For some years now, interest in the development of devices that allow a fast, accurate, and affordable molecular analysis of different types of samples has grown exponentially. But besides on achieving better and better analytical performance, one of the main interests of industry is on taking that technology out from the laboratory to perform the analyses directly on-site. The so-called point-of-care (POC) testing technology allows significantly reducing the response time, as the sample doesn’t need to be taken to a central laboratory, and it can be used by minimally trained personnel or even autonomously operated. These characteristics provide tremendous benefits in fields ranging from medical diagnostics to food safety, environmental monitoring, or detection of biological threats, among others. And today, in the current COVID-19 pandemic situation, the benefits of POC systems are much more evident even for the general population, which has seen how is possible being tested for SARS-CoV-2 antigens/antibodies in their local medical center, pharmacy or even at home, getting the result in few minutes.
Many current POC systems are based on lateral flow immunoassays, being pregnancy tests the most popular example (until the reach of COVID-19 tests). Lateral flow tests are quite simple, easy to use, and affordable, but they only provide a yes/no response and their sensitivity might be quite limited for many applications. Another popular technology for the development of POC systems is based on electrochemical sensing, as for the case of the most important POC devices nowadays: glucometers. These devices allow to perform a quantitative detection of the target analyte (blood glucose concentration in this case) in a fast, simple, and inexpensive way, what is key for the patient to manage the disease.
Many efforts are now focused on boosting the potential of POC devices to carry out a more complex and complete analysis that quickly and easily provides much more relevant information. The objective is obtaining POC devices able to perform almost any analysis, with a superior sensitivity that allows detecting very low concentrations of the target analytes in a label-free format, with minimal/none previous sample preparation, and able to simultaneously detect tens/hundreds (or even thousands) of analytes in order to obtain a more valuable information (e.g. various disease-related biomarkers for a more reliable diagnosis or for the combined diagnosis of various diseases for the implementation of massive general screening procedures). These higher complexity analysis systems are typically referred as Lab-on-Chip (LOC) devices and they are enabled by current advances in miniaturization of the different technologies required for the creation of the cartridges used (fluidics, sensing elements, biochemistry, etc.).
Among the different transduction technologies available for the development of sensing devices, optical/photonic sensors are one of those with a higher potential for LOC technology. Typically, optical sensors have been based on fluorescence or colorimetric methods, where labeling is a must. Label-free optical detection was then achieved using for example surface plasmon resonance (SPR) sensors, a technique in the frontier between optics and electronics, where very high sensitivities can be achieved, but where multiplexed detection is very limited. In this context, there is currently a huge interest in optical sensors based on guided-wave integrated nanophotonics. In this technology, photonic chips comprising different nanometric-scale elements able to guide and control light are created, being the photonic counterpart of microelectronic chips. Integrated nanophotonic sensing structures can be developed to exhibit a high interaction between the optical field and the molecules of interest, thus being able to directly detect the small variations in the refractive index caused by the presence of those substances without the need of labels. In this way, it is possible to develop sensing elements with a very high sensitivity and a size of only a few μm2, so that we can integrate hundreds of them in a photonic chip of few mm2 for highly multiplexed detection (see). And very importantly, these photonic chips can be fabricated using mass-production materials, equipment, and processes coming from the microelectronics industry, thus ensuring high volumes and low costs.
Figure 1. Very small integrated nanophotonic chips can comprise hundreds of sensing structures. A scanning electron microscope image of one of the photonic sensing structures fabricated in our group having a footprint of only 30 μm2 is depicted
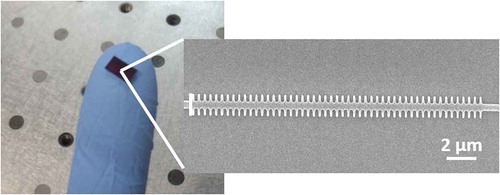
The first proposals of integrated nanophotonic sensors, which were based on grating couplers and on Mach-Zehnder interferometers, date back to the mid-80s and the early 90s of the last century [Citation1,Citation2]. Because the principle of transduction of these integrated nanophotonic sensors is based on their sensitivity to changes in the refractive index, regardless of the origin of those changes, part of the subsequent work focused on providing these photonic structures with specificity toward the analytes of interest. That was achieved by developing biofunctionalization protocols that allowed to adapt immunoassays to these photonic transduction platforms through the immobilization of specific bioreceptors. Since those early works, many other configurations of photonic sensing structures have been proposed, with interferometers, whispering gallery mode resonators or photonic crystals being the most popular ones, and relevant results for a wide range of analytes have been reported, including antibodies, proteins, RNA/DNA, pesticides, etc. [Citation3–7].
After the path traveled since those first results, integrated nanophotonic sensing technology is getting closer to entering our lives. However, several barriers exist in front of it. In the first place, integrated nanophotonics is not the only technology where work targeting the development of novel high-performance sensing devices is being carried out. We can also find developments in the same line for other technologies such as acoustic, nanoelectronic, or micromechanical. These other technologies can also provide relevant sensitivities in many cases, but their main limitations are related to their level of miniaturization and their interrogation method. In addition, all these technologies (including photonics) must compete with already established techniques having a high level of maturity, which are very difficult to displace, despite they may have limitations in sensitivity, speed, cost and/or complexity in many cases. For this reason, it is very important to define an appropriate business strategy that makes it easier for integrated nanophotonic sensing technology to penetrate the market.
