ABSTRACT
Introduction
Cervical cancer affects half a million women worldwide annually. Given the association between high-risk human papillomavirus (hrHPV) infection and carcinogenesis, hrHPV DNA testing became an essential diagnostic tool. However, hrHPV alone does not cause the disease, and, most importantly, many cervical lesions regress to normal in a year because of the host immune system. Hence, the low specificity of hrHPV DNA tests and their inability to predict the outcome of infections have triggered a further search for biomarkers.
Areas covered
We evaluated the latest viral and cellular biomarkers validated for clinical use as primary screening or triage for cervical cancer and assessed their promise for prevention as well as potential use in the future. The literature search focused on effective biomarkers for different stages of the disease, aiming to determine their significance in predicting the outcome of hrHPV infections.
Expert opinion
Biomarkers such as p16/Ki-67, hrHPV genotyping, hrHPV transcriptional status, and methylation patterns have demonstrated promising results. Their eventual implementation in the screening programs may support the prompt diagnosis of hrHPV infection and its progression to cancer. These biomarkers will help in making clinical management decisions on time, thus, saving the lives of hrHPV-infected women, particularly in developing countries.
1. Introduction
Cervical cancer is the third most frequent cancer in women worldwide after breast cancer or colon cancer. Even though the application of screening programs has lowered the incidence and mortality, cervical cancer remains one of the most malignant diseases among women. Over 62% of the deaths related to cervical cancer occur in low and middle-income countries, where access to screening programs and treatment is limited [Citation1].
Human papillomavirus (HPV) is an ancient, non-enveloped double-stranded DNA virus from the family Papillomaviridae that causes the most common sexually transmitted infection with more than 14 million new infections annually. Over 80% of women become infected at some stage in their life. HPV family is divided into five genera based on their DNA sequences, life-cycle characteristics and disease associations. The Beta and Gamma genera are associated with asymptomatic infections causing cutaneous lesions. The genus Alphapapillomaviruses, causing mucosal and cutaneous infections, has an oncogenic role in the progression of HPV infection to cervical cancer. Within this genus, genotypes 16 and 18 are the major carcinogenic types, causing approximately 70% of all cervical cancers. Additionally, the World Health Organization (WHO) also includes genotypes 31, 33, 35, 39, 45, 51, 52, 56, 58, 59, 66, 68 and 73 to the high-risk HPV (hrHPV) grouThe remaining genotypes of the alpha genus, classified as low risk, are rarely associated with neoplasia causing mainly asymptomatic infections or benign papillomas, which are resolved by the host immune system [Citation2–4]. Persistent infection is the etiological agent for the majority of all cervical cancers due to the ability of hrHPV to persist and drive cell proliferation in the cervical transformation zone [Citation2]. Squamous cell carcinoma (SCC) is the most common type of cancer caused by hrHPV infections, while in rare cases, the infection progress to adenocarcinomas (ADC). The latter comprises approximately 10–20% of all cervical cancers, with 60–100% of them being related to hrHPV [Citation5].
The infectious HPV life cycle is linked to the differentiation process of the host squamous stratified cervical epithelium . The life cycle begins with the viral entrance through a microwound in the cervical epithelium. HPV capsid proteins, L1 and L2, get attached to the basement membrane, and after two to four hours, get internalized, allowing the infection of basal epithelial cells. Viral genome is established as an extrachromosomal circular episome with access to the cellular transcription and replication machinery. Viral DNA goes through a restricted number of replication cycles maintaining a latent infection with a low episome copy number per nucleus (around 10–200 copies). Infected basal cells undergo natural keratinocyte terminal differentiation in the transformation zone, carrying the viral genome with them. During this process, the viral genome is going to be expressed gradually. E1 and E2 genes are expressed to maintain the viral DNA as an episome and to facilitate the correct segregation of genomes during cell division [Citation6]. These proteins also allow the initial amplification of the virus and viral genome partitioning during basal cell division. In uninfected epitheliums, basal cells end the cell cycle and migrate to the suprabasal cell layer to undergo terminal differentiation. Upon HPV infection, this process is retarded because the expression of the oncoproteins E6 and E7 at low levels causes cell proliferation. E7 interferes with pRb, preventing the cell from entering S-phase, and E6 inhibits p53, causing inactivation of apoptosis. For a productive infection, HPV virions must amplify their viral genomes to package them into infectious particles. In the mid-layers, the expression of E4, with the contribution of E1, E2, and E5 viral proteins, facilitate viral genome amplification. The presence and accumulation of viral E4 proteins coincide with the formation of koilocytotic cells (cells with an enlarged and irregular nucleus) [Citation7]. Koilocytosis could contribute to viral dissemination in the upper layers of the epithelia due to keratinocyte fragility. In the upper epithelial layer, the E4 positive cells leave the cell cycle and express the two structural proteins, L1 and L2. These particles allow viral genome assembly, packaging, and releasing of new infective virions [Citation6,Citation8–11].
Figure 1. Life cycle of high-risk Human Papillomavirus (hrHPV) in cervical epithelia and the progression from infection to cervical cancer. 1A) First, hrHPV enters through a microwound to the basal membrane of the cervical epithelia, where the infected cells are maintained as a reservoir. The expression of E1 and E2 proteins drives the initial viral amplification in the lower layers of the epithelia. When these cells divide, the expression of E6 and E7 proteins stimulates cell proliferation (orange cells with a light orange nucleus) to the mid-layers of the epithelia. In the mid-layers, the expression of E4 with the help of E1, E2, and E5 facilitates higher viral DNA amplification (orange cells with an enlarged and irregular nucleus, known as koilocytotic cells). Finally, the cells leave the cell cycle (E4+) and express L1 and L2 proteins, allowing the packaging of the amplified virus (orange cells with a dark orange nucleus) and the subsequent release. Gene expression of viral proteins (right arrow), viral DNA amplification, and cell proliferation (left arrows) start from low levels (light orange) at the basal layers and go to high levels (dark orange) at the mid or upper layers of the cervical epithelia in hrHPV-infected women. 1B) After an hrHPV infection, the virus induces cell proliferation, and the infected tissue can progress to CIN. The first lesions, low-grade intraepithelial lesions (LSIL, CIN1), might regress after one year. However, they can also progress to high-grade intraepithelial lesions (HSIL, CIN2 – CIN3) and then to cervical cancer. CIN: cervical intraepithelial neoplasia
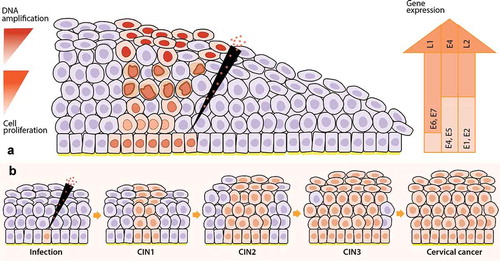
The expression of all HPV genes (including oncogenic E6 and E7) at low levels has a subclinical consequence. Most of such transient infections will clear in non-immunocompromised patients within a year due to the host immune system [Citation12,Citation13]. Progression is closely related to HPV persistence. Persistent infections are associated with a higher risk of HPV DNA integration into the host genome. HPV16 has longer persistence than other high-risk types [Citation14]. Although in high-grade lesions, HPV is frequently integrated in the host cell genome, not all HPV genome integrations lead to cancer [Citation15]. Integration is not a normal process in the HPV life cycle as it is characterized by the deletion of essential viral genes for the synthesis of infecting virions. Therefore, it is disadvantageous for the virus. Upon integration, the circular HPV genome is linearized, disrupting the E2 gene and resulting in its inactivation. Its function was to repress the viral E6 and E7 oncogenes promoter, but E2 inactivation will lead to increased expression and stability of transcripts encoding the E6 and E7 proteins. These oncoproteins mediate degradation of the tumor suppressor genes p53 and pRb [Citation16–19]. Thus, detecting the transcriptionally active HPV is valuable, as well as the analysis of upregulated viral genes, and the consequent cellular implications that result in neoplastic changes.
Three prophylactic vaccines against HPV infection (Gardasil, Gardasil 9, and Cervarix) have been approved by the U.S Food and Drug Administration (FDA). They consist of noninfectious virus-like particles made up of the major HPV capsid protein L1. Gardasil is a quadrivalent vaccine against HPV types 6, 11, 16, and 18, Gardasil 9 is a 9-valent vaccine costing of the same types that Gardasil with additional inclusion of genotypes 31, 33, 45, 52, and 58. Cervarix is a bivalent vaccine against HPV16 and 18. These prophylactic vaccines are administered in three doses to females age 9 to 26 years old in an attempt to prevent infection. Furthermore, HPV vaccines are not intended for the treatment of infections or disease, as they are not effective in treating pre-existing HPV infections. They were found to provide nearly 100% protection against cervical infections with HPV genotypes 16 and 18 and high efficacy in preventing precancerous cervical lesions caused by these genotypes. However, vaccinated women should continue being screened with Papanicolau (PAP) test because no significate reduction has been observed for the borderline/ASCUS and low-grade dyskaryosis/LSIL after vaccination [Citation20–23].
Papanicolau test, which uses cytologic smears, is one of the most effective methods used for screening of cervical cancer since the 1950s. Lesions found by this technique can be graded in different cellular abnormality levels (PAP1, PAP2, PAP3a1, PAP3a2, PAP3b, PAP4, PAP5), (Supplementary Figure 1), in which the increased grading number positively correlates with the number of dysplastic cells observed in the smear. The Bethesda system for cervical cytology has created a widely used terminology, where cervical cancer precursors are classified as low-grade squamous intraepithelial lesions (LSIL) or high-grade squamous intraepithelial lesions (HSIL). An LSIL diagnosis is based on the identification of abnormal squamous cells with the size of a normal superficial or intermediate cell. The diagnose of an HSIL relies on the presence of abnormal squamous cells smaller than those present in low-grade lesions. A normal PAP test (PAP1) is a negative result for intraepithelial lesion or malignancy (NILM). Moreover, detection of atypical squamous cells (ASC), which include cells of undetermined significance (ASCUS) and with a higher risk of being precancerous (ASCUS+), results from morphologic variability of squamous cells in different physiological and pathological states [Citation22,Citation24].
Although squamous lesions are easily detected by cytology, which is designed to detect cells on the surface of the cervix, glandular lesions are not detected by this method. Such lesions are visible in histological examination via biopsy. The epithelial alterations that constitute cervical intraepithelial neoplasia (CIN) have been semiquantitatively divided into three categories: CIN1 to CIN3 ( and Supplementary Figure 1). CIN1 lesions (or low-grade CIN) are flat warts that retain the ability to complete the HPV life cycle and produce virus particles. In this case, proliferation is at a low level, occupying the lower third of the epithelium. CIN2 lesions are characterized by basaloid cells filling the lower one to two-thirds of the epithelium. Lastly, CIN3 lesions hold two-thirds of the thickness of the epithelium, which makes it a preliminary stage of cervical cancer. Both CIN2 and CIN3 are considered high-grade CIN since they are at risk for cervical cancer development [Citation18,Citation19,Citation24].
More than half of new cases of cervical cancer occur among women who are not attending the screening program or in women above the screening age. This shows the need to find alternative ways to improve attendance in the screening programs. HPV self-sampling is a strategy that may help in increasing cervical cancer screening and define a global direction for many national screening programs. For example, in The Netherlands, women that do not wish to have a cervical sample taken at their general practitioner (GP) can request a self-sampling kit [Citation25,Citation26]. The application of this technique has demonstrated to increase the participation of hard-to-reach or ‘non-responders’ women in the screening programs [Citation26,Citation27]. The convenience, privacy, ease-of-use, and cost-effectiveness are valuable benefits of self-sampling kits. Several studies have compared the sensitivity of self-sampling to physician-collection samples and concluded that both have similar results. Nevertheless, there are still barriers such as confidence in self-sampling, cultural implications of sexual behavior, or trust within relationships. In addition, technical errors like incorrect sampling or storage can influence the sensitivity and negative predictive value of the test, leading to false-negative results [Citation27–30].
The cytology test has reduced the incidence of cervical cancer over the last 30 years. Still, it has limited capability to predict the presence of abnormalities in the cervix as well as progression from hrHPV infections to disease. Knowing that cervical cancer is still a prevalent disease, it is imperative to predict which patients have increased risk for cervical lesions. This has stimulated the search for new tools for screening and predicting cervical cancer. As comprehension of the infection increased, and more tests become available with time, the definition of better screening methods can lead to an eventual remodeling of the current diagnosis flowchart. hrHPV DNA analysis is one of the most relevant tests applied worldwide [Citation24,Citation31]. Subsequently, the Netherlands became the first country in the world to implement this method in the national hrHPV population-based screening program in 2017, making the full transition from cytology to primary hrHPV screening [Citation32]. In addition, Italy introduced HPV screening in 2014, which is within an ongoing national implementation along with other European countries such as Spain, and Finland [Citation33,Citation34]. Moreover, the performance of the HPV mRNA test as a primary screening was assessed within the framework of the Norwegian and Swedish population-based screening programs [Citation35–37]. The results were similar to the outcome of initial hrHPV DNA testing but demonstrating an advantage in decreasing cytology assessments. Based on these results, a positive recommendation was given to consider hrHPV mRNA analysis as a primary test for population-based screening of the disease.
In cervical cancer prevention, an ideal biomarker must detect the progression of hrHPV infection to disease and allow clinical management decisions in time. These decisions include further testing, treatment, referral to colposcopy, increased surveillance, or release to routine screening. Biomarkers can be implemented in a wide range of steps within the disease flowchart. Some of these markers seem promising in replacing primary screening, while others are more suitable for triage, or can be used alongside primary tests. Biomarkers can also be used to estimate the prognosis of cancer patients, determine the impact of novel treatments as well as being used to monitor treatment progression.
In general, we can categorize cervical cancer-related biomarkers into two broad groups: viral and cellular biomarkers [Citation38,Citation39]. This review aims to summarize characterized and well-described biomarkers for cervical cancer in hrHPV-infected women and demonstrate their importance in the disease flowchart. Here, we evaluate their advantages and disadvantages according to the analysis of recent research and literature. We also explore the application of biomarkers for specific stages of the disease and their combined use with self-collected samples.
2. The viral biomarkers
hrHPV infection itself is confirmed to be the essential etiological agent of invasive cervical cancer development. Therefore, a large amount of research is focused on biomarkers directly linked to the HPV life cycle and natural history of HPV-dependent cervical carcinogenesis, such as hrHPV DNA testing, genotyping, viral load, transcriptional status, viral DNA methylation, and expression of certain viral proteins relevant in the viral life cycle. In this part, we will discuss the most suitable viral related biomarkers and their potential use for cervical cancer risk assessment and prevention.
Figure 2. Viral biomarkers during HPV infection and progression to cervical cancer. After HPV infection at the basal membrane in the epithelia (virus detectable on DNA level), the virus will replicate its genome at low copy numbers, maintaining a latent infection in the basal keratinocytes. Next, the infection will switch to a productive infection where the virus expresses its genome, while keratinocytes differentiate, forming koilocytotic cells (E4+) and finally resulting in the release of the virus in the upper layers of the epithelia, enabling viral load assessment. After viral release, virions can re-infect the basal membrane leading to a persistent infection. Persistent infections present a higher risk of undergoing HPV genome integration to the host genome in the epithelia, resulting in cervical intraepithelial neoplasia (CIN) and the progression of these lesions cause cervical cancer. Nevertheless, the lesions can also regress thanks to the response of the host immune system that generates infection clearance. Approximately, in 70% of the cases, hrHPV16 and 18 genotypes are the causative agents of the progression to cervical cancer, which makes viral genotyping an essential biomarker. Besides, the progression of HPV infection to cervical cancer is highly related to the viral oncoproteins E4 and E6/E7; therefore, the analysis of their protein and transcriptional levels, respectively, is important as a biomarker testing for the disease. This infection progression is also associated with the HPV DNA methylation patterns that are higher in later stages of the lesions and cervical cancer
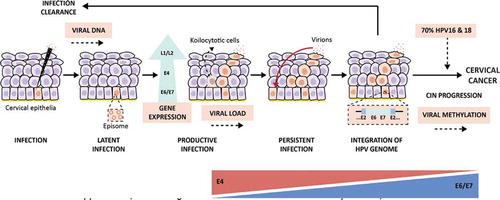
2.1. hrHPV DNA testing
After HPV infects host cells, viral DNA is maintained as an episome establishing a latent infection. A switch into a productive infection is associated with the assembling of infective virions. Persistent infection with hrHPV genotypes triggers the integration of viral DNA into the host genome. Next, the viral oncoproteins will be expressed, enhancing the progression of preinvasive lesions to cervical cancer. Therefore, a necessary condition for squamous intraepithelial lesions (SILs) and cervical cancer is a persistent infection of high-risk Human Papillomavirus [Citation40–42].
