ABSTRACT
Introduction
The search for the ‘perfect’ renal replacement therapy has been paralleled by the search for the perfect biomarkers for assessing dialysis adequacy. Three main families of markers have been assessed: small molecules (prototype: urea); middle molecules (prototype β2-microglobulin); comprehensive and nutritional markers (prototype of the simplified assessment, albumin levels; composite indexes as malnutrition-inflammation score). After an era of standardization of dialysis treatment, personalized dialysis schedules are increasingly proposed, challenging the dogma of thrice-weekly hemodialysis.
Areas covered
In this review, we describe the advantages and limitations of the approaches mentioned above, focusing on the open questions regarding personalized schedules and incremental hemodialysis.
Expert opinion
In the era of personalized dialysis, the assessment of dialysis adequacy should be likewise personalized, due to the limits of ‘one size fits all’ approaches. We have tried to summarize some of the relevant issues regarding the determination of dialysis adequacy, attempting to adapt them to an elderly, highly comorbidity population, which would probably benefit from tailor-made dialysis prescriptions. While no single biomarker allows precisely tailoring the dialysis dose, we suggest using a combination of clinical and biological markers to prescribe dialysis according to comorbidity, life expectancy, residual kidney function, and small and medium-size molecule depuration.
1. Introduction
Identifying the ‘perfect’ renal replacement therapy (RRT) has long been the utopia of dialysis; in the 80s, ‘perfect dialysis’ had been defined as a treatment that would result in a life of quality and duration comparable to that of people without kidney diseases [Citation1,Citation2]. Progress in the search for the best treatment has been paralleled by the search for the perfect biomarkers for assessing dialysis adequacy [Citation3,Citation4]. Neither has yet been achieved.
Three main markers of dialysis adequacy have been tested and found to be insufficient, but nonetheless still remain interesting in the clinical management of dialysis patients: small molecules (prototype: urea, and Kt/V); middle molecules (prototype: β2-microglobulin); comprehensive clinical and nutritional markers (prototype of the simplified assessment: albumin levels; composite indexes such as the malnutrition-inflammation score) [Citation5–7].
After numerous attempts to achieve easy and repeatable standardization of dialysis treatment, in keeping with a similar trend in many other areas of medicine, personalized dialysis schedules are now increasingly being proposed, challenging the traditional dogmas related to thrice-weekly hemodialysis [Citation8].
At the dawn of RRT, the concept of ‘dialysis adequacy’ has been clinically interpreted as the amount of dialysis required to keep a patient alive, in relatively good clinical conditions and relatively asymptomatic [Citation2]. The issue of reaching a compromise between the iatrogenicity of dialysis and blood depuration from noxious toxins led to a progressive decrease in the duration of hemodialysis, from 12 to 6 hours with an increase in the number of sessions, from once to thrice weekly [Citation9,Citation10].
Interestingly, the ‘short dialysis’ schedule, of 4 hours three times per week, was initially proposed by Cambi in Italy, as a way to treat more patients during the same working day, in an era in which dialysis availability was still limited [Citation9,Citation11]. The success of this approach, which was widely applied in the western world, was due to a combination of good short-term results, acceptable metabolic control (as per the standards at the time), lesser impact on everyday life and, probably even more importantly, a better integration into the daily routine of the dialysis wards. Indeed, economic constraints are probably the main reasons why the compromise between number and duration of dialysis sessions was finally reached, i.e. 4 hours three times per week [Citation12,Citation13].
In the early days of hemodialysis, the prescription was mainly based upon dialyzer surface area and time. Targeting prescriptions on pre-dialysis data was clearly not enough to ensure the well-being of the patients. At a time in which malnutrition was common among dialysis patients and cachexia was one of the leading causes of death, low urea and creatinine levels could be different sides of the same coin: malnutrition or good dialysis management. Indeed, in 1981, the National Cooperative Dialysis Study showed that higher pre-dialysis urea levels were associated with higher comorbidity, hospitalizations and mortality [Citation3]. Using data from this study, a dynamic model analyzing the ratio between pre- and post-dialysis urea was conceived. This first kinetic model considered three elements defining dialysis dose: dialyzer urea clearance (K), dialysis time (t), and urea distribution volume (V), as a reflection of body size and corresponding to total body water, leading to the famous Kt/V formula [Citation14].
The ratio between pre- and post-dialysis urea was found to be more important than the actual levels, and this concept was translated in a simple equation: Kt/V. Kt/V was equivalent to the natural logarithm of the ratio between the pre- and post-dialysis urea blood concentration, assuming no ultrafiltration or urea generation. The gold standard for a fully adequate dialysis prescription was set at a Kt/V of 1 [Citation14], which was later increased to 1.2 [Citation15]. This simple equation was further over-simplified in the concept of urea reduction rate (URR), which assumed that in adequate dialysis end of treatment urea had to be ≤33.3% of the initial value [Citation16].
These equations rapidly spread among the scientific community.
However, the initial simplicity left space to the search for the perfect equation, more and more complex calculations were proposed and, somehow, the search for the perfect equation replaced the search for the perfect dialysis. In fact, urea has a multicompartmental distribution to which different mechanisms contribute during the dialysis session: access recirculation, cardiopulmonary recirculation and the distribution of urea between intra- and extracellular compartments and low- and high blood flow compartments [Citation17]. To avoid an overestimation of Kt//V, post-dialysis blood samples should theoretically be drawn at the equilibrium, at least 30 to 60 minutes after the end of the treatment, to account for the urea rebound. As waiting such a long time is impractical, the concept of equilibrated Kt/V (or double-pool Kt/V) was developed and the original Gotch and Sargent’s equation was differently modified in the nineties, to account for the post-dialysis urea rebound, giving rise to a plethora of formulae, which attempted to precisely describe the in vivo kinetics of urea in dialysis patients [Citation18–23].
Furthermore, to acknowledge the importance of nutrition in the determination of dialysis efficacy, even more complex equations were proposed, trying to assess protein intake on the basis of the urea generation rate (the normalized protein catabolic rate – nPCR) [Citation24,Citation25].
Thirty years later, what remains of these massive efforts is relatively limited.
The most commonly used Kt/V formula is a second generation one, proposed by Daugirdas, which results in less overestimation of the dialysis dose for values higher than 1.3 [Citation26].
Conversely, nPCR is no longer considered a reliable tool for assessing adequate nutrition associated with efficiency of the dialysis treatment, as it is influenced by common conditions such as inflammation, liver failure, volume expansion and urinary or dialysate protein losses, and its association with mortality decreases if adjusted for malnutrition and inflammation [Citation27–30]. Moreover, since nPCR does not distinguish between proteins derived from dietary sources or endogenous catabolism and it is valid only in stable patients [Citation25,Citation31]. Within these limits, the most recent guidelines suggest a daily protein intake of 1–1.2 g/kg/day and nPCR is still included among the criteria to diagnose and score protein energy wasting (PEW) to predict survival, as it shows a U-shaped relationship with mortality [Citation27,Citation30,Citation32,Citation33].
Meanwhile, the dialysis population has changed, patients start dialysis at previously unforeseen older ages, dialysis techniques have evolved, membranes have improved and, in line with the paradigm shift observed in several other diseases, standardized treatments have given way to personalized ones [Citation8,Citation34].
Indeed, Kt/V is only validated for three times per week hemodialysis schedules; it is not formally validated for hemodiafiltration (HDF), incremental dialysis, or extended or daily hemodialysis, even if, as will be further discussed, urea-based kinetic models are widely used in all of them and nomograms may allow comparing different dialysis schedules [Citation35].
In this review, we will discuss what may be needed to implement the classic assessment of dialysis adequacy in order to help clinicians optimizing a patient-friendly personalized renal replacement treatment. A schematic representation of the evolution of the concept of dialysis adequacy is depicted in .
Figure 1. Evolution of the concept of dialysis adequacy
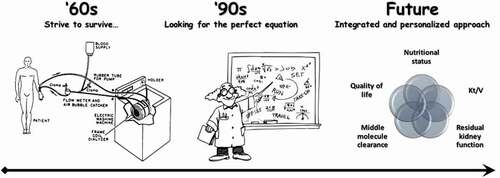
2. Urea and Kt/V: old but not gold?
Urea was the first identified marker of kidney function, hence the name uremic syndrome. It was initially considered more for its correlation with kidney function and ease of measurement rather than its toxicity [Citation36]. Later, creatinine clearance took over in the evaluation of kidney function but urea resurged as a marker of dialysis efficiency.
Despite its long history, the actual role played by urea in uremic syndrome is not fully understood. In older studies, dialysis against a urea enriched bath was not able to reproduce uremic symptoms; however, later, urea has been shown to have direct toxic effects promoting anemia through carbamylation, inducing endothelial cell apoptosis, participating in intestinal barrier dysregulation and insulin resistance [Citation37–41].
Within the limits of an incomplete relationship between urea levels and uremic symptoms, urea remains the main marker of low molecular weight uremic toxins and adequate clearance of small molecules should be considered the first step of dialysis adequacy [Citation42]. Kt/V set the standards of dialysis, at least for thrice-weekly patients, and for determining adequacy on the basis of removal rate rather than on pre-dialysis concentration [Citation43]. However, urea-derived Kt/V has shown several limitations. While its role was suggested by the first large study trying to combine biochemical markers of dialysis and survival, later, two large randomized, controlled trials, i.e. HEMO and ADEMEX, failed to identify differences in mortality according to Kt/V in hemodialysis or peritoneal dialysis patients [Citation44,Citation45]. Post-hoc analyses of the HEMO study suggested that a higher dialysis dose could be associated with better outcomes but also highlighted the pitfalls of the equation, mainly related to the difficult estimation of the urea distribution volume [Citation46,Citation47]. In fact, individuals of small body size are at higher risk of underdialysis due to their lower V [Citation48–50]. It has also been suggested that smaller people, with low body mass index (BMI), have a greater proportion of highly active metabolic compartment (visceral organs), in proportion to their body weight, thus generating more uremic toxins per kilogram, and therefore adding to the risk of insufficient dialysis [Citation51].
Urea is dialyzed against a urea-free dialysate. The ratio between urea at the start and at the end of dialysis, and the speed of the decrease in urea levels is also a result of the initial concentration; consequently, patients starting with higher urea levels may attain a higher Kt/V due to the greater blood to dialysate gradient. In the elderly, urea generation is reduced as a consequence of a reduced metabolism, and this may suggest lowering Kt/V targets in this population [Citation52,Citation53]. Moreover, achieving higher Kt/V values in the elderly may require longer dialysis sessions resulting in poor tolerance and impacting on cardiovascular stability and quality of life [Citation54].
Furthermore, as dialysis patients are often over-hydrated, their V is not always easy to calculate, thereby reducing the clinical applicability of urea kinetic modeling, especially in the elderly [Citation55]. Similar considerations hold true for children, for whom commonly used formulae tend to overestimate total body water [Citation56,Citation57]. Obese patients, who account for 20 to over 50% of the dialysis population, pose a further challenge in volume calculation, particularly when they suffer from sarcopenia [Citation58].
To account for these shortcomings, V has been variously replaced by resting energy expenditure (REE) [Citation59], body surface area (BSA) [Citation60], visceral liver mass [Citation61], mass of high metabolic rate organs (HMRO) [Citation61], body weight, or simply removed from the equation [Citation62]. Nevertheless, none of the modified equations has proven superior, and the current guidelines still adopt Kt/V to determine dialysis dose [Citation61,Citation63].
The decrease in urea is logarithmic, therefore its kinetics is different in short daily dialysis, due to the higher decrease in urea in the first two hours of dialysis, when the gradient is the highest. Conversely, in long nightly dialysis, the urea reduction rate reaches a plateau, but the mass transfer of urea is higher, due to the removal of the urea derived from protein catabolism and compartment shift.
In this regard, the extended hemodialysis experience (≥12 h/week or ≥24 h/week according to different definitions) is of particular interest. Results are contrasting: the DOPPS analysis suggests an association between the duration of the dialysis sessions and survival, independently of Kt/V [Citation64]. On the contrary, the Frequent Hemodialysis Network Nocturnal Trial did not demonstrate a survival advantage for the extended dialysis scheme despite a higher Kt/V [Citation65].
