 26];386(21):1973–1985. doi: 10.1056/NEJMoa2202170
- Provencio M, Serna R, Nadal E, et al. Nivolumab + chemotherapy vs chemotherapy as neoadjuvant treatment for resectable IIIA-B NSCLC. Progression-free survival and overall survival results from the phase 2 NADIM II trial [oral presentation]. Presented at the World Conference on Lung Cancer; 2022 Aug 6–9; Vienna, Austria.
- Waser N, Adam A, Schweikert B, et al. Pathologic response as early endpoint for survival following neo-adjuvant therapy in resectable non-small cell lung cancer: systematic literature review and meta-analysis. Presented At The European Society For Medical Oncology Virtual Congress. [19–21 Sep 2020];31:S806. doi: 10.1016/j.annonc.2020.08.116
- Spineli LM, Pandis N. Prediction interval in random-effects meta-analysis. Am J Orthod Dentofacial Orthop. 2020 Apr;157(4):586–588. doi: 10.1016/j.ajodo.2019.12.011