Due to this causal relationship, hrHPV DNA testing is nowadays one of the most wide-ranging biomarkers for primary screening of cervical cancer. This assay is considered a gold standard for the diagnosis of early HPV infection, allowing the detection of the 14 most carcinogenic hrHPV genotypes: 16, 18, 31, 33, 35, 39, 45, 51, 52, 56, 58, 59, 66, and 68 [Citation2,Citation3,Citation10]. The method has high sensitivity and a high negative predictive value, meaning that when no hrHPV DNA is detected, the risk of CIN3 lesions or cervical cancer is low. On the other hand, hrHPV DNA can also be detected in transient infections that may not persist, and therefore, do not result in cervical cancer.
One outcome of hrHPV infections is viral DNA integration into the host genome, which also demonstrates the utility of hrHPV DNA testing in screening. Hudelist et al. reported that viral integration could be associated with the grade of the lesions, while Wei et al. described similar findings and recommended to include the testing of hrHPV integration in hrHPV DNA-based screening programs [Citation42,Citation43]. Since hrHPV DNA integration is not required for cervical dysplasia, this viral process may not be a good predictor of disease. However, hrHPV DNA itself can be detected in both infection outcomes, showing its utility in disease progression .
The first application of hrHPV DNA testing was for triage of ASCUS cytology since the precancer risk of HPV-positive ASCUS is the same as LSIL [Citation40]. The ASCUS-LSIL Triage Study (ALTS) determined that repeated cytology after an ASCUS result referred more women to colposcopy in comparison with hrHPV DNA testing [Citation44]. The study also explained that this strategy required two visits to cytology to achieve similar sensitivity to viral DNA testing (95.4%). Therefore, hrHPV DNA testing represents a practical approach for managing women with initial ASCUS cytology [Citation44,Citation45]. Nowadays, there is a lot of evidence suggesting that this test offers a significant improvement over the more traditional Pap cytology screening due to its ability to detect more precancerous disease and higher reproducibility [Citation46]. Based on this scientific evidence and cost-effectiveness, hrHPV DNA testing has been proposed to replace cytology in primary screening programs [Citation32,Citation47]. In fact, in some countries, like Turkey, Italy, or The Netherlands, hrHPV DNA testing is the first-line initial screening program [Citation32–34,Citation48]. Women are free to choose between going to the general practitioner (GP), who will take a smear, or use a self-sampling kit that is sent by regular mail. Cytology is performed on hrHPV-positive GP-collected samples. Those positive women with cytological abnormalities are referred to colposcopy, while those with normal cytology will have to be followed-up [Citation32].
Regarding the performance of the hrHPV DNA testing on regular cytology versus self-sampling, it has been observed that hrHPV positivity was higher in clinician-collected samples than in self-collected samples (9.2% vs. 7.6%). However, among hrHPV-positive women, more women had a cytological abnormality after self-sampling than after clinician-collected sampling (37.2% vs. 32.2%) [Citation32]. The same study claims that self-sampling has a higher CIN2+ specificity than the clinician-collected test. In other studies, it was noted that genotypes different than HPV16/18 were more often found in self-sampling material [Citation25,Citation32,Citation48]. Similarly, results from a meta-analysis (2018) show that PCR-based hrHPV assay is sensitive for the detection of CIN2/3+ but slightly less specific on self-samples compared with clinician-collected samples.
The assays based on signal amplification, like Hybrid Capture II (HC2) and Cervista, have lower sensitivity to detect CIN2/3+ and less specificity to exclude CIN2+ on self-samples. On the other hand, when mRNA testing like APTIMA was used, self-sampling was less sensitive but equally specific as clinician-collected samples. Further studies have compared the accuracy of self-samples with clinician samples using specific sampling devices or storage media, and the accuracy does not vary substantially across clinical settings [Citation49].
One novel hrHPV DNA assay is careHPV. The use of this test demonstrates not only the utility of the biomarker but the wide range of benefits that lower-cost testing can provide to women, particularly in developing countries [Citation49,Citation50]. It has a cost around $5 per test, and it is able to detect a pool of 14 hrHPV genotypes in three hours. The method used for this testing does not require specialized training and education, and importantly, it possesses high sensitivity and specificity towards precancerous and cancerous lesions [Citation50]. The test can be performed with both clinician-collected samples and self-collected samples. Furthermore, after the implementation of careHPV as primary screening in China, Zhao et al. showed that the assay has the best cost-effectiveness performance and the highest benefit-cost ratio in comparison to HC2, and Pap cytology [Citation51,Citation52].
The main advantage of implementing the primary hrHPV DNA screening into the nationwide program is a higher detection of CIN2+ lesions. However, at the same time, more colposcopy referrals per screening round are needed in order to detect one CIN2+ lesion compared with cytology-based testing [Citation32]. Further research also confirmed the usefulness of this biomarker, even after CIN treatment. In a systematic review and meta-analysis by Kocken et al.the authors described that hrHPV DNA testing had a higher sensitivity than cytology six months post-treatment in women with CIN2+, without reducing its specificity [Citation53]. Thus, hrHPV DNA testing could also be included in the follow-up of treated women to detect post-treatment lesions. Still, additional triage tests are required to distinguish hrHPV DNA positive women with high risk for cervical disease from women with transient infections, which could reduce overdiagnosis, unnecessary referrals, and follow-ups, and therefore, should be a priority [Citation40].
2.2. hrHPV genotyping
Although the prevalence of specific hrHPV types varies geographically, several epidemiological studies have shown that the most frequent genotype in invasive cervical cancer is HPV16, followed by HPV18, with both accounting 70% of all cases [Citation10,Citation40,Citation41,Citation54] . HPV45 is rarely found in women with normal cytology or low-grade lesions compared to HPV16, but it is the third most common HPV genotype in invasive cervical cancer [Citation55]. Vinokurova et al. reported that HPV16, 18, and 45 are more likely to be integrated into the host cell genome than other HPV genotypes, triggering chromosomal instability that will lead to earlier tumorigenesis by activating cellular oncogenes or inactivating cellular tumor-suppressive genes. Thus, these genotypes are associated with an elevated risk of cervical cancer [Citation54].
There is an increasing interest in using the hrHPV genotyping test as an additional biomarker to better predict the risk for cervical cancer, in part because this method is also applicable for self-sampling material. A study using self-sampling with dry collection medium (FTA elute micro card), instead of a liquid medium, have shown that HPV genotypes found in these samples are almost identical to the ones present in the samples collected by physicians. Some viral genotypes are only positive in self-samples, as the vagina carries HPV genotypes that are not present in the endocervix [Citation56]. Moreover, the American Society for Colposcopy and Cervical Pathology proposes that positive hrHPV women with normal cytopathological test should undergo HPV genotyping analysis, instead of waiting to be rescreened after one year. This will make it possible to triage women with a low risk of progressing to CIN3/cancer from those with a higher risk [Citation10]. Additionally, Ebisch et al. suggested that the combination of HPV16/18 genotyping with cytology testing may improve the referral rate and specificity for detecting CIN3+ lesions, maintaining the sensitivity during triage [Citation57].
The ATHENA study is one of the largest clinical trials to assess the performance of the HPV genotyping test. They showed that the proportion of women positive for the 14 hrHPV genotypes are seen to rise with increasing CIN grade across all age groups [Citation58]. In addition, the Kaiser Permanente Northern Carolina and NHANES study showed that the prevalence of 14 hrHPV genotypes, including HPV16 and 18, may vary according to the age groups. Their prevalence increases in the age group of 14–24 years old and might have a nonsignificant gradual decline in the age group of 25 years old and older [Citation58–60]. HPV genotyping based on the detection of HPV16, HPV18, or a pooled detection of 12 more hrHPV types (31, 33, 35, 39, 45, 51, 52, 56, 58, 59, 66, 68) in cervical scrapes reached a sensitivity of 90.0%, specificity of 70.5%, PPV of 14.0% and NPV of 99.2% according to ATHENA study. If a woman is positive for HPV16 or 18, immediate referral to colposcopy is suggested, whereas positivity for other HPV genotypes leads to cytology referral. Therefore, a negative result provides the safety that CIN3+ lesions will not develop in the next three years [Citation58].
Overall, this viral biomarker may be useful to triage women after a positive primary screening (hrHPV DNA testing, cytology). Therefore, this marker can potentially be used to stratify women with a higher risk to develop CIN3+ lesions and progression to cervical cancer. hrHPV genotyping may also support the categorization of women with ASCUS/LSIL/CIN1, who have a risk of progression to precancerous lesions because hrHPVs tend to progress these lesions to malignancy. However, further research is needed to confirm its utility in abnormal low-grade lesions, and its value with other indicators, which may enhance its performance for cervical cancer screening.
2.3. Viral load
In the HPV life cycle, viral load reflects DNA replication, and the levels define the course of hrHPV infection [Citation61]. Several studies reported that high HPV viral load (defined as the number of HPV DNA copies per cell) is associated with persistent infection and linked to increased risk of cervical intraepithelial neoplasia (CIN) and cervical cancer. In particular, HPV16/18 DNA positive women with high DNA load are more likely to progress to high-grade CIN [Citation61–64]. Halec et al. showed that 93% of high load hrHPV infections present in smears are also HPV DNA and mRNA positive in their corresponding tissues, representing active infections [Citation65]. Based on this information, the viral load could be used as a triage tool for HSIL+ in hrHPV16, 18, and 58 positives, to determine the group of HSIL+ patients who require treatment [Citation62]. Furthermore, increased hrHPV viral load may also lead to immune suppression in the microenvironment and result in therapy resistance, affecting the survival of patients. Therefore, although the viral load has lower levels during early infection, the progression to persistent infection, and cervical cancer may result in an increment of the viral load, which associates with high-grade lesions and malignancy [Citation66,Citation67]. In contrast, among patients with ASCUS diagnosis, the viral load might not be able to predict their progression to CIN [Citation68].
Viral load during infection and disease progression can vary within hrHPV genotypes, which might be a limitation for this marker. Initially, Carcopino et al. found that in women referred to colposcopy, hrHPV16 viral load had a specificity of 91.0%, and a sensitivity of 58.2% for CIN2+ lesions (cut off: 3.0 × 106 copies per million cells); in comparison, they reported that hrHPV18 has a poor predictive value [Citation69]. Nevertheless, Schmitt et al. recently determined that detection of multiple viral loads from different hrHPV genotypes at the same time might be a good predictor of precancerous lesions, including ASCUS, but it is not able to distinguish LSIL from HSIL [Citation70]. Another limitation is the heterogeneity of the samples. If low-grade lesions surround high-grade lesions, cervical scraping might result in heterogeneous cytology samples with a variable fraction of abnormal cells [Citation71]. Therefore, this might have a direct effect on viral load assessment. On the other hand, heterogeneity of cytological samples can be corrected by calculating the viral integration status, which could be done by the quantitative viral load assessment of type-specific HPV E2 and E6 and by defining the ration of the integrated load to the total load [Citation62].
While in cervical cancer, higher viral loads are associated with a higher risk of progression, in HPV-related oropharyngeal cancer, this relation is more variable. Some studies reported strong links between high HPV viral loads and better survival in HPV related oropharyngeal cancer, anal cancer, and vaginal SCC [Citation65]. In contrast, other studies found that HPV copy-number is associated with an increased risk of cervical abnormality, but that a single viral load does not predict the risk of CIN. Besides, it must be noted that viral replication is not necessary for maintaining the malignant phenotype as not all HPV copies are transcriptionally active [Citation63].
Thus, testing for a single HPV viral load might not be relevant for estimating the outcome of hrHPV infections, particularly for LSIL and NILM. However, taking serial samples, detecting multiple hrHPV genotypes viral loads, or combining hrHPV viral load with additional biomarkers such as hrHPV transcriptional status may be useful for evaluating disease progression [Citation63]. Depending on the approach, this marker may be able to detect both LSIL and HSIL, supporting the triage of women with a positive primary screening. Further studies and meta-analyses should clarify these limitations and evaluate its specificity and sensitivity towards the different stages of disease in order to consider it for screening programs. Still, the viral load remains an attractive candidate, mainly because of its application with additional indicators and the ability to use it on self-samples [Citation64].
2.4. hrHPV transcriptional status
In addition to hrHPV DNA testing, which only gives information about the virus presence, a significant interest is focused on the use of viral mRNA as a biomarker, particularly the upregulation of E6/E7 oncoproteins, to further understand the infection state [Citation10,Citation72].
As mentioned, persistent HPV infections are associated with a higher risk of integration of the viral DNA into the host genome. Indeed, frequent viral integration seems to increase with CIN severity [Citation54]. hrHPV integration leads to the disruption of the E2 gene, which results in the upregulation of E6 and E7 oncogenes. Additionally, integration of the HPV genome causes the generation of fusional viral–cellular transcripts with higher stability, resulting in further increased expression of E6 and E7 [Citation73]. The increased levels of E6 and E7 proteins interfere with host cell factors such as p53 and pRb that control the cell cycle and apoptosis . This provides a proliferation advantage to infected epithelial cells, promoting chromosomal instability that finally results in cancer development [Citation54].
Figure 3. Downstream cellular effects of hrHPV E6/7 upregulation. Once expressed, viral E7 interferes with the pRb pathway by binding it and, hence, provoking the release of the E2F transcription factor. E2F will translocate to the nucleus where it will transcribe different proliferation-promoting genes; among these: MCM2 and TOP2A are overexpressed. The E7-pRb binding will avoid the negative feedback loop essential for the p16INK4A regulating protein (p16), which will be overexpressed to compensate for the following high proliferation level. At the same time, E6 binds p53 by promoting its degradation, which will cause the release of the Sp1 transcription factor, and also the impairment of p53-mediated interference with the pRb pathway. In fact, p53 prevents the phosphorylation of pRb; thus, the consequent activation of E2F. This event leads to the inhibition of apoptosis and transcription of Sp1-associated genes, like DNA methyltransferase enzymes (DNMTs) that will enhance the methylation of various promoters associated with HPV-mediated processes. The combination of these changes will provoke the boosted proliferation of the cell, which will be a signal for the production of Ki-67, a nuclear protein. In addition, E6 will upregulate the expression of TERT and TERC, essential for repairing telomeres. Soon, the cell could gain the chromosomal regions containing these two elements (3q and 5p), leading to genomic instability. It is believed that these events will facilitate viral genome integration, resulting in transforming cells. The abnormally over-expressed molecules involved in this process represent useful cellular biomarkers. TERC: telomerase RNA component; TERT: telomerase reverse transcriptase gene; MCM2: minichromosome maintenance protein 2; TOP2A: topoisomerase II alpha; CDK4/6: cyclin-dependent kinase 4 and 6
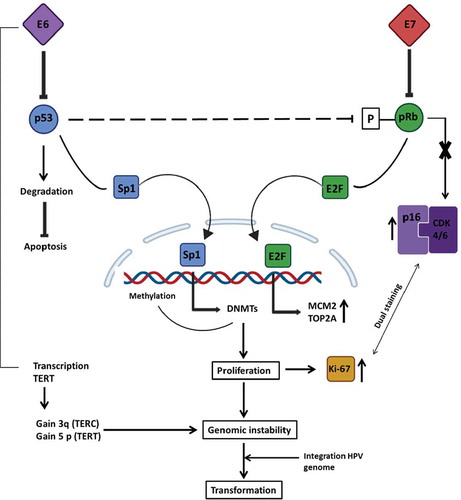
Since E6/E7 overexpression is correlated with neoplastic features, the transcriptional status of these oncogenes is an excellent predictive marker for progression from HPV infection to high-grade CIN [Citation74,Citation75]. It has been observed that E6/E7 mRNA positivity is higher in women with high-grade lesion than with low-grade lesion or without lesion and that both E6/E7 mRNA levels and severity of the cervical disease increase together [Citation76,Citation77]. Consequently, E6/E7 negativity is higher in women that do not have any lesion, which could mean that the virus stays in an episomal state or that the regulation of its transcription is still controlled, allowing a spontaneous clearance of the infection [Citation75] .