Furthermore, the classic Kt/V does not apply to the incremental hemodialysis schedules, which have been recently re-discovered as a suitable means to reduce the ‘dialysis shock’ at the start of RRT, and to improve the quality of life [Citation66–68].
Once- or twice-weekly dialysis schedules provide good results only if they are wisely integrated with the residual kidney function (RKF) which has, in turn, been consistently shown to be positively correlated to survival [Citation69–71]. RKF provides a clearance of middle and protein-bound molecules not accounted for by Kt/V, allows for a more liberal diet and reduces the problems linked to water and salt overload [Citation72].
Indeed, while adaptations of urea kinetic modeling have been proposed to calculate dialysis adequacy, this option is probably reductive and other factors should be emphasized in ‘non-conventional’ hemodialysis.
3. Why urea kinetic models are still useful
Despite several limitations, there are a number of reasons why urea-based kinetic models are still recommended in guidelines as a basis for monitoring the efficiency of dialysis. Firstly, measuring urea is a standardized, low-cost method, which can be easily performed and repeated.
Secondly, the experience is long lasting, and low Kt/V is associated with higher mortality [Citation14,Citation73]. In this regard, Kt/V may identify the minimum dialysis requirement, at least in patients without RKF, on thrice weekly treatments. The target should be modulated by age or clinical conditions.
Thirdly, clearance of the small molecules is more predictable and is a better marker not only of dialysis efficiency, but, more widely, of the dialysis session.
reports some examples of interpretation of the ratio between urea at the start and at the end of the dialysis session. The same pattern may have different explanations: for example, high pre-dialysis urea may be the result of high protein intake, of low dialysis efficiency or of vascular access malfunction. Conversely, very low urea at the end of dialysis may be an indicator of high dialysis efficiency as well as of vascular access recirculation, but it may also be observed in a context of malnutrition. The association between high pre-dialysis and low post-dialysis urea may be the result of good efficiency in a well-nourished patient, of good efficiency in a hypercatabolic patient or suggest fistula recirculation, if the final blood sample is not drawn at the equilibrium. Other biomarkers may help highlight the differences, but more than relying on further biochemical data, clinical evaluation is decisive. In this regard, the meaning of Kt/V shifts from a rigid target that dialysis has to achieve, to an important index to be interpreted in the light of the clinical evaluation.
4. The ‘middle molecules’ issue and the importance of parathyroid hormone and β2-microglobulin
summarizes the advantages and drawbacks of the main middle molecules available for use in the clinical setting.
Table 1. Pros and cons of routinely used dialysis adequacy markers
Clearance of small molecules is only loosely associated with well-being on dialysis [Citation74].
In particular, in the eighties, there was a moment in which researchers hoped that the vast array of uremic toxins could explain the protean picture of uremia. Uremic toxins are a number of molecules excreted by the kidneys, under normal conditions, that are retained and accumulate in the course of chronic kidney disease (CKD) and that have an impact on biological functions [Citation75]. Among them, the so-called ‘middle molecules,’ with a molecular weight of 500 Da to 60 kDa, have important implications in cardiovascular disease and inflammation and contribute to the non-traditional risk factors associated with end-stage kidney disease (ESKD); however, they are also particularly difficult to clear by dialysis due to their size [Citation76,Citation77].
While long lists of uremic toxins have been compiled, only few have acquired a role in clinical practice [Citation75,Citation78]. One of them is the parathyroid hormone (PTH). This molecule, whose molecular weight is 9400 Da, is pivotal to metabolic bone disease in dialysis patients, and it is both a hormone and a uremic toxin. Serum PTH levels depend on multiple factors, including serum calcium and phosphate levels; although international guidelines suggest to keep PTH levels in the range of 2 to 9 times the upper limit of normality [Citation79], in the presence of optimal medical therapy (vitamin D and calcium supplements, calcimimetics, phosphate binders, etc.) and in the absence of tertiary hyperparathyroidism, an increased PTH concentration may be an indicator of an inadequate dialysis. Indeed, the attitude toward hyperparathyroidism management in CKD is not uniform worldwide and the Japanese Society for Dialysis Therapy suggests to keep PTH levels between 60 and 240 pg/ml [Citation80]. This suggestion may be of particular interest on the account of the experience of the Japanese Society with patients with long dialysis vintage. The metabolism of PTH is too complex to allow PTH levels to be used as a self-standing marker of dialysis efficiency; nonetheless, their evaluation could guide dialysis prescription and its intradialytic profile could help tailoring the dialysate calcium content [Citation81–83].
β2-microglobulin (B2M), a component of the class I major histocompatibility complex, with a molecular weight of 11,800 Da, is now considered the paradigm of middle molecules [Citation84]. As in the case of most middle molecules, high B2M levels are correlated with a greater risk of mortality. B2M is, however, not only a by-product of protein metabolism, it is also a marker of inflammation and the main precursor of dialysis-related amyloidosis [Citation4,Citation6,Citation85]. It has been suggested that monitoring B2M might be useful in convective and mixed techniques, and in HDF in particular [Citation86]. B2M clearance is linked to the duration of the dialysis session and to the permeability of the dialysis membranes; unlike small molecules, its clearance is increased in long-hour dialysis [Citation87,Citation88]. Some large European studies found that high-flux hemofiltration was associated with greater B2M clearance compared to standard hemodialysis despite equal Kt/V [Citation89,Citation90]. For instance, although no differences were found in the primary composite outcome of cardiovascular events between high-flux and low-flux membranes, a post-hoc analysis of the Turkish Online Hemodiafiltration Study showed that patients with an arteriovenous fistula and diabetic ones benefited of a longer cardiovascular event-free survival when dialyzed with a high-flux membrane [Citation91]. This difference was paralleled by reduced B2M levels [Citation91]. The benefit in diabetic patients was confirmed by another large trial, although no differences in survival between high- and low-flux membranes was detected in the overall dialysis population [Citation92]. While there are no clear B2M cut-offs identifying efficient dialysis, the Japanese Society for Dialysis Therapy suggests keeping pre-dialysis B2M levels lower or equal to 30 mg/l [Citation4,Citation6,Citation93]. B2M removal is indeed one of the standard pieces of information provided by the dialysis companies to identify the performance of dialyzers and is used to distinguish high-flux from low-flux membranes, replacing hydraulic permeability [Citation94]. Finally, because of its dependence on residual kidney function, B2M levels may be used to monitor RKF in patients unable to collect 24 hour urines [Citation95].
Increased removal of large molecules can be achieved by means of convective treatments or high cutoff membranes. The new medium cutoff dialysis membranes showed optimal performances in removing uremic toxins during HD, comparable to HDF [Citation96]. Polymethyl methacrylate (PMMA) dialyzers, as well as high cutoff membranes, have proven to be effective in removing middle molecules, including free light chains [Citation97,Citation98].
Interest in the intestinal microbiota highlighted the role of toxins derived from this source; in particular of p-cresyl sulfate and indoxyl sulfate [Citation99,Citation100]. They are protein-bound molecules with a molecular weight of 188 Da and 213 Da, respectively, and are derived from the intestinal metabolism of tryptophan and tyrosine, which accumulate in CKD patients [Citation101]. In the course of CKD, there is a change in the microbiota composition, which leads to the selection of bacterial species producing uremic toxins and the downregulation of beneficial strains [Citation102–104]. Gut-derived toxins mediate the gut-kidney crosstalk [Citation105]. In fact, intestinal dysbiosis, coupled with reduced kidney function, leads to increased p-cresyl sulfate and indoxyl sulfate levels, and has been associated with blood vessel inflammation, endothelial dysfunction, increased intestinal permeability, insulin resistance and, in a vicious cycle, altered microbiota composition [Citation106,Citation107]. It has even been suggested that gut-derived uremic toxins could have a role in uremic pruritus and the modulation of the intestinal flora could have a beneficial effect on this difficult-to-treat symptom [Citation108]. P-cresyl sulfate and indoxyl sulfate levels have been associated with mortality in dialysis patients [Citation109,Citation110]. However, while their clearance could be useful for monitoring protein-bound solutes during dialysis, the need for liquid chromatography coupled with mass spectrometry to dose them limits extensive routine clinical use [Citation111,Citation112].
5. Albumin: a survival biomarker in dialysis
Removal of larger molecules comes at a price: increased membrane permeability and increased convective volumes might result in considerable albumin losses during the dialysis session.
In the first studies that attempted to give a mechanistic interpretation of dialysis efficiency, albumin levels emerged as the most important factor associated with survival. This is still true: albumin is one of the most important, comprehensive markers of survival during hemodialysis, underlying as nutritional status is of utmost importance in dialysis patients [Citation113].
The emphasis on albumin has led some scientific societies to use serum albumin levels (usually identified as ≥3.5 or 4 g/dl) as the main, if not the sole, marker of (mal)nutrition in dialysis patients [Citation114]. Of note, the serum albumin assessment method is relevant, as different techniques may yield different results [Citation115]. This simplistic interpretation may, like many over-simplifications, be misleading, and the most recent guidelines on nutritional management in CKD patients advise against considering albumin alone as a reliable nutritional marker and emphasize that it should be combined with other biological markers and a careful clinical assessment (indeed, to stress the subtleties of the nutritional evaluation, at least 15 pages have been dedicated to this topic in the latest published guidelines) [Citation30]. In fact, serum albumin levels are modulated by inflammation, similarly to other nutritional markers, in particular, those of the middle molecule family [Citation116–118].
According to the non-univocal definitions of this condition, the prevalence of malnutrition in dialysis patients varies widely among studies, being reported between 18% and 75% [Citation119–121]. The name itself has changed over time: the initial term ‘malnutrition’ was replaced by protein-energy wasting (PEW), a more extensive term, whose definition is, however, as complex as that of malnutrition [Citation32]. The story has not yet reached the end, and the search for the ‘right term’ to define an elusive condition is still ongoing [Citation122–130].
Moreover, as mentioned above, the dialysis population has dramatically changed over its six decades of history; the young, cachectic, under-dialyzed patient is less frequently encountered, while the prevalence of elderly patients with ‘vascular’ cachexia is increasing, together with that of sarcopenic-obese, protein-wasted patients [Citation131–138]. The potential role of increasing dialysis dose in young under-dialyzed patients is obvious, while increasing dialysis efficiency may be counterproductive in elderly vasculopathic patients, and dialysis modulation is not clear in obese sarcopenic patients.
Several additional markers can be added to albumin levels to better understand the patient’s nutritional status. Among them, pre-albumin is the most widely used one, as also acknowledged by the current National Kidney Foundation’s Kidney Disease Outcomes Quality Initiative (NKF-KDOQI) guidelines, but transferrin, complement, immunoglobulins are also of interest [Citation139,Citation140]. shows the main patterns of albumin pre-albumin combinations encountered in dialysis patients and their potential meaning.
Table 2. Albumin and pre-albumin patterns commonly encountered in dialysis patients
Together with anthropometric measurements [Citation141], a number of scores have been built to describe patient’s nutritional status, and among them, the Malnutrition Inflammation Score (MIS) has gained success for its simplicity, and because it combines the three main constituents, namely comorbidity (and dialysis vintage), biochemical markers and anthropometric assessment [Citation7,Citation142,Citation143].
There is, however, an important point missing in this discussion, i.e. albumin losses [Citation144].
The removal of middle molecules is linked to the structure of the dialysis membrane and, since albumin is one of the smallest, albeit most represented, blood proteins, its intradialytic loss is often the price to pay for better removal of middle molecules. Albumin is incrementally lost from low-flux hemodialysis to high volume pre-dilution HDF [Citation145]. Acceptable albumin losses during a single dialysis session have been suggested to be less than 4 g, which may have a different impact in young, well-nourished patients or in elderly ones [Citation146,Citation147]. Canaud, a paladin of pre-dilutional HDF, even suggested that albumin loss could be favorable for improving nutritional status, postulating that higher albumin losses are associated with greater removal of protein-bound toxins and inflammatory markers, provided that serum albumin levels do not decrease over time. These considerations may suggest reserving highly permeable membranes and post-dilution HDF to patients able to compensate for protein losses [Citation148,Citation149].