An example of strategy adequation can be found in the US company Genalyte (genalyte.com). Genalyte is the reference company in the field of integrated nanophotonic sensing and it has been a pioneer on successfully taking that technology out from the research lab. It began working just over 10 years ago on the development of its photonic sensing platform based on silicon ring resonators and on the implementation of different assays mainly focused on the detection of biomarkers related with medical diagnosis (e.g. cancer biomarkers, microRNAs, autoantibodies, etc.). Genalyte’s initial market strategy was the usual one in these cases: to offer a high-performance analysis platform that could be incorporated by medical centers and certain doctor’s offices into their daily diagnostic routine. However, in recent years the company has redirected its strategy toward a business model in which the company directly offers analytical services using its technology (e.g. for the diagnosis of autoimmune diseases [Citation8]). This change in strategy could indicate a more appropriate path to follow today to begin entering into the market, since the end user may be reluctant to invest in the acquisition of equipment based on a less mature technology, no matter how promising it is, while offering a service in a ‘pay-per-analysis’ mode may make the end user to only be concerned about the reliability and the speed of the analysis without caring too much about how it was carried out (and will have the option of not using the service again if it has not met their expectations).
Another example is that of a younger Spanish company, Lumensia Sensors (lumensia.com), which develops integrated nanophotonic sensing chips based on silicon nitride ring resonators. During its initial steps, the company aimed at the field of medical diagnosis, which is probably the most attractive due to its social and economic benefits. However, it can also be the most complicated due to the amount of requirements and regulation that it implies. In this scenario, the company decided moving its focus to the field of food safety and quality monitoring, raising the interest of several medium-sized food companies from the region which are concerned about the economic impact derived from the long result times when using traditional laboratory techniques. These food companies have invested in Lumensia Sensors to develop its technology for monitoring contaminants and allergens in order to perform a more rapid control that reduces the risk of outbreaks and of stock stoppage.
Finally, the current COVID-19 pandemic situation could also mean an additional key push for integrated nanophotonic sensing technology, since many groups are redirecting their efforts toward this problem, including these two companies. In the case of Genalyte, the company has been offering EUA (Emergency Use Authorization) authorized serological test services for the detection of a panel of antibodies against SARS-CoV-2 in parallel with antibodies against other similar viruses for better discrimination [Citation9]. On the other hand, Lumensia Sensors is adapting its platform for the direct detection of the SARS-CoV-2 virus in nasopharyngeal samples as well as antibodies in blood serum. Another relevant case is that of the ICN2 research institute in Spain, where they are adapting their high-sensitivity bimodal interferometric sensing technology not only for the direct detection of the SARS-CoV-2 virus but also of its genetic material without the need for PCR amplification and using saliva samples [Citation10].
Therefore, it is likely that integrated nanophotonic sensing technology is very close to entering our lives. In addition, we must take into account that the interest on integrated nanophotonic technology spans over many other fields such as communications or computing, where it is not too risky to predict that it will replace current electronic-based technologies in many scenarios. So, that technological progress in each of those fields will be beneficial for the others, generating a virtuous circle that will help to reduce production costs and that we benefit sooner rather than later from the full potential of this sensing technology.
Declaration of interest
The authors have no relevant affiliations or financial involvement with any organization or entity with a financial interest in or financial conflict with the subject matter or materials discussed in the manuscript. This includes employment, consultancies, honoraria, stock ownership or options, expert testimony, grants or patents received or pending, or royalties.
Reviewer disclosures
Peer reviewers on this manuscript have no relevant financial or other relationships to disclose.
Additional information
Funding
References
- Tiefenthaler K, Lukosz W. Integrated optical switches and gas sensors. Opt Lett. 1984;9(4):137–139.
- Heideman RG, Kooyman RPH, Greve J. Development of an optical waveguide interferometric immunosensor. Sens Actuators B Chem. 1991;4(3–4):297–299.
- Chocarro-Ruiz B, Fernández-Gavela A, Herranz S, et al. Nanophotonic label-free biosensors for environmental monitoring. Curr Opin Biotechnol. 2017;45:175.183.
- Luan E, Shoman H, Ratner DM, et al. Silicon photonic biosensors using label-free detection. Sensors. 2018;18(10):3519.
- Ma Y, Dong B, Lee C. Progress of infrared guided-wave nanophotonic sensors and devices. Nano Converg. 2020;7:12.
- Jiang X, Qavi AJ, Huang SH, et al. Whispering-gallery sensors. Matter. 2020;3(2):371–392.
- Wang J, Sanchez MM, Yin Y, et al. Silicon-based integrated label-free optofluidic biosensors: latest advances and roadmap. Adv Mater Technol. 2020;5(6):1901138.
- Miyara M, Charuel JL, Mudumba S, et al. Detection in whole blood of autoantibodies for the diagnosis of connective tissue diseases in near patient testing condition. PLoS ONE. 2018;13(8):e0202736. .
- [ cited 2021 Jan 31] Available from: https://www.genalyte.com/wp-content/uploads/2020/12/SARS-CoV-2-Product-Sheet-12.16.20.pdf
- Soler M, Estevez MC, Cardenosa-Rubio M, et al. How nanophotonic label-free biosensors can contribute to rapid and massive diagnostics of respiratory virus infections: COVID-19 case. ACS Sens. 2020;5(9):2663–2678.