Several studies have compared the performance of E6/E7 mRNA-based assays with hrHPV DNA testing, and they all concluded that E6/E7 mRNA testing had higher specificity and PPV for HSIL and invasive squamous cell carcinoma (ISCC), compared to hrHPV DNA testing [Citation78–81]. Furthermore, Fontecha et al. claim that the mRNA test combined with cytology has more clinical relevance than hrHPV DNA screening in women with low-grade lesions [Citation75]. Similarly, in a recent meta-analysis by Macedo et al.it was reported that E6/E7 mRNA has a sensitivity of 93.9% and a specificity of 61.7% for CIN2+ lesions [Citation82].
The effectiveness of U.S. FDA approved hrHPV E6/7 mRNA assay (APTIMA) was evaluated by Stoler et al.who concluded that among women with ASCUS cytology, detection of hrHPV E6/E7 oncogenic mRNA is a significant triage method for colposcopy referral. This means that fewer women will be exposed to harmful and invasive procedures and that more cost savings in screening programs could be achieved [Citation83]. Bruno et al. confirmed that women with ASCUS/LSIL diagnosis and E6/E7 mRNA positive results have a higher risk of cervical lesions progression, therefore demonstrating the usefulness of this test for cervical cancer screening [Citation84]. Similarly, Rijkaart et al. showed that hrHPV mRNA testing (PreTect HPV Proofer) is also valuable for the detection of hrHPV DNA-positive women with normal cytology that present positivity for E6/E7 mRNA, indicating that the virus is oncogenically active before detecting abnormalities [Citation85]. They claim that hrHPV-DNA positive women with normal cytology, but upregulated E6/7, have a higher risk of developing CIN2 + . Therefore, hrHPV mRNA testing could early predict the potential of oncogene transformation to severe dysplasia, detecting those women who need direct colposcopy referral [Citation78,Citation85]. This could limit unnecessary follow-ups and over referral of patients with transient HPV infections [Citation78,Citation85].
Some studies have demonstrated that hrHPV mRNA testing on self-collected samples might have similar sensitivity and specificity in detecting HSIL as physician-collected cytology. For instance, the negative predictive value (NPV) on HPV self-samples (83.3%) seems lower but similar to the clinician-taken (88.3%) [Citation30,Citation86]. In comparison, another study reported that this collecting method has an acceptable sensitivity and specificity for the detection of CIN2+, and it considered that self-sampling was less sensitive for CIN2+ than physician-collected sample given that hrHPV detected was present in the vagina and was not associated with CIN2 in the cervix [Citation87]. Nonetheless, the E6/E7 mRNA test as a biomarker may have some limitations. Discacciati et al. claim that it is not a good long-term prognostic marker because HPV DNA positive and E6/E7 mRNA negative women could become E6/E7 mRNA positive throughout their lives because of oncogenic activation of the virus, changing the clinical behavior of the lesion. Thus, they should still undergo follow-up examinations or treatment since their risk of CIN2+ is too high [Citation88].
Overall, hrHPV transcriptional status might be a right candidate as a primary or secondary screening for cervical cancer. Its sensitivity and specificity towards cervical lesions are as high as hrHPV DNA testing. As a population-based screening setting, it has proved to decrease the number of cytology assessments at the cost of increased colposcopy referral rate [Citation35]. In addition, it may also help to triage women with other positive primary screening, confirming the need for a colposcopy referral. Likewise, the biomarker’s ability to be used with self-samples and alongside other markers makes it an appealing possible new testing target. However, further research is needed to determine its performance after CIN treatment and clarify its limitations.
2.5. Viral E4 protein
E4 is the most abundant viral protein and is synthesized in the mid-layers of the epithelium for viral replication and virion formation, showing the onset of a productive HPV infection [Citation89]. In the upper layers of the epithelium, E4 proteins accumulate within the lesion in different amounts, depending on the lesion grade. This allows for the identification of sites with an active infection in biopsy material [Citation90].
One of the characteristics of productive HPV infections is the presence of koilocytotic cells in the mid-layers of the squamous epithelium that are visible in Pap smears and cervical biopsies. These cells contain an acentric and hyperchromatic nucleus relocated by a perinuclear vacuole filled with glycogen [Citation7,Citation91]. The onset of E4 expression coincides with the beginning of vacuolation and loss of defined nuclear margins that subsequently form koilocytotic cells [Citation7,Citation91]. This morphology contributes directly to virus replication in the upper epithelial layer while causing keratinocyte fragility and cytopathic effect, hindering the assembly of the cornified envelope [Citation92]. Therefore, koilocytosis is a negative predictor of CIN3+ as it correlates with a low-grade squamous intraepithelial lesion (LSIL) and non-progressive CIN1 lesions [Citation93,Citation94].
Levels of E4 decrease with the severity of the lesion. E4 is highly expressed in CIN1, and low levels of E4 are found in CIN3. E4 is, therefore, a potential biomarker of clinically transient insignificant disease cleared by the immune system [Citation7,Citation89]. It is also of value for detecting and monitoring the extent and persistence of LSIL and its possible progression to higher-grade lesions [Citation90]. Thus, E4, as a biomarker, could facilitate the division of the heterogeneous CIN2 patients into those who resemble the CIN1 group (E4 positive) and those who are more likely to evolve to a CIN3 stage (E4 negative) [Citation95]. Moreover, the utility of this protein as a biomarker has been tested by several studies. One recent research used E4 combined with MCM2 (a host proliferation marker) to identify insignificant cervical transient infections and found that in CIN2+ lesions, MCM2 was highly expressed while E4 was not present. On the contrary, <CIN1 lesions showed most cells stained positive for E4 [Citation11]. Similarly, Griffin et al. compared the expression of E4 to p16INK4A, another host marker, and the hypermethylation of some host-cell genes, which increases with CIN severity. They claim that E4 expression is correlated with low hypermethylation and the absence of p16INK4A, which is not observed in cervical carcinoma, as described in additional studies [Citation7,Citation96,Citation97].
The described significant expression of E4 during the progression of hrHPV infections may allow its implementation in multiple steps of cervical cancer screening and management. E4 testing can act as a triage of women with positive primary screening and stratify them into those with a risk to disease progression or regression. It may support the follow-up of ASCUS/LSIL diagnosis because its detection could serve as an indicator of cervical lesion progression. It may also help with the follow-up of treated women, determining those who may develop therapy resistance. Nevertheless, further studies should confirm these functional activities and indicate the sensitivity and specificity towards cervical lesions during their progression and regression.
2.6. Viral DNA methylation
Epigenetic alterations like DNA methylation are essential mechanisms of gene regulation in cell development and defense. In addition to changes in methylation of the host genome, HPV DNA methylation is being used as a new biomarker for cervical cancer screening in hrHPV-infected women. DNA methylation is a defense cellular mechanism from the host to silence invading foreign viral genomes and to inhibit viral replication [Citation98]; therefore, the methylation of HPV DNA may be the host response to the infection, but it might also be the result of the viral switching from a productive infection to neoplastic transformation [Citation99,Citation100]. The detection of these epigenetic changes, particularly the early (E) and late (L) promoters of HPV, may be useful as a predictive or diagnostic biomarker among HPV-positive women.
The research in viral methylation (m) has been focused on different regions of HPV DNA. For instance, one study recently concluded that the degree of L1m in hrHPV (16, 18, and 52) was associated with the severity of the cervical lesion, but the same pattern was not found in HPV58 [Citation98]. Another study by Wentzensen et al. observed that the HPV genotypes 31, 18, and 45 had hypermethylated regions of E2, L2, and L1 and low methylation in E6, E7, and E1, and that there is increased methylation at the viral CpG sites in CIN3 [Citation95]. Likewise, Kalantari et al. determined that the methylation pattern of viral CpG sites within the HPV16 late promoter may increase with the grade of lesions. They found that 10% to 12.2% of all CpGs were methylated in asymptomatic infection and ASCUS, but in LSIL/CIN1 samples, methylated CpGs increased to 13.6%, while in HSIL/CIN2+ lesions to 31.9%, and in cancer to 83.1%. They also reported similar results to HPV18, 31, and 45, which demonstrate that the methylation pattern of this viral region in disease progression may behave alike among hrHPV genotypes [Citation101]. Thus, these findings suggest that viral DNA methylation patterns might increase with disease progression. Additionally, an investigation from Brazil studied HPV DNA methylation in E1/E2 regions in cervical cancer samples, and they found higher methylation with intact E1/E2 for HPV16 and 18 [Citation102]. Clark et al. also showed that methylation of HPV was associated with 12 genotypes, marking the transition from hrHPV infection to precancer. This methylation assay showed higher sensitivity (80% vs. 76.6%) and lower test positivity compared with cytology (38.5% vs. 48.7%) [Citation103].
The use of viral DNA methylation may be beneficial for the triage of women. This biomarker might be used to detect high-grade lesions, therefore, improving referral to colposcopy . Although its utility looks promising, the overall results suggest that the host cellular epigenetic machinery may respond differently to viral genomic regions such as the early and late promoters, and hrHPV genotypes. Thus, it is necessary to consider these limitations, and evaluate the sensitivity and specificity towards HSIL in future studies and meta-analyses before its application in cervical cancer screening and management.
3. The cellular biomarkers
Since many years are required for a persistent HPV infection to become cervical cancer, eventually, various cellular changes occur in the host. These can reflect an abnormal situation, indicative of an HPV-related cellular transforming process, and serve as host biomarkers. Therefore, to identify reliable biomarkers and how they function, the global picture of the involved altered molecular pathways should be considered [Citation104–106].
3.1. From an HPV-infected to a transforming cell
The expression of HPV oncoproteins, E6 and E7, is the key element in the alteration of the cell cycle, compromising various host pathways . The alteration of such pathways, over the years, could exacerbate, leading to the development of cervical cancer. As shown in , the progressive expression of E6/E7 viral proteins compromises many cell cycle-associated regulatory processes with time, generating the abnormal coexpression of some molecules that can be considered cellular biomarkers in HPV-related pathological circumstances. Thus, these altered-pathways biomarkers can be used and combined in different clinical phases to track the progression of the pathologic development [Citation2].
3.2. p16INK4A
Located on chromosome 9p21, the p16INK4A gene belongs to the CDK inhibitors family. Together with p19ARF, it performs an anti-proliferative activity. p16INK4A and p19ARF proteins interfere, respectively, with pRb and p53 pathway [Citation105,Citation106]. p16INK4A (p16) is a hrHPV specific progression biomarker, and its reliability has been broadly studied. In fact, Carozzi et al. showed that p16 positive women, who were monitored in the follow-up, presented more frequency of CIN2 or worse (8.8%) when compared to the p16 negative women (3.7%), especially in the age interval 35–60 years. Remarkably, the authors prove that p16 positivity combined with HPV positivity is a significant marker to distinguish patient who need an immediate colposcopy referral (HPV-positive, p16-positive) from those who can avoid immediate colposcopy and can be safely managed with repeat screening after 2–3 year intervals (HPV-positive, p16-negative)[Citation107]. In multiple studies, a higher number of p16 positive results were associated with a higher grade CIN, and a weaker expression of p16 correlated to less severe CIN lesions, confirming the role of p16 as a progressive biomarker in the cervical disease development [Citation39,Citation105,Citation106,Citation108]. In fact, p16 expression associates with the trend of cytology grading, an observation reported by Zeng et al.who described lower expression in NILM (9.28%), higher in ASCUS (33.33%), increased in LSIL (53.37%), and even higher in HSIL (95%). Thus, demonstrating its utility in distinguishing high-grade cervical lesions [Citation109]. In practice, p16 staining could be used ancillary in cervical cytology and histology [Citation110–112]. In a population-based screening setting it could be performed on an additional slide from the same scrape, from which cytology was made, using commercially available kit (CINtecPlus, Roche mtm Laboratories, Germany). However clear guidelines should be available regarding its use in routine practice, as a predictive evaluation of the scoring systems to reach a consensus on the threshold of positivity is unclear [Citation113].
The regulatory role of p16 depends on a negative feedback loop given by pRb. In healthy conditions, p16 binds CDK4/6, preventing its linking to cyclin D . They form a complex that would phosphorylate pRb, causing the release of E2F, a transcription factor that enhances the G1/S transition genes. The CDK4/6/p16 interaction avoids this process, leading to cell cycle regulation. However, in an HPV-infected cell, E7 competes with E2F for pRb binding, provoking the activation of the transcription factor and, hence, the E2F-correlated-genes transcription. This mechanism leads to the annulation of the pRb-p16 negative feedback loop, bringing to an abnormal overexpression of p16, preventing its interaction with CDK4/6, and leading to cell cycle re-entry and progression. This process is driven by the virus to allow neoplasia and genome amplification. However, it is important to mention that p16 upregulation is not always HPV-driven [Citation105,Citation106]. p16 overexpression in non-HPV-related cancers was observed in malignant transformation of both pre-malignant lesions as well as in malignant tumors [Citation114–116]. While p16 overexpression in benign lesions is associated with senescence induction in response to oncogenic stimuli, its overexpression in malignant tumors is related to Rb-pathway alterations and almost always correlates with high Ki67 index [Citation117]. Rb loss is a frequent event in many neoplasms and is associated with uncontrolled cell proliferation. In this sense, deregulation of Rb results in increased p16 expression in tumor cells and cancer tissue due to positive feedback [Citation118]. Because of this pathway, p16 dysregulation has been correlated to many malignancies, including cervical cancer. Eventually, E7 mediates the modification of host histone architecture; among these, p16 promoter will also be activated, causing an even higher expression of the protein [Citation119–121]. On the other hand, patients with cervical cancer have less expression of p16 due to hypermethylation of its promoter, leading to unsuccessful suppression of tumor growth, and therefore allowing cancer development [Citation122–124]. The methylation of the p16 promoter also seems to increase with the stages of the disease, particularly in CIN1 and CIN2 lesions [Citation122,Citation125,Citation126]. Similarly, the presence of some deleterious SNPs may lead to high/medium expression of p16 [Citation127]. Thus, even though p16 represents an excellent biomarker, such observations should be considered as limitations of this marker. Interestingly, specific SNPs in p16 have been recently associated with either protection or risk to cervical cancer lesions, which further increases p16 value in screening HSIL [Citation128,Citation129].
Figure 4. Mechanism of regulation mediated by p16INK4A during healthy conditions and HPV infection. In healthy non-HPV-infected cells (left), p16INK4A (p16) serves as a fundamental regulator of the cell cycle induction. When a cell has to go through G1/S transition, the cyclin-dependent kinases 4 and 6 (CDK4/6) forms a complex with cyclin D. The entire complex phosphorylates the retinoblastoma protein (pRb), which, in normal conditions, binds the transcription factor E2F. The phosphorylation mediated by the CDK4/6-cyclinD complex provokes E2F release, which promotes the transcription of those essential genes for the G1/S transition phase. At the same time, when the same healthy cell has to go towards cell cycle arrest, the regulatory protein p16 forms a complex with CDK4/6, preventing its binding to cyclin D and, hence, the transcription of E2F-correlated genes. However, in an HPV-infected cell (right), the viral protein E7 competes for the binding of pRb, and their linkage permits the release of E2F and the transcription of G1/S-genes. The expression of p16 will try to regulate the entire process, but the transcription factor release does not depend on CDK4/6-cyclinD phosphorylation; instead, it depends on E7 viral activity. Negative feedback mediated by pRb-E2F controls the expression of p16; hence, since this complex no longer exists, the loop is disrupted, and this will result in overexpression of p16 even if its regulatory activity is not accomplished
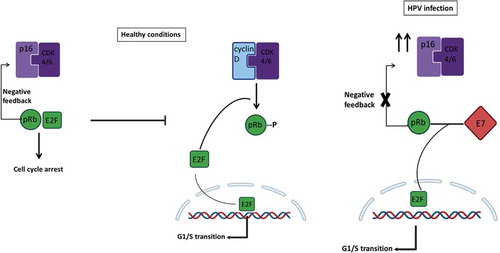
The subcellular location of p16 might play a significant role in its functional activities. The protein can be found in the nucleus and cytoplasm, where it can inhibit CDK4/6 or get sequestered by CDK4, AE1, or other proteins, respectively [Citation106]. Therefore, although the overexpression of p16 will lead to increased inhibition of CDK4/6, it may not be able to induce its anti-tumor activity in the cytoplasm after HPV persistent infection and disease progression. This was recently described by Mendaza et al.who determined that the absence of nuclear p16, as a result of cytoplasmic sequestration, may be an indicator of progression from HSIL to cervical cancer, and poor outcomes [Citation110]. Thus, further research is needed to clarify the subcellular localization of this protein and the meaning of these findings in terms of its utility as a tumor suppressor and as a biomarker for the disease.