There are several differences among dialysis membranes, and recent research has focussed on how to design membranes that allow a significant removal of middle molecules (usually targeted at B2M and myoglobin), with limited albumin loss. Moreover, besides the permeability of the membrane and its sieving coefficient for albumin, the possibility of absorption should be considered. PMMA and Polyester Polymer Alloy (PEPA) are both materials, which have shown a 20% to 60% fractional absorption of albumin, suggesting that analyzing the exhausted dialysate is not enough to account for the losses [Citation150]. Newer high or medium cutoff membranes, employed for expanded hemodialysis, are associated with greater albumin losses (between 2 and 4 g per session for medium cutoff membranes) compared to conventional membranes [Citation151,Citation152]. In particular, high cutoffs are associated with albumin losses of 6–9 g per session and should be reserved to selected patients (multiple myeloma or cast nephropathy) and for a short period of time [Citation151,Citation153]. However, in prospective trials, medium cutoff membranes do not seem to be associated with a significant reduction of albumin levels [Citation154–156]. Nonetheless, the effect may be different in various populations, being less evident in younger patients usually enrolled in randomized controlled trials than in the elderly high comorbidity population at high risk of malnutrition.
The benefits of convective therapies on mortality are still a matter of debate, and the role of albumin loss may be important. Data from the literature are contrasting: an observational analysis of the French REIN registry showed higher survival for patients on HDF compared to hemodialysis, in keeping with another pooled analysis of four randomized controlled trials, which showed a survival benefit associated with on-line HDF for convective volumes greater than 23 l/1.73 m2/session [Citation157,Citation158]. However, a larger Cochrane systematic review of 40 randomized controlled trials found no difference in all-cause mortality, although convective therapies were superior in preventing cardiovascular deaths [Citation159]. In one Spanish study, greater convective volumes in post-dilution HDF were associated with higher albumin losses [Citation160]. Conversely, the Turkish OL-HDF Study and the FRENCHIE trial did not demonstrate a difference in albumin levels between convective therapies and conventional HD over a 2 year follow-up period [Citation161,Citation162]. Differences in the study populations may partially explain the different findings.
Hemodiafiltration is not necessarily performed in the same way in different settings: for instance, in France, for reasons of efficiency, convective therapies are preferred in younger patients with a long life-expectancy or a transplant project, while in Italy, HDF is often chosen to improve cardiovascular stability in fragile subjects [Citation163]. Finally, according to the Japanese experience, HDF should be offered to prevent long-term complications such as dialysis-related amyloidosis () [Citation164–166]. Elderly patients, who are often affected by high comorbidity, might be particularly vulnerable to protein losses and this should be considered together with favoring an incremental dialysis approach [Citation145,Citation167–169].
Table 3. Hemodiafiltration (HDF) treatment approaches to achieve different goals
6. Phosphate balance: an indirect maker of dialysis efficiency
Ideally, RRT should correct electrolyte imbalances assuring their homeostasis even in the interdialytic period. Nevertheless, electrolyte disorders are the rule in dialysis patients.
While some of these disorders are life threatening, others are insidious. Sodium and potassium are easily cleared by dialysis, at difference with phosphorus, due to its higher molecular weight, protein binding and low bioavailability in free form [Citation170].
Serum phosphate levels are major determinants of vascular calcifications and cardiovascular mortality [Citation171–174]. Hyperphosphatemia is common on dialysis, due to a combination of high intake (in particular if the indication of a high intake of proteins is followed) and to the effect of calcium-phosphorus-PTH derangements. In fact, hyperphosphatemia is particularly common in two situations that are independently associated with cardiovascular morbidity: hyperparathyroidism and adynamic bone disease [Citation175,Citation176]. Given all these interferences, efficient dialysis alone may not always allow phosphate control [Citation177]. An exception is extended hours or daily dialysis, in which phosphate levels are often so low as to require supplementation [Citation178].
Dietary management may be difficult, in particular in patients consuming high quantities of processed and preserved foods, containing additives rich in inorganic phosphate, whose role has only recently been acknowledged [Citation179]. Hence, phosphate binders are usually employed, but, although a discussion on this topic is beyond the scope of this review, it should be noted that their use is not without a price, and their effect on mortality is controversial [Citation180].
Once more, the presence of RKF is associated with better phosphate control and preserving it should be a goal, as will be further discussed [Citation181,Citation182].
7. Ultrafiltration: avoiding intradialytic hypotension
Extracellular volume overload is frequently encountered in dialysis patients and is associated to mortality [Citation183]. The aim of ultrafiltration (UF) during the hemodialysis session is to maintain fluid balance, especially in anuric patients. Often, patients are not particularly compliant with fluid restrictions suggested to limit interdialytic weight gain [Citation184,Citation185]. This poses a problem, as higher UF rates have been associated with increased mortality [Citation186,Citation187]. A UF rate >13 ml/kg/h has been identified as a threshold for intradialytic hypotension (IDH), associated with low tolerance and a risk factor for mortality [Citation188,Citation189]. Older patients, with higher UF rates and higher B2M and N-terminal pro-B-type natriuretic peptide (NT-proBNP) are at increased risk of IDH [Citation189,Citation190]. Besides, IDH contributes to vascular access failure and myocardial stunning and remodeling [Citation191,Citation192]. Trying to achieve a better blood pressure control through UF has been shown to increase IDH [Citation193]. Vascular stiffness, myocardial hypertrophy, autonomous neuropathy and delayed refilling are all contributors to IDH [Citation190]. Interdialytic weight gain, obviously, influences IDH and the first sessions of the week are at higher risk for such a complication [Citation194]. Repeated episodes of IDH may reduce patient’s compliance to the dialysis sessions, while dietary restrictions and in-center malaises could impair the patient’s quality of life, up to dialysis discontinuation [Citation195]. Moreover, IDH is associated with lower dialysis efficiency, due to the premature termination of the dialysis session, worst volume control, cerebral ischemia, and rapid loss of residual renal function [Citation196–198]. Thus, a combination of education and promotion of patient’s awareness of the reasons of certain restrictions, adopting a flexible dialysis schedule, frequently assessing the ‘dry weight,’ adapting the dialysate composition and temperature and exploiting the newer blood volume monitoring tools is needed to reduce IDH occurrence [Citation199–206]. Finally, for patients prone to IDH, convective therapies should be considered: HDF, hemofiltration (HF) and acetate-free biofiltration have been shown to reduce IDH [Citation207–209].
8. Acid-base balance and survival
The acid load accumulating because of end-stage kidney disease is a matter of concern [Citation210]. Pre-dialysis bicarbonate levels have shown an inverse association with phosphorus and protein intake. A U-shaped curve is observed as for mortality in analogy with what has been described with other biomarkers including phosphates [Citation211]. Of note, after adjusting for components of the malnutrition-inflammation complex syndrome, bicarbonate levels ≥22 mmol/l were associated with a lower risk of mortality, thus confirming the importance of correcting acidosis [Citation211]. However, bicarbonate levels per se, might not be the best treatment target as another large study did not find a significant relationship between pre- or post-dialysis bicarbonates and all-cause or cardiovascular mortality [Citation212]. On the contrary, a pre-dialysis pH≥7.4 was associated with an increased risk of all-cause and cardiovascular death [Citation212]. Once more, differences in dialysis schedules and populations may account for these partially conflicting results.
9. ‘Non-conventional hemodialysis schedules’: what does non-conventional mean?
The measures and indexes mentioned above have been mostly validated in thrice-weekly dialysis schedules, of ‘standard’ duration (usually 4 hours) and cannot immediately be applied to different treatment regimens. The term ‘non-conventional’ usually indicates dialysis schedules that differ from the standard ‘3 × 4’ frequency and duration, either with longer or more frequent sessions, or their combination (‘intensive dialysis’), with shorter schedules (short daily dialysis) or with shorter and less frequent sessions. When these are progressively increased to compensate for the loss of RKF, they are usually referred to by the term incremental dialysis ().
Table 4. Main ‘non-conventional’ dialysis schedules
It is worth mentioning, for the sake of completeness, decremental dialysis schedules. The term ‘decremental dialysis’ indicates a less intensive dialysis schedule usually applied to ease the transition to end-of-life care in patients on thrice weekly hemodialysis [Citation213]. However, patients started on dialysis because of acute over chronic kidney injury, who partially recover kidney function over time, may be eligible for a reduced dialysis rhythm. Attention toward residual kidney function may allow identifying these cases who might otherwise remain fully dialysis dependent.
10. Daily hemodialysis
The first intensive (daily) hemodialysis experience took place in Los Angeles in 1967 to ameliorate the clinical conditions of seven chronic hemodialysis patients doing badly on a thrice-weekly schedule, because of vascular access malfunctioning, circuit clotting, uncontrolled hypertension and severe anemia [Citation214]. Later, for the same clinical reasons, daily dialysis was started in Bologna, Italy, in six dialysis patients, leading to great clinical improvement, amelioration of nutritional indexes, improved blood pressure control and a dramatic reduction in hemotransfusion needs [Citation215]. Since the pioneering experiences, the interest in daily dialysis increased and diffused in several countries, progressively reducing the session length from the initial 4–5 hours 5 times per week to 1.5–2 hours 5–6 times per week, even adopting a flexible approach [Citation216–218]. In the eighties, Buoncristiani reported several benefits of intensive hemodialysis schedules with a follow-up lasting up to 15 years, including blood pressure control, cardiovascular outcomes, oxidative stress markers, and left ventricular function [Citation219–222]. Even if the rationale behind frequent hemodialysis is strong, the problem of adequacy quantification has been raised [Citation223]. The Frequent Hemodialysis Network Nocturnal Trial, which randomized patients to receive either thrice-weekly hemodialysis for 5 hour or less, or 5–6 dialysis sessions per week for 6 or more hours each, failed to demonstrate a survival benefit in the extended dialysis group [Citation65]. However, the trial, as well as further French and Canadian experiences, confirmed better blood pressure and phosphate control [Citation65,Citation224–226]. Conversely, intensive dialysis has been associated with a more rapid loss of RKF [Citation227].
A paradigmatic example of the clinical advantages of extended dialysis is pregnancy. It is generally agreed that, in order to cope with the increased metabolic needs of pregnancy, hemodialysis patients should be treated by daily dialysis sessions for at least 36 hours per week, as outcomes improve with the number of hours delivered [Citation228,Citation229]. The only available guidelines, proposed by the Italian Society of Nephrology, advise against the use of Kt/V to identify the ideal dialysis dose and refer to the largest Canadian experience suggesting to use pre-dialysis urea levels as a surrogate adequacy marker (namely, <100 mg/dl) [Citation230].
11. Incremental dialysis
The concept of implementing and progressively increasing the duration and frequency of the dialysis sessions to compensate for the reduction in RKF is relatively old, but its application has been limited to particular cases [Citation66,Citation231–234].
The idea that a progressive approach to hemodialysis may be advantageous has recently been systematized from the experiences in peritoneal dialysis, a mode of treatment acknowledged as having the advantage of better preserving kidney function due to a combination of lesser or no intradialytic volume and electrolyte shifts, thus avoiding phases of renal hypoperfusion. The interest in incremental dialysis is enhanced by changes in the dialysis population, which is progressively getting older and who has kidney diseases that frequently present slower progression. In parallel, the advantages of maintaining some RKF (and even just some residual diuresis) have been acknowledged: increased survival, better volume and blood pressure control, better preservation of nutritional status, due also to a more liberal diet, reduced inflammation and reduced plasma levels of middle molecules and protein-bound solutes [Citation235–241].
As already mentioned, while the example of dialysis in pregnancy demonstrates that ‘the more the better’ holds true at least in young patients with high metabolic needs, fragile and elderly patients could benefit from a ‘less is better’ approach [Citation242]. Incremental hemodialysis, challenging the dogma ‘four hours, three times a week’ [Citation68,Citation243], may offer the advantage of a ‘soft’ start of dialysis, aimed at preserving RKF and at reducing ‘dialysis shock’ [Citation227,Citation244].
Strictly speaking, an incremental approach relies on preserved RKF, and, as a consequence, Kt/V, which was developed for a standard thrice weekly schedule, mostly in anuric patients, is ‘inadequate’ as it may overestimate dialysis need [Citation35]. The rate of loss of RKF after the start of dialysis is unpredictable and residual urinary output is the first control that should be regularly performed in incremental dialysis patients [Citation245].