Overall, as a single biomarker of disease, p16 overexpression may facilitate the triage of women with initial positive screening, and its association with HSIL might support prompt diagnosis and progression of LSIL. However, it is important to consider the downregulation of p16 due to hypermethylation of its promoter and the effect of particular SNPs in its half-life. Likewise, the absence of nuclear p16 may support differentiating HSIL from cervical cancer, which might be helpful in patients with CIN2+ diagnosed lesions.
3.3. Ki-67 and dual staining
Due to the E6/E7-caused dysregulation in the cell, the abnormal proliferation triggers the expression of Ki-67. The latter is a nuclear protein that can be found expressed in all the cell cycle phases, excluding G0. In a normal situation, its expression is limited to the basal and parabasal layers of the cervical squamous epithelium but in the HPV-pathological condition, it is expressed in more than one-third of the epithelium. Its extension and high expression in the epithelium in proliferative circumstances make Ki-67 an interesting biomarker to consider [Citation130,Citation131]. Moreover, the coexistence of Ki-67 and p16 is rarely observed in healthy conditions, meaning that, when found overexpressed, these two biomarkers indicate an HPV-related alteration of the cell cycle. Indeed, the coexpression of p16/Ki-67, in 138 cervical biopsies, was detected only in dysplastic lesions [Citation132,Citation133]. In light of these findings, p16/Ki-67 dual staining has been proposed and validated as a triage test after hrHPV positivity [Citation31]. Surprisingly, the p16/Ki-67 dual staining showed a higher sensitivity for CIN3, if compared to p16 staining alone [Citation130]. A recent meta-analysis demonstrated that p16/Ki‐67 staining is more specific for CIN2+/CIN3+ than hrHPV DNA testing. The researchers also pointed out that, although p16 staining is less sensitive for CIN3+ than hrHPV DNA testing, dual staining has similar sensitivity. In addition, the sensitivity of the co-staining in cervical samples was higher than the cytology test, presenting a similar specificity [Citation134–136].
Remarkably, Ebisch et al. suggested that the hrHPV-based screening could benefit from the replacement of the Pap cytology test for p16/Ki-67 dual staining, considering the reduced role of morphology, the higher specificity for CIN3, and the maintained adequate sensitivity. At last, the adding of dual-stain cytology following the low-grade Pap cytology test can also be considered in the diagnosis strategy [Citation137]. Schmidt et al. also reported a similar conclusion, in women with initial ASCUS/LSIL cytology and follow-up testing with p16/Ki-67 staining, this biomarker showed a sensitivity for CIN2+ of 92.2% (ASCUS) and 94.2% (LSIL), while having a specificity of 80.6% and 68.0%, respectively [Citation112]. Thus, showing that this strategy is useful for detecting HSIL, which could avoid unnecessary colposcopy and allow a broader timeframe of follow-up between hrHPV DNA positive, ASCUS/LSIL cytology, and negative triage women [Citation132].
3.4. TOP2A and MCM2
Due to the elevated expression of E6/E7 viral proteins in the cell during the oncogenesis process, E7 binding to pRb promotes E2F transcription factor release, which enhances the prolonged-expression of G1/S phase genes, among those, TOP2A and MCM2. Topoisomerase II alpha (TOP2A) plays a vital role in S-phase because of its enzymatic uncoupling activity during the DNA replication process. On the other hand, minichromosome maintenance protein 2 (MCM2) is essential since it loads complexes onto DNA before replication and permits the synthesis through its helicase activity [Citation108,Citation138]. Both proteins increase in the proliferating cells after the aberrant S-phase induced by hrHPV oncoproteins E6/E7. Thus, the overexpression of both during aberrant S-phase is currently utilized to detect the disease in research settings. In addition, both seem to be considerably expressed during the transition from CIN2/3 to invasive cervical cancer (ICC); in fact, higher levels were detected in more severe cervical lesions, suggesting their potential role as progressive late biomarkers [Citation131,Citation139]. Furthermore, one recent study from Brazil tested the effectiveness of this biomarker with Ki-67, and they concluded that TOP2A is very useful to diagnose high-grade lesions (HSIL). At the same time, Ki-67 is useful for atypical squamous cells of undetermined significance (ASCUS) and low-grade lesions (LSIL) [Citation131]. However, further validation is needed to confirm this finding.
One of the readily available kits that use the detection of TOP2A and MCM2 overexpression is the ProEx™ C immunoassay for in vitro diagnostic. The assay works with monoclonal antibodies to detect MCM2 and TOP2A proteins in abnormal cervical tissue. Dixon et al. concluded that this assay showed intense nuclear staining correlated with cervical lesion severity [Citation121]. When the performance of BD ProEx™C assay was compared to other markers such as p16INK4A, one study concluded that these three biomarkers (MCM2, TOP2A, and p16) have the same sensitivity and specificity in the detection of high degree CIN [Citation108]. Also, when it was compared to hrHPV DNA testing, Hybrid Capture 2 (HC2; Qiagen®), the assay demonstrated high specificity but lower sensitivity for CIN2+ lesions, a limitation to consider for triage of ASCUS and LSIL [Citation140]. Alaghehbandan et al. confirmed this finding, determining that both ProEx™ C and E6/E7 mRNA testing have higher specificity but lower sensitivity than hrHPV DNA testing for the detection of CIN2+ in women with initial ASCUS/LSIL cytology [Citation141]. Additionally, Ding et al. recently demonstrated that women with an initial diagnosis of LSIL by ProEx™ C immunostaining have a higher risk of developing HSIL in the next two years. They showed that this test might achieve a sensitivity of 75.0%, specificity of 64.5%, PPV of 30.8%, and NPV of 92.40% in LSIL lesions that may progress to HSIL [Citation142]. Therefore, these overall results suggest that TOP2A/MCM2 biomarkers have excellent performance for both low and high-grade lesions.
TOP2A/MCM2 biomarker correlates with hrHPV DNA positive cells and has high effectiveness in the detection of cervical lesions. However, its utility should be elucidated with further meta-analyses that corroborate its specificity and sensitivity during cervical cancer progression [Citation139,Citation143]. Although more research is needed, this biomarker may also be helpful to triage women with LSIL before the lesions progress to HSIL and to confirm CIN2+ lesions. Furthermore, one new approach for the use of MCM2 as a biomarker is the detection of its gene in samples. Tang et al.using bioinformatics analysis, concluded that the MCM2 gene plays an essential role in cervical cancer, and it might represent an important marker for diagnosis [Citation144].
3.5. Chromosomal alterations
Cervical cancer is characterized by chromosomal aberrations and the integration of the viral DNA to the host genome. The ICC-correlated aberrations that have been observed are loss regions (2q, 3p, 4p, 5q, 6q, 11q, 13q, and 18q) or gain regions (1q, 3q, 5p, 8q) in the chromosomes, which some of them been found in precancerous lesions; hence, they can function as biomarkers. The most observed chromosomal alteration in precancerous stages is a gain of 3q [Citation31]. Overall, it is now believed that the global genetic instability coming from HPV-induced aberrations would be the cause of HPV-genome integration into host DNA and not the consequence [Citation145].
Additionally, the expression of the viral oncoproteins, among many things, leads to the gain or loss of specific chromosomal regions, depending on the advantage of HPV-infected cells. Remarkably, evidence has been presented on the expression of TERC (telomerase RNA component) and TERT (telomerase reverse transcriptase gene) in an HPV-infected cell. Both are critical for the process of repairing telomeres avoiding cell death, which is useful to obtain the immortalization of the cells. Interestingly, both chromosomal regions containing those elements have been observed in cervical carcinogenesis: 3q for TERC and 5p for TERT. In transforming cervical cells, 3q gain represents an advantage since not only TERC is contained and widely transcribed in this chromosomal region, but also PIK3CA. The latter codifies for the p110a catalytic subunit of phosphatidylinositol-3-kinase, an essential enzyme regulating apoptosis and cell growth [Citation135,Citation145,Citation146]. The gain of 3q26, which is the specific region containing TERC, has been directly associated not only with cervical cancer but also with its severity grade. 3q gain seems to represent an optimal early-stage biomarker due to the generated genomic instability anticipating HPV DNA integration [Citation147].
Wright et al. studied the amplification of this region as a biomarker. The analysis of 199 samples resulted in a sensitivity of 92.9% (95% confidence interval [CI], 76.5–98.9%), a specificity of 100% (95% CI, 97.8–100%), a positive predictive value (PPV) of 100% (95% CI, 86.7–100%), and a negative predictive value (NPV) of 98.8% (95% CI, 95.9–99.8), for distinguishing CIN2 and CIN3 from normal epithelia [Citation148]. The study demonstrated supporting evidence of this marker in the clinical evaluation of hrHPV-infected women. Interestingly, Sokolova et al.by studying the genomic instability in epithelial cells of cervical cytology samples, determined that the gain of 3q26 was positive in 100% of cancer samples, 90% of CIN3, 78% of CIN2, 26% of CIN1, and 0% of normal samples [Citation149]. Therefore, demonstrating the progressive state of this chromosomal gain and its utility for CIN2+ lesions. Likewise, Heitman et al. showed that this biomarker could be used as a negative triage test for LSIL, having a sensitivity and NPV of 80% (95% CI, 28–99%) and 98% (95% CI, 87–100%), respectively, for 3q26 gain in women with LSIL cytology [Citation150]. In contrast, recent research by Koeneman et al.using the FISH technique, showed that the absence of 3q26 gain in diagnostic biopsies might be applied to identify high-grade CIN lesions with a high probability of disease regression [Citation147,Citation150]. 3q26 gain was found in women with persistence and regression of high-grade CIN [Citation147]. Still, none of the women without a 3q26 increase showed disease persistence. They also concluded that a 3q26 increase could be a biomarker for the identification of CIN with a high probability of disease regression instead of progression to cervical cancer. Thus, more studies with a larger sample population are imperative for conclusive results about the value of 3q26 as a biomarker of cervical cancer.
Regarding chromosomal loss of heterozygosity (LOH), the most altered chromosomes are 3 and 6. LOH at 3p14.2 and 6p21.2 have been associated to recurrent cervical dysplasia after progression to cervical cancer and recovery post-treatment, respectively [Citation151,Citation152]. In addition, Arias-Pulido et al. demonstrated that a mutation in the HLA system, which increased the development of carcinoma in situ and ICC in HPV16 positive patients, was also associated to the generation of LOH at chromosome 6. The team further suggested that such chromosomal aberration could be the result of viral persistence [Citation153]. Interestingly, LOH at chromosome 6 has been found as a marker of progression from CIN to cervical cancer [Citation154]. Likewise, Chambuso et al. confirmed that HPV alone can induce LOH at chromosome 6, but additionally the researchers observed that women co-infected with HIV may intensify this alteration, and therefore have higher risk of CIN progression [Citation155].
The chromosomal gain of 3q26 may support the triage of women with positive primary screening. This biomarker might detect high-grade lesions, determining CIN2+ lesions, and the progression of LSIL. However, its use as a marker of regression and the variable expression in different age groups are some characteristics of this indicator that need further research [Citation156]. Similarly, LOH at chromosome 6 might be a good candidate marker for detecting progression of high-grade lesions to cervical cancer and for evaluating recurrent cervical lesions after treatment. Thus, it is also necessary to evaluate the sensitivity and specificity of these chromosomal alterations in additional meta-analyses, which may elucidate their utility as biomarkers of the disease.
3.6. Host DNA methylation
The methylation of host promoters has been widely studied in correlation to many cancer types, given the consequent downregulation of some genes involved in negative regulation of cell growth that grant advantages to transforming cells. Recent studies have demonstrated the association of host DNA methylation (m) with cervical neoplasia and cervical cancer; therefore, this approach is being increasingly adopted in biomarker research [Citation157]. Concerning the global mechanism, several reports revealed that the oncoproteins E6/E7 are able to induce the overexpression of DNA methyltransferase genes as DNMT1, DNMT3A, DNMT3B, allowing them to increase the overall methylation pattern when compared to a healthy condition [Citation98,Citation158].
By using massive deep-sequencing approaches, several genes have been observed to be methylated throughout the transformation from CIN to cervical cancers; among these, CADM1, EPB41L3, FAM19A4, MAL, miR-124, PAX1, and SOX1 have shown to be prominently elevated in high-grade CINs [Citation159]. Notably, the methylation of cell adhesion molecule 1 (CADM1) and T-lymphocyte maturation associated protein (MAL) promoters have explicitly been correlated to CIN3. In fact, the analysis of their epigenetics pattern could distinguish CIN3+ lesions as efficiently as cytology in a triage test [Citation135,Citation160].
One Taiwanese hospital-based study, using TaqMan-based quantitative methylation-specific polymerase chain reaction (QMSP) technique, found that for CIN3+, PAX1m had 64% sensitivity and 91% specificity, while SOX1m had 71% sensitivity and 77% specificity. The research also revealed that hrHPV DNA testing had 89% sensitivity and 68% specificity, and Pap smear conferred the best performance with 91% sensitivity and 90% specificity for CIN3 + . Moreover, combined parallel testing of Pap smears and PAX1m level gave better specificity (84% vs. 66%; p < 0.0001 by chi-square test) and equivalent sensitivity (93% vs. 97%; p = 0.2450 by chi-square test) to the combination of Pap smears and hrHPV DNA testing [Citation160]. These findings led to the approval by the Taiwanese FDA of PAX1 methylation testing as an adjunct to cytology for cervical cancer screening in 2016 [Citation98,Citation161].
The pattern of FAM19A4m has been proposed together with miR-124-2 m for triage in hrHPV-infected women to analyze CIN and progression to cervical cancer in a methylation profile assay [Citation162,Citation163]. One study by Vink et al. demonstrated a positivity rate of over 98% in their evaluation of FAM19A4/miR124‐2 methylation analysis in an extensive analysis of cervical cancer [Citation164]. In 2016, Qiagen® launched the QIAsure test based on FAM19A4m and miR-124-2 m, which possess a sensitivity of 100% for cervical cancer in women with hrHPV positive results [Citation165]. In addition, the combined FAM19A4m and miR-124-2 m analysis have been proven to be particularly efficient in identifying CIN3+ women in HPV-positive lavage and brush self-samples [Citation166]. Hence, it has been proved that the methylation pattern increases with CIN grade, showing significantly higher methylation of host genes in CIN3 if compared to CIN2 and global methylation in invasive cervical cancer. This evidence confirms the possibility of using the methylation pattern of certain genes in screening and triage tests as a potential progressive biomarker for severe CIN, in other words, for late stages lesions [Citation162,Citation167,Citation168]. Regarding the performance of host DNA methylation markers in low-grade intraepithelial lesions, Bu et al. recently determined that FAM19A4m had a positive correlation with the grading of cytology. They observed that the methylation rate was 10.61% for no CIN, 35.48% for LSIL, 56.14% for HSIL, and 93.44% for cervical cancer [Citation169]. Similarly, Dankai et al. reported that when the methylation status of CADM1, FAM19A4, and MAL is compared in different lesions, they all increase in HSIL. In fact, FAM19A4m was better differentiating ASCUS+ from NILM/ASCUS/LSIL, while CADM1 was able to distinguish HSIL+ from negative/LSIL [Citation170]. Thus, proving that these methylation markers may have a better potential for detecting high-grade lesions.
Host DNA methylation is now being considered as a valid alternative triage strategy to cytology for hrHPV DNA positive women. This is due to its molecular-basis assay, not dependant on morphology and subjective interpretation, as well as to its proven higher sensitivity for CIN2+ detection. Worthy of note, Verlaat et al. studied directly on self-samples an effective methylation pattern for three gene promoters, specific for the detection of CIN3 and ICC in hrHPV DNA positive self-collected samples; these genes were ASCL1, LHX8, and ST6GALNAC5 [Citation171]. The methylation analysis for these promoters and others can be performed directly on the self-sample used to detect hrHPV DNA, facilitating the triage test, and avoiding losing women in the follow-up steps [Citation171,Citation172].
Furthermore, Hsu et al.after having studied the different methylation status for various HPV types, proved that the methylation pattern of host genes seems to vary depending on the HPV genotype, suggesting that further investigations on these differences should follow [Citation98]. The discovery of new host DNA methylation genes that could be useful for biomarkers of cervical cancer keeps growing through the years. Xu et al. found, in their study, increased expression of the genes RAB3C, GABRA2, ZNF257, SLC5A8, and the decreased expression of GABRA2, ZNF257, and SLC5A8. Although they have high sensitivity and specificity, further studies are needed to evaluate their clinical significance [Citation157].
In conclusion, host DNA methylation may support the triage of women after hrHPV DNA testing and might be a good candidate for primary screening. Some instances of these methylated genes are FAM19A4, MAL, CADM1, and PAX1, which have been widely studied, but further research is needed to validate their utility. Nowadays, a combination of host promoter methylation patterns is available to be coupled to hrHPV genotyping for a solid molecular-base triage strategy, assuring a good sensitivity for late-stage lesions.
3.7. MicroRNAs
MicroRNAs (miRNAs) are also alternative biomarkers that are involved in the pathogenesis of cervical cancer by posttranscriptional regulation of gene expression. They are small RNA molecules of 20–23 nucleotides that do not encode proteins [Citation173]. Researchers have detected a lot of miRNAs overexpressed or with decreased expression in association with disease, among these, mi-R-9, miR-21, miR-127, mi-R155, miR-199a have been discovered to increase, and miR-143, miR214, miR-218, miR-125b, miR-375, miR-34a to decrease in cervical tumors [Citation31,Citation39]. Specifically, miR-21, miR-27a, miR-34, miR-34a, and miR-196a showed to be particularly highly expressed in squamous cell carcinoma of the cervix [Citation174]. More investigations about miRNAs testing have been done, and recent research has confirmed that miR-29a downregulation is correlated to cervical cancer progression. This has been described to be valid also for the downregulation of miR-34a, miR-125, and miR-375, which seems to be increasingly dysregulated when passing from CIN1 to ICC, suggesting a hypothetical role as progression markers [Citation174]. These miRNAs are also indicated for testing in larger populations to be included in the screening process of the disease. Another study, using the sRNA‐Seq technique in HPV‐positive self‐samples, confirmed their deregulated expression to be associated with CIN3 and cervical cancer development and concluded that this method could become a novel triage strategy for the disease [Citation175].
The evaluation of the utility of miRNAs in low-grade lesions is limited. Recent research by Ye et al. determined that miR-29a has a sensitivity of 92.6, positive predictive value of 46.2%, negative predictive value of 98.3%, and specificity 80.7% for detecting missed high-grade lesions in LSIL/CIN1 diagnosed patients [Citation176]. Therefore, demonstrating that this biomarker may be a good candidate for triage in cervical cancer programs. Furthermore, Okoye et al. found that when miRNAs are analyzed in both serum and cervical samples, they may have a better association with malignancy, and therefore, can distinguish abnormal cervical lesions. They reported an increase of miR-21, miR-146a, miR-155, miR-182, and miR-200 c, and a decrease of miR-145 in serum, which were associated with cervical lesions [Citation177]. Thus, proving the usefulness of miRNAs and proposing a new method for detecting these markers in serum samples.
Similarly, the upregulation of three miRNAs, miR-9, miR-21, and miR-155, has been relevantly pointed out as diagnostic tools. miR-21 is commonly found in many cancers, both from solid and leukemic samples. It is one of the most expressed miRNAs in mammal tissues, and its expression seems to downregulate PDCD4 tumor suppressor [Citation178]. On the other hand, miR-9 enhances cell motility promoting an eventual metastasis, while miR-155 supports the proliferation of the cells by regulating LKB1 expression [Citation179]. Indeed, miR-21 is associated with CIN and cervical cancer. Moreover, Park et al. found that the overexpression of miR1-155 was particularly linked to the elevated risk of cervical cancer development in HPV E6/E7 mRNA positive samples [Citation180]. Overall, the upregulation or downregulation of some miRNAs appears to be potentially reliable biomarkers, even from a progression perspective. These markers might be used to triage women after the primary screening with hrHPV DNA testing, particularly for ASCUS, LSIL, and HSIL cervical lesions. Further studies should uncover their exact cellular mechanisms and diagnostic value to permit their adoption in prevention tests.
Altogether, these viral and cellular biomarkers (summarized in ) have demonstrated being good candidates for cervical cancer screening and triage. They can predict the outcome of hrHPV infection in either progression to disease or regression to normal, and particularly, have shown to increase specificity and sensitivity towards HSIL and specific cervical intraepithelial lesions (CIN) . Therefore, their use within the screening programs might improve the diagnosis of precancerous stages of the disease. In combination with self-sampling, they could become essential diagnostic tools in low- and middle-income countries, which should be studied in further research.
Figure 5. Viral and cellular biomarkers for detecting specific stages of cervical intraepithelial lesions after a hrHPV infection. After hrHPV infection, the cervical epithelia infected starts to proliferate, finally getting transformed to cervical intraepithelial neoplasia (CIN) 1, 2, and 3. Then from CIN3, it progresses to cervical cancer. According to our review, several viral and cellular biomarkers may be useful for detecting different stages of CIN. hrHPV-DNA primary test is the best test so far to apply in population-based screening. Then in CIN1, besides the hrHPV DNA testing we can also use genotyping; for CIN2: TOP2A/MCM2, p16/Ki-67 staining, 3q gain in the chromosome, Viral load, hrHPV transcriptional status (E6/E7 mRNA), and E4 protein, with the latter being able to stratify the heterogenous CIN2 group in safe lesions (E4 positive) and clinically significant lesions (E4 negative); and for CIN3: Host DNA methylation(m), miRNAs, hrHPV genotyping, and Viral DNA methylation (m)
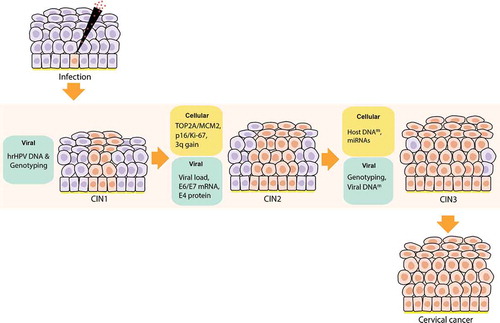
Table 1. Existing and candidate biomarkers for cervical cancer screening and triage
4. Conclusion
hrHPV infections can either progress to disease or regress to normal, and conventional methods such as cytology and the novel hrHPV DNA testing are unable to distinguish these changes. In addition, profiling the risk of cervical cancer means a prompt diagnosis, where hrHPV-infected women are identified with an infection or low-risk lesions in the cervix. The disease has become concentrated in low and middle-income countries, where diagnostic and preventive measures are limited. Therefore, the attempt to predict the progression of hrHPV infection has driven the search and discovery for new testing methods that allow the distinction of disease before it advances to malignancy. In the past decades, biomarkers have been studied and validated as indicators of hrHPV infection and the development of cancer. Although their implementation in the diagnosis flowchart worldwide seems delayed, some countries are taking the first steps in the use of these significant testing markers.
Existing biomarkers with potential for cervical cancer triage and screening include viral markers such as hrHPV genotyping, load, transcriptional status, E4 protein, and HPV methylation, and additional cellular markers such as p16/Ki-67, TOP2A/MCM2, chromosomal gains, miRNAs, and host methylation. All of them hold a great promise: hrHPV genotyping could help in stratifying the risk of women with abnormal low-grade lesions (ASCUS/LSIL) and manage the clinical decision, as it is known that the risk of disease progression is genotype-dependent; E6/E7 mRNA correlates with high-grade lesions, and E4 detection protein might be used to categorize CIN2+ lesions; the p16/Ki-67 dual staining and miRNAs are also remarkably associated with high-grade cervical lesions. Additionally, methylation patterns have been observed in both viral and cellular DNA, and they are highly linked to the generation of precancerous lesions and cervical cancer. In general, the evaluation of these biomarkers suggests that they exhibit a strong correlation with infection, specific cervical intraepithelial lesions, progression to disease, or regression to normal. Thus, these biomarkers may be useful in different stages of cervical cancer screening and management. For instance, hrHPV genotyping, transcriptional status, p16/Ki-67 immunostaining, and quantification of host DNA methylation seem to be useful markers for primary screening of the disease; on the other hand, viral/host DNA methylation, miRNAs, 3q26 chromosomal gain, viral load, E4 protein, and TOP2A/MCM2 may have a noteworthy performance in the triage of women after primary screening. Further research and meta-analyses are needed to have more conclusive results about these observations and their limitations, with additional analysis that clarify their utility in patients diagnosed with abnormal cytology such as ASCUS and LSIL. Similarly, novel approaches for biomarkers discovery include the use of proteomics analysis and the study of the vaginal microbiome. Further viral and cellular proteins might be excellent candidate indicators of disease, e.g., HPV L1 protein, Claudin-1, VCP, and E-cadherin, which have promising results in research [Citation190–194]. Alternatively, the vaginal microbiome might be used to distinguish progression vs. regression of disease [Citation195–197]. The overall results from existing and candidate biomarkers evidence their utility in profiling the risk for disease. Therefore, they should be considered for research, further validations, and future applications in screening programs.
Preventing cervical cancer by the timely detection of hrHPV infections and lesions through biomarkers testing is very effective, but not enough. There is also a need for increasing the involvement of women in screening programs, particularly in developing countries. The use of self-sampling may be the solution to this problem. It can provide comfort, privacy, and an easy-of-use technique to hard-to-reach women, which may increase their participation in the programs. However, incorrect collection of samples may result in low sensitivity and specificity for testing. Therefore, a robust and straightforward collection method is needed for further application strategies. The consideration of this collection method also requires the validation of biomarkers under these conditions. Consequently, the combination of biomarkers testing and self-sampling for detecting hrHPV infections might assist the diagnosis and prevention of the disease, and thus, increment the engagement of women to the programs. One instance of this strategy is the test careHPV (Qiagen®), which is based on the analysis of hrHPV DNA and self-sampling for the detection of hrHPV-infected women in low-resource settings [Citation49–52].
The recent progress of the Next Generation Sequencing (NGS) field will revolutionize the molecular diagnosis of virus-related tumors like cervical cancer. Population-based screening programs need to move towards a more personalized approach, and NGS is driving this change by enabling gene expression profiling, detection of epigenetic changes as well as a wide range of molecular analyses. So far, NGS and ‘omics’ studies have had a significant impact on our understanding of the etiology of HPV-driven cancers. The integration of all these new insights into clinical practice will ultimately lead to better choices of potential biomarkers and more effective personalized treatment options, having a positive impact on both screening programs and the healthcare systems worldwide. It will give clinicians the needed support in decision making during diagnosis, and their application will result in better cost-effectiveness of health policies for cervical cancer prevention and the well-being of women.
5. Expert opinion
The implementation of biomarkers for cervical cancer screening may improve the timely diagnosis of the disease and detect it during an early stage when the high-risk Human papillomaviruses (hrHPVs) have only been able to generate a productive infection and have developed cervical intraepithelial (CIN) lesions. The results of recent research have demonstrated that viral and cellular biomarkers such as hrHPV genotyping, hrHPV transcriptional status, p16/Ki-67 dual staining, and host DNA methylation can be useful in clinical practice, but further research is needed to confirm these findings. Despite the fact that low-resource settings have also implemented screening and prevention methods, their high prevalence of the disease exhibits the limited application and reduced involvement of women in the programs. Therefore, these particular regions need simpler, easier, and high-quality methods to improve their current strategies. New collecting methods, such as the use of self-samples, seem to be a helpful tool in improving the diagnosis rate of the disease. Advances in biomarkers research are still needed due to lack of homogeneity in their discovery and validation, affecting study designs and the production of high standard meta-analyses. Therefore, in order to develop these new screening methods, we should focus on investigating the promising biomarkers based on standardized guidelines that allow easy validations and reproducibility of results, which may lead to the innovation of the current diagnosis flowchart of the disease and higher cost-effectiveness of the screening programs. A ground-breaking biomarker is the one that is able to detect specifically progression or regression of CIN lesions and possess high specificity and sensitivity for stages of the disease. Thus, it must detect hrHPV infections and low-grade intraepithelial lesions (LSIL) before they progress to high-grade intraepithelial lesions (HSIL), and from this to malignancy. The future in this research field depends on finding these biomarkers, confirming and validating their results, and implementing them in screening programs. Additional biomarkers based on proteomics analyses and the study of the vaginal microbiome represent promising areas for further studies. Eventually, biomarkers may be applied into the screening programs worldwide, as it is happening in Europe, and together with the use of self-sampling, they would facilitate taking clinical management decisions on time, avoiding patient anxiety, over referral to colposcopy, and unnecessary treatments in women, especially in developing countries.
Article highlights
Novel viral and cellular biomarkers may improve the timely diagnosis of hrHPV infection and cervical lesions before they progress to malignancy or regress to normal.
Viral indicators such as hrHPV genotyping, load, transcriptional status, and E4 protein, as well as cellular indicators such as p16/Ki-67, TOP2A/MCM2, chromosomal gains, and miRNAs, might be good predictors of hrHPV risk status.
Collection methods based on self-sampling might increase the disease’s detection rate, and combined with biomarkers testing, may improve the screening programs for cervical cancer worldwide, especially in low-resource settings.
Declaration of interest
The authors have no other relevant affiliations or financial involvement with any organization or entity with a financial interest in or financial conflict with the subject matter or materials discussed in the manuscript apart from those disclosed.
Reviewer disclosures
Peer reviewers on this manuscript have no relevant financial or other relationships to disclose.
Supplemental Material
Download MS Word (15.2 KB)Acknowledgments
The work was supported by a research grant obtained from the Ruby and Rose Foundation. MAM was further supported by a scholarship from Secretaría Nacional de Ciencia, Tecnología e Innovación de Panamá (SENACYT, #270-2019-136). MCQ, a student from Universitat de Barcelona, was further supported by the Erasmus + scholarship from Servicio Español para la Internalización de la Educación, and by the MOBINT-MIF scholarship from Generalitat de Catalunya (#BDNS 467025). All authors declare that they have no conflicting or dual interests.
Supplementary material
Supplemental data for this article can be accessed here.
Additional information
Funding
References
- Ferlay J, e.a. global cancer observatory: cancer today. Lyon, France: international agency for research on cancer. 2018 [cited 2020 January 1]; Available from: https://gco.iarc.fr/today
- Doorbar J, Quint W, Banks L, et al. The biology and life-cycle of human papillomaviruses. Vaccine. 2012;30(Suppl 5):F55–70. DOI:10.1016/j.vaccine.2012.06.083.
- Heideman DAM, Hesselink AT, Berkhof J, et al. Clinical validation of the cobas 4800 HPV test for cervical screening purposes. J Clin Microbiol. 2011;49(11):3983–3985. DOI:10.1128/JCM.05552-11.
- Ghosh T, VandeHaar MA, Rivera M, et al. High-risk HPV genotype distribution in HPV co-test specimens: study of a predominantly Midwestern population. J Am Soc Cytopathol. 2018;7(2):99–105. DOI:10.1016/j.jasc.2017.09.007
- Molijn A, Jenkins D, Chen W, et al. The complex relationship between human papillomavirus and cervical adenocarcinoma. Int J Cancer. 2016;138(2):409–416. DOI:10.1002/ijc.29722.
- Doorbar J. The papillomavirus life cycle. J Clin Virol. 2005;32:7–15. (1386–6532 (Print)). DOI:10.1016/j.jcv.2004.12.006.
- Griffin H, Soneji Y, Van Baars R, et al. Stratification of HPV-induced cervical pathology using the virally encoded molecular marker E4 in combination with p16 or MCM. Mod Pathol. 2015;28(7): 977–993. DOI:10.1038/modpathol.2015.52. .
- Schiller JT, Day PM, Kines RC. Current understanding of the mechanism of HPV infection. Gynecol Oncol. 2010;118(1 Suppl):S12–S17.
- Horvath CAJ, Boulet GA, Renoux VM, et al. Mechanisms of cell entry by human papillomaviruses: an overview. Virol J. 2010;7(1):11–11. DOI:10.1186/1743-422X-7-11.
- Tsakogiannis D, Gartzonika C, Levidiotou-Stefanou S, et al. Molecular approaches for HPV genotyping and HPV-DNA physical status. Expert Rev Mol Med. 2017;19:e1.