The first approach to overcoming this limit was the equivalent renal urea clearance, developed by Casino and Lopez, that was initially applied to patients starting incremental dialysis, but that later found application in daily treatments [Citation35]. More recently, some authors suggest considering residual renal urea clearance (Kru) in addition to Kt/V to achieve a standard weekly Kt/Vurea of 2.3, considering a Kru of 3 ml/min equivalent to a standard Kt/Vurea of 1 volume per week [Citation246]. However, this recommendation is based on the assumption that 1 ml/min of dialysis clearance is the equivalent of 1 ml/min of Kru, and this is not altogether true [Citation68]. In fact, 1 ml/min of Kru results in a greater survival benefit than 1 ml/min of dialysis urea clearance, most likely because of better removal of larger solutes and volume control [Citation247–250].
Assuming that a fixed dialysis dose should be delivered and that this is the result of the sum of dialysis clearance plus RKF, the concept of equivalent continuous clearance (ECC) could come in handy: as RKF decreases, dialysis clearance should increase to compensate [Citation35]. However, as extensively discussed, RKF and dialysis clearance are not equivalent and, given the prominent role of RKF over dialysis clearance, recently a variable target model was proposed [Citation251]. According to this model, the ECC target varies as an inverse function of residual renal urea clearance. The authors suggest that patients with a residual renal urea clearance of about 5 mL/min/35 L could start dialysis with a once weekly schedule, increasing progressively as the residual renal urea clearance decreases [Citation251].
A long list of ideal characteristics has been suggested to select a patient candidate for incremental hemodialysis. This includes a urinary output >500 mL/24 h, a Kru >3 mL/min, no fluid overload, absence or stable pharmacological control of hyperkalemia, well-controlled phosphorus levels, good nutritional status, good hemoglobin levels, infrequent hospitalization, and satisfactory health-related quality of life [Citation66].
Starting dialysis once weekly usually requires nutritional management. Patients may be kept on moderately protein-restricted diets, trying to mediate between diets that are usually prescribed in the pre-dialytic phase and the high protein intake suggested for patients on a thrice weekly schedule [Citation143]. Low protein diets, supplemented or not with ketoanalogues, have been shown to preserve RKF, possibly extending the patient’s time on incremental dialysis [Citation252,Citation253]. In this respect, wise and personalized nutritional management may be one of the keys for success. Traditionally, the nPCR has been employed to estimate the dietary protein intake in hemodialysis patients [Citation254]. Recently, a new equation has been proposed for patients on once-weekly incremental schedules [Citation255], based upon the same parameters used to estimate nPCR (pre-dialysis urea nitrogen concentration, Kt/V and the ratio between Kru and post-dialysis urea distribution volume) [Citation246]. To simplify the assessment, the authors even provided a nomogram for clinical use [Citation255].
While these elegant approaches are of interest, they do not escape from the attrition between standardization and personalization, and the authors of this review indeed employ a more personalized approach, overall exploiting the same integrated clinical criteria that are used for the start of hemodialysis, including well-being, PTH, nutritional status, extracellular volume balance, urea, signs of uremic syndrome and urea kinetic modeling whose weight and importance may differ across ages and in single cases ().
Table 5. How to monitor dialysis adequacy in incremental dialysis
12. Conclusions
According to the Merriam-Webster dictionary, something is adequate if it is sufficient or acceptable.
The challenge of personalized treatments is closely followed by the challenge of personalized monitoring. In the context of personalized dialysis, this review suggests that the answer may come from a wise combination of different biomarkers, together with regular clinical assessments. No mathematical formula or complex algorithm can replace the latter.
13. Expert opinion
If we want to tailor the prescription of renal replacement therapy to the needs of each patient, a personalized approach should be adopted also in its control. While no single biomarker allows precisely tailoring the dialysis dose, we suggest using a combination of clinical and biological markers to prescribe dialysis according to comorbidity, life expectancy, residual kidney function, and small and medium-size molecule depuration. On thrice weekly hemodialysis, Kt/V remains a valid tool to determine adequacy and, with regard to all schedules, a starting point for a global assessment of dialysis needs. However, no single objective and exhaustive marker exists: different patients have different needs, especially in a setting like hemodialysis, which has been revolutionized by technical advances and whose prevalent population has drastically changed. A comprehensive clinical assessment should guide decisions from the start of hemodialysis. At least a marker of middle molecules (B2M), calcium-phosphate and PTH balance, and nutritional status should be integrated into this clinical evaluation.
We believe that an incremental approach should be proposed to every patient who starts hemodialysis with a substantial RKF and good nutritional status. In individuals who start hemodialysis at a very old age (≥85 years) or have a very high comorbidity burden, the policy that ‘less may be better’ is probably wiser. Conversely, younger subjects, with high metabolic requirements and a transplant plan, may benefit from more intensive schedules; the paradigm of these increased needs is hemodialysis during pregnancy.
Based on our experience, we suggest performing a weekly biochemical evaluation at the beginning and at the end of the dialysis session in all incremental schedules, including at least urea, creatinine, sodium, potassium, calcium, phosphorus, bicarbonates, total proteins and albumin. Moreover, PTH, B2M, C-reactive protein and creatinine clearance should be monitored at least monthly, to modulate dialysis frequency and duration, to confirm the choice of the membrane and the dialysis technique, and to assess RKF. Dialysis prescription should be combined with nutritional evaluation, whenever possible performed by a renal dietitian; while this is usually advised at least twice yearly, in the absence of other clinical indications, in all hemodialysis patients, we suggest that frequency should be increased in patients on incremental schedules. Protein intake may be modulated according to the dialysis schedule: moderate protein restriction, similar to that prescribed in the pre-dialysis phase, could be coupled with one weekly dialysis sessions to control urea generation, phosphate intake and preserve RKF. Protein intake could be gradually liberalized by increasing dialysis frequency, eventually focusing on phosphate, potassium, and fluid intake in oligo-anuric patients.
Since an incremental approach is aimed at reducing the ‘dialysis shock,’ we suggest starting with conventional hemodialysis (HD) and low permeability membranes to limit albumin loss in patients in whom residual clearance allows natural depuration of middle molecules. Convective techniques should be preferred in patients without substantial kidney function, with well-functioning vascular access, long life-expectancy, and good nutritional status. Long dialysis vintage and dialysis-related amyloidosis are likewise indications for focusing on the depuration of middle molecules, preferring post-dilutional HDF with high permeability membranes. Patients on HDF could be switched to HD in case of vascular access malfunctioning or vice-versa. To limit albumin losses but achieve good removal of middle and inflammatory molecules, we favor pre-dilution HDF. The depuration rate for both small and middle molecules is lower in pre-dilution HDF, unless greater convective volumes are employed. This may be a disadvantage in young patients with high depuration needs. However, this may not be the case in elderly patients with low metabolic needs and high comorbidity, in whom a moderate advantage in the removal of middle molecules may be sufficient and pre-dilution HDF may allow it without increasing anticoagulation and albumin losses [Citation256–258].
When depuration needs increase as a result of a progressive loss of RRF, MCO membranes in HD may be considered before increasing the session frequency.
More intensive dialysis schedules should be employed to improve efficiency, hypertension or volume control, especially avoiding the long interdialytic interval over the weekend. Session length, and consequently the number of sessions, should be tailored to the patient’s tolerance. In a context of personalized approach, duration and number of sessions may be modulated over time, to timely adapt to the frequent clinical changes of this population ().
Article highlights
Dialysis efficiency evaluation should rely on clinical assessment and determination of different biomarkers.
No single molecule or mathematical formula can precisely determine dialysis efficiency or be used to tailor the dialysis schedule.
A combination of biomarkers reflecting small and middle molecule depuration, comorbidity and nutritional status may allow the identification of the best treatment strategy for each patient.
An incremental dialysis starts to avoid the ‘dialysis shock’ and better preserve residual renal function, should be favored in all patients without contraindications.
Convective techniques should be preferred in patients without residual kidney function, with well-functioning vascular access, long life-expectancy, and good nutritional status, especially if they have a long life expectancy.
Declaration of Interest
The authors have no relevant affiliations or financial involvement with any organization or entity with a financial interest in or financial conflict with the subject matter or materials discussed in the manuscript. This includes employment, consultancies, honoraria, stock ownership or options, expert testimony, grants or patents received or pending, or royalties.
Acknowledgments
The authors would like to acknowledge Valerie Perricone for her careful language review.
Additional information
Funding
References
- Charra B, Calemard E, Ruffet M, et al. Survival as an index of adequacy of dialysis. Kidney Int. 1992 May;41(5):1286–1291.
- Mehta AN, Fenves AZ. Hemodialysis adequacy: a review. Dialysis Trans. 2010;39(1):20–22.
- Lowrie EG, Laird NM, Parker TF, et al. Effect of the hemodialysis prescription of patient morbidity: report from the national cooperative dialysis study. N Engl J Med. 1981 Nov 12;305(20):1176–1181.
- Cheung AK, Rocco MV, Yan G, et al. Serum beta-2 microglobulin levels predict mortality in dialysis patients: results of the HEMO study. J Am Soc Nephrol. 2006 Feb;17(2):546–555.
- Daugirdas JT. Kt/V (and especially its modifications) remains a useful measure of hemodialysis dose. Kidney Int. 2015 Sep;88(3):466–473.
- Okuno S, Ishimura E, Kohno K, et al. Serum beta2-microglobulin level is a significant predictor of mortality in maintenance haemodialysis patients. Nephrol Dial Transplant. 2009 Feb;24(2):571–577.
- Kalantar-Zadeh K, Kopple JD, Block G, et al. A malnutrition-inflammation score is correlated with morbidity and mortality in maintenance hemodialysis patients. Am J Kidney Diseases. 2001 December 01;38(6):1251–1263.
- Rhee CM, Obi Y, Mathew AT, et al. Precision medicine in the transition to dialysis and personalized renal replacement therapy. Semin Nephrol. 2018 Jul;38(4):325–335.
- Cambi V, Dall’aglio P, Savazzi G, et al. Clinical assessment of haemodialysis patients with reduced small molecules removal. Proc Eur Dial Transplant Assoc. 1972;9:67–73.
- Cambi V, Savazzi G, Arisi L, et al. Short dialysis schedules (SDS)–finally ready to become routine? Proc Eur Dial Transplant Assoc. 1975;11:112–120.
- Cambi V, Arisi L, Bignardi L, et al. Preliminary results obtained with short dialysis schedules. Ateneo Parmense Acta Biomed. 1975 Sep-Oct;46(5):349–358.
- Agar JWM, Barraclough KA, Piccoli GB. Home haemodialysis: how it began, where it went wrong, and what it may yet be. J Nephrol. 2019 Jun;32(3):331–333.
- Blagg C. What went wrong with home hemodialysis in the United States and what can be done now? Hemodial Int. 2000 Jan;4(1):55–58.
- Gotch FA, Sargent JA. A mechanistic analysis of the National Cooperative Dialysis Study (NCDS). Kidney Int. 1985 Sep;28(3):526–534.
- NKF-DOQI clinical practice guidelines for hemodialysis adequacy. National kidney foundation. Am J Kidney Dis. 1997 Sep;30(3 Suppl 2):S15–66.
- Owen WF Jr., Lew NL, Liu Y, et al. The urea reduction ratio and serum albumin concentration as predictors of mortality in patients undergoing hemodialysis. N Engl J Med. 1993 Sep 30;329(14):1001–1006.
- Daugirdas JT, Smye SW. Effect of a two compartment distribution on apparent urea distribution volume. Kidney Int. 1997 Apr;51(4):1270–1273.
- Smye SW, Evans JH, Will E, et al. Paediatric haemodialysis: estimation of treatment efficiency in the presence of urea rebound. Clin Phys Physiol Meas. 1992 Feb;13(1):51–62.
- Smye SW, Dunderdale E, Brownridge G, et al. Estimation of treatment dose in high-efficiency haemodialysis. Nephron. 1994;67(1):24–29.
- Daugirdas JT, Schneditz D. Overestimation of hemodialysis dose depends on dialysis efficiency by regional blood flow but not by conventional two pool urea kinetic analysis. ASAIO J. 1995 Jul-Sep;41(3):M719–24.
- Maduell F, Garcia-Valdecasas J, Garcia H, et al. Validation of different methods to calculate Kt/V considering postdialysis rebound. Nephrol Dial Transplant. 1997 Sep;12(9):1928–1933.