- Stevenson A, Kavanagh K, Pan J, et al. Risk stratification of cervical disease using detection of human papillomavirus (HPV) E4 protein and cellular MCM protein in clinical liquid based cytology samples. J Clin Virol. 2018;108:19–25.
- Middleton K, Peh W, Southern S, et al. Organization of human papillomavirus productive cycle during neoplastic progression provides a basis for selection of diagnostic markers. J Virol. 2003;77(19):10186–10201. DOI:10.1128/JVI.77.19.10186-10201.2003.
- Mello V, S.R. cancer, cervical intraepithelial neoplasia (CIN). Treasure Island (FL): StatPearls Publishing; 2020 Jan- 2019 [cited 2020 January 2]; Available from: https://www.ncbi.nlm.nih.gov/books/NBK544371/
- Ebisch RMF, Ketelaars PJW, van der Sanden WMH, et al. Screening for persistent high-risk HPV infections may be a valuable screening method for young women; a retrospective cohort study. Plos One. 2018;13(10):e0206219. DOI:10.1371/journal.pone.0206219.
- Liu Y, Zhang C, Gao W, et al. Genome-wide profiling of the human papillomavirus DNA integration in cervical intraepithelial neoplasia and normal cervical epithelium by HPV capture technology. Sci Rep. 2016;6(1):35427. DOI:10.1038/srep35427.
- Melsheimer P, Vinokurova S, Wentzensen N, et al. DNA aneuploidy and integration of human papillomavirus type 16 E6/E7 oncogenes in intraepithelial neoplasia and invasive squamous cell carcinoma of the cervix uteri. Clin Cancer Res. 2004;10(9):3059. DOI:10.1158/1078-0432.CCR-03-0565.
- Van Tine BA, Kappes JC, Banerjee NS, et al. Clonal selection for transcriptionally active viral oncogenes during progression to cancer. J Virol. 2004;78(20):11172–11186. DOI:10.1128/JVI.78.20.11172-11186.2004.
- Pett M, Coleman N. Integration of high-risk human papillomavirus: a key event in cervical carcinogenesis? J Pathol. 2007;212(4):356–367.
- Wilting SM, Steenbergen RDM, Tijssen M, et al. Chromosomal signatures of a subset of high-grade premalignant cervical lesions closely resemble invasive carcinomas. Cancer Res. 2009;69(2):647. DOI:10.1158/0008-5472.CAN-08-2478.
- Huh W, Einstein MH, Herzog TJ, et al. What is the role of HPV typing in the United States now and in the next five years in a vaccinated population? Gynecol Oncol. 2010;117(3):481–485. DOI:10.1016/j.ygyno.2010.01.037.
- Dillner J, Rebolj M, Birembaut P, et al. Long term predictive values of cytology and human papillomavirus testing in cervical cancer screening: joint European cohort study. BMJ. 2008;337(oct13 1):a1754–a1754. DOI:10.1136/bmj.a1754.
- Cibas ES. Chapter 1 - cervical and vaginal cytology. In: Cibas ES, Ducatman BS, editors. Cytology. Third ed. Philadelphia: W.B. Saunders; 2009. p. 1–63.
- Palmer T, Wallace L, Pollock KG, et al. Prevalence of cervical disease at age 20 after immunisation with bivalent HPV vaccine at age 12-13 in Scotland: retrospective population study. BMJ. 2019;365:l1161.
- Felix JC, Wright TC, Amezcua CA. CHAPTER 36 - Cervix. Weidner N, Cote RJ, et al., editors. Modern Surgical Pathology (Second Edition), W.B. Saunders, 2009, p. 1263–1294, ISBN 9781416039662. http://www.sciencedirect.com/science/article/pii/B9781416039662000369 DOI 10.1016/B978–1–4160–3966–2.00036–9
- Ketelaars PJW, Bosgraaf RP, Siebers AG, et al. High-risk human papillomavirus detection in self-sampling compared to physician-taken smear in a responder population of the Dutch cervical screening: results of the VERA study. Prev Med. 2017;101:96–101.
- Polman NJ, Ebisch RM, Heideman DA, et al. Performance of human papillomavirus testing on self-collected versus clinician-collected samples for the detection of cervical intraepithelial neoplasia of grade 2 or worse: a randomised, paired screen-positive, non-inferiority trial. Lancet Oncol. 2019;20(2):229–238.
- Schmeink CE, Bekkers RL, Massuger LF, et al. The potential role of self-sampling for high-risk human papillomavirus detection in cervical cancer screening. Rev Med Virol. 2011;21(3):139–153.
- Winer RL, Lin J, Tiro JA, et al. Effect of mailed human papillomavirus test kits vs usual care reminders on cervical cancer screening uptake, precancer detection, and treatment: a randomized clinical trial. JAMA Network Open. 2019;2(11):e1914729–e1914729.
- Madzima T, Vahabi M, Lofters A. Emerging role of HPV self-sampling in cervical cancer screening for hard-to-reach women. Can Family Physician. 2017;63:597–601.
- Asciutto KC, Ernstson A, Foorslund O, et al. Self-sampling with HPV mRNA analyses from vagina and urine compared with cervical samples. J Clin Virol. 2018;101:69–73.
- Sahasrabuddhe VV, Luhn P, Wentzensen N. Human papillomavirus and cervical cancer: biomarkers for improved prevention efforts. Future Microbiol. 2011;6(9):1083–1098.
- Aitken CA, van Agt HME, Siebers AG, et al. Introduction of primary screening using high-risk HPV DNA detection in the Dutch cervical cancer screening programme: a population-based cohort study. BMC Med. 2019;17(1):228–228. DOI:10.1186/s12916-019-1460-0.
- Maver PJ, Poljak M, Primary HP. V-based cervical cancer screening in Europe: implementation status, challenges, and future plans. Clin Microbiol Infect. 2020;26(5):579–583.
- Frayle H, Gori S, Rizzi M, et al. HPV testing for cervical cancer screening: technical improvement of laboratory logistics and good clinical performance of the cobas 6800 in comparison to the 4800 system. BMC Women’s Health. 2019;19(1):47. DOI:10.1186/s12905-019-0743-0.
- Lindroth Y, Borgfeldt C, Thorn G, et al. Population-based primary HPV mRNA cervical screening compared with cytology screening. Prev Med. 2019;124:61–66.
- Authority DH, Cancer Screening Fact Sheet, Denmark – cervix – 2017. 2017.
- Sørbye SW, Fismen S, Gutteberg TJ, et al. Primary cervical cancer screening with an HPV mRNA test: a prospective cohort study. BMJ Open. 2016;6(8):e011981. DOI:10.1136/bmjopen-2016-011981.
- Katki HA, Wacholder S, Solomon D, et al. Risk estimation for the next generation of prevention programmes for cervical cancer. Lancet Oncol. 2009;10(11):1022–1023. DOI:10.1016/S1470-2045(09)70253-0.
- Tornesello ML, Buonaguro L, Giorgi-Rossi P, et al. Viral and cellular biomarkers in the diagnosis of cervical intraepithelial neoplasia and cancer. Biomed Res Int. 2013;2013:519619–519619.
- Wentzensen N, Schiffman M, Palmer T, et al. Triage of HPV positive women in cervical cancer screening. J Clin Virol. 2016;76:S49–S55.
- Godoy-Vitorino F, Romaguera J, Zhao C, et al. Cervicovaginal fungi and bacteria associated with cervical intraepithelial neoplasia and high-risk human papillomavirus infections in a hispanic population. Front Microbiol. 2018;9:2533.
- Hudelist G, Manavi M, Pischinger KID, et al. Physical state and expression of HPV DNA in benign and dysplastic cervical tissue: different levels of viral integration are correlated with lesion grade. Gynecol Oncol. 2004;92(3):873–880. DOI:10.1016/j.ygyno.2003.11.035.
- Wei WF, Su GD, Wu LF, et al. [Study of integrated state of HPV-16 infection in cervical cancer and precancerous tissues]. 2015;35(1):47–50.
- Group TA-LTSA. Results of a randomized trial on the management of cytology interpretations of atypical squamous cells of undetermined significance. Am J Obstet Gynecol. 2003;188(6):1383–1392.
- Wright TC, Massad LS, Dunton CJ, et al. 2006 consensus guidelines for the management of women with abnormal cervical cancer screening tests. Am J Obstet Gynecol. 2007;197(4):346–355. DOI:10.1016/j.ajog.2007.07.047.
- Siebers AG, Arbyn M, Melchers WJG, et al. Effectiveness of two strategies to follow-up ASC-US and LSIL screening results in The Netherlands using repeat cytology with or without additional hrHPV testing: a retrospective cohort study. Cancer Causes Control. 2014;25(9):1141–1149. (1573–7225 (Electronic)). DOI:10.1007/s10552-014-0414-2.
- El-Zein M, Richardson L, Franco EL. Cervical cancer screening of HPV vaccinated populations: cytology, molecular testing, both or none. J Clin Virol. 2016;76:S62–S68.
- Polman NJ, Snijders PJF, Kenter GG, et al. HPV-based cervical screening: rationale, expectations and future perspectives of the new Dutch screening programme. Prev Med. 2019;119:108–117.
- Arbyn M, Smith SB, Temin S, et al. Detecting cervical precancer and reaching underscreened women by using HPV testing on self samples: updated meta-analyses. BMJ. 2018;363:k4823.
- Kang L-N, Jeronimo J, Qiao Y-L, et al. Optimal positive cutoff points for careHPV testing of clinician- and self-collected specimens in primary cervical cancer screening: an analysis from Rural China. J Clin Microbiol. 2014;52(6):1954. DOI:10.1128/JCM.03432-13.
- Zhao FH, Chen JF, Gao XH, et al. [Effectiveness and health economic analysis of strategies on cervical cancer screening and early diagnosis and treatment]. Zhonghua Zhong Liu Za Zhi. 2012;34(8):632–636.
- Levin CE, Sellors J, Shi J-F, et al. Cost-effectiveness analysis of cervical cancer prevention based on a rapid human papillomavirus screening test in a high-risk region of China. Int J Cancer. 2010;127(6): 1404–1411. DOI:10.1002/ijc.25150. .
- Kocken M, Uijterwaal MH, de Vries ALM, et al. High-risk human papillomavirus testing versus cytology in predicting post-treatment disease in women treated for high-grade cervical disease: a systematic review and meta-analysis. Gynecol Oncol. 2012;125(2):500–507. DOI:10.1016/j.ygyno.2012.01.015.
- Vinokurova S, Wentzensen N, Kraus I, et al. Type-dependent integration frequency of human papillomavirus genomes in cervical lesions. Cancer Res. 2008;68(1):307. DOI:10.1158/0008-5472.CAN-07-2754.
- de Sanjose S, Quint WG, Alemany L, et al. Human papillomavirus genotype attribution in invasive cervical cancer: a retrospective cross-sectional worldwide study. Lancet Oncol. 2010;11(11):1048–1056. DOI:10.1016/S1470-2045(10)70230-8.
- Gustavsson I, Sanner K, Lindell M, et al. Type-specific detection of high-risk human papillomavirus (HPV) in self-sampled cervicovaginal cells applied to FTA elute cartridge. J Clin Virol. 2011;51(4):255–258. DOI:10.1016/j.jcv.2011.05.006.
- Ebisch RMF, de Kuyper-de Ridder GM, Bosgraaf RP, et al. The clinical value of HPV genotyping in triage of women with high-risk-HPV-positive self-samples. Int J Cancer. 2016;139(3):691–699. DOI:10.1002/ijc.30090.
- Wright TC, Stoler MH, Behrens CM, et al. The ATHENA human papillomavirus study: design, methods, and baseline results. Am J Obstet Gynecol. 2012;206(1):46.e1-46.e11. DOI:10.1016/j.ajog.2011.07.024.
- Castle PE, Fetterman B, Poitras N, et al. Five-year experience of human papillomavirus DNA and papanicolaou test cotesting. Obstetrics Gynecol. 2009;113(3):595–600. DOI:10.1097/AOG.0b013e3181996ffa.
- Dunne EF, Unger ER, Sternberg M, et al. Prevalence of HPV infection among females in the United States. JAMA. 2007;297(8):813–819. DOI:10.1001/jama.297.8.813.
- Xi LF, Hughes JP, Castle PE, et al. Viral load in the natural history of human papillomavirus type 16 infection: a nested case-control study. J Infect Dis. 2011;203(10):1425–1433. DOI:10.1093/infdis/jir049.
- Kim J, Kim BK, Jeon D, et al. Type-specific viral load and physical state of HPV type 16, 18, and 58 as diagnostic biomarkers for high-grade squamous intraepithelial lesions or cervical cancer. Cancer Res Treat. 2020;52(2): 396–405. DOI:10.4143/crt.2019.152. .
- Constandinou-Williams C, Collins SI, Roberts S, et al. Is human papillomavirus viral load a clinically useful predictive marker? A longitudinal study. Cancer Epidemiology Biomarkers & Prevention. 2010;19(3):832. DOI:10.1158/1055-9965.EPI-09-0838.
- Berggrund M, Gustavsson I, Aarnio R, et al. HPV viral load in self-collected vaginal fluid samples as predictor for presence of cervical intraepithelial neoplasia. Virol J. 2019;16(1):146–146. DOI:10.1186/s12985-019-1253-2.
- Halec G, Dondog B, Pawlita M, et al. Concordance of HPV load and HPV mRNA for 16 carcinogenic/possibly carcinogenic HPV types in paired smear/tissue cervical cancer specimens. Arch Virol. 2017;162(11):3313–3327. DOI:10.1007/s00705-017-3452-8.
- Cao M, Wang Y, Wang D, et al. Increased high-risk human papillomavirus viral load is associated with immunosuppressed microenvironment and predicts a worse long-term survival in cervical cancer patients. Am J Clin Pathol. 2019;153(4):502–512. DOI:10.1093/ajcp/aqz186.
- Wu Y, Chen Y, Li L, et al. Associations of high-risk HPV types and viral load with cervical cancer in China. J Clin Virol. 2006;35(3):264–269. DOI:10.1016/j.jcv.2005.07.011.
- Lee SJ, Kim WY, Shim S-H, et al. Semi-quantitative HPV viral load in patients with ASC-US cytology: viral load correlates strongly with the presence of CIN but only weakly with its severity. Cytopathology. 2015;26(1):19–25. DOI:10.1111/cyt.12146.
- Carcopino X, Henry M, Mancini J, et al. Significance of HPV 16 and 18 viral load quantitation in women referred for colposcopy. J Med Virol. 2012;84(2):306–313. DOI:10.1002/jmv.23190.
- Schmitt M, Depuydt C, Benoy I, et al. Multiple human papillomavirus infections with high viral loads are associated with cervical lesions but do not differentiate grades of cervical abnormalities. J Clin Microbiol. 2013;51(5):1458–1464. DOI:10.1128/JCM.00087-13.
- Boulet GAV, Benoy IH, Depuydt CE, et al. Human papillomavirus 16 load and E2/E6 ratio in HPV16-positive women: biomarkers for cervical intraepithelial neoplasia ≥2 in a liquid-based cytology setting? Cancer Epidemiology Biomarkers & Prevention. 2009;18(11):2992. DOI:10.1158/1055-9965.EPI-09-0025.
- van den Heuvel CNAM, Loopik DL, Ebisch RMF, et al. RNA-based high-risk HPV genotyping and identification of high-risk HPV transcriptional activity in cervical tissues. Mod Pathol. 2020;33(4):748–757. DOI:10.1038/s41379-019-0369-7.
- Tsakogiannis D, Kyriakopoulou Z, Ruether IGA, et al. Determination of human papillomavirus 16 physical status through E1/E6 and E2/E6 ratio analysis. J Med Microbiol. 2014;63(12):1716–1723. DOI:10.1099/jmm.0.076810-0.
- Galarowicz B, Jach R, Kidzierska J, et al. The role of mRNA E6/E7 HPV high oncogenic risk expression in colposcopy of cervical intraepithelial neoplasia (CIN). Przegl Lek. 2012 ;69(9):651–657.
- Fontecha N, Basaras M, Hernáez S, et al. Assessment of human papillomavirus E6/E7 oncogene expression as cervical disease biomarker. BMC Cancer. 2016;16(1):852–852. DOI:10.1186/s12885-016-2885-x.
- Benevolo M, Vocaturo A, Caraceni D, et al. Sensitivity, specificity, and clinical value of human papillomavirus (HPV) E6/E7 mRNA assay as a triage test for cervical cytology and HPV DNA Test. J Clin Microbiol. 2011;49(7):2643. DOI:10.1128/JCM.02570-10.
- Benevolo M, Terrenato I, Mottolese M, et al. Diagnostic and prognostic validity of the human papillomavirus E6/E7 mRNA test in cervical cytological samples of HC2-positive patients. Cancer Causes Control. 2011;22(6):869–875. DOI:10.1007/s10552-011-9757-0.
- Duvlis S, Popovska-Jankovic K, Arsova ZS, et al. HPV E6/E7 mRNA versus HPV DNA biomarker in cervical cancer screening of a group of Macedonian women. J Med Virol. 2015;87(9):1578–1586. DOI:10.1002/jmv.24199.
- Dabeski D, Duvlis S, Basheska N, et al. Comparison between HPV DNA testing and HPV E6/E7 MRNA testing in women with squamous cell abnormalities of the uterine cervix. Pril (Makedon Akad Nauk Umet Odd Med Nauki). 2019;40(1):51–58.
- Cui M, Chan N, Liu M, et al. Clinical performance of roche cobas 4800 HPV Test. J Clin Microbiol. 2014;52(6):2210–2211. DOI:10.1128/JCM.00883-14.
- Ge Y, Christensen P, Luna E, et al. Performance of Aptima and Cobas HPV testing platforms in detecting high-grade cervical dysplasia and cancer. Cancer Cytopathol. 2017;125(8):652–657. DOI:10.1002/cncy.21875.
- Macedo ACL, Gonçalves JCN, Bavaresco DV, et al. Accuracy of mRNA HPV tests for triage of precursor lesions and cervical cancer: a systematic review and meta-analysis. J Oncol. 2019;2019:6935030. DOI:10.1155/2019/6935030
- Stoler MH, Wright TC, Cuzick J, et al. APTIMA HPV assay performance in women with atypical squamous cells of undetermined significance cytology results. Am J Obstet Gynecol. 2013;208(2):144.e1-144.e8. DOI:10.1016/j.ajog.2012.12.003.
- Bruno MT, Ferrara M, Fava V, et al. A prospective study of women with ASCUS or LSIL pap smears at baseline and HPV E6/E7 mRNA positive: a 3-year follow-up. Epidemiol Infect. 2018;146(5):612–618. DOI:10.1017/S0950268818000250.
- Rijkaart DC, Heideman DAM, Coupe VMH, et al. High-risk human papillomavirus (hrHPV) E6/E7 mRNA testing by PreTect HPV-Proofer for detection of cervical high-grade intraepithelial neoplasia and cancer among hrHPV DNA-positive women with normal cytology. J Clin Microbiol. 2012;50(7):2390–2396. DOI:10.1128/JCM.06587-11.
- Ting J, Mugo N, Kwatampora J, et al. High-risk human papillomavirus messenger RNA testing in physician- and self-collected specimens for cervical lesion detection in high-risk women, Kenya. Sex Transm Dis. 2013;40(7):7. DOI:10.1097/OLQ.0b013e31828e5a91.
- Nieves L, Enerson CL, Belinson S, et al. Primary cervical cancer screening and triage using an mRNA human papillomavirus assay and visual inspection. Int J Gynecologic Cancer. 2013;23(3):513. DOI:10.1097/IGC.0b013e318280f3bc.
- Discacciati MG, da Silva ID, Villa LL, et al. Prognostic value of DNA and mRNA e6/e7 of human papillomavirus in the evolution of cervical intraepithelial neoplasia grade 2. Biomark Insights. 2014;9:15–22.
- Zummeren MV, Kremer WW, Leeman A, et al. HPV E4 expression and DNA hypermethylation of CADM1, MAL, and miR124-2 genes in cervical cancer and precursor lesions. Mod Pathol. 2018;31(12):1842–1850.
- Griffin H, Wu Z, Marnane R, et al. E4 antibodies facilitate detection and type-assignment of active HPV infection in cervical disease. PloS One. 2012;7(12):e49974–e49974. DOI:10.1371/journal.pone.0049974.
- Krawczyk E, Suprynowicz FA, Liu X, et al. Koilocytosis: a cooperative interaction between the human papillomavirus E5 and E6 oncoproteins. Am J Pathol. 2008;173(3):682–688. DOI:10.2353/ajpath.2008.080280.
- Alves de Sousa NL, Alves RR, Martins MR, et al. Cytopathic effects of human papillomavirus infection and the severity of cervical intraepithelial neoplasia: a frequency study. Diagn Cytopathol. 2012;40(10):871–875.
- Siebers AG, van der Linden H, Vedder JEM, et al. Presence of koilocytosis in low-grade smears of high-risk HPV-positive women is a negative predictor for cervical intraepithelial neoplasia grade 3 or more. Cytopathology. 2018;29(3):275–280. DOI:10.1111/cyt.12536.
- Vrdoljak-Mozetič D, Krašević M, Verša Ostojić D, et al. HPV16 genotype, p16/Ki-67 dual staining and koilocytic morphology as potential predictors of the clinical outcome for cervical low-grade squamous intraepithelial lesions. Cytopathology. 2015;26(1):10–18. DOI:10.1111/cyt.12121.
- Wentzensen N, Sun C, Ghosh A, et al. Methylation of HPV18, HPV31, and HPV45 genomes and cervical intraepithelial neoplasia grade 3. J Natl Cancer Inst. 2012;104(22):1738–1749. DOI:10.1093/jnci/djs425.
- Leeman A, Jenkins D, Marra E, et al. Grading immunohistochemical markers p16 INK4a and HPV E4 identifies productive and transforming lesions caused by low- and high-risk HPV within high-grade anal squamous intraepithelial lesions. Br J Dermatol. 2020;182(4):1026–1033. DOI:10.1111/bjd.18342.
- Leeman AA-O, Jenkins D, Pino M, et al. Expression of p16 and HPV E4 on biopsy samples and methylation of FAM19A4 and miR124-2 on cervical cytology samples in the classification of cervical squamous intraepithelial lesions. Cancer Med. 2020;9(7):2454–2461. (2045–7634 (Electronic)). DOI:10.1002/cam4.2855.
- Hsu Y-W, Huang R-L, Su P-H, et al. Genotype-specific methylation of HPV in cervical intraepithelial neoplasia. J Gynecol Oncol. 2017;28(4). DOI:10.3802/jgo.2017.28.e56.
- Vinokurova S, von Knebel Doeberitz M, Ramqvist T. Differential methylation of the HPV 16 upstream regulatory region during epithelial differentiation and neoplastic transformation. PloS One. 2011;6(9):e24451–e24451.
- Clarke MA, Wentzensen N, Mirabello L, et al. Human papillomavirus DNA methylation as a potential biomarker for cervical cancer. Cancer Epidemiology Biomarkers & Prevention. 2012;21(12):2125. DOI:10.1158/1055-9965.EPI-12-0905.
- Kalantari M, Osann K, Calleja-Macias IE, et al. Methylation of human papillomavirus 16, 18, 31, and 45 L2 and L1 genes and the cellular DAPK gene: considerations for use as biomarkers of the progression of cervical neoplasia. Virology. 2014;448:314–321.
- Amaro-Filho SM, Pereira Chaves CB, Felix SP, et al. HPV DNA methylation at the early promoter and E1/E2 integrity: a comparison between HPV16, HPV18 and HPV45 in cervical cancer. Papillomavirus Res. 2018;5:172–179.
- Clarke MA, Gradissimo A, Schiffman M, et al. Human papillomavirus DNA methylation as a biomarker for cervical precancer: consistency across 12 genotypes and potential impact on management of HPV-positive women. Clin Cancer Res off J Am Assoc Cancer Res. 2018;24(9):2194–2202. DOI:10.1158/1078-0432.CCR-17-3251.
- Woodman CB, Collins Si Fau - Young LS, Young LS. The natural history of cervical HPV infection: unresolved issues. Nature Reviews Cancer. 2007;7(1):11–22. DOI:10.1038/nrc2050. (1474-175X (Print))
- Nam EJ, Kim JW, Hong JW, et al. Expression of the p16 and Ki-67 in relation to the grade of cervical intraepithelial neoplasia and high-risk human papillomavirus infection. J Gynecol Oncol. 2008;19(3):162–168. DOI:10.3802/jgo.2008.19.3.162.
- Romagosa C, Simonetti S, López-Vicente L, et al. p16(Ink4a) overexpression in cancer: a tumor suppressor gene associated with senescence and high-grade tumors. Oncogene. 2011;30(18):2087–2097.
- Carozzi F, Gillio-Tos A, Confortini M, et al. Risk of high-grade cervical intraepithelial neoplasia during follow-up in HPV-positive women according to baseline p16-INK4A results: a prospective analysis of a nested substudy of the NTCC randomised controlled trial. Lancet Oncol. 2013;14(2):168–176. DOI:10.1016/S1470-2045(12)70529-6.
- Yang QC, Zhu Y, Liou H-B, et al. A cocktail of MCM2 and TOP2A, p16INK4a and Ki-67 as biomarkers for the improved diagnosis of cervical intraepithelial lesion. Pol J Pathol. 2013;64(1):21–27. DOI:10.5114/pjp.2013.34599.
- Zeng W-J, Li Y, Fei H-L, et al. The value of p16ink4a expression by fluorescence in situ hybridization in triage for high risk HPV positive in cervical cancer screening. Gynecol Oncol. 2011;120(1):84–88. DOI:10.1016/j.ygyno.2010.09.008.
- Mendaza S, Fernández-Irigoyen J, Santamaría E, et al. Absence of nuclear p16 is a diagnostic and independent prognostic biomarker in squamous cell carcinoma of the cervix. Int J Mol Sci. 2020;21(6): 2125. DOI:10.3390/ijms21062125. .
- Group R Improving consistency in the diagnosis of cervical pre-cancers: roche CINtec Histology test receives FDA clearance. 2017 April 5 [cited 2020 May 5]; Available from: https://www.roche.com/dam/jcr:37a72ad4-c338-4be9-b6d6-79147a9c0029/en/med-cor-2017-04-05-e.pdf
- Schmidt D, Bergeron C, Denton KJ, et al. p16/ki-67 dual-stain cytology in the triage of ASCUS and LSIL papanicolaou cytology. Cancer Cytopathol. 2011;119(3):158–166. DOI:10.1002/cncy.20140.
- Lin J, Albers AE, Qin J, et al. Prognostic significance of overexpressed p16INK4a in patients with cervical cancer: a meta-analysis. PloS One. 2014;9(9):e106384–e106384. DOI:10.1371/journal.pone.0106384.
- Milde-Langosch K, Bamberger A-M, Rieck G, et al. Overexpression of the p16 cell cycle inhibitor in breast cancer is associated with a more malignant phenotype. Breast Cancer Res Treat. 2001;67(1):61–70. DOI:10.1023/A:1010623308275.
- Dai CY, Furth EE, Mick R, et al. p16(INK4a) expression begins early in human colon neoplasia and correlates inversely with markers of cell proliferation. Gastroenterology. 2000;119(4):929–942. DOI:10.1053/gast.2000.17952.
- Guida M, Sanguedolce F, Bufo PA, et al. Aberrant DNA hypermethylation of hMLH-1 and CDKN2A/p16 genes in benign, premalignant and malignant endometrial lesions. Eur J Gynaecol Oncol. 2009;30(3):267–270.
- Romagosa C, Simonetti S, López-Vicente L, et al. p16Ink4a overexpression in cancer: a tumor suppressor gene associated with senescence and high-grade tumors. Oncogene. 2011;30(18):2087–2097.
- Schwartz B, Avivi-Green C Fau - Polak-Charcon S, Polak-Charcon S. Sodium butyrate induces retinoblastoma protein dephosphorylation, p16 expression and growth arrest of colon cancer cells. Mol Cell Biochem. 1998;188(1/2):21–30. (0300–8177 (Print)). DOI:10.1023/A:1006831330340.
- Inoue K, Fry EA. Aberrant expression of p16(INK4a) in human cancers - a new biomarker? Cancer Rep Rev. 2018;2(2). DOI:10.15761/CRR.1000145
- Suman S, Mishra A. An interaction network driven approach for identifying biomarkers for progressing cervical intraepithelial neoplasia. Sci Rep. 2018;8(1):12927.
- Dixon EP, King LM, Nelson R, et al. Characterization and clinical validation of MCM2 and TOP2A monoclonal antibodies in the BD ProEx™ C assay: an immunoassay which detects aberrant S-phase induction in cervical tissue. J Immunol Methods. 2017;442:35–41.
- Wang FL, Yang Y, Liu ZY, et al. Correlation between methylation of the p16 promoter and cervical cancer incidence. Eur Rev Med Pharmacol Sci. 2017;21(10):2351–2356.
- Jeong DH, Youm MY, Kim YN, et al. Promoter methylation of p16, DAPK, CDH1, and TIMP-3 genes in cervical cancer: correlation with clinicopathologic characteristics. Int J Gynecological Cancer. 2006;16(3):1234–1240. DOI:10.1136/ijgc-00009577-200605000-00043.
- Jha AK, NIKBAKHT M, JAIN V, et al. p16INK4a and p15INK4b gene promoter methylation in cervical cancer patients. Oncol Lett. 2012;3(6):1331–1335. DOI:10.3892/ol.2012.655.
- Carestiato FN, Amaro-Filho SM, Moreira MA, et al. Methylation of p16 ink4a promoter is independent of human papillomavirus DNA physical state: a comparison between cervical pre-neoplastic and neoplastic samples. Memórias Inst Oswaldo Cruz. 2019;114:e180456.
- Li RN, Li C-Y, Lee C-H, et al. Promoter methylation status of the tumor suppressor genes p16 and cadherin 1 in cervical intraepithelial neoplasia. Oncol Lett. 2017;13(6):4397–4401. DOI:10.3892/ol.2017.5975.
- Chakraborty C, Roychowdhury A, Samadder S, et al. Association of P16-RBSP3 inactivation with phosphorylated RB1 overexpression in basal–parabasal layers of normal cervix unchanged during CACX development. Biochem J. 2016;473(19):3221–3236. DOI:10.1042/BCJ20160323.
- Tsakogiannis D, Moschonas GD, Bella E, et al. Association of p16 (CDKN2A) polymorphisms with the development of HPV16-related precancerous lesions and cervical cancer in the Greek population. J Med Virol. 2018;90(5):965–971. DOI:10.1002/jmv.24996.
- Thakur N, Hussain S, Nasare V, et al. Association analysis of p16 (CDKN2A) and RB1 polymorphisms with susceptibility to cervical cancer in Indian population. Mol Biol Rep. 2012;39(1):407–414. DOI:10.1007/s11033-011-0752-z.
- van Zummeren M, Leeman A, Kremer WW, et al. Three-tiered score for Ki-67 and p16 ink4a improves accuracy and reproducibility of grading CIN lesions. J Clin Pathol. 2018;71(11):981–988. DOI:10.1136/jclinpath-2018-205271.
- Peres AL, Paz E Silva KM, de Araújo RFF, et al. Immunocytochemical study of TOP2A and Ki-67 in cervical smears from women under routine gynecological care. J Biomed Sci. 2016;23(1):42. DOI:10.1186/s12929-016-0258-z.
- Tjalma WAA. Diagnostic performance of dual-staining cytology for cervical cancer screening: a systematic literature review. Eur J Obstet Gynecol Reprod Biol. 2017;210:275–280.
- Reuschenbach M, Seiz M, Doeberitz CVK, et al. Evaluation of cervical cone biopsies for coexpression of p16INK4a and Ki-67 in epithelial cells. International Journal of Cancer. 2012;130(2):388–394. (1097–0215 (Electronic)). DOI:10.1002/ijc.26017.
- Gustinucci D, Rossi PG, Cesarini E, et al. Use of cytology, E6/E7 mRNA, and p16 INK4a–Ki-67 to define the management of human papillomavirus (HPV)–positive women in cervical cancer screening. Am J Clin Pathol. 2016;145(1):35–45. DOI:10.1093/ajcp/aqv019.
- Litjens R, Hopman AH, van de Vijiver KK, et al. Molecular biomarkers in cervical cancer diagnosis: a critical appraisal. Expert Opin Med Diagn. 2013;7(4):365–377.