- Tattersall JE, DeTakats D, Chamney P, et al. The post-hemodialysis rebound: predicting and quantifying its effect on Kt/V. Kidney Int. 1996 Dec;50(6):2094–2102.
- Casino FG, Basile C, Gaudiano V, et al. A modified algorithm of the single pool urea kinetic model. Nephrol Dial Transplant. 1990;5(3):214–219.
- Borah MF, Schoenfeld PY, Gotch FA, et al. Nitrogen balance during intermittent dialysis therapy of uremia. Kidney Int. 1978 Nov;14(5):491–500.
- Flanigan MJ, Lim VS, Redlin J. The significance of protein intake and catabolism. Adv Ren Replace Ther. 1995 Oct;2(4):330–340.
- Daugirdas JT. Second generation logarithmic estimates of single-pool variable volume Kt/V: an analysis of error. J Am Soc Nephrol. 1993Nov4;4(5):1205–1213.
- Shinaberger CS, Kilpatrick RD, Regidor DL, et al. Longitudinal associations between dietary protein intake and survival in hemodialysis patients. Am J Kidney Dis. 2006 Jul;48(1):37–49.
- Kim Y, Molnar MZ, Rattanasompattikul M, et al. Relative contributions of inflammation and inadequate protein intake to hypoalbuminemia in patients on maintenance hemodialysis. Int Urol Nephrol. 2013 Feb;45(1):215–227.
- Uribarri J. Protein catabolic rate may underestimate dietary protein intake in hemodialysis patients. Nephron. 1999 Jun;82(2):97–99.
- Ikizler TA, Burrowes JD, Byham-Gray LD, et al. KDOQI clinical practice guideline for nutrition in CKD: 2020 update. Am J Kidney Diseases. 2020;76(3):S1–S107.
- Bastani B, McNeely M, Schmitz PG. Serum bicarbonate is an independent determinant of protein catabolic rate in chronic hemodialysis. Am J Nephrol. 1996;16(5):382–385.
- Fouque D, Kalantar-Zadeh K, Kopple J, et al. A proposed nomenclature and diagnostic criteria for protein-energy wasting in acute and chronic kidney disease. Kidney Int. 2008 Feb;73(4):391–398.
- Moreau-Gaudry X, Jean G, Genet L, et al. A simple protein-energy wasting score predicts survival in maintenance hemodialysis patients. J Ren Nutr. 2014 Nov;24(6):395–400.
- Gupta N, Wish JB. Is it time for precision dialysis? Clin J Am Soc Nephrol. 2021 Feb 8;16(2):316–318.
- Casino FG, Lopez T. The equivalent renal urea clearance: a new parameter to assess dialysis dose. Nephrol Dial Transplant. 1996 Aug;11(8):1574–1581.
- Johnson WJ, Hagge WW, Wagoner RD, et al. Effects of urea loading in patients with far-advanced renal failure. Mayo Clin Proc. 1972 Jan;47(1):21–29.
- Pietrement C, Gorisse L, Jaisson S, et al. Chronic increase of urea leads to carbamylated proteins accumulation in tissues in a mouse model of CKD. PLoS One. 2013;8(12):e82506.
- Park KD, Mun KC, Chang EJ, et al. Inhibition of erythropoietin activity by cyanate. Scand J Urol Nephrol. 2004;38(1):69–72.
- Trecherel E, Godin C, Louandre C, et al. Upregulation of BAD, a pro-apoptotic protein of the BCL2 family, in vascular smooth muscle cells exposed to uremic conditions. Biochem Biophys Res Commun. 2012 Jan 6;417(1):479–483.
- Vaziri ND, Yuan J, Norris K. Role of urea in intestinal barrier dysfunction and disruption of epithelial tight junction in chronic kidney disease. Am J Nephrol. 2013;37(1):1–6.
- D’Apolito M, Du X, Zong H, et al. Urea-induced ROS generation causes insulin resistance in mice with chronic renal failure. J Clin Invest. 2010 Jan;120(1):203–213.
- Lau WL, Vaziri ND. Urea, a true uremic toxin: the empire strikes back. Clin Sci (Lond). 2017Jan1;131(1):3–12.
- Meyer TW, Sirich TL, Hostetter TH. Dialysis cannot be dosed. Semin Dial. 2011 Sep-Oct;24(5):471–479.
- Eknoyan G, Beck GJ, Cheung AK, et al. Effect of dialysis dose and membrane flux in maintenance hemodialysis. N Engl J Med. 2002 Dec 19;347(25):2010–2019.
- Paniagua R, Amato D, Vonesh E, et al. Effects of increased peritoneal clearances on mortality rates in peritoneal dialysis: ADEMEX, a prospective, randomized, controlled trial. J Am Soc Nephrol. 2002 May;13(5):1307–1320.
- Depner T, Daugirdas J, Greene T, et al. Dialysis dose and the effect of gender and body size on outcome in the HEMO Study. Kidney Int. 2004 Apr;65(4):1386–1394.
- Greene T, Daugirdas J, Depner T, et al. Association of achieved dialysis dose with mortality in the hemodialysis study: an example of “dose-targeting bias.” J Am Soc Nephrol. 2005 Nov;16(11):3371–3380.
- Lowrie EG, Li Z, Ofsthun N, et al. Body size, dialysis dose and death risk relationships among hemodialysis patients. Kidney Int. 2002 Nov;62(5):1891–1897.
- Spalding EM, Chandna SM, Davenport A, et al. Kt/V underestimates the hemodialysis dose in women and small men. Kidney Int. 2008 Aug;74(3):348–355.
- Ramirez SP, Kapke A, Port FK, et al. Dialysis dose scaled to body surface area and size-adjusted, sex-specific patient mortality. Clin J Am Soc Nephrol. 2012 Dec;7(12):1977–1987.
- Sarkar SR, Kotanko P, Heymsfeld SB, et al. Quest for V: body composition could determine dialysis dose. Semin Dial. 2007 Sep-Oct;20(5):379–382.
- Manini TM. Energy expenditure and aging. Ageing Res Rev. 2010 Jan;9(1):1–11.
- Tattersall J, Farrington K, Gentile G, et al. Is Kt/V useful in elderly dialysis patients? Pro and con arguments. Nephrol Dial Transplant. 2018 May 1;33(5):742–750.
- Maduell F, Ramos R, Palomares I, et al. Impact of targeting Kt instead of Kt/V. Nephrol Dial Transplant. 2013 Oct;28(10):2595–2603.
- Wabel P, Moissl U, Chamney P, et al. Towards improved cardiovascular management: the necessity of combining blood pressure and fluid overload. Nephrol Dial Transplant. 2008 Sep;23(9):2965–2971.
- Morgenstern BZ, Wuhl E, Nair KS, et al. Anthropometric prediction of total body water in children who are on pediatric peritoneal dialysis. J Am Soc Nephrol. 2006 Jan;17(1):285–293.
- Mendley SR, Majkowski NL, Schoeller DA. Validation of estimates of total body water in pediatric dialysis patients by deuterium dilution. Kidney Int. 2005 May;67(5):2056–2062.
- Das SK. Body composition measurement in severe obesity. Curr Opin Clin Nutr Metab Care. 2005 Nov;8(6):602–606.
- Morton AR, Singer MA. The problem with Kt/V: dialysis dose should be normalized to metabolic rate not volume. Semin Dial. 2007 Jan-Feb;20(1):12–15.
- Lowrie EG, Li Z, Ofsthun N, et al. The online measurement of hemodialysis dose (Kt): clinical outcome as a function of body surface area. Kidney Int. 2005 Sep;68(3):1344–1354.
- Daugirdas JT, Levin NW, Kotanko P, et al. Comparison of proposed alternative methods for rescaling dialysis dose: resting energy expenditure, high metabolic rate organ mass, liver size, and body surface area. Semin Dial. 2008 Sep-Oct;21(5):377–384.
- Lowrie EG, Chertow GM, Lew NL, et al. The urea [clearance x dialysis time] product (Kt) as an outcome-based measure of hemodialysis dose. Kidney Int. 1999 Aug;56(2):729–737.
- National Kidney Foundation. KDOQI clinical practice guideline for hemodialysis adequacy: 2015 update. Am J Kidney Dis. 2015; 66 (5):884–930.
- Saran R, Bragg-Gresham JL, Levin NW, et al. Longer treatment time and slower ultrafiltration in hemodialysis: associations with reduced mortality in the DOPPS. Kidney Int. 2006 Apr;69(7):1222–1228.
- Rocco MV, Lockridge RS Jr., Beck GJ, et al. The effects of frequent nocturnal home hemodialysis: the frequent hemodialysis network nocturnal trial. Kidney Int. 2011 Nov;80(10):1080–1091.
- Kalantar-Zadeh K, Unruh M, Zager PG, et al. Twice-weekly and incremental hemodialysis treatment for initiation of kidney replacement therapy. Am J Kidney Dis. 2014 Aug;64(2):181–186.
- Wong J, Vilar E, Davenport A, et al. Incremental haemodialysis. Nephrol Dial Transplant. 2015 Oct;30(10):1639–1648.
- Basile C, Casino FG, Kalantar-Zadeh K. Is incremental hemodialysis ready to return on the scene? From empiricism to kinetic modelling. J Nephrol. 2017 Aug;30(4):521–529.
- Termorshuizen F, Dekker FW, van Manen JG, et al. Relative contribution of residual renal function and different measures of adequacy to survival in hemodialysis patients: an analysis of the Netherlands Cooperative Study on the Adequacy of Dialysis (NECOSAD)-2. J Am Soc Nephrol. 2004 Apr;15(4):1061–1070.
- Obi Y, Rhee CM, Mathew AT, et al. Residual kidney function decline and mortality in incident hemodialysis patients. J Am Soc Nephrol. 2016 Dec;27(12):3758–3768.
- Mathew AT, Fishbane S, Obi Y, et al. Preservation of residual kidney function in hemodialysis patients: reviving an old concept. Kidney Int. 2016 Aug;90(2):262–271.
- Eloot S, Van Biesen W, Glorieux G, et al. Does the adequacy parameter Kt/V(urea) reflect uremic toxin concentrations in hemodialysis patients? PLoS One. 2013;8(11):e76838.
- Parker TF 3rd, Husni L, Huang W, et al. Survival of hemodialysis patients in the United States is improved with a greater quantity of dialysis. Am J Kidney Dis. 1994 May;23(5):670–680.
- Vanholder R, Argiles A, Jankowski J, et al. A history of uremic toxicity and of the European Uremic Toxin work group (EUTox). Clin Kidney J. 2021. https://doi.org/10.1093/ckj/sfab011.
- Vanholder R, De Smet R, Glorieux G, et al. Review on uremic toxins: classification, concentration, and interindividual variability. Kidney Int. 2003 May;63(5):1934–1943.
- Wolley MJ, Hutchison CA. Large uremic toxins: an unsolved problem in end-stage kidney disease. Nephrol Dial Transplant. 2018 Oct 1;33(suppl_3):iii6–iii11.
- Wu P-H, Lin Y-T, Chiu Y-W, et al. The relationship of indoxyl sulfate and p-cresyl sulfate with target cardiovascular proteins in hemodialysis patients. Sci Rep. 2021 February 15;11(1):3786.
- Duranton F, Cohen G, De Smet R, et al. Normal and pathologic concentrations of uremic toxins. J Am Soc Nephrol. 2012 Jul;23(7):1258–1270.
- Kidney Disease: Improving Global Outcomes (KDIGO) CKD-MBD Update Work Group. KDIGO 2017 clinical practice guideline update for the diagnosis, evaluation, prevention, and treatment of Chronic Kidney Disease–Mineral and Bone Disorder (CKD-MBD). Kidney Int Suppl. 2017;2017(7):1–59.
- Fukagawa M, Yokoyama K, Koiwa F, et al. Clinical practice guideline for the management of chronic kidney disease-mineral and bone disorder. Ther Apher Dial. 2013 Jun;17(3):247–288.
- Duque EJ, Elias RM, Moysés RMA. Parathyroid Hormone: a Uremic Toxin. Toxins (Basel). 2020;12(3):189.
- Zhan Z, Smyth B, Toussaint ND, et al. Effect of extended hours dialysis on markers of chronic kidney disease-mineral and bone disorder in the ACTIVE Dialysis study. BMC Nephrol. 2019 Jul 12;20(1):258.
- Ferraresi M, Pia A, Guzzo G, et al. Calcium-phosphate and parathyroid intradialytic profiles: a potential aid for tailoring the dialysate calcium content of patients on different hemodialysis schedules. Hemodialysis Int. 2015;19(4):572–582.
- Masakane I, Sakurai K. Current approaches to middle molecule removal: room for innovation. Nephrol Dial Transplant. 2018Oct1;33(suppl_3):iii12–iii21.
- Gejyo F, Yamada T, Odani S, et al. A new form of amyloid protein associated with chronic hemodialysis was identified as beta 2-microglobulin. Biochem Biophys Res Commun. 1985 Jun 28;129(3):701–706.
- European Best Practice Guidelines Expert Group on Hemodialysis ERA. II.2 Haemodialysis dose quantification: middle molecules (MM). Nephrol Dialysis Transplantation. 2002;17(suppl_7):21–23.
- Roumelioti ME, Trietley G, Nolin TD, et al. Beta-2 microglobulin clearance in high-flux dialysis and convective dialysis modalities: a meta-analysis of published studies. Nephrol Dial Transplant. 2018 Jun 1;33(6):1025–1039.
- Eloot S, Van Biesen W, Dhondt A, et al. Impact of hemodialysis duration on the removal of uremic retention solutes. Kidney Int. 2008 Mar;73(6):765–770.
- Santoro A, Mancini E, Bolzani R, et al. The effect of on-line high-flux hemofiltration versus low-flux hemodialysis on mortality in chronic kidney failure: a small randomized controlled trial. Am J Kidney Dis. 2008 Sep;52(3):507–518.
- Locatelli F, Martin-Malo A, Hannedouche T, et al. Effect of membrane permeability on survival of hemodialysis patients. J Am Soc Nephrol. 2009 Mar;20(3):645–654.
- Asci G, Tz H, Ozkahya M, et al. The impact of membrane permeability and dialysate purity on cardiovascular outcomes. J Am Soc Nephrol. 2013 May;24(6):1014–1023.
- Locatelli F, Martin-Malo A, Hannedouche T, et al. Effect of membrane permeability on survival of hemodialysis patients. J Am Soc Nephrol. 2009 Mar;20(3):645–654.
- Watanabe Y, Kawanishi H, Suzuki K, et al. Japanese society for dialysis therapy clinical guideline for “Maintenance hemodialysis: hemodialysis prescriptions.” Ther Apher Dial. 2015 Mar;19(Suppl 1):67–92.
- Haroon S, Davenport A. Choosing a dialyzer: what clinicians need to know. Hemodial Int. 2018 Oct;22(S2):S65–S74.
- Jaques DA, Davenport A. Serum beta2-microglobulin as a predictor of residual kidney function in peritoneal dialysis patients. J Nephrol. 2021 Apr;34(2):473–481.
- Kirsch AH, Lyko R, Nilsson LG, et al. Performance of hemodialysis with novel medium cut-off dialyzers. Nephrol Dial Transplant. 2017 Jan 1;32(1):165–172.
- Oshihara W, Nagao H, Megano H, et al. Trial use of a polymethylmethacrylate membrane for the removal of free immunoglobulin light chains in dialysis patients. NDT Plus. 2010 May;3(Suppl 1):i3–i7.
- Hutchison CA, Cockwell P, Reid S, et al. Efficient removal of immunoglobulin free light chains by hemodialysis for multiple myeloma: in Vitro and In Vivo studies. J Am Soc Nephrol. 2007;18(3):886–895.
- Vanholder R, Schepers E, Pletinck A, et al. The uremic toxicity of indoxyl sulfate and p-cresyl sulfate: a systematic review. J Am Soc Nephrol. 2014 Sep;25(9):1897–1907.
- Sirich TL, Luo FJ, Plummer NS, et al. Selectively increasing the clearance of protein-bound uremic solutes. Nephrol Dial Transplant. 2012 Apr;27(4):1574–1579.
- Rysz J, Franczyk B, Lawinski J, et al. The Impact of CKD on uremic toxins and gut microbiota. Toxins (Basel). 2021 Mar 31;13:4.
- Poesen R, Windey K, Neven E, et al. The Influence of CKD on colonic microbial metabolism. J Am Soc Nephrol. 2016 May;27(5):1389–1399.
- Wang X, Yang S, Li S, et al. Aberrant gut microbiota alters host metabolome and impacts renal failure in humans and rodents. Gut. 2020 Dec;69(12):2131–2142.
- Wong J, Piceno YM, DeSantis TZ, et al. Expansion of urease- and uricase-containing, indole- and p-cresol-forming and contraction of short-chain fatty acid-producing intestinal microbiota in ESRD. Am J Nephrol. 2014;39(3):230–237.
- Yang T, Richards EM, Pepine CJ, et al. The gut microbiota and the brain-gut-kidney axis in hypertension and chronic kidney disease. Nat Rev Nephrol. 2018 Jul;14(7):442–456.
- Koppe L, Pillon NJ, Vella RE, et al. p-Cresyl sulfate promotes insulin resistance associated with CKD. J Am Soc Nephrol. 2013 Jan;24(1):88–99.
- Ito S, Yoshida M. Protein-bound uremic toxins: new culprits of cardiovascular events in chronic kidney disease patients. Toxins (Basel). 2014 Feb 20;6(2):665–678.
- Cupisti A, Piccoli GB, Gallieni M. Charcoal for the management of pruritus and uremic toxins in patients with chronic kidney disease. Curr Opin Nephrol Hypertens. 2020 Jan;29(1):71–79.
- Wu IW, Hsu KH, Hsu HJ, et al. Serum free p-cresyl sulfate levels predict cardiovascular and all-cause mortality in elderly hemodialysis patients–a prospective cohort study. Nephrol Dial Transplant. 2012 Mar;27(3):1169–1175.
- Barreto FC, Barreto DV, Liabeuf S, et al. Serum indoxyl sulfate is associated with vascular disease and mortality in chronic kidney disease patients. Clin J Am Soc Nephrol. 2009 Oct;4(10):1551–1558.
- Meijers BK, De Loor H, Bammens B, et al. p-Cresyl sulfate and indoxyl sulfate in hemodialysis patients. Clin J Am Soc Nephrol. 2009 Dec;4(12):1932–1938.
- Lin CN, Wu IW, Huang YF, et al. Measuring serum total and free indoxyl sulfate and p-cresyl sulfate in chronic kidney disease using UPLC-MS/MS. J Food Drug Anal. 2019 Apr;27(2):502–509.
- Locatelli F, Fouque D, Heimburger O, et al. Nutritional status in dialysis patients: a European consensus. Nephrol Dial Transplant. 2002 Apr;17(4):563–572.
- Clinical practice guidelines for nutrition in chronic renal failure. K/DOQI, national kidney foundation. Am J Kidney Dis. 2000 Jun;35(6Suppl 2):S1–140.
- van de Logt AE, Rijpma SR, Vink CH, et al. The bias between different albumin assays may affect clinical decision-making. Kidney Int. 2019 Jun;95(6):1514–1517.
- Friedman AN, Fadem SZ. Reassessment of albumin as a nutritional marker in kidney disease. J Am Soc Nephrol. 2010 Feb;21(2):223–230.
- De Mutsert R, Grootendorst DC, Indemans F, et al. Association between serum albumin and mortality in dialysis patients is partly explained by inflammation, and not by malnutrition. J Ren Nutr. 2009 Mar;19(2):127–135.
- Kaysen GA, Dubin JA, Muller HG, et al. Inflammation and reduced albumin synthesis associated with stable decline in serum albumin in hemodialysis patients. Kidney Int. 2004 Apr;65(4):1408–1415.
- Kopple JD. McCollum Award Lecture, 1996: protein-energy malnutrition in maintenance dialysis patients. Am J Clin Nutr. 1997 May;65(5):1544–1557.
- Mehrotra R, Kopple JD. Nutritional management of maintenance dialysis patients: why aren’t we doing better? Annu Rev Nutr. 2001;21:343–379.
- Kalantar-Zadeh K, Ikizler TA, Block G, et al. Malnutrition-inflammation complex syndrome in dialysis patients: causes and consequences. Am J Kidney Dis. 2003 Nov;42(5):864–881.
- Oliveira EA, Zheng R, Carter CE, et al. Cachexia/Protein energy wasting syndrome in CKD: causation and treatment. Semin Dial. 2019 Nov;32(6):493–499.
- Piccoli G, Bonello F, Massara C, et al. Death in conditions of cachexia: the price for the dialysis treatment of the elderly? Kidney Int Suppl. 1993;41:S282–6.
- Obi Y, Qader H, Kovesdy CP, et al. Latest consensus and update on protein-energy wasting in chronic kidney disease. Curr Opin Clin Nutr Metab Care. 2015 May;18(3):254–262.
- Beddhu S, Pappas LM, Ramkumar N, et al. Malnutrition and atherosclerosis in dialysis patients. J Am Soc Nephrol. 2004 Mar;15(3):733–742.
- Pecoits-Filho R, Lindholm B, Stenvinkel P. The malnutrition, inflammation, and atherosclerosis (MIA) syndrome – the heart of the matter. Nephrol Dial Transplant. 2002;17(Suppl 11):28–31.
- Maraj M, Kuśnierz-Cabala B, Dumnicka P, et al. Malnutrition, Inflammation, Atherosclerosis Syndrome (MIA) and diet recommendations among end-stage renal disease patients treated with maintenance hemodialysis. Nutrients. 2018;10(1):69.
- Koppe L, Fouque D, Kalantar-Zadeh K. Kidney cachexia or protein-energy wasting in chronic kidney disease: facts and numbers. J Cachexia Sarcopenia Muscle. 2019 Jun;10(3):479–484.
- Carrero JJ, Stenvinkel P, Cuppari L, et al. Etiology of the protein-energy wasting syndrome in chronic kidney disease: a consensus statement from the International Society of Renal Nutrition and Metabolism (ISRNM). J Ren Nutr. 2013 Mar;23(2):77–90.
- Anton-Perez G, Santana-Del-Pino A, Henriquez-Palop F, et al. Diagnostic usefulness of the protein energy wasting score in prevalent hemodialysis patients. J Ren Nutr. 2018 Nov;28(6):428–434.
- Santoro A, Mancini E. Hemodialysis and the elderly patient: complications and concerns. J Nephrol. 2010 Sep-Oct;23(Suppl 15):S80–9.
- Abdulan IM, Onofriescu M, Stefaniu R, et al. The predictive value of malnutrition for functional and cognitive status in elderly hemodialysis patients. Int Urol Nephrol. 2019 Jan;51(1):155–162.
- Piccoli GB, Mezza E, Anania P, et al. Patients on renal replacement therapy for 20 or more years: a clinical profile. Nephrol Dial Transplant. 2002 Aug;17(8):1440–1449.
- Omari AM, Omari LS, Dagash HH, et al. Assessment of nutritional status in the maintenance of haemodialysis patients: a cross-sectional study from Palestine. BMC Nephrol. 2019 Mar 15;20(1):92.
- Kittiskulnam P, Chertow GM, Carrero JJ, et al. Sarcopenia and its individual criteria are associated, in part, with mortality among patients on hemodialysis. Kidney Int. 2017 Jul;92(1):238–247.
- Malhotra R, Deger SM, Salat H, et al. Sarcopenic obesity definitions by body composition and mortality in the hemodialysis patients. J Ren Nutr. 2017 Mar;27(2):84–90.
- Kittiskulnam P, Carrero JJ, Chertow GM, et al. Sarcopenia among patients receiving hemodialysis: weighing the evidence. J Cachexia Sarcopenia Muscle. 2017;8(1):57–68.
- Sabatino A, Cuppari L, and Stenvinkel P, et al. Sarcopenia in chronic kidney disease: what have we learned so far? J Nephrol. 2021 34;1347–1372 Sep 2.
- Riella MC. Nutritional evaluation of patients receiving dialysis for the management of protein-energy wasting: what is old and what is new? J Ren Nutr. 2013 May;23(3):195–198.
- Rodrigues J, Santin F, Brito F, et al. Nutritional status of older patients on hemodialysis: which nutritional markers can best predict clinical outcomes? Nutrition. 2019 Sep;65:113–119.