- Peeters E, Wentzensen N, Bergeron C, et al. Meta-analysis of the accuracy of p16 or p16/Ki-67 immunocytochemistry versus HPV testing for the detection of CIN2+/CIN3+ in triage of women with minor abnormal cytology. Cancer Cytopathol. 2019;127(3):169–180. DOI:10.1002/cncy.22103.
- Ebisch RM, van der Horst J, Hermsen M, et al. Evaluation of p16/Ki-67 dual-stained cytology as triage test for high-risk human papillomavirus-positive women. Mod Pathol. 2017;30(7):1021–1031. DOI:10.1038/modpathol.2017.16.
- Gonzalez MA, Tachibana K-EK, Laskey RA, et al. Control of DNA replication and its potential clinical exploitation. Nat Rev Cancer. 2005;5(2):135–141. DOI:10.1038/nrc1548.
- Zheng J. Diagnostic value of MCM2 immunocytochemical staining in cervical lesions and its relationship with HPV infection. Int J Clin Exp Pathol. 2015;8(1):875–880.
- Ratnam S, Coutlee F, Fontaine D, et al. Clinical performance of the PreTect HPV-Proofer E6/E7 mRNA assay in comparison with that of the Hybrid Capture 2 test for identification of women at risk of cervical cancer. Journal of Clinical Microbiology. 2010;48(8):2779–2785. (1098-660X (Electronic)). DOI:10.1128/JCM.00382-10.
- Alaghehbandan R, Fontaine D, Bentley J, et al. Performance of proex c and pretect hpv-proofer e6/e7 mrna tests in comparison with the hybrid capture 2 hpv dna test for triaging ascus and lsil cytology. Diagn Cytopathol. 2013;41(9):767–775. DOI:10.1002/dc.22944.
- Ding L, Song L, Zhao W, et al. Predictive value of p16INK4a, Ki-67 and ProExC immuno‑qualitative features in LSIL progression into HSIL. Exp Ther Med. 2020;19(4):2457–2466.
- Amaro Filho SM, Nuovo GJ, Cunha CB, et al. Correlation of MCM2 detection with stage and virology of cervical cancer. Int J Biol Markers. 2014;29(4):e363–71. DOI:10.5301/jbm.5000081.
- Tang X, Xu Y, Lu L, et al. Identification of key candidate genes and small molecule drugs in cervical cancer by bioinformatics strategy. Cancer Management and Research. 2018;Volume 10:3533–3549. (1179–1322 (Print)). DOI: 10.2147/CMAR.S171661.
- Thomas LK, Bermejo JL, Vinokurova S, et al. Chromosomal gains and losses in human papillomavirus-associated neoplasia of the lower genital tract - a systematic review and meta-analysis. Eur J Cancer. 2014;50(1):85–98. DOI:10.1016/j.ejca.2013.08.022.
- Seppo A, Jalali GR, Babkowski R, et al. Gain of 3q26: a genetic marker in low-grade squamous intraepithelial lesions (LSIL) of the uterine cervix. Gynecol Oncol. 2009;114(1):80–83. DOI:10.1016/j.ygyno.2009.03.031.
- Koeneman MM, Ovestad IT, Janssen EAM, et al. Gain of chromosomal region 3q26 as a prognostic biomarker for high-grade cervical intraepithelial neoplasia: literature overview and pilot study. Pathol Oncol Res. 2019;25(2):549–557. DOI:10.1007/s12253-018-0480-y.
- Wright TC, Compagno J, Romano P, et al. Amplification of the 3q chromosomal region as a specific marker in cervical cancer. Am J Obstet Gynecol. 2015;213(1):51 e1–51 e8. DOI:10.1016/j.ajog.2015.02.001.
- Sokolova I, Algeciras-Schimnich A, Song M, et al. Chromosomal biomarkers for detection of human papillomavirus associated genomic instability in epithelial cells of cervical cytology specimens. J Mol Diagn. 2007;9(5):604–611. DOI:10.2353/jmoldx.2007.070007.
- Heitmann ER, Lankachandra KM, Wall J, et al. 3q26 amplification is an effective negative triage test for LSIL: a historical prospective study. Plos One. 2012;7(7):e39101. DOI:10.1371/journal.pone.0039101.
- Lin WM, Michalopulos EA, Dhurander N, et al. Allelic loss and microsatellite alterations of chromosome 3p14.2 are more frequent in recurrent cervical dysplasias. Clin Cancer Res. 2000;6(4):1410.
- Harima Y, Harima K, Sawada S, et al. Loss of heterozygosity on chromosome 6p21.2 as a potential marker for recurrence after radiotherapy of human cervical cancer. Clin Cancer Res. 2000;6(3):1079.
- Arias-Pulido H, Joste N, Wheeler CM. Loss of heterozygosity on chromosome 6 in HPV-16 positive cervical carcinomas carrying the DRB1*1501-DQB1*0602 haplotype. Genes Chromosomes Cancer. 2004;40(4):277–284.
- Mazurenko Nn Fau - Bliev AI, Bliyev AY, Bidzhieva BA, et al. [Loss of heterozygosity at chromosome 6 as a marker of early genetic alterations in cervical intraepithelial neoplasias and microinvasive carcinomas]. Mol Biol (Mosk). 2006;40(3):385–395. (0026–8984 (Print)). DOI:10.1134/S0026893306030058.
- Chambuso R, Kaambo E, Denny L, et al. Investigation of cervical tumor biopsies for chromosomal loss of heterozygosity (LOH) and microsatellite instability (MSI) at the HLA II Locus in HIV-1/HPV Co-infected Women. Front Oncol. 2019;9:951–951.
- Beiersdorf J, Scheungraber C, Wunsch K, et al. Combined assessment of 3q26 amplification and promoter methylation in patients with high grade cervical lesions show age specific differences. Genes Chromosomes Cancer. 2020;59(3):168–177. DOI:10.1002/gcc.22818.
- Xu W, Xu M, Wang L, et al. Integrative analysis of DNA methylation and gene expression identified cervical cancer-specific diagnostic biomarkers. Signal Transduct Target Ther. 2019;4(1):55. DOI:10.1038/s41392-019-0081-6.
- Steenbergen RD, Snijders PJF, Heideman DAM, et al. Clinical implications of (epi)genetic changes in HPV-induced cervical precancerous lesions. Nat Rev Cancer. 2014;14(6):395–405. DOI:10.1038/nrc3728.
- Lorincz AT. Virtues and weaknesses of DNA methylation as a test for cervical cancer prevention. Acta Cytol. 2016;60(6):501–512.
- Lai HC, Ou Y-C, Chen T-C, et al. PAX1/ SOX1 DNA methylation and cervical neoplasia detection: a taiwanese gynecologic oncology group (TGOG) study. Cancer Med. 2014;3(4):1062–1074. DOI:10.1002/cam4.253.
- Su P-H, Lai H-C, Huang R-L, et al. Paired box-1 (PAX1) activates multiple phosphatases and inhibits kinase cascades in cervical cancer. Sci Rep. 2019;9(1):9195. DOI:10.1038/s41598-019-45477-5.
- Verhoef VM, Heideman DAM, van Kemenade FJ, et al. Methylation marker analysis and HPV16/18 genotyping in high-risk HPV positive self-sampled specimens to identify women with high grade CIN or cervical cancer. Gynecol Oncol. 2014;135(1):58–63. DOI:10.1016/j.ygyno.2014.08.003.
- Leeman A, Ebisch RMF, Kasius A, et al. Defining hrHPV genotypes in cervical intraepithelial neoplasia by laser capture microdissection supports reflex triage of self-samples using HPV16/18 and FAM19A4/miR124-2 methylation. Gynecol Oncol. 2018;151(2):311–318. DOI:10.1016/j.ygyno.2018.09.006.
- Vink FJA-OHOO, Meijer CJLM, Clifford GM, et al. FAM19A4/miR124-2 methylation in invasive cervical cancer: a retrospective cross-sectional worldwide study. International Journal of Cancer. 2020;147(4):1215–1221. (1097–0215 (Electronic)). DOI:10.1002/ijc.32614.
- Qiagen. QIAGEN launches qiasure methylation test to determine cervical cancer risk. 2016 [cited 2020 May 10]; Available from: https://corporate.qiagen.com/newsroom/press-releases/2016/20160614-qiasure
- De Strooper LMA, Verhoef VMJ, Berkhof J, et al. Validation of the FAM19A4/mir124-2 DNA methylation test for both lavage- and brush-based self-samples to detect cervical (pre)cancer in HPV-positive women. Gynecol Oncol. 2016;141(2):341–347. DOI:10.1016/j.ygyno.2016.02.012.
- Kelly H, Benavente Y, Pavon MA, et al. Performance of DNA methylation assays for detection of high-grade cervical intraepithelial neoplasia (CIN2+): a systematic review and meta-analysis. Br J Cancer. 2019;121(11):954–965. DOI:10.1038/s41416-019-0593-4.
- Verhoef VMJ, van Kemenade FJ, Rozendaal L, et al. Follow-up of high-risk HPV positive women by combined cytology and bi-marker CADM1/MAL methylation analysis on cervical scrapes. Gynecol Oncol. 2015;137(1):55–59. DOI:10.1016/j.ygyno.2015.01.550.
- Bu Q, Wang S, Ma J, et al. The clinical significance of FAM19A4 methylation in high-risk HPV-positive cervical samples for the detection of cervical (pre)cancer in Chinese women. BMC Cancer. 2018;18(1):1182. DOI:10.1186/s12885-018-4877-5.
- Dankai W, Khunamornpong S, Siriaunkgul S, et al. Role of genomic DNA methylation in detection of cytologic and histologic abnormalities in high risk HPV-infected women. Plos One. 2019;14(1):e0210289. DOI:10.1371/journal.pone.0210289.
- Verlaat W, Snoek BC, Heideman DAM, et al. Identification and validation of a 3-gene methylation classifier for HPV-based cervical screening on self-samples. Clin Cancer Res off J Am Assoc Cancer Res. 2018;24(14):3456–3464. DOI:10.1158/1078-0432.CCR-17-3615.
- Verhoef VMJ, Bosgraaf RP, van Kemenade FJ, et al. Triage by methylation-marker testing versus cytology in women who test HPV-positive on self-collected cervicovaginal specimens (PROHTECT-3): a randomised controlled non-inferiority trial. Lancet Oncol. 2014;15(3):315–322. DOI:10.1016/S1470-2045(14)70019-1.
- Banno K, Iida M, Yanokura M, et al. MicroRNA in cervical cancer: oncomiRs and tumor suppressor miRs in diagnosis and treatment. ScientificWorldJournal. 2014;2014:178075.
- Pardini B, De Maria D, Francavilla A, et al. MicroRNAs as markers of progression in cervical cancer: a systematic review. BMC Cancer. 2018;18(1):696. DOI:10.1186/s12885-018-4590-4.
- Snoek BC, Verlaat W, Babion I, et al. Genome-wide microRNA analysis of HPV-positive self-samples yields novel triage markers for early detection of cervical cancer. International Journal of Cancer. 2019;144(2):372–379. (1097–0215 (Electronic)). DOI:10.1002/ijc.31855.
- Ye J, Cheng X-D, Cheng B, et al. MiRNA detection in cervical exfoliated cells for missed high-grade lesions in women with LSIL/CIN1 diagnosis after colposcopy-guided biopsy. BMC Cancer. 2019;19(1):112. DOI:10.1186/s12885-019-5311-3.
- Okoye JO, Ngokere AA, Onyenekwe CC, et al. Comparable expression of miR-let-7b, miR-21, miR-182, miR-145, and p53 in serum and cervical cells: diagnostic implications for early detection of cervical lesions. Int J Health Sci (Qassim). 2019;13(4):29–38.
- Asangani IA, Rasheed SAK, Nikolova DA, et al. MicroRNA-21 (miR-21) post-transcriptionally downregulates tumor suppressor Pdcd4 and stimulates invasion, intravasation and metastasis in colorectal cancer. Oncogene. 2008;27(15):2128–2136. DOI:10.1038/sj.onc.1210856.
- Lao G, Liu P, Wu Q, et al. Mir-155 promotes cervical cancer cell proliferation through suppression of its target gene LKB1. Tumour Biol. 2014;35(12):11933–11938. DOI:10.1007/s13277-014-2479-7.
- Park S, Eom K, Kim J, et al. MiR-9, miR-21, and miR-155 as potential biomarkers for HPV positive and negative cervical cancer. BMC Cancer. 2017;17(1):658. DOI:10.1186/s12885-017-3642-5.
- Group R, Roche wins the first HPV primary screening tender in Europe. 2015.
- FDA. P890064/S009. Digene hybrid capture® 2 (HC2) high-risk HPV DNA test 2003 [cited 2020 May 1]; Available from: https://www.accessdata.fda.gov/cdrh_docs/pdf/p890064s009a.pdf
- FDA. P080015. CERVISTA HPV 16/18. 2009 [cited 2020 May 1]; Available from: https://www.accessdata.fda.gov/cdrh_docs/pdf8/P080015a.pdf
- FDA. P160037. BD Onclarity HPV Assay. 2018 [cited 2020 July 15]; Available from: https://www.accessdata.fda.gov/cdrh_docs/pdf16/P160037a.pdf
- FDA. P100020. cobas HPV test. 2011. [[cited 2020 May 1]]. Available from: https://www.accessdata.fda.gov/cdrh_docs/pdf10/p100020a.pdf
- FDA. P100042. APTIMA HPV assay. 2011. [[cited 2020 May 1]]. Available from: https://www.accessdata.fda.gov/cdrh_docs/pdf10/p100042a.pdf
- Carozzi F, Visioli CB, Confortini M, et al. hr-HPV testing in the follow-up of women with cytological abnormalities and negative colposcopy. Br J Cancer. 2013;109(7):1766–1774. DOI:10.1038/bjc.2013.519.
- Kudela E, Farkasova A, Visnovsky J, et al. Amplification of 3q26 and 5p15 regions in cervical intraepithelial neoplasia. Acta Obstet Gynecol Scand. 2014;93(10):997–1002. DOI:10.1111/aogs.12485.
- Jiang Y, Hu Z, Zuo Z, et al. Identification of circulating MicroRNAs as a promising diagnostic biomarker for cervical intraepithelial neoplasia and early cancer: a meta-analysis. Biomed Res Int. 2020;2020:4947381.
- Jin Y, Kim SC, Kim HJ, et al. Use of protein-based biomarkers of exfoliated cervical cells for primary screening of cervical cancer. Arch Pharm Res. 2018;41(4):438–449. DOI:10.1007/s12272-018-1015-5.
- Kontostathi G, Zoidakis J, Anagnou NP, et al. Proteomics approaches in cervical cancer: focus on the discovery of biomarkers for diagnosis and drug treatment monitoring. Expert Rev Proteomics. 2016;13(8):731–745. (1744–8387 (Electronic)). DOI:10.1080/14789450.2016.1210514.
- Benczik M, Galamb Á, Koiss R, et al. Claudin-1 as a biomarker of cervical cytology and histology. Pathol Oncol Res. 2016;22(1):179–188. (0253–6269 (Print)). DOI:10.1007/s12253-015-9990-z.
- Zacapala-Gómez AE, Navarro-Tito N, Alarcón-Romero LDC, et al. Ezrin and E-cadherin expression profile in cervical cytology: a prognostic marker for tumor progression in cervical cancer. BMC Cancer. 2018;18(1):349. DOI:10.1186/s12885-018-4243-7.
- Mohammed SI, Ren W, Flowers L, et al. Point-of-care test for cervical cancer in LMICs. Oncotarget. 2016;7(14):18787–18797. DOI:10.18632/oncotarget.7709.
- Usyk M, Zolnik CP, Castle PE, et al. Cervicovaginal microbiome and natural history of HPV in a longitudinal study. PLoS Pathog. 2020;16(3):e1008376. DOI:10.1371/journal.ppat.1008376.
- Oh HY, Kim B-S, Seo -S-S, et al. The association of uterine cervical microbiota with an increased risk for cervical intraepithelial neoplasia in Korea. Clin Microbiol Infect. 2015;21(7):674.e1-674.e9. DOI:10.1016/j.cmi.2015.02.026.
- Curty G, Costa RL, Siqueira JD, et al. Analysis of the cervical microbiome and potential biomarkers from postpartum HIV-positive women displaying cervical intraepithelial lesions. Sci Rep. 2017;7(1):17364. DOI:10.1038/s41598-017-17351-9.