- Chumlea WC, Dwyer J, Bergen C, et al. Nutritional status assessed from anthropometric measures in the HEMO study. J Ren Nutr. 2003 Jan;13(1):31–38.
- Enia G, Sicuso C, Alati G, et al. Subjective global assessment of nutrition in dialysis patients. Nephrol Dialysis Transplantation. 1993;8(10):1094–1098.
- Piccoli GB, Lippi F, Fois A, et al. Intradialytic nutrition and hemodialysis prescriptions: a personalized stepwise approach. Nutrients. 2020;12(3):785.
- Eriguchi R, Obi Y, Streja E, et al. Longitudinal associations among renal urea clearance–corrected normalized protein catabolic rate, serum albumin, and mortality in patients on hemodialysis. Clin J Am Soc Nephrol. 2017;12(7):1109–1117.
- Susantitaphong P, Siribamrungwong M, Jaber BL. Convective therapies versus low-flux hemodialysis for chronic kidney failure: a meta-analysis of randomized controlled trials. Nephrol Dialysis Transplantation. 2013;28(11):2859–2874.
- Kalantar-Zadeh K, Ficociello LH, Bazzanella J, et al. Slipping through the pores: hypoalbuminemia and albumin loss during hemodialysis. Int J Nephrol Renovasc Dis. 2021;14:11–21.
- Tsuchida K, Minakuchi J. Albumin loss under the use of the high-performance membrane. Contrib Nephrol. 2011;173:76–83.
- Krieter DH, Canaud B. High permeability of dialysis membranes: what is the limit of albumin loss? Nephrol Dial Transplant. 2003 Apr;18(4):651–654.
- Canaud B, Bragg-Gresham JL, Marshall MR, et al. Mortality risk for patients receiving hemodiafiltration versus hemodialysis: European results from the DOPPS. Kidney Int. 2006 Jun;69(11):2087–2093.
- Tomisawa N, Yamashita AC. Amount of adsorbed albumin loss by dialysis membranes with protein adsorption. J Artif Organs. 2009;12(3):194–199.
- Ronco C, Marchionna N, Brendolan A, et al. Expanded haemodialysis: from operational mechanism to clinical results. Nephrol Dialysis Transplantation. 2018;33(suppl_3):iii41–iii47.
- van Gelder MK, Abrahams AC, Joles JA, et al. Albumin handling in different hemodialysis modalities. Nephrol Dial Transplant. 2018 Jun 1;33(6):906–913.
- Hutchison CA, Harding S, Mead G, et al. Serum free-light chain removal by high cutoff hemodialysis: optimizing removal and supportive care. Artif Organs. 2008 Dec;32(12):910–917.
- Cho NJ, Park S, Islam MI, et al. Long-term effect of medium cut-off dialyzer on middle uremic toxins and cell-free hemoglobin. PLoS One. 2019;14(7):e0220448.
- Boschetti-de-Fierro A, Voigt M, Storr M, et al. MCO membranes: enhanced selectivity in high-flux class. Sci Rep. 2015 Dec;16(5):18448.
- Belmouaz M, Diolez J, Bauwens M, et al. Comparison of hemodialysis with medium cut-off dialyzer and on-line hemodiafiltration on the removal of small and middle-sized molecules. Clin Nephrol. 2018 Jan;89(1):50–56.
- Mercadal L, Franck JE, Metzger M, et al. Hemodiafiltration versus hemodialysis and survival in patients with ESRD: the French Renal Epidemiology and Information Network (REIN) registry. Am J Kidney Dis. 2016 Aug;68(2):247–255.
- Peters SA, Bots ML, Canaud B, et al. Haemodiafiltration and mortality in end-stage kidney disease patients: a pooled individual participant data analysis from four randomized controlled trials. Nephrol Dial Transplant. 2016 Jun;31(6):978–984.
- Nistor I, Palmer SC, Craig JC, et al. Haemodiafiltration, haemofiltration and haemodialysis for end-stage kidney disease. Cochrane Database Syst Rev. 2015 May;20(5):Cd006258.
- Vega A, Quiroga B, Abad S, et al. Albumin leakage in online hemodiafiltration, more convective transport, more losses? Ther Apher Dial. 2015 Jun;19(3):267–271.
- Ok E, Asci G, Toz H, et al. Mortality and cardiovascular events in online haemodiafiltration (OL-HDF) compared with high-flux dialysis: results from the Turkish OL-HDF Study. Nephrol Dialysis Transplantation. 2012;28(1):192–202.
- Morena M, Jaussent A, Chalabi L, et al. Treatment tolerance and patient-reported outcomes favor online hemodiafiltration compared to high-flux hemodialysis in the elderly. Kidney Int. 2017;91(6):1495–1509.
- Piccoli GB, Cabiddu G, Moio MR, et al. Efficiency and nutritional parameters in an elderly high risk population on hemodialysis and hemodiafiltration in Italy and France: different treatments with similar names? BMC Nephrol. 2018 Jul 9;19(1):171.
- Tsuchida K, Minakuchi J. Effect of large-size dialysis membrane and hemofiltration/hemodiafiltration methods on long-term dialysis patients. Contrib Nephrol. 2011;168:179–187.
- Hoshino J, Yamagata K, Nishi S, et al. Significance of the decreased risk of dialysis-related amyloidosis now proven by results from Japanese nationwide surveys in 1998 and 2010. Nephrol Dial Transplant. 2016 Apr;31(4):595–602.
- Masakane I. How to prescribe hemodialysis or hemodiafiltration in order to ameliorate dialysis-related symptoms and complications. Contrib Nephrol. 2011;168:53–63.
- Schiffl H. Online hemodiafiltration and mortality risk in end-stage renal disease patients: a critical appraisal of current evidence. Kidney Res Clin Pract. 2019;38(2):159–168.
- Locatelli F, Karaboyas A, Pisoni RL, et al. Mortality risk in patients on hemodiafiltration versus hemodialysis: a ‘real-world’ comparison from the DOPPS. Nephrol Dialysis Trans. 2018;33(4):683–689.
- Locatelli F, Carfagna F, Del Vecchio L, et al. Haemodialysis or haemodiafiltration: that is the question. Nephrol Dial Transplant. 2018 Nov 1;33(11):1896–1904.
- Locatelli F, Di Filippo S, Pontoriero G. Fluid and electrolyte balance during extracorporeal therapies. In: Ronco C, Bellomo R, editors. Critical care nephrology. Dordrecht: Springer Netherlands; 1998. p. 249–259.
- Block GA, Hulbert-Shearon TE, Levin NW, et al. Association of serum phosphorus and calcium x phosphate product with mortality risk in chronic hemodialysis patients: a national study. Am J Kidney Dis. 1998 Apr;31(4):607–617.
- Young EW, Albert JM, Satayathum S, et al. Predictors and consequences of altered mineral metabolism: the Dialysis Outcomes and Practice Patterns Study. Kidney Int. 2005 Mar;67(3):1179–1187.
- Cozzolino M, Ciceri P, Galassi A, et al. The key role of phosphate on vascular calcification. Toxins (Basel). 2019;11(4):213.
- Tentori F, Blayney MJ, Albert JM, et al. Mortality risk for dialysis patients with different levels of serum calcium, phosphorus, and PTH: the Dialysis Outcomes and Practice Patterns Study (DOPPS). Am J Kidney Dis. 2008 Sep;52(3):519–530.
- Bover J, Urena P, Brandenburg V, et al. Adynamic bone disease: from bone to vessels in chronic kidney disease. Semin Nephrol. 2014 Nov;34(6):626–640.
- Messa P, Alfieri CM. Secondary and tertiary hyperparathyroidism. Front Horm Res. 2019;51:91–108.
- Vilar E, Fry AC, Wellsted D, et al. Long-term outcomes in online hemodiafiltration and high-flux hemodialysis: a comparative analysis. Clin J Am Soc Nephrol. 2009;4(12):1944–1953.
- Daugirdas JT, Chertow GM, Larive B, et al. Effects of frequent hemodialysis on measures of CKD mineral and bone disorder. J Am Soc Nephrol. 2012 Apr;23(4):727–738.
- D’Alessandro C, Piccoli GB, Cupisti A. The “phosphorus pyramid”: a visual tool for dietary phosphate management in dialysis and CKD patients. BMC Nephrol. 2015 Jan;20(16):9.
- Ruospo M, Palmer SC, Natale P, et al. Phosphate binders for preventing and treating chronic kidney disease-mineral and bone disorder (CKD-MBD). Cochrane Database Syst Rev. 2018 Aug 22;8(8):Cd006023.
- Barreto FC, Barreto DV, Massy ZA, et al. Strategies for phosphate control in patients with CKD. Kidney Int Rep. 2019;4(8):1043–1056.
- Shen J, Li W, Wang Y, et al. Higher serum phosphorus predicts residual renal function loss in male but not female incident peritoneal dialysis patients. J Nephrol. 2020 Aug;33(4):829–837.
- Zoccali C, Moissl U, Chazot C, et al. Chronic fluid overload and mortality in ESRD. J Am Soc Nephrol. 2017 Aug;28(8):2491–2497.
- Beerendrakumar N, Ramamoorthy L, Haridasan S. Dietary and fluid regime adherence in chronic kidney disease patients. J Caring Sci. 2018;7(1):17–20.
- Ozen N, Cinar FI, Askin D, et al. Nonadherence in hemodialysis patients and related factors: a multicenter study. J Nurs Res. 2019;27(4):e36.
- Assimon MM, Wenger JB, Wang L, et al. Ultrafiltration rate and mortality in maintenance hemodialysis patients. Am J Kidney Dis. 2016;68(6):911–922.
- Kim TW, Chang TI, Kim TH, et al. Association of ultrafiltration rate with mortality in incident hemodialysis patients. Nephron. 2018;139(1):13–22.
- Chou JA, Streja E, Nguyen DV, et al. Intradialytic hypotension, blood pressure changes and mortality risk in incident hemodialysis patients. Nephrol Dial Transplant. 2018 Jan 1;33(1):149–159.
- Yu J, Liu Z, Shen B, et al. Intradialytic hypotension as an independent risk factor for long-term mortality in maintaining hemodialysis patients: a 5-Year follow-up cohort study. Blood Purif. 2018;45(4):320–326.
- Sars B, van der Sande FM, Kooman JP. Intradialytic hypotension: mechanisms and outcome. Blood Purif. 2020;49(1–2):158–167.
- Chang TI, Paik J, Greene T, et al. Intradialytic hypotension and vascular access thrombosis. J Am Soc Nephrol. 2011 Aug;22(8):1526–1533.
- Burton JO, Jefferies HJ, Selby NM, et al. Hemodialysis-induced cardiac injury: determinants and associated outcomes. Clin J Am Soc Nephrol. 2009;4(5):914–920.
- Davenport A, Cox C, Thuraisingham R. Achieving blood pressure targets during dialysis improves control but increases intradialytic hypotension. Kidney Int. 2008;73(6):759–764.
- Rocha A, Sousa C, Teles P, et al. Effect of dialysis day on intradialytic hypotension risk. Kidney Blood Press Res. 2016;41(2):168–174.
- Kuipers J, Oosterhuis JK, Paans W, et al. Association between quality of life and various aspects of intradialytic hypotension including patient-reported intradialytic symptom score. BMC Nephrol. 2019 May 14;20(1):164.
- Jansen MA, Hart AA, Korevaar JC, et al. Predictors of the rate of decline of residual renal function in incident dialysis patients. Kidney Int. 2002 Sep;62(3):1046–1053.
- Santoro A, Mancini E, Basile C, et al. Blood volume controlled hemodialysis in hypotension-prone patients: a randomized, multicenter controlled trial. Kidney Int. 2002 Sep;62(3):1034–1045.
- MacEwen C, Sutherland S, Daly J, et al. Relationship between Hypotension and Cerebral Ischemia during Hemodialysis. J Am Soc Nephrol. 2017 Aug;28(8):2511–2520.
- van der Sande FM, Cheriex EC, van Kuijk WH, et al. Effect of dialysate calcium concentrations on intradialytic blood pressure course in cardiac-compromised patients. Am J Kidney Dis. 1998 Jul;32(1):125–131.
- Penne EL, Sergeyeva O. Sodium gradient: a tool to individualize dialysate sodium prescription in chronic hemodialysis patients? Blood Purif. 2011;31(1–3):86–91.
- Keen ML, Gotch FA. The association of the sodium “setpoint” to interdialytic weight gain and blood pressure in hemodialysis patients. Int J Artif Organs. 2007 Nov;30(11):971–979.
- Kotanko P, Garg AX, Depner T, et al. Effects of frequent hemodialysis on blood pressure: results from the randomized frequent hemodialysis network trials. Hemodial Int. 2015 Jul;19(3):386–401.
- Leung KC, Quinn RR, Ravani P, et al. Ultrafiltration biofeedback guided by blood volume monitoring to reduce intradialytic hypotensive episodes in hemodialysis: study protocol for a randomized controlled trial. Trials. 2014 Dec;10(15):483.
- Reddan DN, Szczech LA, Hasselblad V, et al. Intradialytic blood volume monitoring in ambulatory hemodialysis patients: a randomized trial. J Am Soc Nephrol. 2005 Jul;16(7):2162–2169.
- Hur E, Usta M, Toz H, et al. Effect of fluid management guided by bioimpedance spectroscopy on cardiovascular parameters in hemodialysis patients: a randomized controlled trial. Am J Kidney Dis. 2013 Jun;61(6):957–965.
- Kanbay M, Ertuglu LA, Afsar B, et al. An update review of intradialytic hypotension: concept, risk factors, clinical implications and management. Clin Kidney J. 2020;13(6):981–993.
- Locatelli F, Altieri P, Andrulli S, et al. Hemofiltration and hemodiafiltration reduce intradialytic hypotension in ESRD. J Am Soc Nephrol. 2010;21(10):1798–1807.
- Tessitore N, Santoro A, Panzetta GO, et al. Acetate-free biofiltration reduces intradialytic hypotension: a European multicenter randomized controlled trial. Blood Purif. 2012;34(3–4):354–363.
- Petrović DG. Influence of acetate-free biofiltration on intradialytic cardiovascular stability. Dialysis Trans. 2006;35(6):376–384.
- Adamczak M, Masajtis-Zagajewska A, Mazanowska O, et al. Diagnosis and treatment of metabolic acidosis in patients with chronic kidney disease - position statement of the working group of the polish society of nephrology. Kidney Blood Press Res. 2018;43(3):959–969.
- Wu DY, Shinaberger CS, Regidor DL, et al. Association between serum bicarbonate and death in hemodialysis patients: is it better to be acidotic or alkalotic? Clin J Am Soc Nephrol. 2006 Jan 1;1:70–78.
- Yamamoto T, Shoji S, Yamakawa T, et al. Predialysis and Postdialysis pH and Bicarbonate and risk of all-cause and cardiovascular mortality in long-term hemodialysis patients. Am J Kidney Dis. 2015 Sep;66(3):469–478.
- Murea M. Precision medicine approach to dialysis including incremental and decremental dialysis regimens. Curr Opin Nephrol Hypertens. 2021;30(1):85–92.
- DePalma JR, Pecker EA, Maxwell MH. A new automatic coil dialyzer system for ‘daily’ dialysis. Hemodial Int. 2004 Jan 1;8(1):19–23.
- Bonomini V, Mioli V, Albertazzi A, et al. Daily-dialysis programme: indications and results. Proc Eur Dial Transplant Assoc. 1972;9:44–52.
- Kjellstrand CM, Brief A. History of daily hemodialysis. Home Hemodial Int (1997). 1998 Jan;2(1):8–11.
- Suri RS, Nesrallah GE, Mainra R, et al. Daily hemodialysis: a systematic review. Clin J Am Soc Nephrol. 2006 1; Jan(1): 33–42.
- Piccoli GB, Calderini M, Bechis F, et al. Daily dialysis Kt/V and flexible schedules: is it possible to control efficiency, when and how? Int J Artif Organs. 2001 Jun;24(6):347–356.
- Buoncristiani U, Quintaliani G, Cozzari M, et al. Daily dialysis: long-term clinical metabolic results. Kidney Int Suppl. 1988 Mar;24:S137–40.
- Buoncristiani U, Fagugli RM, Pinciaroli MR, et al. Reversal of left-ventricular hypertrophy in uremic patients by treatment with daily hemodialysis (DHD). Contrib Nephrol. 1996;119:152–156.
- Fagugli RM, Reboldi G, Quintaliani G, et al. Short daily hemodialysis: blood pressure control and left ventricular mass reduction in hypertensive hemodialysis patients. Am J Kidney Dis. 2001 Aug;38(2):371–376.
- Floridi A, Antolini F, Galli F, et al. Daily haemodialysis improves indices of protein glycation. Nephrol Dial Transplant. 2002 May;17(5):871–878.
- Buoncristiani U, Fagugli R, Quintaliani G, et al. Rationale for daily dialysis. Home Hemodial Int (1997). 1997 1; Jan(1): 12–18.
- Culleton BF, Walsh M, Klarenbach SW, et al. Effect of frequent nocturnal hemodialysis vs conventional hemodialysis on left ventricular mass and quality of life: a randomized controlled trial. JAMA. 2007 Sep 19;298(11):1291–1299.
- Traeger J, Galland R, Delawari E, et al. Six years’ experience with short daily hemodialysis: do the early improvements persist in the mid and long term? Hemodial Int. 2004 Apr 1;8(2):151–158.
- Charra B, Terrat JC, Vanel T, et al. Long thrice weekly hemodialysis: the Tassin experience. Int J Artif Organs. 2004 Apr;27(4):265–283.
- Daugirdas JT, Greene T, Rocco MV, et al. Effect of frequent hemodialysis on residual kidney function. Kidney Int. 2013 May;83(5):949–958.
- Hladunewich MA, Hou S, Odutayo A, et al. Intensive hemodialysis associates with improved pregnancy outcomes: a Canadian and United States cohort comparison. J Am Soc Nephrol. 2014 May;25(5):1103–1109.
- Barua M, Hladunewich M, Keunen J, et al. Successful pregnancies on nocturnal home hemodialysis. Clin J Am Soc Nephrol. 2008 3; Mar(2): 392–396.
- Cabiddu G, Castellino S, Gernone G, et al. Best practices on pregnancy on dialysis: the Italian study group on kidney and pregnancy. J Nephrol. 2015 Jun;28(3):279–288.
- Caria S, Cupisti A, Sau G, et al. The incremental treatment of ESRD: a low-protein diet combined with weekly hemodialysis may be beneficial for selected patients. BMC Nephrol. 2014 Oct;29(15):172.
- Bolasco P, Cupisti A, Locatelli F, et al. Dietary management of incremental transition to dialysis therapy: once-weekly hemodialysis combined with low-protein diet. J Ren Nutr. 2016 Nov;26(6):352–359.
- Kalantar-Zadeh K, Casino FG. Let us give twice-weekly hemodialysis a chance: revisiting the taboo. Nephrol Dial Transplant. 2014 Sep;29(9):1618–1620.
- Libetta C, Esposito P, Dal Canton A. Once-weekly hemodialysis: a single-center experience. Am J Kidney Dis. 2015 Feb;65(2):343.
- Kabanda A, Jadoul M, Pochet JM, et al. Determinants of the serum concentrations of low molecular weight proteins in patients on maintenance hemodialysis. Kidney Int. 1994 Jun;45(6):1689–1696.
- Marquez IO, Tambra S, Luo FY, et al. Contribution of residual function to removal of protein-bound solutes in hemodialysis. Clin J Am Soc Nephrol. 2011 Feb;6(2):290–296.
- Delaney MP, Stevens PE, Al Hasani M, et al. Relationship of serum cystatin C to peritoneal and renal clearance measures in peritoneal dialysis: a cross-sectional study. Am J Kidney Dis. 2008 Feb;51(2):278–284.
- Van Der Wal WM, Noordzij M, Dekker FW, et al. Full loss of residual renal function causes higher mortality in dialysis patients; findings from a marginal structural model. Nephrol Dial Transplant. 2011 Sep;26(9):2978–2983.
- Penne EL, van der Weerd NC, Grooteman MP, et al. Role of residual renal function in phosphate control and anemia management in chronic hemodialysis patients. Clin J Am Soc Nephrol. 2011 Feb;6(2):281–289.
- Wang AY, Sea MM, Ip R, et al. Independent effects of residual renal function and dialysis adequacy on actual dietary protein, calorie, and other nutrient intake in patients on continuous ambulatory peritoneal dialysis. J Am Soc Nephrol. 2001 Nov;12(11):2450–2457.
- Menon MK, Naimark DM, Bargman JM, et al. Long-term blood pressure control in a cohort of peritoneal dialysis patients and its association with residual renal function. Nephrol Dial Transplant. 2001 Nov;16(11):2207–2213.
- Tattersall J. Hemodialysis time and kt/v: less may be better. Semin Dial. 2017 Jan;30(1):10–14.
- Garofalo C, Borrelli S, De Stefano T, et al. Incremental dialysis in ESRD: systematic review and meta-analysis. J Nephrol. 2019 Oct;32(5):823–836.
- Gedney N, Kalantar-Zadeh K. Dialysis patient-centeredness and precision medicine: focus on incremental home hemodialysis and preserving residual kidney function. Semin Nephrol. 2018 Jul;38(4):426–432.
- Murea M, Moossavi S, Garneata L, et al. Narrative review of incremental hemodialysis. Kidney Int Rep. 2020 Feb;5(2):135–148.
- Daugirdas JT, Depner TA, Inrig J, et al. KDOQI clinical practice guideline for hemodialysis adequacy: 2015 update. Am J Kidney Diseases. 2015 November 01 66(5):884–930.
- Vilar E, Wellsted D, Chandna SM, et al. Residual renal function improves outcome in incremental haemodialysis despite reduced dialysis dose. Nephrol Dial Transplant. 2009 Aug;24(8):2502–2510.
- Penne EL, van der Weerd NC, Blankestijn PJ, et al. Role of residual kidney function and convective volume on change in beta2-microglobulin levels in hemodiafiltration patients. Clin J Am Soc Nephrol. 2010 Jan;5(1):80–86.
- Oates T, Pinney JH, Davenport A. Haemodiafiltration versus high-flux haemodialysis: effects on phosphate control and erythropoietin response. Am J Nephrol. 2011;33(1):70–75.
- Vilar E, Boltiador C, Viljoen A, et al. Removal and rebound kinetics of cystatin C in high-flux hemodialysis and hemodiafiltration. Clin J Am Soc Nephrol. 2014 Jul;9(7):1240–1247.
- Casino FG, Basile C. The variable target model: a paradigm shift in the incremental haemodialysis prescription. Nephrol Dial Transplant. 2017 Jan 1; 32(1):182–190.
- Garneata L, Stancu A, Dragomir D, et al. Ketoanalogue-supplemented vegetarian very low-protein diet and CKD progression. J Am Soc Nephrol. 2016 Jul;27(7):2164–2176.
- Garneata L, Mocanu CA, Mocanu AE, et al. Fo012vegetarian severe hypoproteic diet supplemented with keto-analogues for predialysis chronic kidney disease patients: the influence on long term prognosis. Nephrol Dialysis Transplantation. 2019;34(Supplement_1). https://doi.org/10.1093/ndt/gfz096.FO012.
- Depner TA, Daugirdas JT. Equations for normalized protein catabolic rate based on two-point modeling of hemodialysis urea kinetics. J Am Soc Nephrol. 1996 May;7(5):780–785.
- Casino FG, Mostacci SD, and Sabato A, et al. The lacking equation that estimates the protein catabolic rate in patients on once-weekly haemodialysis. J Nephrol. 2020. 34:459–464.
- Schiffl H. High-volume online haemodiafiltration treatment and outcome of end-stage renal disease patients: more than one mode. Int Urol Nephrol. 2020 Aug;52(8):1501–1506.
- Kikuchi K, Hamano T, Wada A, et al. Predilution online hemodiafiltration is associated with improved survival compared with hemodialysis. Kidney Int. 2019 Apr;95(4):929–938.
- Masakane I, Kikuchi K, Kawanishi H. Evidence for the clinical advantages of predilution on-line hemodiafiltration. Contrib Nephrol. 2017;189:17–23.